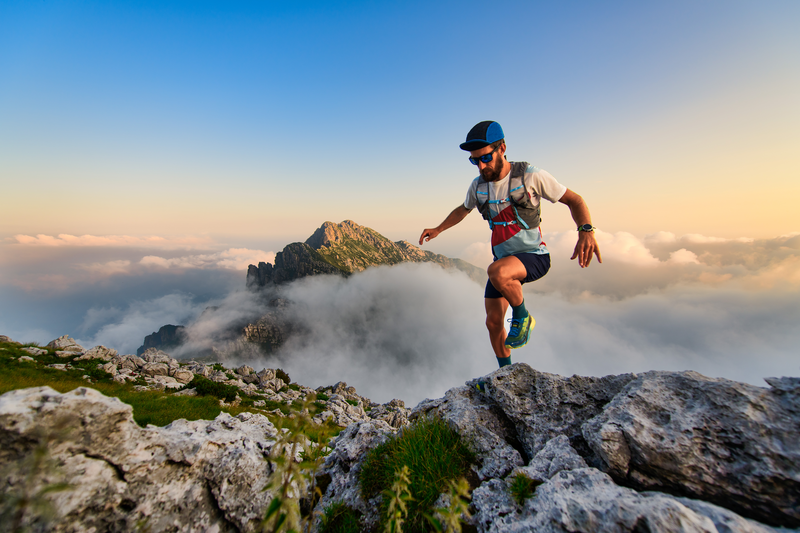
95% of researchers rate our articles as excellent or good
Learn more about the work of our research integrity team to safeguard the quality of each article we publish.
Find out more
ORIGINAL RESEARCH article
Front. Public Health , 19 March 2025
Sec. Environmental Health and Exposome
Volume 13 - 2025 | https://doi.org/10.3389/fpubh.2025.1457630
This article is part of the Research Topic Toxicity Mechanisms of Environmental Pollutants and Health Risk Assessment View all 21 articles
Background: Acrylamide (AA) is a ubiquitous environmental contaminant linked to systemic inflammation and oxidative stress in animal studies; however, the epidemiological evidence is still lacking. This study aimed to evaluate the association of AA exposure with markers of systemic inflammation and serum concentrations of an anti-aging protein, α-klotho.
Methods: The study used data of 1,545 adults aged 40–79 years from the National Health and Nutrition Examination Survey (NHANES) 2013–2016. Internal AA exposure was assessed using hemoglobin adducts of acrylamide and glycidamide (HbAA and HbGA, respectively), the sum of HbAA and HbGA (HbAA + HbGA), and the ratio of HbGA and HbAA (HbGA/HbAA). Two novel indicators, systemic immune-inflammation index (SII) and system inflammation response index (SIRI), were calculated using the lymphocyte, platelet, neutrophil, and monocyte counts. The serum concentration of soluble α-klotho was measured using enzyme-linked immunosorbent assay. Multivariable linear regression models were used to estimate the associations of AA hemoglobin biomarkers with systemic inflammation indicators and serum concentration of α-klotho.
Results: Each one-unit increase in ln-transformed HbAA, HbGA, and HbAA+HbGA was associated with an increase in SII in models adjusted for age, sex, and race/ethnicity [regression coefficient (β) = 32.16, 95% confidence interval (CI): 3.59, 60.73; β =36.37, 95% CI: 5.59, 67.15; and β = 37.17, 95% CI: 6.79, 67.55, respectively]. However, the associations were no longer significant after additional adjustment for lifestyle factors. Higher HbAA and HbAA+HbGA predicted lower serum α-klotho concentrations (β = −35.76 pg./mL, 95% CI: −63.27, −8.25; β = −33.82 pg./mL, 95% CI: −62.68, −4.96, respectively).
Conclusion: The hemoglobin adducts of AA parameters, as biomarkers of internal AA exposure, were associated with reduced serum concentrations of α-klotho among the United States population in their middle-late adulthood. The findings indicated that exposure to AA may have impacts on the molecular pathways of aging and related diseases by influencing α-klotho concentrations.
