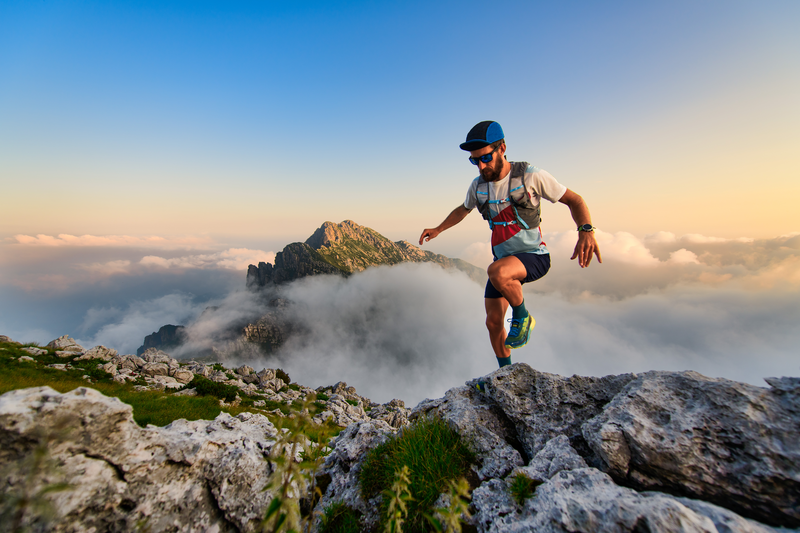
94% of researchers rate our articles as excellent or good
Learn more about the work of our research integrity team to safeguard the quality of each article we publish.
Find out more
ORIGINAL RESEARCH article
Front. Public Health
Sec. Infectious Diseases: Epidemiology and Prevention
Volume 13 - 2025 | doi: 10.3389/fpubh.2025.1502036
The final, formatted version of the article will be published soon.
You have multiple emails registered with Frontiers:
Please enter your email address:
If you already have an account, please login
You don't have a Frontiers account ? You can register here
Introduction. Acute respiratory tract infections impose a considerable burden on the health services. The development of improved prevention and treatment measures requires a better understanding of the mechanisms of infection. Since sex has been shown to be an important biological variable in the immune response to infections, we aimed to assess sex differences in the incidence rates of respiratory infections.We obtained data on cases hospitalized with diagnosed respiratory tract infections by sex and age group over a period of 11 years (2012-2022) from the Sheba Medical Centre (SMC), the largest tertiary care medical center in Israel. Nasopharyngeal samples collected from the patients with symptoms of a respiratory tract infection were examined for adenovirus, influenza, rhinovirus, parainfluenza-3, human metapneumovirus (hMPV) and respiratory syncitial virus (RSV) in the Central Virology Laboratory and. Viral RNA/DNA was extracted and tested using a real-time reverse transcription-PCR (rRT-PCR) assay. We calculated annual male to female incidence rate ratios (IRRs) which were combined over the period of the study using meta-analysis methodology.Results. There was a male excess in infection rates for all viruses, particularly in the youngest age groups of <0 and 1-4 years. Our analyses revealed that the influenza incidence rates were 42% and 28% higher in males in infants and toddlers. The male dominance was similar for adenovirus with 33% and 38% in infancy and age group 1-4. For RSV, the male to female IRR was higher at ages <1 and 1-4 (22% and 21% respectively). Males were more likely to be positive for rhinovirus in infancy and toddlers, by 40% and 25% respectively.There is evidence of an excess incidence of respiratory diseases in males. The mechanism is unclear. Other than behavioral factors, there is a need to study the role of sex hormones and genetic factors.
Keywords: sex differences, Acute respiratory tract infections, adenovirus, influenza, Rhinovirus, Parainfluenza-3, human metapneumovirus, respiratory syncytial virus
Received: 26 Sep 2024; Accepted: 03 Mar 2025.
Copyright: © 2025 Peer, Mandelboim, Jurkowicz and Green. This is an open-access article distributed under the terms of the Creative Commons Attribution License (CC BY). The use, distribution or reproduction in other forums is permitted, provided the original author(s) or licensor are credited and that the original publication in this journal is cited, in accordance with accepted academic practice. No use, distribution or reproduction is permitted which does not comply with these terms.
* Correspondence:
Victoria Peer, School of Public Health, Faculty of Social Welfare and Health Sciences, University of Haifa, Mount Carmel, Israel
Disclaimer: All claims expressed in this article are solely those of the authors and do not necessarily represent those of their affiliated organizations, or those of the publisher, the editors and the reviewers. Any product that may be evaluated in this article or claim that may be made by its manufacturer is not guaranteed or endorsed by the publisher.
Research integrity at Frontiers
Learn more about the work of our research integrity team to safeguard the quality of each article we publish.