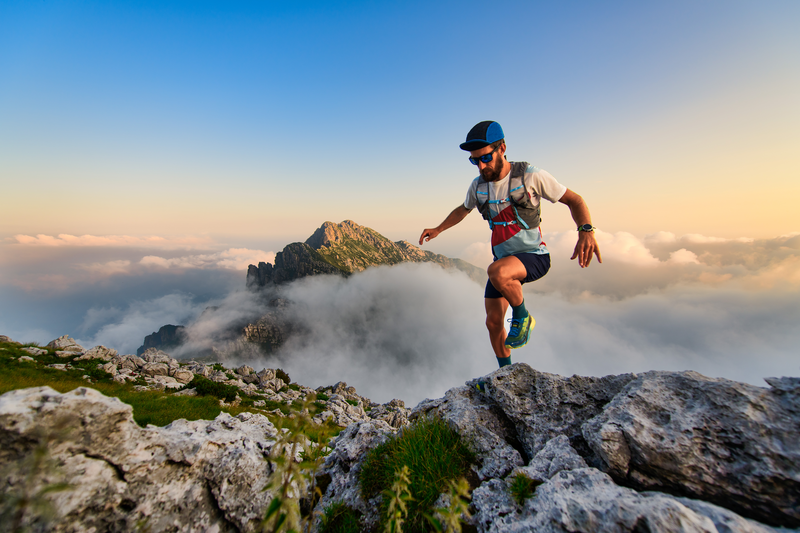
95% of researchers rate our articles as excellent or good
Learn more about the work of our research integrity team to safeguard the quality of each article we publish.
Find out more
ORIGINAL RESEARCH article
Front. Public Health , 18 March 2025
Sec. Environmental Health and Exposome
Volume 13 - 2025 | https://doi.org/10.3389/fpubh.2025.1484223
Background: While the correlation between cancer and air pollutants is well-established, research on the delayed effects of NO2 on oral cancer remains limited.
Methods: We collected data on nitrogen dioxide (NO2) along with diagnosed cases of oral cancer in Guangxi, China, and analyzed the correlation between exposure to NO2 and the prevalence of oral cancer.
Results: The study included 1,841 participants diagnosed with oral malignancies, consisting of 1,179 males (64.0%) and 662 females (36.0%), with a mean age of 55.9 ± 14.0 years. The NO2 concentration is 20.2 ± 10.4 μg/m3. The highest cumulative effects of NO2 exposure were observed at a 3-year cumulative lag, with a relative risk (RR) of 1.115 (95% CI: 1.102–1.128). For males, the most pronounced effect of NO2 also occurred at a 3-year lag (RR = 1.110, 95% CI: 1.094–1.127). Similarly, among females, the significant cumulative impact of NO2 was found at a 3-year lag (RR = 1.123, 95% CI: 1.101–1.145). For individuals under 60 years of age, the cumulative impact of NO2 peaked at the same 3-year lag (RR = 1.102, 95% CI: 1.085–1.120). For individuals aged 60 and above, the highest cumulative impact of NO2 was also detected at a 3-year lag (RR = 1.132, 95% CI: 1.112–1.152). For the group with normal BMI, the highest cumulative effect of NO2 exposure was also observed at the 3-year lag period (RR = 1.289, 95% CI: 1.217–1.365), consistent with the findings for other groups.
Conclusion: These findings suggest a significant lagged effect of long-term NO2 exposure on oral cancer, with varying associations between NO2 and oral cancer across different ages and genders.
Oral cancer remains a prevalent malignancy affecting the head and neck region (1–3). The increasing incidence of this disease has resulted in significant socio-economic implications (4–6). According to data from the International Agency for Research on Cancer, oral cancer was responsible for approximately 377,000 newly diagnosed cases and 177,000 deaths globally in 2020 (7). Between 1990 and 2019, China experienced a notable increase in oral cancer incidence, with the age-standardized rate surpassing the global average. In 2022, China is expected to report 31,733 new cases of oral cancer, reflecting a rate 1.26 times higher than that of the United States (8). Therefore, it is crucial to explore the risk factors associated with oral cancer to effectively reduce its burden.
The rapid development of the global economy and the transportation industry, coupled with accelerating industrialization and urbanization, resulted in atmospheric contamination that poses a significant risk to community well-being and increases morbidity rates (9, 10). The primary airborne contaminants that threaten human health include fine and coarse particles (PM2.5, PM10) as well as harmful gases such as CO, NO2, SO2, and O3 (10). Emerging research indicates that exposure to ambient atmospheric contamination is associated with a heightened risk of adverse health outcomes, particularly malignancies such as pulmonary carcinoma (11). Findings from meta-analyses suggest that indoor contaminants also pose a risk for oral malignancies, indicating that issues related to residential air quality may be linked to an increased likelihood of various tumor types beyond lung cancer (12). Evidence indicates that atmospheric contamination adversely affects the oral cavity and its surrounding structures, resulting in issues such as tooth decay, pulp inflammation, and gum diseases (13–16). This suggests that the mouth reacts to exposure to airborne toxins (17). Air pollutants can either dissolve or deposit within the oral environment, leading to inflammation and oxidative damage, which may compromise the body’s immune response and exacerbate symptoms of oral diseases (18). Furthermore, additional data suggest that exposure to atmospheric contamination during pregnancy is correlated with an increased risk of facial and palatal malformations in children (19, 20). Additionally, exposure to airborne toxins may increase susceptibility to premalignant oral lesions and cancers of the mouth (17, 21, 22).