Acrylamide (AA) is a reactant extensively used to synthesize polyacrylamide polymers, gels, and binding agents (1). AA has attracted public attention in the last decades because it can be developed via Maillard reaction during food processing at high temperatures, such as frying and baking (2). Meanwhile, it is also found in the smoke generated when tobacco burns in a lit cigarette (3). Thus, AA can be absorbed into the body through ingestion, inhalation, and dermal contact with AA-containing products (3, 4). Diet contributes to an average daily intake of 0.02–1.53 μg/(kg body weight · day) AA for the general population (5). Once absorbed, AA is widely distributed to various organs and metabolized to a major metabolite, glycidamide (GA), in the liver (6). Hemoglobin adducts of AA (HbAA) and GA (HbGA) are validated biomarkers in human biomonitoring and commonly found in the United States (US) population (7). The ubiquitous presence of AA has raised health concerns worldwide owing to its toxicological effects (1, 2).
AA exposure has been related to various adverse health outcomes, such as cancer (8, 9), cardiovascular diseases (10), respiratory diseases (11), diabetes (12), and depression (13, 14). It impacts human health through multiple mechanisms. Particularly, AA exposure increases systemic inflammation (2). In vitro and in vivo studies indicated that AA treatment activated the nuclear factor-κB (NF-κB) pathway and enhanced the release of pro-inflammatory cytokines (15, 16). However, evidence of AA exposure associated with systemic inflammation in humans is still scarce. Recently, two novel indicators derived from lymphocyte, neutrophil, monocyte, and platelet counts were introduced: the systemic immune-inflammation index (SII) and the system inflammation response index (SIRI) (17). Initially, SII was used to assess the prognosis of patients with liver cancer, whereas SIRI predicted survival after chemotherapy in patients with cancer (18, 19). These indicators were widely used for evaluating systemic inflammation response in the general population because of their easy access and biological significance (20, 21).
Another toxicological mechanism of AA-associated health outcomes is oxidative stress damage. AA or GA contributes to the depletion of glutathione, overproduction of reactive oxygen species (ROS), and alteration of the nuclear factor erythroid 2-related factor 2 pathway (22). Oxidative stress may be inhibited by soluble α-klotho, which is a transmembrane protein related to the aging process (23). α-klotho downregulates ROS-associated stress and prolongs cellular lifespan (24, 25). It also maintains the anti-aging process and prevents aging-related diseases. Therefore, exploring a potential link between AA exposure and soluble α-klotho may have significant public health implications.
AA exposure induces systemic inflammation and oxidative stress in animals (1); however, the epidemiological evidence related to this is quite limited. Previous epidemiological studies suggested that hemoglobin or urinary biomarkers of AA and GA were associated with increased levels of pro-inflammatory cytokines and inflammatory markers, including low-grade inflammation score (INFLA-score), C-reactive protein (CRP), circulating mean platelet volume (MPV), and high-sensitivity interleukin-6 (IL-6) (10, 26–28). However, few studies have addressed the associations of HbAA and HbGA with novel markers of systemic inflammation and serum concentrations of α-klotho. Therefore, this study aimed to explore the associations of AA exposure with markers of systemic inflammation and serum concentrations of α-klotho in general adults aged 40–79 years using the National Health and Nutrition Examination Survey (NHANES) 2013–2016 cycles.
The study data were extracted from the NHANES database (29). NHANES is a population-based survey aiming to evaluate the health and nutrition of participants in the US. This nationally representative survey included physical examinations, laboratory tests, dietary information, and health-related questionnaires. The NHANES team captured informed consent from each participant prior to enrollment. The study protocol (Protocol #2011–17) was reviewed and approved by the NCHS Research Ethics Review Board.
The NHANES 2013–2014 and 2015–2016 cycles were selected owing to data availability. A total of 20,146 participants were enrolled in NHANES 2013–2016 cycles (Figure 1). Adults aged 40–79 years were included (N = 6,853). Pregnant women were excluded at the examination (N = 7). Furthermore, participants with missing blood cell counts (N = 478), missing HbAA and HbGA measurements (N = 4,495), and missing α-klotho concentrations in serum (N = 328) were excluded from the analysis.
The concentrations of HbAA and HbGA in human whole blood or erythrocytes were measured (7) as described in a previous study (30). Briefly, the adducts of AA and GA were cleaved using a modified Edman reaction. The Edman products were prepared by liquid–liquid extraction and quantified using high-performance liquid chromatography–tandem mass spectrometry. The limit of detection of AA and GA was 3.90 pmol/g Hb and 4.90 pmol/g Hb, respectively. Laboratory quality assurance and quality control protocols are available on the NHANES website (29).
