- 1Department of Industrial, Electronics and Mechanical Engineering, Roma Tre University, Rome, Italy
- 2Department of Engineering, University of Messina, Messina, Italy
- 3Department of Medicine, Epidemiology, Occupational and Environmental Hygiene, National Institute for Insurance Against Accidents at Work (INAIL), Rome, Italy
- 4Department of Medical-Surgical Sciences and Biotechnologies, Sapienza University of Rome, Latina, Italy
- 5Department of Human Neurosciences, Faculty of Medicine and Dentistry, Sapienza University of Rome, Rome, Italy
- 6Department of Systems Medicine and Center of Space Biomedicine, University of Rome Tor Vergata, Rome, Italy
Prosthetic gait implies the use of compensatory motor strategies, including alterations in gait biomechanics and adaptations in the neural control mechanisms adopted by the central nervous system. Despite the constant technological advancements in prostheses design that led to a reduction in compensatory movements and an increased acceptance by the users, a deep comprehension of the numerous factors that influence prosthetic gait is still needed. The quantitative prosthetic gait analysis is an essential step in the development of new and ergonomic devices and to optimize the rehabilitation therapies. Nevertheless, the assessment of prosthetic gait is still carried out by a heterogeneous variety of methodologies, and this limits the comparison of results from different studies, complicating the definition of shared and well-accepted guidelines among clinicians, therapists, physicians, and engineers. This perspective article starts from the results of a project funded by the Italian Worker's Compensation Authority (INAIL) that led to the generation of an extended dataset of measurements involving kinematic, kinetic, and electrophysiological recordings in subjects with different types of amputation and prosthetic components. By encompassing different studies published along the project activities, we discuss the specific information that can be extracted by different kinds of measurements, and we here provide a methodological perspective related to multimodal prosthetic gait assessment, highlighting how, for designing improved prostheses and more effective therapies for patients, it is of critical importance to analyze movement neural control and its mechanical actuation as a whole, without limiting the focus to one specific aspect.
Introduction
The amputation of a lower limb is a complex and invasive surgery that is often needed due to traumatic events, vascular diseases, or tumors. The changes in demographics and the increasing incidence of the pathologies leading to an amputation will potentially impact the healthcare services, including the demand of prosthetic devices, and the number of persons living with the loss of a limb has been estimated to significantly increase in the next few decades (1). People with a lower limb amputation reported a quality of life (QoL) that is significantly lower with respect to the general population. Among others, factors such as the use of a specific prosthesis and close related factors such as residual stump pain and type of suspension were found to predict QoL scores significantly (2–6).
After the surgery, people with amputation must undergo a rehabilitation phase and a considerable walking training (7) to gain the ability to walk autonomously and safely with a prosthetic device (8, 9). People with mono-lateral amputation typically adopt a series of compensatory motor strategies involving the prosthetic side and the contralateral sound limb (10, 11), plus an increased involvement of pelvis and trunk (12–16). As a matter of fact, prosthetic gait reflects a mixture of deviations from normal gait and adaptive and compensatory motions dictated by residual limb functions. From a motor control standpoint, during the rehabilitation process, people with amputation must adapt their walking patterns to their new physical conditions, and this adaptation may result in changes in the way the central nervous system (CNS) controls the movement. Lower limb amputation leads to significant neural reorganization within the CNS, mostly due to the loss of the sensorimotor function caused by amputation (17) and to the new biomechanical condition induced by the type of amputation and by the used prosthetic device. The two factors influencing the gait in people with amputation are the level of the amputation (18, 19) and the type of prostheses (20–27). Regarding the former factor, the gait in people with transfemoral amputation (TFA) seems to be more asymmetric than that in people with transtibial amputation (TTA), with increased compensatory strategies, which, over time, may prove damaging to individuals (28). Concerning the latter factor, in recent years, the prostheses have improved in design, materials, and technology (29–32) to be more effective in terms of efficiency of ambulation, minimization of the asymmetries, and reduction of compensatory movements.
In this scenario, quantifying and characterizing the gait of persons with a prosthesis is an essential element to improve the development of new and ergonomic prosthetic devices, and to optimize the rehabilitation programs (33–36). Nevertheless, the heterogeneous variety of methodologies used to assess prosthetic gait limits the comparison of results from different studies and complicates the definition of shared guidelines. The quantitative prosthetic gait assessment should be focused on indicators of effective and ecological gait, such as the traditional gait parameters, level of gait asymmetries, metabolic consumption, and the amount of compensatory muscle activation, but the assessment of such an heterogeneous scenario requires novel research methodologies (37–41).
From this standpoint, the adoption of a multimodal approach is needed for a proper prosthetic gait evaluation. The project “Modular motor control of the contralateral sound limb in people with lower limb amputation: neuromechanical assessment of the prosthetic components in the control of locomotion,” funded by the Italian Worker's Compensation Authority (INAIL), led to the generation of an extended dataset comprising multimodal measurements involving all the common gait analysis instruments. By discussing the results of different studies published within the project, identifying some peculiarities in the used instrumentation and highlighting the importance of the related indices, we here provide a methodological perspective related to multimodal prosthetic gait assessment.
Starting from the dataset recorded during the project activities in the first section, we then describe the results obtained in five different studies, published along the project activities that analyze specific aspects of gait of people with amputation. Each study yields both direct evidence, coming from the recorded data and based on the specific indices used to quantify the gait performance, and indirect evidence emerging from the interpretation of the results. These direct evidence and indirect interpretations are then summed up and integrated in the final perspective section of the article, where we provide a methodological perspective supporting the importance of multimodal prosthetic gait assessment.
Population, Measurements, and Protocol
The population enrolled during the project activities underwent a typical gait analysis protocol executed with a multimodal set of measurements in terms of instrumentation and variable number of subjects, as reported in Table 1. This perspective article takes into account the results of 5 different studies. All the experiments were carried out at the Rome Branch of Prosthetics Center of INAIL, at the CTO Andrea Alesini hospital of Rome.