Research conducted in Taiwan has established a significant correlation between levels of PM2.5 exposure and an increased likelihood of developing oral malignancies. The findings indicate that exposure to elevated PM2.5 concentrations is associated with a 43% higher risk of oral cancer, even after controlling for potential confounding factors such as tobacco use and habitual betel quid chewing (23). Furthermore, a meta-analysis has identified a strong association between indoor air pollution and malignancies of the oral cavity, nasopharynx, pharynx, and larynx (12). This has led some researchers to suggest that home air quality may independently elevate the risk of oral cancer (24). Nevertheless, evidence remains insufficient to substantiate a significant delayed effect of NO2 on the incidence of oral cancer. To address these research gaps, we conducted an investigation into oral cancer cases in Guangxi, a province in China. The primary objective of this study is to evaluate whether exposure to NO2 has a cumulative delayed effect on the oral cancer. Applying a time-series model, we examined the delayed temporal relationship between NO2 exposure and the prevalence of oral cancer.
In this time series analysis, data were obtained from The Affiliated Stomatology Hospital of Guangxi Medical University, the only Grade A tertiary oral specialty hospital in Guangxi. The dataset includes oral cancer diagnosed cases from January 2016 to December 2020, accounting for 16.98% of the total number of hospitalized patients in the hospital. The analysis focused on patients who were recently diagnosed with oral cancer and hospitalized for the first time for therapeutic intervention. All participants in the study were residents of Guangxi, China. For each case, we extracted data on age, sex, marital status, residential address (at the county level), body mass index, and admission date, as shown in Table 1. The study protocol received approval from the Ethics Committee of Guangxi Medical University (No. 2023KY0271). Given that this study involved a retrospective analysis of oral cancer cases retrieved from the hospital’s case management system, informed consent was waived for individual patients.
Data on NO2 was derived from the Chinese Academy of Sciences Resource and Environmental Science and Data Center, covering the period from November 1, 2013, to December 31, 2020. Additionally, meteorological data, primarily comprising daily average temperature (T, °C) and relative humidity (RH, %), were obtained from the National Meteorological Science Center,1 spanning from November 1, 2013, to December 31, 2020.
The data on oral cancer followed a quasipoisson distribution. To evaluate the associations between air pollutants and oral cancer cases, a Quasi-Poisson regression model, based on a generalized linear model and combined with a distributed lag non-linear model (DLNM), was employed. The model utilized daily average concentrations of NO2, as predictor variables. When constructing the regression model between daily average NO2 concentrations and daily case counts, average temperature was included as a cross-basis function to account for potential meteorological influences. Average relative humidity and indicator variables for day of the week were also incorporated into the model as a covariate. Based on previous studies, a natural cubic spline function with three degrees of freedom (df = 3) was applied to fit the lag-response relationship (25, 26).
Additionally, to ensure the robustness of the model fit, a sensitivity analysis was performed using a dual-pollutant model and by adjusting the degrees of freedom for meteorological variables. This study aims to evaluate the impact of NO2 on the prevalence of oral cancer. The findings will quantify the effect of a 1 μg/m3 increase in concentrations of NO2 in μg/m3 on the prevalence of oral cancer within the study population. Relative risk (RR) will be used as the primary metric for this assessment.
Statistical significance was assessed using a two-tailed p-value threshold of <0.05. All analyses were conducted using R software version 4.3.1.
This study enrolled 1,841 participants, consisting of 1,179 males (64.0%) and 662 females (36.0%), with a mean age of 55.9 ± 14.0 years. The majority of participants were married (91.8%), and 58.2% had a BMI within the Chinese standard normal range (18.5–24). Furthermore, the distribution of oral hygiene status indicated that 43.7% had bad oral hygiene, 31.8% had ordinary oral hygiene, and 22.3% had good oral hygiene, as show in Figure 1 and Table 1. The NO2 concentration is 20.2 ± 10.4 μg/m3. Figure 2A illustrates that from 2013 to 2020, the overall trend of NO2 concentration exhibited fluctuations, accompanied by seasonal variations. Figure 2B predicts the impact of NO2 exposure on the prevalence of oral cancer, indicating an increasing trend correlated with longer exposure durations and higher concentrations. Figure 3 illustrates the effects of NO2 exposure and its lagged impacts on oral cancer across various subgroups. The findings indicate that the heterogeneity observed between different genders and age groups is minimal.
