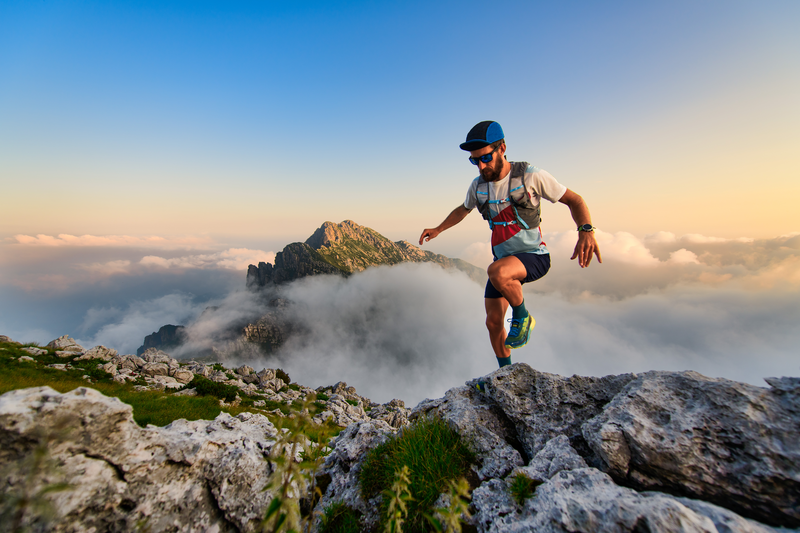
94% of researchers rate our articles as excellent or good
Learn more about the work of our research integrity team to safeguard the quality of each article we publish.
Find out more
ORIGINAL RESEARCH article
Front. Rehabil. Sci. , 08 November 2021
Sec. Rehabilitation for Musculoskeletal Conditions
Volume 2 - 2021 | https://doi.org/10.3389/fresc.2021.764686
This article is part of the Research Topic Exercise and Aging with Musculoskeletal Conditions View all 6 articles
Introduction: Growing research suggests that aerobic high-intensity interval training (HIIT) improves cardiovascular function and physical performance compared with moderate intensity continuous training (MICT). However relatively few animal models of HIIT are available to inform about the benefits of this exercise—particularly among older animals. In addition, there is little evidence for how HIIT training interacts with adjuvant pharmacological therapies known to enhance the impact of MCIT in older individuals such as Angiotensin Converting Enzyme (ACE) Inhibitors.
Purpose: The aim of the present study was to establish a HIIT protocol in aged rats based on forced running wheel-bed, and to subsequently (1) establish the feasibility of the HIIT protocol in a proof-of-concept study evaluating interactions between HIIT and (2) the result of combining HIIT + ACE inhibitor treatment using the ACE inhibitor enalapril.
Methods: Two groups of rats were used in this study. The feasibility of using wheel-bed for HIIT training was tested in group one (15- and 30-month-old male rats). In the second group, 37 24-month-old Fisher 344 x Brown Norway male rats were randomly divided into four subgroups: control, enalapril, HIIT training group, and HIIT training combined with enalapril administration. The training and administration lasted for 4 weeks. After the intervention, locomotor activity, exercise tolerance, and grip strength were tested.
Results: Our feasibility study suggested that middle-aged and aged rats were able to successfully complete the HIIT training. In our intervention study, HIIT training alone, regardless of adjuvant enalapril intervention, did raise treadmill exercise tolerance vs. the sedentary condition. Measures of healthspan were not negatively impacted by HIIT training.
Conclusion: The novel HIIT protocol based on forced running wheel-bed was successfully employed in aged rats. We conclude that future studies should compare the results and of multi-modal intervention strategies which include both HIIT and MICT in combination with adjuvant therapies such as enalapril to improve exercise tolerance and other global indices of healthspan.
High-intensity interval training (HIIT) is described as high-intensity exercise with intervals that are characterized by repeated sessions of high-intensity exercise period followed by rest or low-intensity exercise for recovery (1–3). For years, moderate-intensity continuous training (MICT) has been the most preferred exercise modality for improving body composition and cardiorespiratory fitness (4). Long-term devotion to this training method has however been low as many people stop exercising as a result of lack of time (4, 5). In comparison, HIIT is undoubtedly a time-efficient strategy to increase metabolism and enhance skeletal muscle capacity (3, 6). HIIT is also less monotonous, thereby increasing participation and adherence (7, 8). Furthermore, aerobically-based HIIT has been reported to extensively improve cardiorespiratory fitness—a strong determinant of morbidity and mortality (5)—and to stimulate an increase of serum brain-derived neurotrophic factor (BDNF) concentrations—a critical indicator for mental health (9). Additionally, aerobic HIIT leads to mitochondrial biogenesis and induces multiple factors involved in tissue regeneration (10, 11).