In total, 57 recordings from subjects with unilateral TFA and 20 recordings from subjects with unilateral TTA were performed. The subjects with TFA wore three different types of prostheses: mechanical prosthesis (TFAM) and two types of prostheses with microprocessor-controlled knees (MPKs), namely C-Leg (TFAC) and Genium (TFAG) prosthesis (Ottobock, Duderstadt, Germany). All subjects with TFA and TTA were provided with the same type of prosthetic foot (Ossur Variflex, Reykjavik, Iceland), whereas the socket was custom-made and adapted to the single user needs before the gait analysis protocol by an experienced physician. All subjects with lower limb amputation were experienced prosthesis users (i.e., able to walk safely with a prosthetic device for more than 2 years). In addition to the TFA and TTA populations, 40 healthy subjects were recruited as the control group (C), and they were age–sex–speed matched with the amputees group.
Walking tests at a self-selected comfortable speed were performed on a 9-m long walkway instrumented with two force platforms (Kistler9286AA, Winterthur, Switzerland). Control subjects were requested to walk also at a lower speed to match the TFA and TTA groups. A six-infrared camera optoelectronic motion analysis system (SMART-DX 6000 System, BTS, Milan, Italy) was used, with passive spherical markers placed according to a modified Davis' protocol (47). In subjects with TTA and TFA, the amputated limb markers were placed over symmetrical points with respect to the homologous marker's position on the non-amputated limb. Electromyographic (EMG) signals were recorded using a wireless system (FreeEMG 1000 System, BTS, Milan, Italy). Muscle activity was recorded from 12 muscles of the sound side (right side for the controls).
Results
The results coming from the multimodal analysis underlying this paper are briefly reported in Table 2 in terms of their direct and indirect interpretations. The following paragraphs report details on the mentioned studies that will serve as a base for the final perspective about the emergent features of prosthetic gait that are shown by adopting this kind of analytical approach.
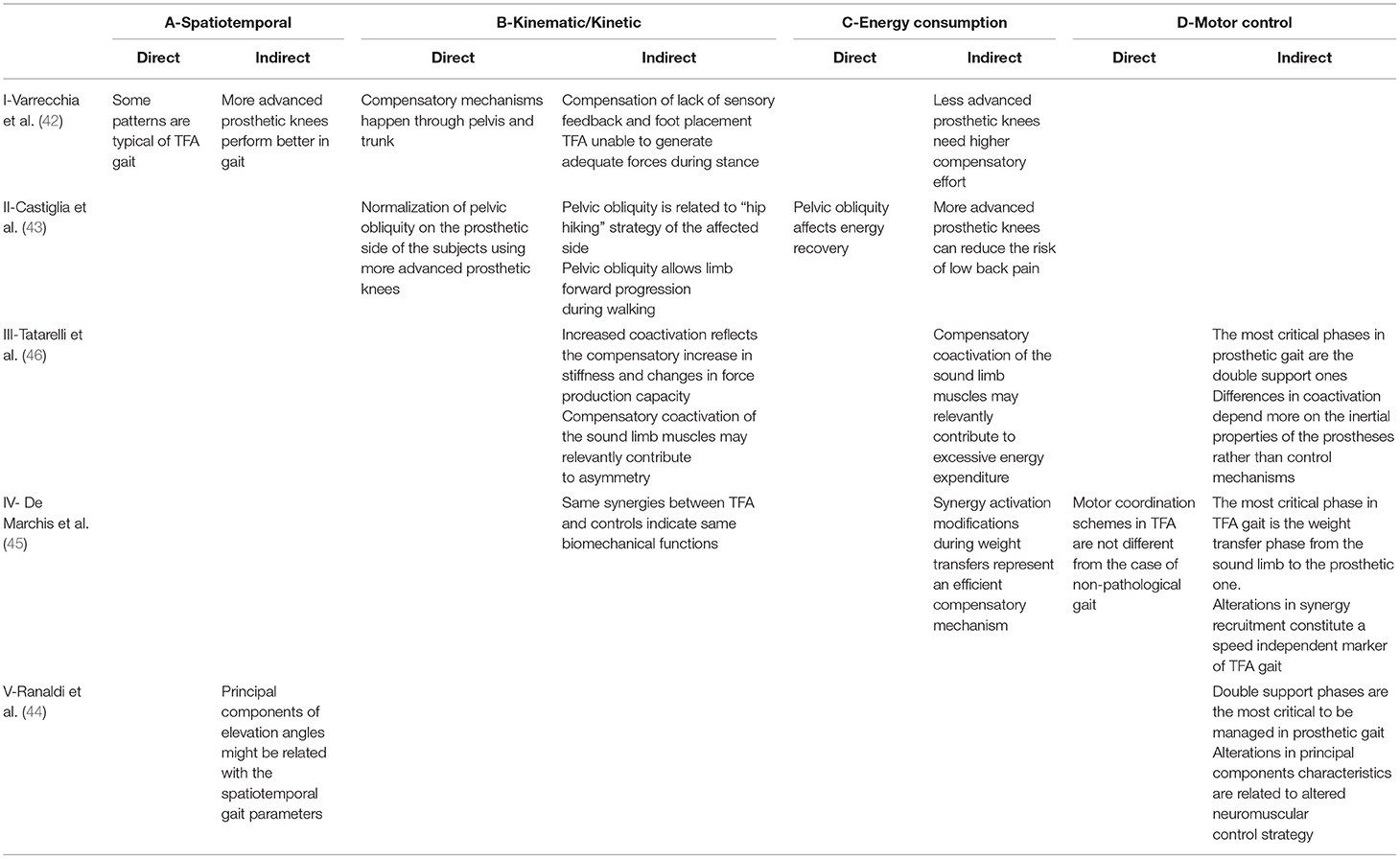
Table 2. Direct and indirect evidence and interpretations emerging from the findings of 5 different studies.