Figure 2. NO2 concentration time sequence diagram and the predicted heat map of its impact on oral cancer. Panel (A) shows the changes in NO2 concentration from 2013 to 2020. Panel (B) shows the predicted heat map of the impact of NO2 on oral cancer under different exposure times and different concentrations.
Figure 3. The subgroup analysis of the predictive heatmap of NO2’s impact on oral cancer. Panels (A–D) represent the predictive heat maps of the impact of NO2 on oral cancer in males, females, individuals aged 60 years and older, and those younger than 60, respectively.
Table 2 summarize the associations for patients associated with NO2 exposure during different cumulative lag periods and the prevalence of oral cancer. In cumulative lag1-lag3 years, the highest association of NO2 with oral cancer was observed at the cumulative lag of 3 years (RR = 1.115, 95% CI: 1.102–1.128).
Figure 4A presents the cumulative lag model illustrating the impact of air pollutants on the prevalence of oral cancer by gender. For females, the maximum cumulative lagged effects of NO2 was observed at a cumulative lag of 3 years. Specifically, for each 1 μg/m3 increase in the concentrations of NO2, the prevalence of oral cancer increased by 12.3% (RR = 1.123, 95% CI: 1.101–1.145). For males, the highest cumulative lagged effects of NO2 was seen at a lag of 3 years. A 1 μg/m3 increase in NO2 concentrations resulted in a 11.0% (RR = 1.110, 95% CI: 1.094–1.127) increase in the prevalence of oral cancer, respectively.
Figure 4. DLNM analysis results stratified by age, gender and BMI. Panel (A) shows the results of the gender-stratified analysis, capturing the lag effects of NO₂ exposure on different gender groups over time. Panel (B) shows the results of the age-stratified analysis, illustrating the lag effects of NO2 exposure on various age groups. Panel (C) shows the results of the analysis stratified by BMI, illustrating the lag effects of NO2 exposure on different BMI categories.
In the age-stratified cumulative lag model for NO2 and oral cancer, as illustrated in Figure 4B, the maximum cumulative lag effect of NO2 on the prevalence of oral cancer in individuals under 60 years occurs at a lag of 3 years. For each 1 μg/m3 increase in NO2 concentrations at this cumulative lag, the prevalence of oral cancer increases by 10.2% (RR = 1.102, 95% CI: 1.085–1.120). In the aged 60 and above population the maximum cumulative lag effect for NO2 also occurs at 3 years, with an associated increase in the prevalence of oral cancer by 13.2% (RR = 1.132, 95% CI: 1.112–1.152). Figure 5 categorizes all study participants into four distinct age groups: under 45 years, 45–60 years, 60–75 years, and 75 years or older. Across all four groups, the maximum cumulative lag effect of NO2 on oral cancer is observed at a 3-year lag, with minimal heterogeneity in the results among the groups.
Figure 5. Age subgroup analysis results of DLNM. The results of the DLNM analysis are detailed for these four age subgroups: under 45 years, 45–60 years, 60–75 years, and 75 years or older.
Figure 4C illustrates the influence of NO2 exposure on oral cancer incidence across various BMI categories. The most significant cumulative lag effect of NO2 on the three BMI groups—underweight (<18.5), normal weight (18.5–24), and overweight/obese (≥24)—is observed at a cumulative lag of 3 years, with RR of 1.256 (95% CI: 1.152–1.370), 1.289 (95% CI: 1.217–1.365), and 1.325 (95% CI: 1.191–1.473), respectively.
As indicated in Table 3, NO2 was selected for the dual-pollutant models at lags of 3 years, respectively. This selection implies that each additional pollutant was introduced at the lag point where the maximum effect was observed in the single-pollutant models. The inclusion of other pollutants did not result in significant alterations to the model outcomes for NO2. After integrating SO2, CO, O3, PM2.5, and PM10 into the model, the impact of NO2 on oral cancer was quantified as follows: 11.6% (RR = 1.116, 95% CI: 1.105–1.128), 13.4% (RR = 1.134, 95% CI: 1.121–1.148), 12.0% (RR = 1.120, 95% CI: 1.108–1.132), 13.0% (RR = 1.130, 95% CI: 1.118–1.142), and 14.2% (RR = 1.142, 95% CI: 1.128–1.155).
Supplementary Table S1 details the variations in maximum effect values associated with exposure to NO2, analyzed across different degrees of freedom for meteorological factors (df = 1, 2, 4, 5). The findings suggest that altering the degrees of freedom for meteorological factors does not significantly impact the effect values. Consequently, the sensitivity analysis confirms the robustness of the model fit used in this study.
This research explores the potential long-term effects of ambient NO2 exposure on the frequency of oral cancer. Previous studies have established a correlation between air contaminants and oral cancer (27); however, our study offers further insights into the impact of chronic exposure to air pollutants on the progression of this disease. Our research findings indicate that the trend in NO₂ concentration changes exhibits seasonal fluctuations. This may be attributed to seasonal variations in wind and precipitation patterns, which can influence the dispersion and deposition of pollutants. Furthermore, our study found that exposure to nitrogen dioxide is associated with an increased likelihood of developing oral cancer, even after controlling for weather conditions. These results enhance our understanding of the relationship between environmental air pollution and oral health. We suggest that long-term exposure to NO2 poses risks that extend beyond respiratory health (28), with significant implications for oral well-being. Our findings contribute to the growing body of research highlighting the detrimental effects of air pollution on oral cancer.
