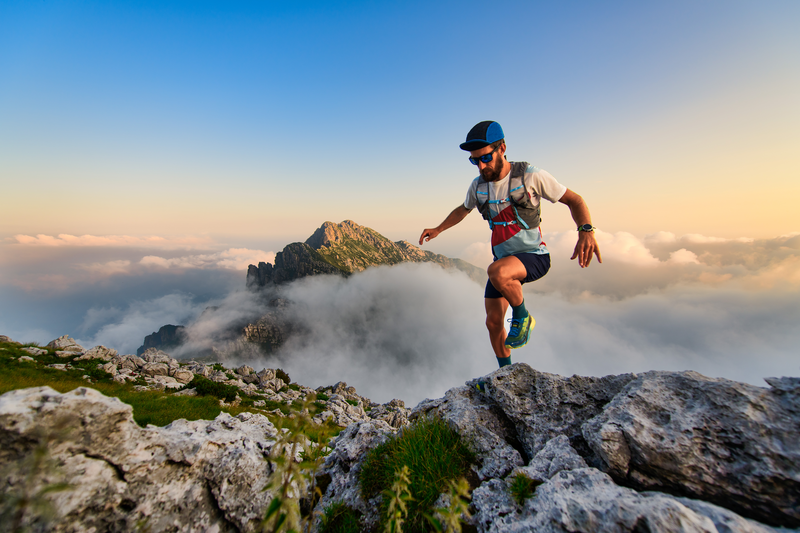
94% of researchers rate our articles as excellent or good
Learn more about the work of our research integrity team to safeguard the quality of each article we publish.
Find out more
ORIGINAL RESEARCH article
Front. Public Health
Sec. Environmental Health and Exposome
Volume 13 - 2025 | doi: 10.3389/fpubh.2025.1532507
The final, formatted version of the article will be published soon.
You have multiple emails registered with Frontiers:
Please enter your email address:
If you already have an account, please login
You don't have a Frontiers account ? You can register here
Background:Whether ambient air pollutants affect the transmission of respiratory pathogens in central Wuhan is unknown. So, we conducted a series of statistical analyses to discover the correlation between the two.: We enrolled a total of 47,668 outpatient and hospitalized patients who underwent IgM antibody tests for nine types of respiratory pathogens, namely Legionella Pneumophila type 1 (LP1), Mycoplasma Pneumoniae (MP), Q Fever Rickettsia (QFR), Chlamydia Pneumoniae (CP), Adenovirus (ADV), Respiratory Syncytial Virus (RSV), Influenza Virus A (FluA), Influenza Virus B (FluB) and Parainfluenza Virus (PIVs) between January 2014 and December 2022. Monthly measurements were taken for specific air pollutants including Fine Particulate Matter 2.5 (PM2.5), Inhalable Particulate Matter 10 (PM10), Ozone (O3), Nitrogen Dioxide (NO2), Sulfur Dioxide (SO2) and Carbon monoxide (CO) at the same periods. The association between different respiratory pathogen infections and major air pollutants was primarily analyzed using Spearman Correlation Analysis. Conclusions: MP, LP1, and FluB are correlated with respiratory infections and have been identified as potential causative agents. Elevated levels of O3 were found to augment the incidence of MP infection, we have firstly discovered the positive correlation between SO2 and ADV infection, CO and LP1 infection. The presence of air pollutants in Wuhan showed a significant correlation with respiratory pathogens, and elevated levels of air pollution facilitated their transmission to individuals.
Keywords: respiratory pathogens, Respiratory Tract Infections, Epidemiological characteristics, Air Pollutants, Prevalence
Received: 03 Dec 2024; Accepted: 06 Feb 2025.
Copyright: © 2025 Chen, Zhu, Hou, Xiang, Wu, Xiaojian, Wu, Ding and Hui. This is an open-access article distributed under the terms of the Creative Commons Attribution License (CC BY). The use, distribution or reproduction in other forums is permitted, provided the original author(s) or licensor are credited and that the original publication in this journal is cited, in accordance with accepted academic practice. No use, distribution or reproduction is permitted which does not comply with these terms.
* Correspondence:
Xiaohua Chen, General Hospital of Central Theater Command, Wuhan, China
Disclaimer: All claims expressed in this article are solely those of the authors and do not necessarily represent those of their affiliated organizations, or those of the publisher, the editors and the reviewers. Any product that may be evaluated in this article or claim that may be made by its manufacturer is not guaranteed or endorsed by the publisher.
Research integrity at Frontiers
Learn more about the work of our research integrity team to safeguard the quality of each article we publish.