Kinematic, Kinetic, and Energy Consumption Patterns
By including two different amputation levels (i.e., TFA and TTA) and three different types of prostheses for the TFA (i.e., mechanical, C-Leg, and Genium), a comparison of spatiotemporal parameters, plus kinematic and kinetic indicators, as compared to a speed-matched control group, was conducted in Varrecchia et al. (42).
The study highlighted that some patterns characterize prosthetic gait in general, regardless of the type of amputation and the kind of used prosthesis, whereas the others are specific for TFA and are dependent on the type of prosthetic knee (I-ADIR).
From a purely kinematic standpoint, TFA and TTA subjects show an increased step width, step length, and double support duration. These subjects also show an increased pelvic obliquity and a higher range of motion (RoM) in trunk movements when compared with controls, regardless of whether the leading limb was the prosthetic one or the sound one, indicating that most of the compensation happens through the pelvis and trunk (I-BDIR). An increased stance/swing ratio characterizes the sound side. From a kinetic standpoint, the prosthetic gait is characterized by an increased initial peak in the ground reaction force (GRF) on the sound side. All these alterations might be due to a lack of sensory feedback and to an absence of perception regarding foot placement (I-BIND).
However, besides these common alterations in gait patterns, some additional changes characterize the gait of people with TFA. Kinematic alterations include a reduced stance/swing ratio in the prosthetic side and a higher hip and knee RoM in the sound side. Kinetic alterations include an increased initial peak in the GRF on the prosthetic side, suggesting that TFA are not able to generate adequate forces during stance (I-BIND).
When considering the effect of the device, subjects using a Genium prosthesis have a lower pelvic obliquity when compared to TFAM, a higher hip and knee RoM on the prosthetic side and an increased step length when the sound limb leads. This might indicate that more advanced knee prostheses have a general better performance in gait (I-AIND), leading to a reduced compensatory effort (I-CIND).
Since the main alterations are present in the TFA gait, the potentially induced increase in the metabolic consumption could be explained by an energy-related indicator able to discriminate among different types of prostheses, shedding light onto the efficacy of different prosthetic components. Compared with speed-matched healthy controls, subjects with TFA, indeed, are characterized by a lower ability to recover mechanical energy at each walking step, regardless of the type of prostheses. Among the various spatiotemporal and kinematic modifications in the TFA gait, the only variable that is related to the lower energy recovery is the pelvic obliquity on the prosthetic side (43) (II-CDIR). The pelvic obliquity is the rotation of the pelvis around the coronal plane, defined as the angle between the horizontal plane and the mediolateral axis of the pelvis, and its increase has been shown to be a compensation strategy used to propel the limb and recover energy (II-BIND). This parameter not only correlates with relevant energy consumption measurements, but also highlighted that most advanced technological prostheses, such as Genium, likely require less compensation in the pelvic obliquity to recover the same amount of energy at each walking step (II-BDIR), thus potentially reducing the risk of low back pain (II-CIND).
Electrophysiological Features of Prosthetic Gait
Although these considerations allow us to better understand the effect of prosthetic gait on the movement mechanical outcome, yet the causes leading to such modifications can only be estimated, and the underlying changes in the control strategies adopted by the neuromuscular system can be roughly inferred but cannot be quantitatively accessed. A multimuscle activity measurement involving the sound limb has been performed to further advance our comprehension on the underlying neuromuscular strategies, by recording the EMG activity of 12 mono- and bi-articular muscles acting at the ankle, knee, and hip joints.
To better understand the coordination mechanisms of such muscles, a compact indicator, consisting of a time-varying function, has been used to describe the global neuromuscular strategy adopted by a subject in modulating the simultaneous activation/deactivation of many muscles during gait (48). The analysis on a population of TTA, TFA, and controls highlighted that people with amputation had a coactivation profile similar to the control population. However, the prosthetic gait led to an increased level of simultaneous activation during the loading response and push-off phases, whereas this coactivation was decreased during midstance and swing (46). This increased coactivation probably plays a role in the prosthetic gait asymmetry and altered energy consumption (III-CIND). Among people with TFA, the used kind of prosthesis had an effect on the global coactivation, as it resulted lower in C-Leg users when compared with Genium and mechanical prostheses users. In detail, the increased coactivation levels that are recorded during the general prosthetic gait can be seen as a cause for the decreased force generation capacity and as an additional source of asymmetry (III-BIND). Moreover, the same coactivation can also be seen as an important feature of motor control, isolating the double support phases of gait as the most critical for walking with a prosthesis, in which the different inertia properties of a prosthetic leg with respect to the intact limb might play a key role (III-DIND).
Neuromechanics and Motor Control
The aforementioned multi-muscle EMG recording can also take advantage of the nowadays widespread and clinically relevant theory of modularity in motor control (49). By using the synchronous muscle synergy model, it was possible to identify low-dimensional control structures characterizing the muscle coordination of TFA subjects. In De Marchis et al. (45), it was shown that, despite the visible alterations in muscle activity, the complexity in muscle coordination did not change, as the TFA group exhibited 4 modules, which is the same number of muscle synergies typically expressed by control populations (IV-DDIR). When analyzing the spatial structure of these modules (i.e., the groups of muscles working synergistically), it was shown that it is shared between TFA and controls, consisting of a weight acceptance module at sound limb heel strike, a propulsion module before toe-off, a swing module, and a late swing deceleration module before heel strike. This indicates that the main underlying biomechanical functions were preserved (IV-BIND). However, the difference between TFA and controls was clearly visible in the activation of two out of the four identified modules: a significantly prolonged activation of the propulsion module (calf muscles) and an abnormal activation of the late swing deceleration module (hamstring muscles) during the second double support phase with respect to speed-matched controls (IV-DIND). This result indicates that the most critical phase in gait of people with TFA is the second double support phase, corresponding to the weight transfer from the sound limb to the prosthetic one (IV-DIND), potentially reflecting an efficient compensatory mechanism that enforces the interpretation of the results on the coactivation strategies (IV-CIND).