Other studies have established a connection between NO2 and cancer incidence (29, 30). For instance, a study conducted in Germany found a correlation between NO2 exposure and elevated mortality rates, while Canadian research indicated that even low levels of NO2 increased the risk of premenopausal breast cancer (31, 32). When NO2 is inhaled in excessive amounts, it can cause oxidative damage to the blood, leading to oxidative stress. This process results in the carbonylation of proteins, oxidative injury to cell membranes, and ultimately, apoptosis (33).
Our research indicates a significant correlation between exposure to NO2 and the frequency of oral cancer, particularly after a cumulative exposure duration of 3 years. Specifically, 1 year post-exposure to NO2, the risk of is estimated at 7.2%, which escalates to 11.5% after 3 years of exposure, suggesting a trend of increasing risk with extended exposure duration. The heightened prevalence of cancer associated with chronic NO2 exposure may be attributed to its strong association with combustion byproducts, including volatile organic compounds and carbon-containing materials, many of which are recognized as carcinogenic (34, 35). These findings corroborate conclusions from other studies, which suggest that the delayed effects of atmospheric pollutants may contribute to an elevated cancer risk (29, 36).
The development of cancer is typically a protracted process influenced by the gradual accumulation of atmospheric contaminants, which can inflict enduring damage to human health (37, 38). This cumulative damage progressively heightens the risk of cancer over time. Our research demonstrates that NO2 have significant delayed effects on the likelihood of developing oral cancer. Furthermore, as both the duration of lag time and the concentration of pollutants increase, the associated risk of disease escalates correspondingly. Studies indicate that in populations with metabolic abnormalities, the lagged effects of air pollutants can result in chronic vascular damage (39). Such persistent vascular injury may also contribute to the development of various other health conditions (40). Nitrogen oxides can be converted into nitrite in the body, which can subsequently be transformed into carcinogenic nitrosamines. Nitrosamines are regarded as one of the primary contributors to elevated cancer risk. The specific mechanism underlying this process involves complex biochemical reactions; particularly in an acidic environment, the combination of nitrite and amine substances leads to the formation of nitrosamines (41). Exposure to nitrogen oxides may lead to persistent inflammatory reactions, thereby promoting cancer development. Studies have demonstrated a correlation between nitrogen oxides and various types of cancer, which is closely linked to the chronic inflammatory response they induce (42). Systematic reviews have established a robust association between chronic exposure to NO2 and urological cancer (43), highlighting the persistent potential impact of air pollution on community health, particularly in relation to cancer risk and other chronic illnesses.
In addition, research indicates that exposure to NO2 with a lag of 0 to 2 years, 3 to 5 years, and 6 to 10 years is associated with an increased risk of breast cancer (44). This indicates that individuals exposed to these pollutants may not exhibit symptoms of cancer until a considerable period has elapsed (45). The latency effect may contribute to an underestimation of the health risks posed by air pollution, potentially leading to more severe health complications (46). Given the geographical disparities in air pollution management and economic inequalities, we recommend the establishment of localized guidelines for airborne NO2 levels. This approach may yield more significant health benefits by ensuring that economically disadvantaged areas can pursue growth opportunities while allowing more developed regions to continue contributing to global efforts to mitigate and control air pollution (47).
This research conducted stratified analyses by gender and age to identify vulnerable populations exposed to various air pollutants, examining the association between atmospheric contamination and health outcomes within these subgroups. The findings indicate that female demonstrate greater sensitivity to the effects of air pollution, particularly NO2, compared to male (48). Several biological and environmental factors may contribute these gender disparities. Female sensitivity to air pollutants is partly attributed to hormonal influences, which may render female more susceptible to pollutants like PM2.5 and NO2 (49). Additionally, fluctuations in hormone levels during the menstrual cycle and pregnancy may further heighten sensitivity, as these changes can affect airway function and immune response, especially during periods of elevated hormones, such as the premenstrual phase or pregnancy (49). Furthermore, differences in occupational exposure, and lifestyle habits between genders contribute to the observed variability in outcomes (50). The current investigation also found that the impact of air pollution on oral cancer is significantly more pronounced among individuals aged 60 years and older. This heightened effect may be linked to the decreased immune resistance commonly seen in the older adult (51). In comparison to younger individuals, middle-aged and older adults typically exhibit diminished physical function and reduced levels of physical activity, which may lead to increased sensitivity to air pollution (52, 53).