For decades, HIIT was primarily used in athletic practice for runners and swimmers (12). More recently, HIIT gained much attention for not only athletes but also as an effective exercise module in healthy adults in maintaining or enhancing body composition, body weight control and disease rehabilitation (5, 13). However, most of these studies were carried out with young adults. Fewer studies have been done on older adults, especially healthy older adults. Preliminary studies have demonstrated the low risk and feasibility of HIIT in older adults. High-intensity exercise training program has shown well-tolerance in individuals of 45–80 years old with Parkinson's disease (95% adherence) (14).
Using animal models in exercise research has long provided insights into the physiologic adaptations to exercise. Recently, animal models of HIIT have been developed, exclusively using mice as a model (15). However, as with any model, mice have certain disadvantages—particularly for studying aging. For instance, the advantage of using a rat model in comparison to a mice model lies in the ease of sample collection and the quantity of samples that can be collected (16). In exercise physiology and behavior studies, rats showed less data variability and are more repeatable (16). Therefore, the rat appears to be an important animal model in exercise-related research. Indeed, the rat is the preclinical model of choice for the Molecular Transducers of Activity Consortium (MoTrPAC), a national research consortium designed to discover and perform preliminary characterization of the range of molecular transducers (the “molecular map”) that underlie the effects of physical activity in humans. However, to our knowledge, the MoTrPAC group is currently not exploring the use of HIIT. The purpose of this study is to develop a rat HIIT protocol based on the forced running wheel-bed.
Presently, the treadmill is the most preferred apparatus for HIIT training in rats (17–19). However, a main limitation of treadmill training lies in that most treadmill systems utilize electrical shock or aversive air-puffs to motivate the animal to maintain activity (20). These stimuli are potentially confounding factors of exercise effects due to their stressful nature (21). Some animals refuse to run on the treadmill after a period of time and although not reported in the literature, personal communications indicate that there may be as much as a 20% dropout rate out due to the aversive nature of the protocol (22). Moreover, the flat treadmill surface does not necessarily imitate the natural environment (23).
Therefore, we explored the feasibility and effectiveness of a new apparatus for HIIT training. The forced running wheel-bed is designed as a forced exercise apparatus. Without the need for electric shock or other stimuli, the rat must continue to run as the wheel rotates. The circular track of the wheel-bed mimics the tunnel structures in the rat cave, with uphill and downhills, which are closer to their natural environment. We based the HIIT protocol on three pieces of evidence. First, our past studies (under revision for publication) have demonstrated that moderate intensity continuous training (MICT) treadmill exercise in rats (10 min/day—without the use of shock as a motivator) for as little as 4 weeks confers a benefit to exercise tolerance. Secondly, we have also previously demonstrated that very old male rats (28 months of age) (24) undergoing treadmill MICT for 25 min/day demonstrate improved exercise tolerance, although using shock in this paradigm was highly stressful to subjects. Thirdly, we utilized a protocol based in older frail mice demonstrating that a HIIT protocol is well-tolerated and improves physical performance (15). This mouse study was a 10 min protocol, like that used previously in our rats with MIT. However, in the mouse protocol, the time span was for 16 as opposed to 4 weeks. We did not use the speeds used in mice as our anchor. Instead, we first tested these older rats to see what they were capable of, hence our approach that our first experiment was necessary to help to establish these speeds. Thus, our protocol was based upon our own studies and the literature.
After pilot testing of the protocol, we also aimed to conduct a proof-of-concept/intervention study to demonstrate the utility of the protocol. For this study, we evaluated the interaction of HIIT with enalapril treatment, a commonly used pharmacologic agent with which our laboratory has extensive experience. Enalapril is an orally effective long-acting angiotensin-converting enzyme inhibitor, used to treat hypertension and chronic heart disease (25). Various clinical trials have shown that for patients with congestive heart failure, enalapril or related angiotensin-converting enzyme (ACE) inhibitor can reduce its mortality and morbidity (26, 27). Further studies have shown that enalapril has an important role in promoting the exercise capacity of patients with heart failure with reduced left ventricular ejection fraction (HFrEF) (28, 29). Compared with placebo, enalapril significantly increased the walking tolerance after treatment (28). In addition, genetic, epidemiological, and clinical studies in older adults demonstrate that multi-modal interventions of exercise/physical activity and ACE inhibitors improve physical function (30–34). However, none of these studies have investigated the impact of HIIT in combination with ACE inhibitor treatment. Therefore, we introduced this assessment into our animal model of aging.