Analogous results can be obtained by exploiting the planar covariation law of elevation angles. Following the same rationale adopted for the muscle synergy analysis, it is possible to define a common spatial organization for the behavior of the elevation angles of the three lower limb segments (50). With this description, both limbs of the patients and healthy subjects share the same covariation domain, with differences that are limited to the trajectories of the three angles in this space (44) (V-DIND). Coherently with all the other studies presented before, most of the differences are to be ascribed to the management of the body weight and on the contact phase of the limb with the ground (i.e., the stance phase), with the prosthetic limb showing a higher degree of correlation among the three leg segments, as a direct consequence of the control mechanisms of the prosthetic knee (V-DIND). Moreover, the planar covariation law of elevation angles is a compact description that directly approximates the kinematics of the two legs; as a consequence, it is ideally possible to exploit this economic description of gait for predicting different quantitative measures of walking behavior, such as the spatiotemporal parameters, giving rise to a variety of applications for prosthetic control and rehabilitation (V-AIND).
Discussion
Previous studies have highlighted some important features of prosthetic gait, including stability, spatiotemporal gait parameters, and symmetry, gathering relevant information by using a reduced set of sensors (51–57). However, due to the heterogeneous nature of the prosthetic components, type of rehabilitation, experience with prosthesis use, and the amputation characteristics themselves, a multimodal approach to such gait analysis could be able to shed light on some important features related to these multiple factors (38, 58, 59), thus supporting the clinical practice (60–62). The neuromechanical analysis of gait, bringing together the information on biomechanical aspects and neural control aspects, takes advantage of this multimodal approach. This adds to the lack of studies including an EMG analysis of the contralateral limb, which provides a powerful insight into how the CNS is adapting to walking with a prosthesis (63–65), in addition to the changes appearing at the level of the residual musculature (66, 67). Overall, kinematic, kinetic, and surface EMG gait findings reflect the compensatory efforts developed by people with amputation to protect the soft tissues of the prosthetic limb and to deal with the new prosthetic limb condition.
Within the framework of the INAIL-funded project activities, the aim of this perspective is to fill the gap between the complexity of prosthetic gait and the necessity of a complete set of measurements. The summary of the discussions of the outcomes of the project presented here, reported in Table 2, highlights how different analyses can yield a wide overview of the characteristics of the prosthetic gait, reducing the number of indirect considerations (i.e., speculations) that are needed to describe all the factors starting from the results of an incomplete set of analyses. Table 2 reports, for each of the five studies, both the direct evidence, as obtained from the analysis of specific quantities of prosthetic gait, and the indirect interpretations related to aspects that are not explicitly considered in the analysis. These are discussed in the following sections.
Spatiotemporal, Kinematic, Kinetic Metabolic, and Motor Control Aspects of Prosthetic Gait
From a spatiotemporal parameters point of view, a typical gait analysis can be combined with the analysis of the coordination of the lower limb segments, linking concepts related to the biomechanics to motor control investigations. Although the analysis of the spatiotemporal parameters of gait is well-established in the scientific literature, it leads to results that are not directly linked to how the movement is controlled, and thus might fail in identifying the key adaptation mechanisms that underlie walking with a prosthesis and the variables relevant to patient's satisfaction (68). In this scenario, the methodological approaches from I (42) and V (44) could take a strong advantage from a joint analysis, with important implications in engineering (e.g., development of advanced control systems for prostheses based on the underlying motor control mechanisms) and clinical practice (e.g., the use of motor control theories as a benchmark for functional gait recovery).
When dealing with kinematic and kinetic analysis of gait, the multimodal approach is of critical importance for a correct interpretation of the results. This happens with the interpretation of the results of both standard gait and coactivation analyses, from which it is possible to only indirectly suppose that prosthetic gait is characterized by a lower capacity of force generation, whereas the combination of the two studies reinforces this hypothesis. These considerations suggest that the methodologies of I (42) and III (46) have an important complementary role in the assessment of prosthetic gait dynamics.
Moreover, the synergy analysis confirms that the same biomechanical functions of physiological gait are preserved but controlled with different timings; by combining these results with those on pelvic obliquity and general kinematics, it is possible to understand how the abnormal activations in the synergy profiles reflect on the altered movement biomechanics. In the same manner, both the discussions about motor control and energy consumption can be summarized by focusing on the difficulties and asymmetries in the management of the weight shift phases (at the beginning and the end of the stance phase of both legs); this, combined with the results on the pelvic obliquity and with the characterization of the energetic inefficiency, confirms in a quantitative way the already published results, that identify the double support phases of prosthetic gait as the most critical, both from a stability and an energetic point of view. These considerations highlight the importance of connecting the multi-muscle EMG measurement and synergy analysis of IV (45) with the methodologies used in II (43) related to the body center of mass and to pelvic kinematics, for a complete understanding of the interplay between compensation mechanisms and energy consumption.
Perspective of the Multimodal Analysis of Prosthetic Gait
Future studies should explore whether the adoption of a multimodal approach can capture the alterations in performance-based walking measures (69), the metabolic cost of walking (32, 70), self-perceived mobility and balance outcomes (37, 71), and the acceptance of prostheses (3). In the clinical practice, the outcomes of the rehabilitation therapies are often measured by means of qualitative scales, such as the K-Level, which to date is the main scale used by physicians to choose the most adequate prosthetic device. Some semi-quantitative scales have also been proposed, like the Amputee Mobility Predictor (AMP) scale (72), in which some spatiotemporal parameters of gait are used to refine the information provided by the K-level classification. In addition to these indices, several clinical scales describing the patient's QoL are adopted as a description of the follow-up of the therapies, such as the Amputee Activity Survey or the 12-min walking test (73). In general, all the current clinical scales are pseudo-subjective, based on questionnaires that are dependent on the personal perception of either the physician or the patient. Nevertheless, they might fail in providing insight into the interplay between the prosthesis and the patient so that the adopted solutions might not be always optimal. Although it remains still feasible to use those measures in the clinical practice, the recent advancement in prosthesis technology could make the patients reach high scores for most of these scales. In this sense, the definition of more objective scales, exploiting engineering tools, can help in reaching a higher level of understanding of how the choices of the prosthetic component affect the movement control; this can lead to the development of prosthetic devices that reach a higher degree of integration with the subject's motor control strategies.