Given the observed lagged effects of air pollution, it is imperative to consider their long-term implications for public health, particularly among vulnerable populations. Policy recommendations should encompass both short-term and long-term strategies aimed at reducing exposure. Enhancing air quality monitoring systems and issuing health advisories during periods of elevated pollution are critical, especially in regions with high-risk groups such as the older adult and children. Furthermore, public health education campaigns can effectively raise awareness about the dangers of air pollution. These campaigns should highlight the importance of minimizing outdoor activities during high pollution events, promoting indoor air purification, and advocating for the use of protective measures such as masks during episodes of significant pollution.
This investigation is subject to several limitations. The primary limitation is the method of exposure assessment, which relied on the residential addresses of participants at the county level. This approach fails to capture detailed personal exposure levels, including individual daily activities, specific locations, and the distinction between indoor and outdoor environments. Consequently, this limitation may introduce potential exposure misclassification, as the actual exposure levels of individuals may differ significantly from those estimated based solely on their residential addresses. In addition, since the cases are from a single-center dataset, it may not accurately reflect the true occurrence of the condition, and the sample may lack representativeness. We recommend that future research employ a prospective design to more accurately assess relevant factors, thereby enhancing the reliability and validity of the findings.
In summary, this study demonstrates that prolonged exposure to NO2 is associated with an increased prevalence of oral cancer, highlighting a significant lag effect. Furthermore, the influence of environmental air pollution on oral cancer is notably greater among females and individuals aged over 60. Consequently, it is essential to improve environmental quality to reduce pollution levels and promote of public health advancements.
The original contributions presented in the study are included in the article/Supplementary material, further inquiries can be directed to the corresponding authors.
The studies involving humans were approved by Ethics Committee Guangxi Medical University. The studies were conducted in accordance with the local legislation and institutional requirements. Written informed consent for participation was not required from the participants or the participants’ legal guardians/next of kin in accordance with the national legislation and institutional requirements.
HP: Data curation, Methodology, Validation, Writing – original draft, Writing – review & editing. XW: Data curation, Methodology, Writing – original draft. YL: Methodology, Writing – original draft. LL: Data curation, Writing – original draft. DW: Data curation, Writing – original draft. YHX: Data curation, Writing – original draft. LX: Data curation, Writing – original draft. YXL: Data curation, Writing – original draft. XL: Data curation, Writing – original draft. YX: Visualization, Writing – original draft. FL: Conceptualization, Data curation, Writing – review & editing. HC: Conceptualization, Data curation, Writing – review & editing. CN: Conceptualization, Data curation, Writing – review & editing.
The author(s) declare that financial support was received for the research and/or publication of this article. This work was supported by the Middle/Young aged Teachers’ Research Ability Improvement Project of Guangxi Higher Education [gant numbers 2024KY0117] and Outstanding Youth Fund of the Natural Science Foundation of Guangxi in China [grant numbers 2023GXNSFFA026007].
The author would like to thank China High Air Pollutant dataset, Tracking Air Pollution in China project and the Chinese Academy of Sciences Resource and Environmental Science and Data Center for providing the access to the air pollution data.
The authors declare that the research was conducted in the absence of any commercial or financial relationships that could be construed as a potential conflict of interest.
All claims expressed in this article are solely those of the authors and do not necessarily represent those of their affiliated organizations, or those of the publisher, the editors and the reviewers. Any product that may be evaluated in this article, or claim that may be made by its manufacturer, is not guaranteed or endorsed by the publisher.
The Supplementary material for this article can be found online at: https://www.frontiersin.org/articles/10.3389/fpubh.2025.1484223/full#supplementary-material