The whole blood specimens were analyzed at NHANES mobile examination centers using automated hematology analyzing devices (29). After analysis in duplicate, the observed results were averaged to improve the data quality. We calculated SII using the counts of peripheral blood cells (1,000 cells/μL):
SIRI was also calculated as follows (19):
Serum concentrations of soluble α-klotho from the participants were quantified using an extensively validated IBL enzyme-linked immunosorbent assay method (31). The sensitivity of the assay was 6 pg./mL. The samples were analyzed in duplicate to ensure the precision. The final values were calculated using the average of the two observed values.
As reported in previous studies (10, 28), several potential confounding factors in relation to AA exposure and systemic inflammation/α-klotho were considered, including socio-demographic characteristics, physical examination, dietary information, and lifestyles. The covariables were included if they changed the coefficient of AA hemoglobin biomarkers by greater than 10% in simple linear regression models. The following covariables were selected: sex, age, race/ethnicity, educational level, family poverty-income ratio (PIR), body mass index (BMI), cigarette smoking (smoker or non-smoker), alcohol consumption (days per year) and physical activity (minutes per week). Cigarette smoking was assessed by individual’s self-report. Smoker was defined as participants who smoked at least 100 cigarettes in life. In the smoking subgroup analysis, an additional continuous variable of an average number of cigarettes smoked per day over the past 30 days was adjusted in the models for smokers. A continuous variable of alcohol consumption was generated from three components: consumption of at least 12 alcohol drinks/lifetime (yes or no), frequency of drinking alcohol over past 12 months (0 to 365) and days of alcohol consumption per week, month, or year. “0” was assigned to individuals who did not have at least 12 alcohol drinks/lifetime. The amount of alcohol consumption was calculated by day × frequency for those who had 12 or more alcohol drinks. Physical activity was calculated as the total weekly minutes of vigorous work activities, moderate work activities, walking or bicycling, vigorous recreational activities, and moderate recreational activities (32).
The general characteristics of participants were summarized using the median and interquartile range for continuous variables and frequency and proportion for categorical variables. HbAA, HbGA, HbAA + HbGA, and HbGA/HbAA were natural logarithm (ln) transformed owing to the skewed distribution of residuals. Spearman correlation coefficients were calculated to evaluate pairwise correlations of AA hemoglobin indicators. Multiple imputations with chained equations were applied for a few missing covariables, including family PIR, BMI, cigarette smoking, and alcohol consumption.
Multivariable linear regression models were used to explore the associations of AA hemoglobin biomarkers with SII, SIRI, and serum concentration of α-klotho. The collinearity of the linear regression models was assessed using a variance inflation factor, revealing no multi-collinearity. Regarding covariables, three models were used. Model 1 was a crude model without any adjustment. Model 2 was a basic model adjusted for sex, age, and race/ethnicity. Model 3 was adjusted for all the aforementioned covariables as the primary model. Generalized additive models with 3-degrees-of-freedom natural cubic splines were fitted to estimate the potential nonlinear associations of AA hemoglobin biomarkers with markers of systemic inflammation and serum concentrations of α-klotho. Tobacco smoke is a major source of AA exposure (33). Therefore, an interaction term between cigarette smoking and target biomarkers (data shown in Supplementary Table S1) was further introduced, and then stratified analysis based on cigarette smoking was performed.
Statistical analysis was performed using Stata version 17.0 (Stata Corp, TX, United States) and R version 4.2.1.1 Statistical significance was considered as a two-sided p < 0.05 and p < 0.10 for interaction terms.
A total of 1,545 participants were included in the present analysis. The general characteristics and outcomes are presented in Table 1. The frequency and proportion of adults aged 40–59 years were 851 and 55.1%, respectively. A majority of participants were non-Hispanic White (39.7%) and had higher educational levels (54.0%).
Table 1. Descriptive statistics of general characteristics of 1,545 participants from NHANES 2013–2016.