Conclusions
The meta-discussion presented here was elicited by the heterogeneous framework of results obtained within the project “Modular motor control of the contralateral sound limb in people with lower limb amputation: neuromechanical assessment of the prosthetic components in the control of locomotion” funded by INAIL. All the considerations from the related studies strongly highlight the importance of applying a multimodal approach when analyzing gait in people with a lower limb amputation; as a matter of fact, despite the huge scientific effort of the last two decades, this condition is still partially unknown to date, and the compensations that are necessary for reaching stable gait with a prosthesis are highly complex and cannot be characterized as a whole without a complete recording and analysis of all the influence factors. Consequently, this strongly recommends that, for designing improved prosthetic device, develop more advanced and physiologically inspired prosthesis control systems, and plan more effective therapies for these patients, it is of critical importance to analyze movement neural control and mechanical actuation as a whole, without limiting the focus to one specific aspect.
Data Availability Statement
The original contributions presented in the study are included in the article/supplementary material, further inquiries can be directed to the corresponding author.
Ethics Statement
Ethical approval was not provided for this study on human participants because it analyses previously published results. Details on ethics can be found in the original referenced articles. The patients/participants provided their written informed consent to participate in this study.
Author Contributions
CD, SR, and SFC conceptualized the study. CD and SR prepared the original draft of the manuscript. SC, AR, MS, FD, and FL provided resources and administered the project. All authors reviewed and edited the manuscript for important intellectual content.
Funding
This research was funded by INAIL, Bando Ricerche in Collaborazione 2016.
Conflict of Interest
The authors declare that the research was conducted in the absence of any commercial or financial relationships that could be construed as a potential conflict of interest.
Publisher's Note
All claims expressed in this article are solely those of the authors and do not necessarily represent those of their affiliated organizations, or those of the publisher, the editors and the reviewers. Any product that may be evaluated in this article, or claim that may be made by its manufacturer, is not guaranteed or endorsed by the publisher.
References
1. Ziegler-Graham K, MacKenzie EJ, Ephraim PL, Travison TG, Brookmeyer R. Estimating the prevalence of limb loss in the United States: 2005 to 2050. Arch Phys Med Rehabil. (2008) 89:422–9. doi: 10.1016/j.apmr.2007.11.005
2. Sinha R, van den Heuvel WJ, Arokiasamy P. Factors affecting quality of life in lower limb amputees. Prosthet Orthot Int. (2011) 35:90–6. doi: 10.1177/0309364610397087
3. Gholizadeh H, Osman NAA, Eshraghi A, Ali S. Transfemoral prosthesis suspension systems: a systematic review of the literature. Am J Phys Med Rehabil. (2014) 93:809–23. doi: 10.1097/PHM.0000000000000094
4. Highsmith MJ, Kahle JT, Miro RM, Lura DJ, Dubey RV, Carey SL, et al. Perceived differences between the genium and the C-Leg microprocessor prosthetic knees in prosthetic-related function and quality of life. Technol Innov. (2014) 15:369–75. doi: 10.3727/194982413X13844489091297
5. Davie-Smith F, Carse B. Comparison of patient-reported and functional outcomes following transition from mechanical to microprocessor knee in the low-activity user with a unilateral transfemoral amputation. Prosthet Orthot Int. (2021) 45:198–204. doi: 10.1097/PXR.0000000000000017
6. Sen EI, Aydin T, Bugdayci D, Kesiktaş FN. Effects of microprocessor-controlled prosthetic knees on self-reported mobility, quality of life, and psychological states in patients with transfemoral amputations. Acta Orthop Traumatol Turc. (2020) 54:502–6. doi: 10.5152/j.aott.2020.19269
7. Hebenton J, Scott H, Seenan C, Davie-Smith F. Relationship between models of care and key rehabilitation milestones following unilateral transtibial amputation: a national cross-sectional study. Physiotherapy. (2019) 105:476–82. doi: 10.1016/j.physio.2018.11.307
8. Ülger Ö, Yildirim Sahan T, Çelik SE. A systematic literature review of physiotherapy and rehabilitation approaches to lower-limb amputation. Physiother Theory Pract. (2018) 34:821–34. doi: 10.1080/09593985.2018.1425938
9. Fajardo-Martos I, Roda O, Zambudio-Periago R, Bueno-Cavanillas A, Hita-Contreras F, Sánchez-Montesinos I. Predicting successful prosthetic rehabilitation in major lower-limb amputation patients: a 15-year retrospective cohort study. Braz J Phys Ther. (2018) 22:205–14. doi: 10.1016/j.bjpt.2017.08.002
10. Prinsen EC, Nederhand MJ, Rietman JS. Adaptation strategies of the lower extremities of patients with a transtibial or transfemoral amputation during level walking: a systematic review. Arch Phys Med Rehabil. (2011) 92:1311–25. doi: 10.1016/j.apmr.2011.01.017
11. Hisano G, Hashizume S, Kobayashi T, Major MJ, Nakashima M, Hobara H. Unilateral above-knee amputees achieve symmetric mediolateral ground reaction impulse in walking using an asymmetric gait strategy. J Biomech. (2021) 115:110201. doi: 10.1016/j.jbiomech.2020.110201