Thus, the first aim of the present study was to determine if aged rats of typical used in aging research will participate in the new HIIT training protocol based on forced running wheel-bed. The second aim was to investigate the interactive effects of HIIT wheel-bed running and enalapril treatment on exercise tolerance in aged rats.
Two groups of rats were used in this study. The first group included four 30-month-old Brown Norway × Fischer 344 male (F1) male rats and six 15-month-old F344 male rats. These rats were available in our colony and were primarily used to test the feasibility of the wheel-bed device and the HIIT training protocols. It should be noted that these groups of animals were not tested in any subsequent tasks.
In the second group, 37 24-month-old Fisher 344 x Brown Norway rats were used. The age of these animals represents a translation to humans of ~60-years-old (35). The rats were randomly subdivided into the control group, enalapril administration group, HIIT training group, and HIIT training combined enalapril administration group. HIIT training was performed 5 times a week, 10 min per trial for 4 weeks. Enalapril doses used were administered subcutaneously by injection at 40 mg/kg body weight per day. This dose was based upon our previous studies (36). The training program and/or enalapril administration lasted for 4 weeks. Before and after the conclusion of the study, all animals were assessed using an exercise tolerance paradigm adjusted for older animals as well as grip strength and locomotor activity, both measures of relative healthspan as described previously by our group in the context of exercise and enalapril treatment experiments in older animals (24, 36). We assessed body weight weekly over the course of the experiment as an indicium of health in our animals.
For both experiments, animals were maintained on a 12 h light/dark cycle, with free access to a standard pellet rodent diet (18% kcal from fat, no sucrose, 3.1 kcal/g, diet 2018; Harlan Teklad, Madison, WI) and water ad libitum. All experiments described herein were pre-approved by the University of Alabama at Birmingham Animal Care Committee for the care and use of animals for scientific purposes.
The six-wheel rodent walking wheel-bed (Lafayette Instrument, Lafayette, IN) was utilized in our study (Figure 1A). Two weeks prior to testing, all rats were moved to the testing room for handling and acclimation to the wheel-bed and room. All rats were acclimatized to wheel-bed 10 min per day by walking with an increase of speed by 2 m/min each day, starting with 2 m/min until the maximum speed was reached. The maximum speed was usually reached after 5–7 days acclimation. The maximum running speed was recorded based on the performance defined by when the rat fell on the wheel three times within 60 s and could not gain back momentum on the wheel-bed (Figure 1B).
Figure 1. Wheel-bed and the HIIT acclimation and training protocol. (A) Wheel-bed used on aged rats. (B) Acclimation protocol in aged rats. (C) The HIIT training diagram based on wheel-bed.
Following acclimation, the low speed, high speed, and dash speed for each rat was determined based on the calculation of 40, 80, and 100% of their individual maximum speed. HIIT habituated rats underwent 3-min warm-up period at low speed, followed by three intervals of 1-min at a constant high speed alternatively with 1-min at base speed, finishing with a final 1-min interval of dash speed (Figure 1C).
After 4 weeks of intervention, an exercise tolerance test was performed. All rats were acclimatized to a five-lane rodent treadmill (Panlab/Harvard Apparatus) by walking at a speed of 8 cm/s, 10 min/day, for 3 days. Then all rats were running on the treadmill starting at 12 cm/s for 1 min, increased by 2 cm/s each min until 16 cm/s was achieved. Running time to exhaustion was recorded using a treadmill rather than the wheel-bed to compare across our previous studies (24).
Grip strength and locomotor activity are well-characterized measures known to translationally relate to health- and life-span across multiple species (37). We used these measures to identify if this potentially stressful HIIT protocol would impact healthspan.