1. Bawaskar, HS, and Bawaskar, PH. Oral diseases: a global public health challenge. Lancet. (2020) 395:185–6. doi: 10.1016/s0140-6736(19)33016-8
2. Miranda-Filho, A, and Bray, F. Global patterns and trends in cancers of the lip, tongue and mouth. Oral Oncol. (2020) 102:104551. doi: 10.1016/j.oraloncology.2019.104551
3. Gemrc, C. Burden of cancer in the eastern Mediterranean region, 2005-2015: findings from the global burden of disease 2015 study. Int J Public Health. (2018) 63:151–64. doi: 10.1007/s00038-017-0999-9
4. Peres, MA, Macpherson, LMD, Weyant, RJ, Daly, B, Venturelli, R, Mathur, MR, et al. Oral diseases: a global public health challenge. Lancet. (2019) 394:249–60. doi: 10.1016/s0140-6736(19)31146-8
5. Valdez, JA, and Brennan, MT. Impact of Oral Cancer on quality of life. Dent Clin N Am. (2018) 62:143–54. doi: 10.1016/j.cden.2017.09.001
6. Ribeiro-Rotta, RF, Rosa, EA, Milani, V, Dias, NR, Masterson, D, da Silva, EN, et al. The cost of oral cancer: a systematic review. PLoS One. (2022) 17:e0266346. doi: 10.1371/journal.pone.0266346
7. Grf, C. Global burden of 87 risk factors in 204 countries and territories, 1990-2019: a systematic analysis for the global burden of disease study 2019. Lancet. (2020) 396:1223–49. doi: 10.1016/s0140-6736(20)30752-2
8. Xia, C, Dong, X, Li, H, Cao, M, Sun, D, He, S, et al. Cancer statistics in China and United States, 2022: profiles, trends, and determinants. Chin Med J. (2022) 135:584–90. doi: 10.1097/cm9.0000000000002108
9. Yin, P, Brauer, M, Cohen, AJ, Wang, H, Li, J, Burnett, RT, et al. The effect of air pollution on deaths, disease burden, and life expectancy across China and its provinces, 1990-2017: an analysis for the global burden of disease study 2017. Lancet Planet Health. (2020) 4:e386–98. doi: 10.1016/s2542-5196(20)30161-3
10. Cohen, AJ, Brauer, M, Burnett, R, Anderson, HR, Frostad, J, Estep, K, et al. Estimates and 25-year trends of the global burden of disease attributable to ambient air pollution: an analysis of data from the global burden of diseases study 2015. Lancet (London, England). (2017) 389:1907–18. doi: 10.1016/s0140-6736(17)30505-6
11. Solomon, PA. Air pollution and health: bridging the gap from sources to health outcomes. Environ Health Perspect. (2011) 119:A156–7. doi: 10.1289/ehp.1103660
12. Josyula, S, Lin, J, Xue, X, Rothman, N, Lan, Q, Rohan, TE, et al. Household air pollution and cancers other than lung: a meta-analysis. Environ Health. (2015) 14:24. doi: 10.1186/s12940-015-0001-3
13. Folayan, MO, El Tantawi, M, Schroth, RJ, Kemoli, AM, Gaffar, B, Amalia, R, et al. Association between environmental health, ecosystem vitality, and early childhood caries. Front Pediatr. (2020) 8:196. doi: 10.3389/fped.2020.00196
14. Li, W, Zong, X, He, YS, Meng, T, Tang, Y, Yang, Q, et al. Association between short-term exposure to ambient air pollution and outpatient visits for pulpitis in Hefei, China: a time series study. Environ Sci Pollut Res Int. (2023) 30:78607–18. doi: 10.1007/s11356-023-28095-7
15. Huang, K, Feng, LF, Liu, ZY, Li, ZH, Mao, YC, Wang, XQ, et al. The modification of meteorological factors on the relationship between air pollution and periodontal diseases: an exploration based on different interaction strategies. Environ Geochem Health. (2023) 45:8187–202. doi: 10.1007/s10653-023-01705-6
16. Marruganti, C, Shin, HS, Sim, SJ, Grandini, S, Laforí, A, and Romandini, M. Air pollution as a risk Indicator for periodontitis. Biomedicines. (2023) 11:443. doi: 10.3390/biomedicines11020443
17. Gregorczyk-Maga, I, Celejewska-Wojcik, N, Gosiewska-Pawlica, D, Darczuk, D, Kesek, B, Maga, M, et al. Exposure to air pollution and oxidative stress markers in patients with potentially malignant oral disorders. J Physiol Pharmacol. (2019) 70:9. doi: 10.26402/jpp.2019.1.09
18. He, L, Norris, C, Cui, X, Li, Z, Barkjohn, KK, Teng, Y, et al. Oral cavity response to air pollutant exposure and association with pulmonary inflammation and symptoms in asthmatic children. Environ Res. (2022) 206:112275. doi: 10.1016/j.envres.2021.112275
19. Wright, CY, Kapwata, T, Wernecke, B, Malherbe, H, Bütow, KW, Naidoo, N, et al. The risk of orofacial cleft lip/palate due to maternal ambient air pollution exposure: a call for further research in South Africa. Ann Glob Health. (2023) 89:6. doi: 10.5334/aogh.4007
20. Liu, Y, Zhou, L, Zhang, W, Yang, Y, Yang, Y, Pan, L, et al. Time series analysis on association between ambient air pollutants and orofacial clefts during pregnancy in Lanzhou, China. Environ Sci Pollut Res Int. (2022) 29:72898–907. doi: 10.1007/s11356-022-19855-y
21. Datzmann, T, Markevych, I, Trautmann, F, Heinrich, J, Schmitt, J, and Tesch, F. Outdoor air pollution, green space, and cancer incidence in Saxony: a semi-individual cohort study. BMC Public Health. (2018) 18:715. doi: 10.1186/s12889-018-5615-2