12. Devan H, Carman A, Hendrick P, Hale L, Ribeiro DC. Spinal, pelvic, and hip movement asymmetries in people with lower-limb amputation: systematic review. J Rehabil Res Dev. (2015) 52:1–20. doi: 10.1682/JRRD.2014.05.0135
13. Goujon-Pillet H, Sapin E, Fodé P, Lavaste F. Three-dimensional motions of trunk and pelvis during transfemoral amputee gait. Arch Phys Med Rehabil. (2008) 89:87–94. doi: 10.1016/j.apmr.2007.08.136
14. Hendershot BD, Bazrgari B, Nussbaum MA. Persons with unilateral lower-limb amputation have altered and asymmetric trunk mechanical and neuromuscular behaviors estimated using multidirectional trunk perturbations. J Biomech. (2013) 46:1907–12. doi: 10.1016/j.jbiomech.2013.04.018
15. Gaffney BM, Murray AM, Christiansen CL, Davidson BS. Identification of trunk and pelvis movement compensations in patients with transtibial amputation using angular momentum separation. Gait Posture. (2016) 45:151–6. doi: 10.1016/j.gaitpost.2016.01.015
16. Butowicz CM, Krupenevich RL, Acasio JC, Dearth CL, Hendershot BD. Relationships between mediolateral trunk-pelvic motion, hip strength, and knee joint moments during gait among persons with lower limb amputation. Clin Biomech. (2020) 71:160–6. doi: 10.1016/j.clinbiomech.2019.11.009
17. Chen R, Corwell B, Yaseen Z, Hallett M, Cohen LG. Mechanisms of cortical reorganization in lower-limb amputees. J Neurosci. (1998) 18:3443–50. doi: 10.1523/JNEUROSCI.18-09-03443.1998
18. Baum BS, Schnall BL, Tis JE, Lipton JS. Correlation of residual limb length and gait parameters in amputees. Injury. (2008) 39:728–33. doi: 10.1016/j.injury.2007.11.021
19. Bell JC, Wolf EJ, Schnall BL, Tis JE, Tis LL, Potter MBK. Transfemoral amputations: the effect of residual limb length and orientation on gait analysis outcome measures. JBJS. (2013) 95:408–14. doi: 10.2106/JBJS.K.01446
20. Kannenberg A, Zacharias B, Pröbsting E. Benefits of microprocessor-controlled prosthetic knees to limited community ambulators: systematic review. J Rehabil Res Dev. (2014) 51:1469. doi: 10.1682/JRRD.2014.05.0118
21. Segal AD, Orendurff MS, Klute GK, McDowell ML. Kinematic and kinetic comparisons of transfemoral amputee gait using C-Leg® and Mauch SNS® prosthetic knees. J Rehabil Res Dev. (2006) 43:857. doi: 10.1682/JRRD.2005.09.0147
22. Highsmith MJ, Kahle JT, Miro RM, Cress ME, Lura DJ, Quillen WS, et al. Functional performance differences between the Genium and C-Leg prosthetic knees and intact knees. J Rehabil Res Dev. (2016) 53:753. doi: 10.1682/JRRD.2014.06.0149
23. Kahle JT, Highsmith MJ, Hubbard SL. Comparison of nonmicroprocessor knee mechanism versus C-Leg on prosthesis evaluation questionnaire, stumbles, falls, walking tests, stair descent, and knee preference. J Rehabil Res Dev. (2008) 45:1–14. doi: 10.1682/JRRD.2007.04.0054
24. Thiele J, Westebbe B, Bellmann M, Kraft M. Designs and performance of microprocessor-controlled knee joints. Biomed Tech. (2014) 59:65–77. doi: 10.1515/bmt-2013-0069
25. Kaufman KR, Bernhardt KA, Symms K. Functional assessment and satisfaction of transfemoral amputees with low mobility (FASTK2): a clinical trial of microprocessor-controlled vs. non-microprocessor-controlled knees. Clin Biomech. (2018) 58:116–22. doi: 10.1016/j.clinbiomech.2018.07.012
26. Uchytil J, Jandacka D, Zahradnik D, Farana R, Janura M. Temporal–spatial parameters of gait in transfemoral amputees: comparison of bionic and mechanically passive knee joints. Prosthet Orthot Int. (2014) 38:199–203. doi: 10.1177/0309364613492789
27. Mileusnic MP, Rettinger L, Highsmith MJ, Hahn A. Benefits of the Genium microprocessor controlled prosthetic knee on ambulation, mobility, activities of daily living and quality of life: a systematic literature review. Disabil Rehabil Assist Technol. (2021) 16:453–64. doi: 10.1080/17483107.2019.1648570
28. Sivapuratharasu B, Bull AM, McGregor AH. Understanding low back pain in traumatic lower limb amputees: a systematic review. Arch Rehabil Res Clin Transl. (2019) 1:100007. doi: 10.1016/j.arrct.2019.100007
29. Chitragari G, Mahler DB, Sumpio BJ, Blume PA, Sumpio BE. Prosthetic options available for the diabetic lower limb amputee. Clin Podiatr Med Surg. (2014) 31:174–85. doi: 10.1016/j.cpm.2013.09.008
30. Ingraham KA, Fey NP, Simon AM, Hargrove LJ. Assessing the relative contributions of active ankle and knee assistance to the walking mechanics of transfemoral amputees using a powered prosthesis. PLoS ONE. (2016) 11:e0147661. doi: 10.1371/journal.pone.0147661
31. Shell CE, Segal AD, Klute GK, Neptune RR. The effects of prosthetic foot stiffness on transtibial amputee walking mechanics and balance control during turning. Clin Biomech. (2017) 49:56–63. doi: 10.1016/j.clinbiomech.2017.08.003
32. Li S, Cao W, Yu H, Meng Q, Chen W. Physiological parameters analysis of transfemoral amputees with different prosthetic knees. Acta Bioeng Biomech. (2019) 21:135–42. doi: 10.5277/ABB-01321-2019-02
33. Schafer ZA, Perry JL, Vanicek N. A personalised exercise programme for individuals with lower limb amputation reduces falls and improves gait biomechanics: a block randomised controlled trial. Gait Posture. (2018) 63:282–9. doi: 10.1016/j.gaitpost.2018.04.030