The forelimb grip strength was determined using an automated grip strength meter (Columbus Instruments, Columbus Ohio). The rats were grasped by the tail and suspended above a grip ring. After about 3 s, the animal was gently lowered toward the grip ring and allowed to grasp the ring with its forepaws. The experimenter then quickly lowered the remainder of the animal's body to a horizontal position and tugged the animal's tail until its grasp of the ring was broken. The mean force in grams was determined with a computerized electronic pull strain gauge that is fitted directly to the grasping ring, and the resulting value normalized to body weight. Successful trials were defined as those in which the animal grasped the ring with both forepaws without jerking (38). The maximum measurement from three successful trials was taken as the outcome. For locomotor activity, animals were placed in activity chambers (42 × 56 cm) for 5-min sessions. Spontaneous activity was recorded with an overhead camera using the tracking system Ethovision XT (Noldus Information Technology Inc., Wageningen, the Netherlands). Total distance traveled (cm) in the arena was computed as the outcome measure.
For the feasibility experiment we ran two-tailed unpaired t-test to compare maximum speed achieved during HIIT training between the 15- and 30- month-old male animals to evaluate the impact of age.
For the intervention experiment, two-way analyses of variance (ANOVAs) with exercise (HIIT vs. sedentary) and treatment (enalapril vs. vehicle) are variables were used to establish main effects and interactions. Tukey's multiple comparisons test was then employed to confirm any specific interaction effects. All analyses were conducted using commercially available software (GraphPad Prism). Differences were considered statistically significant at p < 0.05.
For the feasibility study, all 10 rats managed to complete the acclimation period and physically fit well on the forced running wheel-bed. The 30-month-old rats were able to adapt to HIIT training at a maximum speed of 12 m/min, while the 15-month-old rats were able to adapt to HIIT training at a maximum speed of 16 m/min (Figure 2). These speeds were statistically different from each other (t = 14.07, p < 0.01) indicating that middle-aged animals may train at higher speeds than the older animals. This finding helped us to determine the target maximal training speed for the older animals in our intervention study.
Rats randomized to the various exercise and treatment groups were gradually adapted to the wheel-bed device from low speed to high speed. Then, based on each individual rat's maximum speed, the actual speed for training was determined for each individual rat (Table 1). There were no observed main effects observed for exercise (p = 0.44) or treatment (p = 0.35) group assignment, nor any interactions (p = 0.06). All of animals were able to complete the training and the results below characterize the observed changes.
Due to the stressful nature of pre-behavioral testing and wheel-bed habituation, the average body weight of rats in each group dropped during pre-testing and habituation (Figure 3A). At the end of the intervention, % change in body weight was calculated for each animal and analyzed between groups. There were no effects of exercise (p = 0.87), treatment (p = 0.30) or any interactions (p = 0.91), although there was a tendency for animals in the HIIT condition to lose less weight (Figure 3B).
Figure 3. The body weight changes. (A) The time course of mean body weight changes during intervention and the timeline for training sessions and tests. (B) Body weight changes after the intervention. Con, control; Sed, sedentary; Ex, exercise; ENA, Enalapril. *p < 0.05.
After four weeks of intervention, exercise tolerance was measured. The 2-way ANOVA revealed a main effect of exercise (p < 0.001) such that all exercised animals performed better than all sedentary animals (Figure 4A). There was also an interaction of exercise and treatment (p = 0.03). Tukey's post-hoc analysis, after correction for multiple comparisons, revealed no effect of treatment within exercise conditions.
Figure 4. Exercise tolerance, relative grip strength, and locomotive assay. (A) Exercise tolerance after the intervention. (B) Grip strength by body weight after the intervention. (C) Total traveled distance in locomotor test. ***p < 0.001, ****p < 0.0001.
There were no differences between and pre- and post- intervention testing, therefore for simplicity we report results from the post-intervention testing.
There were no main effects were observed (ps > 0.05) for grip strength, but the interaction between treatment and exercise suggested there were significant differences amongst groups (p = 0.04) (Figure 4B). However, after running the Tukey's multiple comparisons, no interactive effects were observed (all comparisons p > 0.05). For locomotor activity, no main effects or interactions were observed for either factor (Figure 4C).
In the present study, we present a novel forced running wheel-bed HIIT training protocol that can be used in aged rats. This is the first rat study, to our knowledge, that adopted wheel-bed to this protocol.
Traditionally, treadmill is the most accepted apparatus for rats in HIIT training. The major advantage of the present protocol is that the wheel-bed provides a more natural environment for the rats as opposed to the treadmill and involves more skeletal muscles, both walking muscles and climbing muscles. Meanwhile, the treadmill only benefits walking muscles. Wheel running involves more muscle grip strength training. Indeed, all aged and middle-aged rats successfully accomplished the wheel-bed exercise. The new apparatus is feasible in terms of safety, technology, and operation.