HbAA and HbGA were detected in all the samples. The median values (25th percentile, 75th percentile) of HbAA, HbGA, and HbAA + HbGA were 41.5 (32.2, 60.6) pmol/g Hb and 36.2 (26.7, 51.0) pmol/g Hb and 78.2 (60.1, 111) pmol/g Hb, respectively. The median value of HbGA/HbAA was 0.822 (0.689, 0.982) (Table 1). HbAA and HbGA were highly correlated with a Spearman correlation coefficient of 0.822 (p < 0.001). The median (25th percentile, 75th percentile) SII and SIRI values were 455 (332, 623) and 1.07 (0.75, 1.58), respectively. A high correlation was observed between SII and SIRI (Spearman correlation coefficient: 0.747, p < 0.001). The median α-klotho concentration in serum was 779 (649, 961) pg./mL (Table 1).
Nonlinear and linear associations between AA hemoglobin biomarkers and markers of systemic inflammation are displayed in Figure 2 and Table 2, respectively. No evidence of statistically significant nonlinear associations between AA hemoglobin biomarkers and systemic inflammation markers was found (p nonlinearity > 0.05, Figure 2). HbAA, HbGA, and HbAA + HbGA were significantly positively correlated with SII and SIRI in the crude models (Model 1) and basic adjusted models (Model 2). No statistically significant association between AA hemoglobin biomarkers and SII or SIRI was observed after adjusting for potential confounders in Model 3.
Figure 2. Dose–response relationships of AA hemoglobin biomarkers and markers of systemic inflammation and α-Klotho concentrations in serum. (A) HbAA and SII; (B) HbAA+HbGA and SII; (C) HbAA and SIRI; (D) HbAA+HbGA and SIRI; (E) HbAA and α-Klotho; (F) HbAA+HbGA and α-Klotho. LNAA, ln-transformed HbAA; LNAA+LNGA, ln-transformed HbAA+HbGA. The dose–response relationships were assessed by generalized additive models with adjustment for age, sex, race/ethnicity, educational level, body mass index, family poverty income ratio, cigarette smoking, alcohol consumption, and physical activity.
Table 2. Estimated regression coefficients and 95% CI for markers of systemic inflammation and AA hemoglobin biomarkers.
Table 3 illustrates the associations of AA hemoglobin biomarkers with serum concentrations of α-klotho. Higher HbAA concentration in whole blood was statistically significantly related to decreased serum concentrations of α-klotho (β = −35.76 pg./mL, 95% CI: −63.27, −8.25; p = 0.011), after adjustment for potential confounders. A negative association between HbAA + HbGA and serum concentrations of α-klotho was also observed (β = −33.82 pg./mL, 95% CI: −62.68, −4.96; p = 0.022).
Table 3. Estimated regression coefficients and 95% CI for serum α-Klotho concentrations and AA hemoglobin biomarkers.
The interaction term based on the multiplication of cigarette smoking and AA hemoglobin biomarkers was statistically significant in the associations between HbAA/HbGA and SII, as well as between HbAA+HbGA and α-klotho (Supplementary Table S1). After stratification by cigarette smoking, HbAA and HbAA + HbGA were borderline significantly associated with decreased serum concentrations of α-klotho only in smokers (β = −40.69 pg./mL, 95% CI: −87.15, 5.77; p = 0.086; β = −39.88 pg./mL, 95% CI: −88.23, 8.47; p = 0.106, respectively). No statistically significant association was observed with other AA hemoglobin biomarkers in the stratified analyses (Figure 3).
Figure 3. Stratified analysis by cigarette smoking for associations of AA hemoglobin biomarkers with SIRI (A) or serum α-Klotho concentrations (B). SIRI, system inflammation response index. Models for smokers were adjusted for sex, age, race/ethnicity, educational level, family poverty-income ratio, body mass index, average cigarettes per day during past 30 days, alcohol consumption and physical activity. Models for non-smokers were adjusted for sex, age, race/ethnicity, educational level, family poverty-income ratio, body mass index, alcohol consumption, and physical activity.
This cross-sectional analysis revealed negative associations of HbAA and HbAA + HbGA with serum concentrations of α-klotho; the associations were more pronounced in smokers. HbAA and HbAA + HbGA showed no association with SII and SIRI, as calculated using blood cell counts.
