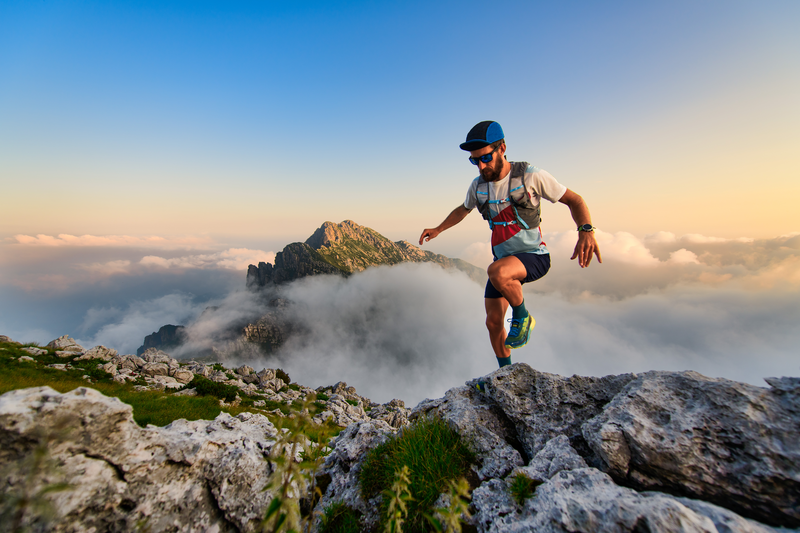
94% of researchers rate our articles as excellent or good
Learn more about the work of our research integrity team to safeguard the quality of each article we publish.
Find out more
ORIGINAL RESEARCH article
Front. Public Health
Sec. Environmental Health and Exposome
Volume 13 - 2025 | doi: 10.3389/fpubh.2025.1544174
The final, formatted version of the article will be published soon.
You have multiple emails registered with Frontiers:
Please enter your email address:
If you already have an account, please login
You don't have a Frontiers account ? You can register here
The aim of this study was to investigate the relationship between mixed chemicals in urine and the prevalence of cancers in men.Methods: A total of 1068 male subjects were included in this study. Analyses were performed by several analytical methods to ensure the stability of the results: one-way analysis, WQS analysis, Qgcomp analysis, BKMR analysis, and Restricted Cubic Spline (RCS).In the final adjusted model, each 1 increase in ln-transformed BPS increased the risk of developing cancerous prostate by 49% (95% CI:1.00-2.20). The results of multiple sensitivity analyses by WQS and Qgcomp showed that the mixed chemicals was positively correlated with the prevalence of cancers and prostate cancer in men. In the final adjusted model, each quartile increase in the WQS index was associated with a 78% (OR:1.78,95% CI:1.10-2.87) increase in the risk of cancers and a 148% (OR:2.48,95% CI:1.07-5.71) increase in the risk of prostate cancer. Each quartile increase in the Qgcomp index was associated with a 59% (OR:1.59,95% CI:1.09-2.33) increase in the risk of cancers, and a 105% (OR:2.05,95% CI:1.04-4.06) increase in the risk of prostate cancer.In conclusion, this study showed a positive correlation between the concentrations of the three groups of mixed chemicals in urine and the prevalence of cancers in men, as well as a positive correlation with the prevalence of prostate cancer.
Keywords: Cancers, NHANES, Men, Prostate, Mixed Chemicals, Urine 1.Introduction
Received: 12 Dec 2024; Accepted: 21 Feb 2025.
Copyright: © 2025 Zhang, Sun, Zhu, Wang, Zuo and Dai. This is an open-access article distributed under the terms of the Creative Commons Attribution License (CC BY). The use, distribution or reproduction in other forums is permitted, provided the original author(s) or licensor are credited and that the original publication in this journal is cited, in accordance with accepted academic practice. No use, distribution or reproduction is permitted which does not comply with these terms.
* Correspondence:
Jiuming Dai, Binhai County People's Hospital, Yancheng, China
Disclaimer: All claims expressed in this article are solely those of the authors and do not necessarily represent those of their affiliated organizations, or those of the publisher, the editors and the reviewers. Any product that may be evaluated in this article or claim that may be made by its manufacturer is not guaranteed or endorsed by the publisher.
Research integrity at Frontiers
Learn more about the work of our research integrity team to safeguard the quality of each article we publish.