34. Crenshaw JR, Kaufman KR, Grabiner MD. Compensatory-step training of healthy, mobile people with unilateral, transfemoral or knee disarticulation amputations: a potential intervention for trip-related falls. Gait Posture. (2013) 38:500–6. doi: 10.1016/j.gaitpost.2013.01.023
35. Major MJ, Fey NP. Considering passive mechanical properties and patient user motor performance in lower limb prosthesis design optimization to enhance rehabilitation outcomes. Phys Ther Rev. (2017) 22:202–16. doi: 10.1080/10833196.2017.1346033
36. Mohanty RK, Mohanty RC, Sabut SK. A systematic review on design technology and application of polycentric prosthetic knee in amputee rehabilitation. Phys Eng Sci Med. (2020) 43:781–98. doi: 10.1007/s13246-020-00882-3
37. Czerniecki JM, Morgenroth DC. Metabolic energy expenditure of ambulation in lower extremity amputees: what have we learned and what are the next steps?. Disabil Rehabil. (2017) 39:143–51. doi: 10.3109/09638288.2015.1095948
38. Price MA, Beckerle P, Sup FC. Design optimization in lower limb prostheses: a review. IEEE Trans Neural Syst Rehabil Eng. (2019) 27:1574–88. doi: 10.1109/TNSRE.2019.2927094
39. Ghillebert J, De Bock S, Flynn L, Geeroms J, Tassignon B, Roelands B, et al. Guidelines and recommendations to investigate the efficacy of a lower-limb prosthetic device: a systematic review. IEEE Trans Med Robot Bionics. (2019) 1:279–96. doi: 10.1109/TMRB.2019.2949855
40. Gouelle A, Highsmith MJ. Instrumented four square step test in adults with transfemoral amputation: test-retest reliability and discriminant validity between two types of microprocessor knees. Sensors. (2020) 20:4782. doi: 10.3390/s20174782
41. Cutti AG, Verni G, Migliore GL, Amoresano A, Raggi M. Reference values for gait temporal and loading symmetry of lower-limb amputees can help in refocusing rehabilitation targets. J Neuroeng Rehabil. (2018) 15:1–12. doi: 10.1186/s12984-018-0403-x
42. Varrecchia T, Serrao M, Rinaldi M, Ranavolo A, Conforto S, De Marchis C, et al. Common and specific gait patterns in people with varying anatomical levels of lower limb amputation and different prosthetic components. Hum Mov Sci. (2019) 66:9–21. doi: 10.1016/j.humov.2019.03.008
43. Castiglia SF, Ranavolo A, Varrecchia T, De Marchis C, Tatarelli A, Magnifica F, et al. Pelvic obliquity as a compensatory mechanism leading to lower energy recovery: characterization among the types of prostheses in subjects with transfemoral amputation. Gait Posture. (2020) 80:280–4. doi: 10.1016/j.gaitpost.2020.06.013
44. Ranaldi S, De Marchis C, Serrao M, Ranavolo A, Draicchio F, Lacquaniti F, et al. Modular control of kinematics in prosthetic gait: low-dimensional description based on the planar covariation law. In: European Medical and Biological Engineering Conference. Cham: Springer (2020). p. 833–9.
45. De Marchis C, Ranaldi S, Serrao M, Ranavolo A, Draicchio F, Lacquaniti F, et al. Modular motor control of the sound limb in gait of people with trans-femoral amputation. J Neuroeng Rehabil. (2019) 16:1–11. doi: 10.1186/s12984-019-0616-7
46. Tatarelli A, Serrao M, Varrecchia T, Fiori L, Draicchio F, Silvetti A, et al. Global muscle coactivation of the sound limb in gait of people with transfemoral and transtibial amputation. Sensors. (2020) 20:2543. doi: 10.3390/s20092543
47. Davis III RB, Ounpuu S, Tyburski D, Gage JR. A gait analysis data collection and reduction technique. Hum Mov Sci. (1991) 10:575–87. doi: 10.1016/0167-9457(91)90046-Z
48. Ranavolo A, Mari S, Conte C, Serrao M, Silvetti A, Iavicoli S, et al. A new muscle co-activation index for biomechanical load evaluation in work activities. Ergonomics. (2015) 58:966–79. doi: 10.1080/00140139.2014.991764
49. Ting LH, Chiel HJ, Trumbower RD, Allen JL, McKay JL, Hackney ME, et al. Neuromechanical principles underlying movement modularity and their implications for rehabilitation. Neuron. (2015) 86:38–54. doi: 10.1016/j.neuron.2015.02.042
50. Ivanenko YP, Cappellini G, Dominici N, Poppele RE, Lacquaniti F. Modular control of limb movements during human locomotion. J Neurosci. (2007) 27:11149–61. doi: 10.1523/JNEUROSCI.2644-07.2007
51. Howcroft J, Lemaire ED, Kofman J, Kendell C. Understanding dynamic stability from pelvis accelerometer data and the relationship to balance and mobility in transtibial amputees. Gait Posture. (2015) 41:808–12. doi: 10.1016/j.gaitpost.2015.03.001
52. Tura A, Raggi M, Rocchi L, Cutti AG, Chiari L. Gait symmetry and regularity in transfemoral amputees assessed by trunk accelerations. J Neuroeng Rehabil. (2010) 7:1–10. doi: 10.1186/1743-0003-7-4
53. Iosa M, Paradisi F, Brunelli S, Delussu AS, Pellegrini R, Zenardi D, et al. Assessment of gait stability, harmony, and symmetry in subjects with lower-limb amputation evaluated by trunk accelerations. J Rehabil Res Dev. (2014) 51:623–34. doi: 10.1682/JRRD.2013.07.0162
54. Clemens S, Kim KJ, Gailey R, Kirk-Sanchez N, Kristal A, Gaunaurd I. Inertial sensor-based measures of gait symmetry and repeatability in people with unilateral lower limb amputation. Clin Biomech. (2020) 72:102–7. doi: 10.1016/j.clinbiomech.2019.12.007
55. Bastas G, Fleck JJ, Peters RA, Zelik KE. IMU-based gait analysis in lower limb prosthesis users: Comparison of step demarcation algorithms. Gait Posture. (2018) 64:30–7. doi: 10.1016/j.gaitpost.2018.05.025