The present HIIT protocol involving the wheel-bed has several advantages, namely,
i) No electrical shock or other stimulations were used in this forced exercise system.
ii) It is easy to control both exercise intensity and duration with the wheel-bed.
iii) The acclimation process helped to mitigate the stressful effects of the new instrument and room environment, which diminishes the variability in training effects.
iv) With ascending and descending slopes and planes, the wheel-bed provides a more flexible environment to get more muscles involved in rat exercise.
Despite these advantages, certain limitations of the wheel-bed HIIT model were also observed. In our feasibility experiments, the maximum speed achieved in older animals was lower than what was achieved by the middle-aged rats. It is still unclear if this effect is strain or age-based given that two different strains were used and at different ages. Rats can tear and bleed their toenails during climbing and running. To avoid this, it is necessary to trim the toenails before the protocol.
In our intervention study, animals undergoing the HIIT protocol doubled their endurance time relative to their sedentary controls. While there was a trend for animals in the HITT + enalapril group to show better performance than HIIT alone, that difference was not significant. This this may be because the impact of HIIT was so profound that any further contribution of enalapril to the effect is masked.
A limitation of this study is that we used the treadmill to assess “exercise tolerance” as opposed to using the wheel-bed. Our thought was to use the treadmill to assess this outcome as this is the gold standard in preclinical studies. In fact, many labs use shock on the treadmill to motivate rats to run at high speeds. We have found that high speeds of training are not necessary to convey benefits to exercise tolerance, especially in older animals. And we have found that it is not necessary to use shock or air puffs to motivate rats to engage in “physical activity” at low speeds. However, some laboratories will continue to investigate high running speeds and will continue to suffer from attrition. Thus, if the wheel-bed, could we used as a forced exercise model, our study could provide an alternative approach.
HIIT is being used in human aging studies, (over 139 citations in PubMed) and most notably regarding age-related diseases, especially Parkinson's (39–41). However, to explore mechanisms, there is a need to use appropriate animal models and protocols to address these hypotheses. We do continue to question whether “Stressful” exercise paradigms in rats are translatable. Although in the current study, there was no indication that HIIT impaired general healthspan given that we observed no differences amongst groups on either grip strength or locomotor activity. This should be addressed in future studies with direct comparisons between “forced” and “voluntary” exercise. In addition, all researchers should consider if low level exercise, whether MICT or HIIT, could be differentially beneficial and whether adjuvant therapies could enhance these protocols. Our paper, to be sure, represents a first foray into this question and are hopeful that this information will be useful to other groups interested in implementing HIIT protocols in studies utilizing rats as a model.
To conclude, we present a new apparatus for HIIT protocol, using a forced running wheel-bed instead of the traditional treadmill. We also demonstrated that rats of different age and strain groups tolerated the exercise protocol very well. This rat HIIT model could benefit further studies exploring the pattern and mechanism of HIIT in the aged population in the context of aging and/or age-related disease.
The original contributions presented in the study are included in the article/supplementary material, further inquiries can be directed to the corresponding author/s.
The animal study was reviewed and approved by Robert A. Kesterson, University of Alabama at Birmingham, IACUC Chair.
TB, CC, and YY: conceived and designed the experiments. YY, AB, YS, and CC: performed the experiments. YY, YS, and CC: analyzed the data. YY, CC, YS, AB, and TB: wrote the paper. All authors contributed to the article and approved the submitted version.
This work was supported by the National Institutes of Health/National Institute on Aging (R01AG054538), the UAB Nathan Shock Center (P30AG50886), and the UAB Center for Exercise Medicine.
The authors declare that the research was conducted in the absence of any commercial or financial relationships that could be construed as a potential conflict of interest.
All claims expressed in this article are solely those of the authors and do not necessarily represent those of their affiliated organizations, or those of the publisher, the editors and the reviewers. Any product that may be evaluated in this article, or claim that may be made by its manufacturer, is not guaranteed or endorsed by the publisher.
We would like to thank Marcas Bamman, PhD for providing us the wheel-bed apparatus.
