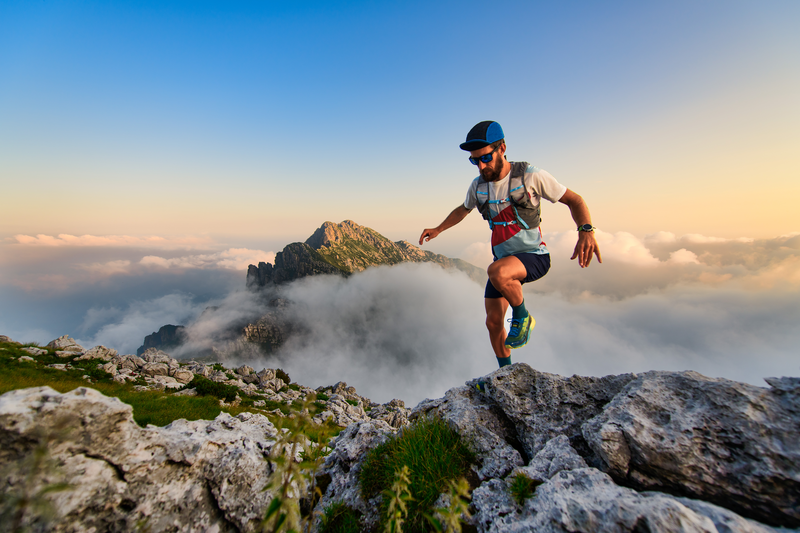
94% of researchers rate our articles as excellent or good
Learn more about the work of our research integrity team to safeguard the quality of each article we publish.
Find out more
ORIGINAL RESEARCH article
Front. Public Health
Sec. Injury Prevention and Control
Volume 13 - 2025 | doi: 10.3389/fpubh.2025.1526105
The final, formatted version of the article will be published soon.
You have multiple emails registered with Frontiers:
Please enter your email address:
If you already have an account, please login
You don't have a Frontiers account ? You can register here
Aim: Anterior cruciate ligament (ACL) reconstruction patients, particularly young athletes, often face delayed rehabilitation due to postoperative pain and unclear weight-bearing guidelines. This study evaluates an Intelligent Weight-Bearing Rehabilitation Robot's efficacy in improving postoperative outcomes.Methods: In this retrospective cohort study, 92 ACL reconstruction patients were sequentially allocated to control (n=46, standard rehabilitation) and intervention (n=46, robot-assisted training) groups. Outcomes were compared through knee function metrics, pain scores, and hospitalization duration.Results: The intervention group demonstrated superior knee function recovery, with range of motion (ROM) increasing from 41.63±5.97° to 55.89±5.13° versus 40.65±3.43° to 49.78±5.27° in controls (P<0.001). Health Status Scores (HSS) improved more substantially in the intervention group (43.07±3.83→59.93±3.30 vs 43.76±4.06→54.39±4.39; P<0.001). Pain relief was faster with robotic assistance: Visual Analog Scale (VAS) scores at 24h (3.45±0.96 vs 3.98±0.93, P=0.010) and 48h (2.37±0.49 vs 3.09±0.66, P<0.001) post-surgery showed significant differences, though this gap diminished by discharge (P=0.096). Notably, the intervention group's hospital stay was 0.9 days shorter than controls (7.07±0.83 vs 7.96±1.01 days, P<0.001). Both groups maintained equivalent safety profiles with no complications reported.Conclusion: Robotic weight-bearing rehabilitation significantly enhances functional recovery, accelerates pain resolution, and reduces hospitalization for ACL reconstruction patients. These findings advocate for technology-driven rehabilitation protocols to optimize postoperative care efficiency.
Keywords: Anterior cruciate ligament injury, Anterior Cruciate Ligament Reconstruction, robot, Knee joint function, knee joint mobility, Pain
Received: 11 Nov 2024; Accepted: 05 Mar 2025.
Copyright: © 2025 Wen, Wang, Mao, Sun, Xu and Han. This is an open-access article distributed under the terms of the Creative Commons Attribution License (CC BY). The use, distribution or reproduction in other forums is permitted, provided the original author(s) or licensor are credited and that the original publication in this journal is cited, in accordance with accepted academic practice. No use, distribution or reproduction is permitted which does not comply with these terms.
* Correspondence:
Xiaoqing Han, Department of Spinal Degeneration and Oncology, Weifang People's Hospital, Weifang, 261000, Shandong Province, China
Disclaimer: All claims expressed in this article are solely those of the authors and do not necessarily represent those of their affiliated organizations, or those of the publisher, the editors and the reviewers. Any product that may be evaluated in this article or claim that may be made by its manufacturer is not guaranteed or endorsed by the publisher.
Research integrity at Frontiers
Learn more about the work of our research integrity team to safeguard the quality of each article we publish.