56. Miller KT, Russell M, Jenks T, Surratt K, Poretti K, Eigenbrot SS, et al. The feasibility and validity of a wearable sensor system to assess the stability of high-functioning lower-limb prosthesis users. J Prosthet Orthot. (2020). doi: 10.1097/JPO.0000000000000332
57. Lansade C, Bonnet X, Marvisi N, Facione J, Villa C, Pillet H. Estimation of the body center of mass velocity during gait of people with transfemoral amputation from force plate data integration. Clin Biomech. (2021) 88:105423. doi: 10.1016/j.clinbiomech.2021.105423
58. Esquenazi A. Gait analysis in lower-limb amputation and prosthetic rehabilitation. Phys Med Rehabil Clin N Am. (2014) 25:153–67. doi: 10.1016/j.pmr.2013.09.006
59. Wanamaker AB, Andridge RR, Chaudhari AM. When to biomechanically examine a lower-limb amputee: a systematic review of accommodation times. Prosthet Orthot Int. (2017) 41:431–45. doi: 10.1177/0309364616682385
60. Czerniecki JM, Gitter AJ. Gait analysis in the amputee: has it helped the amputee or contributed to the development of improved prosthetic components?. Gait Posture. (1996) 4:258–68. doi: 10.1016/0966-6362(96)01073-9
61. Sagawa Jr Y, Turcot K, Armand S, Thevenon A, Vuillerme N, Watelain E. Biomechanics and physiological parameters during gait in lower-limb amputees: a systematic review. Gait Posture. (2011) 33:511–26. doi: 10.1016/j.gaitpost.2011.02.003
62. Gard SA. Use of quantitative gait analysis for the evaluation of prosthetic walking performance. J Prosthet Orthot. (2006) 18:P93–104. doi: 10.1097/00008526-200601001-00011
63. Wentink EC, Prinsen EC, Rietman JS, Veltink PH. Comparison of muscle activity patterns of transfemoral amputees and control subjects during walking. J Neuroeng Rehabil. (2013) 10:1–11. doi: 10.1186/1743-0003-10-87
64. Fey NP, Silverman AK, Neptune RR. The influence of increasing steady-state walking speed on muscle activity in below-knee amputees. J Electromyogr Kinesiol. (2010) 20:155–61. doi: 10.1016/j.jelekin.2009.02.004
65. Mehryar P, Shourijeh MS, Rezaeian T, Khandan AR, Messenger N, O'Connor R, et al. Muscular activity comparison between non-amputees and transfemoral amputees during normal transient-state walking speed. Med Eng Phys. (2021) 95:39–44. doi: 10.1016/j.medengphy.2021.07.004
66. Huang S, Ferris DP. Muscle activation patterns during walking from transtibial amputees recorded within the residual limb-prosthetic interface. J Neuroeng Rehabil. (2012) 9:1–16. doi: 10.1186/1743-0003-9-55
67. Pantall A, Ewins D. Muscle activity during stance phase of walking: comparison of males with transfemoral amputation with osseointegrated fixations to nondisabled male volunteers. J Rehabil Res Dev. (2013) 50:499–514. doi: 10.1682/JRRD.2011.10.0204
68. Kark L, Simmons A. Patient satisfaction following lower-limb amputation: the role of gait deviation. Prosthet Orthot Int. (2011) 35:225–33. doi: 10.1177/0309364611406169
69. Seth M, Coyle PC, Pohlig RT, Beisheim EH, Horne JR, Hicks GE, et al. Gait asymmetry is associated with performance-based physical function among adults with lower-limb amputation. Physiother Theory Pract. (2021) 1–11. doi: 10.1080/09593985.2021.1990449
70. Highsmith MJ, Kahle JT, Bongiorni DR, Sutton BS, Groer S, Kaufman KR. Safety, energy efficiency, and cost efficacy of the C-Leg for transfemoral amputees: a review of the literature. Prosthet Orthot Int. (2010) 34:362–77. doi: 10.3109/03093646.2010.520054
71. Miller WC, Deathe AB, Speechley M. Psychometric properties of the activities-specific balance confidence scale among individuals with a lower-limb amputation. Arch Phys Med Rehabil. (2003) 84:656–61. doi: 10.1016/S0003-9993(02)04807-4
72. Gailey RS, Roach KE, Applegate EB, Cho B, Cunniffe B, Licht S, et al. The amputee mobility predictor: an instrument to assess determinants of the lower-limb amputee's ability to ambulate. Arch Phys Med Rehabil. (2002) 83:613–27. doi: 10.1053/apmr.2002.32309
Keywords: gait analysis, neuromechanics, prostheses, multimodal characterization, electromyograhy (EMG), muscle synergies, lower limb amputation
Citation: De Marchis C, Ranaldi S, Varrecchia T, Serrao M, Castiglia SF, Tatarelli A, Ranavolo A, Draicchio F, Lacquaniti F and Conforto S (2022) Characterizing the Gait of People With Different Types of Amputation and Prosthetic Components Through Multimodal Measurements: A Methodological Perspective. Front. Rehabilit. Sci. 3:804746. doi: 10.3389/fresc.2022.804746
Received: 29 October 2021; Accepted: 03 February 2022;
Published: 17 March 2022.
Edited by:
Areerat Suputtitada, Chulalongkorn University, ThailandReviewed by:
Timothy Hasenoehrl, Medical University of Vienna, AustriaJan Veneman, Hocoma, Switzerland
Copyright © 2022 De Marchis, Ranaldi, Varrecchia, Serrao, Castiglia, Tatarelli, Ranavolo, Draicchio, Lacquaniti and Conforto. This is an open-access article distributed under the terms of the Creative Commons Attribution License (CC BY). The use, distribution or reproduction in other forums is permitted, provided the original author(s) and the copyright owner(s) are credited and that the original publication in this journal is cited, in accordance with accepted academic practice. No use, distribution or reproduction is permitted which does not comply with these terms.
*Correspondence: Cristiano De Marchis, Y3Jpc3RpYW5vLmRlbWFyY2hpc0B1bmlyb21hMy5pdA==
†These authors have contributed equally to this work