1. Laursen PB, Jenkins DG. The scientific basis for high-intensity interval training: optimising training programmes and maximising performance in highly trained endurance athletes. Sports Med. (2002) 32:53–73. doi: 10.2165/00007256-200232010-00003
2. Feito Y, Heinrich KM, Butcher SJ, Poston WSC. High-Intensity Functional Training (HIFT): definition and research implications for improved fitness. Sports (Basel). (2018) 6:76. doi: 10.3390/sports6030076
3. Buchheit M, Laursen PB. High-intensity interval training, solutions to the programming puzzle: part I: cardiopulmonary emphasis. Sports Med. (2013) 43:313–38. doi: 10.1007/s40279-013-0029-x
4. Donnelly JE, Blair SN, Jakicic JM, Manore MM, Rankin JW, Smith BK, et al. American College of Sports Medicine position stand. Appropriate physical activity intervention strategies for weight loss and prevention of weight regain for adults. Med Sci Sports Exerc. (2009) 41:459–71. doi: 10.1249/MSS.0b013e3181949333
5. Weston KS, Wisloff U, Coombes JS. High-intensity interval training in patients with lifestyle-induced cardiometabolic disease: a systematic review and meta-analysis. Br J Sports Med. (2014) 48:1227–34. doi: 10.1136/bjsports-2013-092576
6. Weston AR, Myburgh KH, Lindsay FH, Dennis SC, Noakes TD, Hawley JA. Skeletal muscle buffering capacity and endurance performance after high-intensity interval training by well-trained cyclists. Eur J Appl Physiol Occup Physiol. (1997) 75:7–13. doi: 10.1007/s004210050119
7. Gaesser GA, Angadi SS. High-intensity interval training for health and fitness: can less be more? J Appl Physiol (1985). (2011) 111:1540-1. doi: 10.1152/japplphysiol.01237.2011
8. Kong Z, Fan X, Sun S, Song L, Shi Q, Nie J. Comparison of high-intensity interval training and moderate-to-vigorous continuous training for cardiometabolic health and exercise enjoyment in obese young women: a randomized controlled trial. PLoS ONE. (2016) 11:e0158589. doi: 10.1371/journal.pone.0158589
9. Jimenez-Maldonado A, Renteria I, Garcia-Suarez PC, Moncada-Jimenez J, Freire-Royes LF. The impact of high-intensity interval training on brain derived neurotrophic factor in brain: a mini-review. Front Neurosci. (2018) 12:839. doi: 10.3389/fnins.2018.00839
10. Little JP, Safdar A, Wilkin GP, Tarnopolsky MA, Gibala MJ. A practical model of low-volume high-intensity interval training induces mitochondrial biogenesis in human skeletal muscle: potential mechanisms. J Physiol. (2010) 588(Pt 6):1011-22. doi: 10.1113/jphysiol.2009.181743
11. Naderi N, Hemmatinafar M, Gaeini AA, Bahramian A, Ghardashi-Afousi A, Kordi MR, et al. High-intensity interval training increase GATA4, CITED4 and c-Kit and decreases C/EBPbeta in rats after myocardial infarction. Life Sci. (2019) 221:319–26. doi: 10.1016/j.lfs.2019.02.045
12. Gibala MJ, Hawley JA. Sprinting toward fitness. Cell Metab. (2017) 25:988–90. doi: 10.1016/j.cmet.2017.04.030
13. Wewege M, van den Berg R, Ward RE, Keech A. The effects of high-intensity interval training vs. moderate-intensity continuous training on body composition in overweight and obese adults: a systematic review and meta-analysis. Obes Rev. (2017) 18:635–46. doi: 10.1111/obr.12532
14. Kelly NA, Ford MP, Standaert DG, Watts RL, Bickel CS, Moellering DR, et al. Novel, high-intensity exercise prescription improves muscle mass, mitochondrial function, and physical capacity in individuals with Parkinson's disease. J Appl Physiol (1985). (2014) 116:582-92. doi: 10.1152/japplphysiol.01277.2013
15. Seldeen KL, Lasky G, Leiker MM, Pang M, Personius KE, Troen BR. High intensity interval training improves physical performance and frailty in aged mice. J Gerontol A Biol Sci Med Sci. (2018) 73:429–37. doi: 10.1093/gerona/glx120
16. Carter CS, Richardson A, Huffman DM. Austad S. Bring back the rat!. J Gerontol A Biol Sci Med Sci. (2020) 75:405–15. doi: 10.1093/gerona/glz298