22. Jenwitheesuk, K, Peansukwech, U, and Jenwitheesuk, K. Predictive MERRA-2 aerosol diagnostic model for oral, oropharyngeal and laryngeal cancer caused by air pollution in Thai population. Toxicol Rep. (2022) 9:970–6. doi: 10.1016/j.toxrep.2022.04.015
23. Chu, YH, Kao, SW, Tantoh, DM, Ko, PC, Lan, SJ, and Liaw, YP. Association between fine particulate matter and oral cancer among Taiwanese men. J Investig Med. (2019) 67:34–8. doi: 10.1136/jim-2016-000263
24. Raj, AT, Patil, S, Sarode, SC, Sarode, GS, and Rajkumar, C. Evaluating the association between household air pollution and oral cancer. Oral Oncol. (2017) 75:178–9. doi: 10.1016/j.oraloncology.2017.11.012
25. Chen, H, Cheng, Z, Li, M, Luo, P, Duan, Y, Fan, J, et al. Ambient air pollution and hospitalizations for ischemic stroke: a time series analysis using a distributed lag nonlinear model in Chongqing, China. Front Public Health. (2021) 9:762597. doi: 10.3389/fpubh.2021.762597
26. Huang, K, Ding, K, Yang, XJ, Hu, CY, Jiang, W, Hua, XG, et al. Association between short-term exposure to ambient air pollutants and the risk of tuberculosis outpatient visits: a time-series study in Hefei, China. Environ Res. (2020) 184:109343. doi: 10.1016/j.envres.2020.109343
27. Zhan, H, Liu, D, Deji, Z, Liang, W, and Li, J. Exposure to mixture particulate contaminants in the air and the risk of oral cancer: an updated systematic review and meta-analysis. Heliyon. (2024) 10:e38568. doi: 10.1016/j.heliyon.2024.e38568
28. Li, Z, Mao, X, Liu, Q, Song, H, Ji, Y, Xu, D, et al. Long-term effect of exposure to ambient air pollution on the risk of active tuberculosis. Int J Infect Dis. (2019) 87:177–84. doi: 10.1016/j.ijid.2019.07.027
29. Liu, CS, Wei, Y, Danesh Yazdi, M, Qiu, X, Castro, E, Zhu, Q, et al. Long-term association of air pollution and incidence of lung cancer among older Americans: a national study in the Medicare cohort. Environ Int. (2023) 181:108266. doi: 10.1016/j.envint.2023.108266
30. Stafoggia, M, Oftedal, B, Chen, J, Rodopoulou, S, Renzi, M, Atkinson, RW, et al. Long-term exposure to low ambient air pollution concentrations and mortality among 28 million people: results from seven large European cohorts within the ELAPSE project. Lancet Planet Health. (2022) 6:e9–e18. doi: 10.1016/s2542-5196(21)00277-1
31. Heinrich, J, Thiering, E, Rzehak, P, Krämer, U, Hochadel, M, Rauchfuss, KM, et al. Long-term exposure to NO2 and PM10 and all-cause and cause-specific mortality in a prospective cohort of women. Occup Environ Med. (2013) 70:179–86. doi: 10.1136/oemed-2012-100876
32. Goldberg, MS, Villeneuve, PJ, Crouse, D, To, T, Weichenthal, SA, Wall, C, et al. Associations between incident breast cancer and ambient concentrations of nitrogen dioxide from a national land use regression model in the Canadian National Breast Screening Study. Environ Int. (2019) 133:105182. doi: 10.1016/j.envint.2019.105182
33. Trushina, E, and McMurray, CT. Oxidative stress and mitochondrial dysfunction in neurodegenerative diseases. Neuroscience. (2007) 145:1233–48. doi: 10.1016/j.neuroscience.2006.10.056
34. Hamra, GB, Laden, F, Cohen, AJ, Raaschou-Nielsen, O, Brauer, M, and Loomis, D. Lung Cancer and exposure to nitrogen dioxide and traffic: a systematic review and Meta-analysis. Environ Health Perspect. (2015) 123:1107–12. doi: 10.1289/ehp.1408882
35. Curren, KC, Dann, TF, and Wang, DK. Ambient air 1, 3-butadiene concentrations in Canada (1995–2003): seasonal, day of week variations, trends, and source influences. Atmos Environ. (2006) 40:170–81. doi: 10.1016/j.atmosenv.2005.09.025
36. Li, W, and Wang, W. Causal effects of exposure to ambient air pollution on cancer risk: insights from genetic evidence. Sci Total Environ. (2024) 912:168843. doi: 10.1016/j.scitotenv.2023.168843
37. Turner, MC, Andersen, ZJ, Baccarelli, A, Diver, WR, Gapstur, SM, Pope, CA 3rd, et al. Outdoor air pollution and cancer: an overview of the current evidence and public health recommendations. CA Cancer J Clin. (2020) 70:460–79. doi: 10.3322/caac.21632
38. Balmain, A. Air pollution's role in the promotion of lung cancer. Nature. (2023) 616:35–6. doi: 10.1038/d41586-023-00929-x
39. Lin, L, Huang, H, Lei, F, Sun, T, Chen, Z, Qin, K, et al. Long-term exposure to fine particulate constituents and vascular damage in a population with metabolic abnormality in China. J Atheroscler Thromb. (2023) 30:1552–67. doi: 10.5551/jat.64062
40. Ambler, WG, and Kaplan, MJ. Vascular damage in systemic lupus erythematosus. Nat Rev Nephrol. (2024) 20:251–65. doi: 10.1038/s41581-023-00797-8
41. Wang, Y-T, and Thomas, DD. Nitrogen oxides and their roles in cancer etiology. Curr Pharmacol Rep. (2017) 3:151–61. doi: 10.1007/s40495-017-0092-3