Several epidemiological studies have addressed the association of AA exposure at environmentally relevant doses with systemic inflammation measured using various indicators. Our findings were consistent with those of previous studies (10, 26–28). In a pilot study, 14 healthy volunteers (6 smokers) were administered 160 g/day AA-containing potato chips (27). After 4 weeks, the concentrations of inflammation markers, plasma high-sensitivity CRP, high-sensitivity IL-6, gamma-glutamyltransferase significantly increased (p < 0.10) compared with baseline (before consumption) among smokers and nonsmokers (27). The urinary AA biomarkers in a Chinese population were significantly associated with increased concentrations of systemic inflammatory marker plasma CRP (28). This increase in plasma CRP concentration mediated 6.34–11.1% of the associations of urinary AA biomarkers with reduced pulmonary function. In a prospective study, Wang et al. (10) reported an association of the urinary AA biomarkers with 10-year cardiovascular disease risks in general adults, mediated by systemic inflammation (plasma CRP and circulating MPV), oxidative stress, and plasma transforming growth factor-β1. In NHANES 2003–2014 cycles, AA hemoglobin biomarkers were related to an increase in cancer mortality (mediated by low-grade INFLA-score), an inflammatory marker derived from CRP, white blood cell and platelet counts, and granulocyte/lymphocyte ratio (26). On the contrary, the present study indicated that AA exposure might not increase systemic inflammation in general adults. The reasons for inconsistent findings may be due to the heterogeneity between populations, outcome measurements, and time window of assessment. We cannot be ruled out that manifestations of inflammatory effects of AA may be temporarily masked by compensatory processes in this population and maybe become apparent in other study population. Moreover, CRP used in the previous studies may be a more sensitive biomarker in measuring inflammation, compared with SII and SIRI (21). Furthermore, given the short half-life of HbAA in humans, there may be exposure misclassification and the relevant time window for AA exposure and inflammation may not overlap.
Both in vitro and in vivo experimental studies have indicated that AA exposure increased inflammation in various tissues, including the neurons, brain, liver, and kidney (1). AA exposure induced an inflammatory response in vitro via the nuclear factor-κB (NF-κB) pathway in human PC12 cells (16). The transcription of inflammatory genes was enhanced after NF-κB activation, and pro-inflammatory cytokines, such as tumor necrosis factor-α (TNF-α), interleukin 6 (IL-6), pro-IL-1β, and pro-IL-18, were released. These findings were further observed in rodent models. AA treatment enhanced the serum concentrations of cytokines, including TNF-α, pro-IL-1β, and IL-6 (15, 34).
Several epidemiological studies have reported that reductions in serum α-Klotho levels were associated with several environmental contaminants, including heavy metals (35), perfluoroalkyl and polyfluoroalkyl substances (36), and polycyclic aromatic hydrocarbons (37). The exact biological mechanisms of action of AA exposure on the reduction in serum concentrations of α-klotho are still unclear. The most possible underlying mechanism was AA-induced oxidative stress. Several animal and epidemiological studies showed that AA exposure increases the levels of oxidative stress markers, such as urinary 8-hydroxydeoxyguanosine and 8-iso-prostaglandin-F2α (10, 15). The α-klotho stimulation upregulated the expression of phosphorylation forkhead box protein O3a, inhibiting ROS-related oxidative stress damage (24). ROS production and oxidative stress damage were negatively correlated with serum concentrations of α-klotho (25).
Stratified analyses revealed more prominent associations between AA exposure and serum concentrations of α-klotho in smokers than nonsmokers. This was also observed previously in associations of AA exposure with other health-related outcomes, including diabetes (12), cardiovascular diseases (38, 39), depressive symptoms (13). Cigarette smoking, a critical source of AA exposure, was associated with increases in local and systemic inflammation (40) and a reduction in serum concentrations of α-klotho (3, 41). Smokers had higher exposure levels of AA and other toxic chemicals, such as tar, formaldehyde, polycyclic aromatic hydrocarbons, and heavy metals (40), compared with nonsmokers. We cannot exclude the possibility that AA in combination with a series of toxic chemicals in tobacco smoke contributes to the decrease in serum concentrations of α-klotho in smokers. Residual confounding by smoking may also play a role in association between AA exposure and serum α-klotho concentrations. More epidemiological studies should be conducted to assess the exposure to a mixture of toxic chemicals associated with systemic inflammation and biological aging among active smokers.