17. Wisloff U, Helgerud J, Kemi OJ, Ellingsen O. Intensity-controlled treadmill running in rats: VO(2 max) and cardiac hypertrophy. Am J Physiol Heart Circ Physiol. (2001) 280:H1301–10. doi: 10.1152/ajpheart.2001.280.3.H1301
18. Cha J, Heng C, Reinkensmeyer DJ, Roy RR, Edgerton VR, De Leon RD. Locomotor ability in spinal rats is dependent on the amount of activity imposed on the hindlimbs during treadmill training. J Neurotrauma. (2007) 24:1000–12. doi: 10.1089/neu.2006.0233
19. Rahimi M, Shekarforoush S, Asgari AR, Khoshbaten A, Rajabi H, Bazgir B, et al. The effect of high intensity interval training on cardioprotection against ischemia-reperfusion injury in wistar rats. EXCLI J. (2015) 14:237–46. doi: 10.17179/excli2014-58
20. Brown DA, Johnson MS, Armstrong CJ, Lynch JM, Caruso NM, Ehlers LB, et al. Short-term treadmill running in the rat: what kind of stressor is it? J Appl Physiol (1985). (2007) 103:1979-85. doi: 10.1152/japplphysiol.00706.2007
21. Burgess ML, Davis JM, Borg TK, Buggy J. Intracranial self-stimulation motivates treadmill running in rats. J Appl Physiol (1985). (1991) 71:1593-7. doi: 10.1152/jappl.1991.71.4.1593
22. Oladehin A, Waters RS. Location and distribution of Fos protein expression in rat hippocampus following acute moderate aerobic exercise. Exp Brain Res. (2001) 137:26–35. doi: 10.1007/s002210000634
23. Voloshina AS, Ferris DP. Design and validation of an instrumented uneven terrain treadmill. J Appl Biomech. (2018) 34:236–9. doi: 10.1123/jab.2016-0322
24. Marzetti E, Groban L, Wohlgemuth SE, Lees HA, Lin M, Jobe H, et al. Effects of short-term GH supplementation and treadmill exercise training on physical performance and skeletal muscle apoptosis in old rats. Am J Physiol Regul Integr Comp Physiol. (2008) 294:R558–67. doi: 10.1152/ajpregu.00620.2007
25. Piepho RW. Overview of the angiotensin-converting-enzyme inhibitors. Am J Health Syst Pharm. (2000) 57(Suppl. 1):S3-7. doi: 10.1093/ajhp/57.suppl_1.S3
26. Pfeffer MA, Braunwald E, Moye LA, Basta L, Brown EJ. Jr., Cuddy TE, et al. Effect of captopril on mortality and morbidity in patients with left ventricular dysfunction after myocardial infarction Results of the survival and ventricular enlargement trial The SAVE investigators. N Engl J Med. (1992) 327:669–77. doi: 10.1056/NEJM199209033271001
27. Sleight P. Angiotensin II and trials of cardiovascular outcomes. Am J Cardiol. (2002) 89:11A-6A. discussion: 6A-7A. doi: 10.1016/S0002-9149(01)02322-0
28. Creager MA, Massie BM, Faxon DP, Friedman SD, Kramer BL, Weiner DA, et al. Acute and long-term effects of enalapril on the cardiovascular response to exercise and exercise tolerance in patients with congestive heart failure. J Am Coll Cardiol. (1985) 6:163–73. doi: 10.1016/S0735-1097(85)80269-2
29. Cleland JG, Dargie HJ, Ball SG, Gillen G, Hodsman GP, Morton JJ, et al. Effects of enalapril in heart failure: a double blind study of effects on exercise performance, renal function, hormones, and metabolic state. Br Heart J. (1985) 54:305–12. doi: 10.1136/hrt.54.3.305
30. Buford TW, Manini TM, Hsu FC, Cesari M, Anton SD, Nayfield S, et al. Angiotensin-converting enzyme inhibitor use by older adults is associated with greater functional responses to exercise. J Am Geriatr Soc. (2012) 60:1244–52. doi: 10.1111/j.1532-5415.2012.04045.x
31. Buford TW, Hsu FC, Brinkley TE, Carter CS, Church TS, Dodson JA, et al. Genetic influence on exercise-induced changes in physical function among mobility-limited older adults. Physiol Genomics. (2014) 46:149–58. doi: 10.1152/physiolgenomics.00169.2013
32. Harper SA, Baptista LC, Roberts LM, Wherry SJ, Boxer RS, Hildreth KL, et al. Angiotensin Converting Enzyme Inhibitors Combined with Exercise for Hypertensive Seniors (The ACES Trial): study protocol of a randomized controlled trial. Front Med (Lausanne). (2019) 6:327. doi: 10.3389/fmed.2019.00327