42. World Health Organization. WHO guidelines for indoor air quality: selected pollutants. Geneva: WHO (2010).
43. Li, J, Deng, Z, Soerensen, SJC, Kachuri, L, Cardenas, A, Graff, RE, et al. Ambient air pollution and urological cancer risk: a systematic review and meta-analysis of epidemiological evidence. Nat Commun. (2024) 15:5116. doi: 10.1038/s41467-024-48857-2
44. Wei, Y, Danesh Yazdi, M, Ma, T, Castro, E, Liu, CS, Qiu, X, et al. Additive effects of 10-year exposures to PM(2.5) and NO(2) and primary cancer incidence in American older adults. Environ Epidemiol. (2023) 7:e265. doi: 10.1097/ee9.0000000000000265
45. Sarode, SC, Sarode, GS, and Sharma, N. Outdoor air pollution and oral cancer: critical viewpoints and future prospects. Future Oncol. (2023) 19:409–11. doi: 10.2217/fon-2022-0986
46. Manisalidis, I, Stavropoulou, E, Stavropoulos, A, and Bezirtzoglou, E. Environmental and health impacts of air pollution: a review. Front Public Health. (2020) 8:14. doi: 10.3389/fpubh.2020.00014
47. Kan, H. World Health Organization air quality guidelines 2021: implication for air pollution control and climate goal in China. Chin Med J. (2022) 135:513–5. doi: 10.1097/cm9.0000000000002014
48. Yang, T, Deng, W, Liu, Y, Zhao, W, Liu, J, Cao, Y, et al. Association between ambient air pollution and laryngeal neoplasms incidence in twelve major Chinese cities, 2006-2013. Environ Sci Pollut Res Int. (2020) 27:39274–82. doi: 10.1007/s11356-020-09948-x
49. Silveyra, P, Al Housseiny, H, and Rebuli, ME. Sex and gender differences in the susceptibility to environmental exposures In: P Silveyra and XT Tigno, editors. Sex-based differences in lung physiology. Cham: Springer International Publishing (2021). 251–90.
50. Zhao, L, Liang, HR, Chen, FY, Chen, Z, Guan, WJ, and Li, JH. Association between air pollution and cardiovascular mortality in China: a systematic review and meta-analysis. Oncotarget. (2017) 8:66438–48. doi: 10.18632/oncotarget.20090
51. Pilleron, S, Sarfati, D, Janssen-Heijnen, M, Vignat, J, Ferlay, J, Bray, F, et al. Global cancer incidence in older adults, 2012 and 2035: a population-based study. Int J Cancer. (2019) 144:49–58. doi: 10.1002/ijc.31664
52. Wang, X, Yang, C, Lu, L, Bai, J, Wu, H, Chen, T, et al. Assessing the causal effect of long-term exposure to air pollution on cognitive decline in middle-aged and older adults – empirical evidence from a nationwide longitudinal cohort. Ecotoxicol Environ Saf. (2023) 255:114811. doi: 10.1016/j.ecoenv.2023.114811
Keywords: air pollution, oral cancer, distributed lag non-linear model, NO2, prevalence
Citation: Peng H, Wang X, Liao Y, Lan L, Wang D, Xiong Y, Xu L, Liang Y, Luo X, Xu Y, Li F, Chen H and Ning C (2025) Long-term exposure to ambient NO2 increase oral cancer prevalence in Southern China: a 3-year time-series analysis. Front. Public Health. 13:1484223. doi: 10.3389/fpubh.2025.1484223
Received: 21 August 2024; Accepted: 03 March 2025;
Published: 18 March 2025.
Edited by:
Tong Wang, Duke University, United StatesReviewed by:
Bruna Sinjari, University of Studies G. d’Annunzio Chieti and Pescara, ItalyCopyright © 2025 Peng, Wang, Liao, Lan, Wang, Xiong, Xu, Liang, Luo, Xu, Li, Chen and Ning. This is an open-access article distributed under the terms of the Creative Commons Attribution License (CC BY). The use, distribution or reproduction in other forums is permitted, provided the original author(s) and the copyright owner(s) are credited and that the original publication in this journal is cited, in accordance with accepted academic practice. No use, distribution or reproduction is permitted which does not comply with these terms.
*Correspondence: Chuanyi Ning, bmluZ2NodWFueWlAMTI2LmNvbQ==; Hao Chen, aGFvY2hlbnhAc3IuZ3htdS5lZHUuY24=; Feiyan Li, MjAyMDQxOTA0ODBAc3IuZ3htdS5lZHUuY24=
†These authors have contributed equally to this work
Disclaimer: All claims expressed in this article are solely those of the authors and do not necessarily represent those of their affiliated organizations, or those of the publisher, the editors and the reviewers. Any product that may be evaluated in this article or claim that may be made by its manufacturer is not guaranteed or endorsed by the publisher.
Research integrity at Frontiers
Learn more about the work of our research integrity team to safeguard the quality of each article we publish.