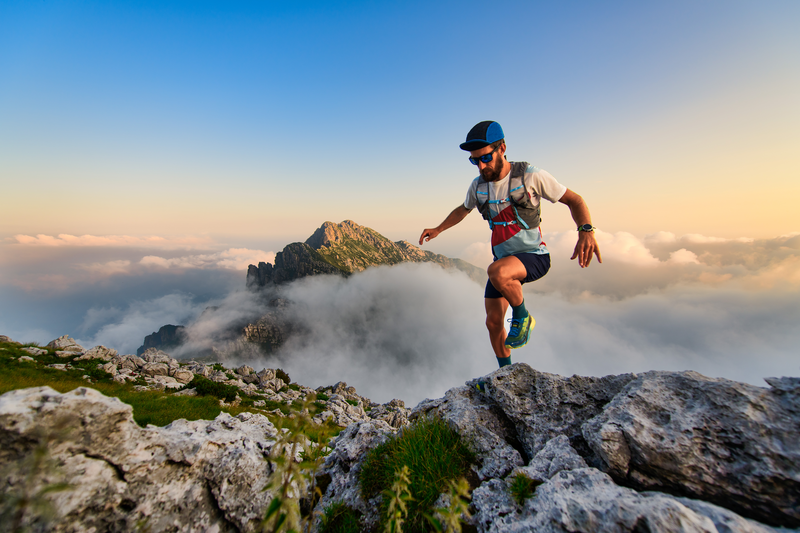
94% of researchers rate our articles as excellent or good
Learn more about the work of our research integrity team to safeguard the quality of each article we publish.
Find out more
ORIGINAL RESEARCH article
Front. Radiol.
Sec. Neuroradiology
Volume 5 - 2025 | doi: 10.3389/fradi.2025.1554017
This article is part of the Research Topic Current Challenges and Future Perspectives in Neuro-Oncological Imaging View all 3 articles
The final, formatted version of the article will be published soon.
You have multiple emails registered with Frontiers:
Please enter your email address:
If you already have an account, please login
You don't have a Frontiers account ? You can register here
Radiation necrosis is a significant late adverse effect of stereotactic radiotherapy (fSRT) for brain metastases, characterized by inflammatory processes and necrotic degeneration of healthy brain tissue.To evaluate the relationship between the incidence of radiation necrosis and the distribution of lesions across different brain regions treated with fSRT, with a focus on the potential involvement of stem cell niches.We conducted a post-hoc analysis of two separate prospective datasets consisting of data from 41 patients previously treated for brain metastases at Campus Bio-Medico University Hospital. Patients underwent fSRT using volumetric-modulated arc radiotherapy (VMAT), with MRI data collected pre-and post-treatment. Lesions were assessed for the presence of radiation necrosis based on radiological and clinical criteria, with a specific focus on their proximity to stem cell niches. A mixed-effects logistic regression model, including age and sex as covariates, was used to identify associations between brain region, stem cell niches, and the likelihood of radiation necrosis.Of 167 lesions observed, 42 (25.1%) were classified as radiation necrosis. The Deep-Periventricular region showed a significantly higher likelihood of radiation necrosis compared to other brain regions (log-OR: 1.25, 95% CI: 0.20-2.30, p = 0.02). Lesions in proximity to stem cell niches were significantly associated with an increased risk of radiation necrosis (log-OR: 1.61, 95% CI: 0.27-2.94, p = 0.018). These findings highlight the differential vulnerability of brain regions and suggest a potential role of stem cell niches in the pathogenesis of radiation necrosis.This study underscores the importance of brain region and stem cell niche involvement in the development of radiation necrosis following stereotactic ha eliminato: This observational study analyzed data from 41 patients treated for brain metastases at Campus Bio-Medico University Hospital.radiotherapy. These findings might have implications for optimizing radiotherapy planning and developing targeted strategies to mitigate the risk of radiation necrosis. Future research should focus on exploring the molecular mechanisms underlying these associations and evaluating potential neuroprotective interventions.
Keywords: brain MRI, Radiation necrosis, stem cell niches, stereotactic radiotherapy, brain metastases
Received: 31 Dec 2024; Accepted: 24 Mar 2025.
Copyright: © 2025 Mallio, Ferrari, Di Gennaro, Pileri, Bernetti, Polo, Gangemi, Giannetti, Matteucci, Beomonte Zobel, Ippolito and Ramella. This is an open-access article distributed under the terms of the Creative Commons Attribution License (CC BY). The use, distribution or reproduction in other forums is permitted, provided the original author(s) or licensor are credited and that the original publication in this journal is cited, in accordance with accepted academic practice. No use, distribution or reproduction is permitted which does not comply with these terms.
* Correspondence:
Carlo Augusto Mallio, Campus Bio-Medico University, Rome, Italy
Edy Ippolito, Campus Bio-Medico University, Rome, Italy
Disclaimer: All claims expressed in this article are solely those of the authors and do not necessarily represent those of their affiliated organizations, or those of the publisher, the editors and the reviewers. Any product that may be evaluated in this article or claim that may be made by its manufacturer is not guaranteed or endorsed by the publisher.
Research integrity at Frontiers
Learn more about the work of our research integrity team to safeguard the quality of each article we publish.