33. Brown JD, Smith SM, Strotmeyer ES, Kritchevsky SB, Gill TM, Blair SN, et al. Comparative effects of angiotensin-converting enzyme inhibitors and angiotensin receptor blockers on response to a physical activity intervention in older adults: results from the lifestyle interventions and independence for Elders study. J Gerontol A Biol Sci Med Sci. (2020) 75:1010–6. doi: 10.1093/gerona/glz120
34. Simon CB, Lee-McMullen B, Phelan D, Gilkes J, Carter CS, Buford TW. The renin-angiotensin system and prevention of age-related functional decline: where are we now? Age (Dordr). (2015) 37:9753. doi: 10.1007/s11357-015-9753-5
35. Andreollo NA, Santos EF, Araujo MR, Lopes LR. Rat's age versus human's age: what is the relationship? Arq Bras Cir Dig. (2012) 25:49–51. doi: 10.1590/S0102-67202012000100011
36. Carter CS, Giovannini S, Seo DO, DuPree J, Morgan D, Chung HY, et al. Differential effects of enalapril and losartan on body composition and indices of muscle quality in aged male Fischer 344 x Brown Norway rats. Age (Dordr). (2011) 33:167–83. doi: 10.1007/s11357-011-9243-3
37. Carter CS, Marzetti E, Leeuwenburgh C, Manini T, Foster TC, Groban L, et al. Usefulness of preclinical models for assessing the efficacy of late-life interventions for sarcopenia. J Gerontol A Biol Sci Med Sci. (2012) 67:17–27. doi: 10.1093/gerona/glr042
38. Carter CS, Cesari M, Ambrosius WT, Hu N, Diz D, Oden S, et al. Angiotensin-converting enzyme inhibition, body composition, and physical performance in aged rats. J Gerontol A Biol Sci Med Sci. (2004) 59:416–23. doi: 10.1093/gerona/59.5.B416
39. Fernandes B, Barbieri FA, Arthuso FZ, Silva FA, Moretto GF, Imaizumi LFI, et al. High-intensity interval versus moderate-intensity continuous training in individuals with Parkinson's disease: hemodynamic and functional adaptation. J Phys Act Health. (2020) 17:85–91. doi: 10.1123/jpah.2018-0588
40. van Wegen EEH, Hirsch MA, van de Berg WDJ, Vriend C, Rietberg MB, Newman MA, et al. High-intensity interval cycle ergometer training in Parkinson's disease: protocol for identifying individual response patterns using a single-subject research design. Front Neurol. (2020) 11:569880. doi: 10.3389/fneur.2020.569880
Keywords: aging, forced exercise, renin-angiotensin system, multi-modal intervention, strength
Citation: Yang Y, Banerjee A, Sun Y, Carter CS and Buford TW (2021) Interactive Effects of Enalapril Administration and Novel HIIT Wheel-Bed Training in Aged Rats. Front. Rehabilit. Sci. 2:764686. doi: 10.3389/fresc.2021.764686
Received: 25 August 2021; Accepted: 19 October 2021;
Published: 08 November 2021.
Edited by:
Alice Ryan, University of Maryland, United StatesReviewed by:
Brice T. Cleland, University of Illinois at Chicago, United StatesCopyright © 2021 Yang, Banerjee, Sun, Carter and Buford. This is an open-access article distributed under the terms of the Creative Commons Attribution License (CC BY). The use, distribution or reproduction in other forums is permitted, provided the original author(s) and the copyright owner(s) are credited and that the original publication in this journal is cited, in accordance with accepted academic practice. No use, distribution or reproduction is permitted which does not comply with these terms.
*Correspondence: Christy S. Carter, Y2FydGVyY3NAdWFiLmVkdQ==; Y2FydGVyY3NAdWFibWMuZWR1; Thomas W. Buford, dHdidWZvcmRAdWFibWMuZWR1
Disclaimer: All claims expressed in this article are solely those of the authors and do not necessarily represent those of their affiliated organizations, or those of the publisher, the editors and the reviewers. Any product that may be evaluated in this article or claim that may be made by its manufacturer is not guaranteed or endorsed by the publisher.
Research integrity at Frontiers
Learn more about the work of our research integrity team to safeguard the quality of each article we publish.