This epidemiological study was novel in exploring the associations of internal AA exposure with novel systemic inflammation markers and serum concentrations of α-klotho in the general population. However, this study had several limitations. First, causality could not be inferred between AA exposure and systemic inflammation or serum concentrations of α-klotho owing to the observational study design, especially for the cross-sectional study design. Second, despite adjusting for a broad set of covariables, we could not exclude the possibility of residual confounders, such as occupational factors (42), and other environmental contaminants (35, 36). These may have confounding effects on the exposure-outcome associations. Third, HbAA biomarkers were assessed only once and thus reflect AA exposure over a short time window (42). This single measurement may lead to exposure misclassification, as the relevant time window for AA exposure and inflammation may not overlap. Repeated measurements would have provided a more accurate or long-term assessment of exposure levels.
AA exposure assessed using hemoglobin biomarkers was associated with decreased serum concentrations of α-klotho in general adults aged 40–79 years. The findings of this study provide suggestive evidence regarding the potential health effects of AA exposure at environmentally relevant doses. Future studies are warranted to identify potential biological mechanisms and develop intervention strategies.
The raw data supporting the conclusions of this article will be made available by the authors, without undue reservation.
The study protocol (Protocol #2011–17) was reviewed and approved by the NCHS Research Ethics Review Board (ERB). The studies were conducted in accordance with the local legislation and institutional requirements. The participants provided their written informed consent to participate in this study.
LG: Conceptualization, Data curation, Formal analysis, Methodology, Writing – original draft, Writing – review & editing. JW: Data curation, Formal analysis, Methodology, Software, Supervision, Writing – review & editing. KQ: Data curation, Formal analysis, Supervision, Writing – review & editing. WJ: Conceptualization, Formal analysis, Supervision, Writing – review & editing. YL: Conceptualization, Formal analysis, Writing – review & editing. MD: Conceptualization, Funding acquisition, Project administration, Supervision, Writing – review & editing.
The author(s) declare that financial support was received for the research and/or publication of this article. This work was supported by the Jilin Provincial Health Science and Technology Capacity Enhancement Program Project (2024A015)and the National Key Research and Development Program of China (2023YFC2508701).
We appreciated the adults for participation in the NHANES survey. We are also grateful for the U.S. Centers for Disease Control and Prevention (CDC) for providing the publicly available data.
The authors declare that the research was conducted in the absence of any commercial or financial relationships that could be construed as a potential conflict of interest.
All claims expressed in this article are solely those of the authors and do not necessarily represent those of their affiliated organizations, or those of the publisher, the editors and the reviewers. Any product that may be evaluated in this article, or claim that may be made by its manufacturer, is not guaranteed or endorsed by the publisher.
The Supplementary material for this article can be found online at: https://www.frontiersin.org/articles/10.3389/fpubh.2025.1457630/full#supplementary-material
1. Yan, F, Wang, L, Zhao, L, Wang, C, Lu, Q, and Liu, R. Acrylamide in food: occurrence, metabolism, molecular toxicity mechanism and detoxification by phytochemicals. Food Chem Toxicol. (2023) 175:113696. doi: 10.1016/j.fct.2023.113696
2. Koszucka, A, Nowak, A, Nowak, I, and Motyl, I. Acrylamide in human diet, its metabolism, toxicity, inactivation and the associated European Union legal regulations in food industry. Crit Rev Food Sci Nutr. (2020) 60:1677–92. doi: 10.1080/10408398.2019.1588222
3. Esposito, F, Squillante, J, Nolasco, A, Montuori, P, Macri, PG, and Cirillo, T. Acrylamide levels in smoke from conventional cigarettes and heated tobacco products and exposure assessment in habitual smokers. Environ Res. (2022) 208:112659. doi: 10.1016/j.envres.2021.112659
4. Liao, KW, Chang, FC, Chang, CH, Huang, YF, Pan, WH, and Chen, ML. Associating acrylamide internal exposure with dietary pattern and health risk in the general population of Taiwan. Food Chem. (2022) 374:131653. doi: 10.1016/j.foodchem.2021.131653
5. Timmermann, CAG, Molck, SS, Kadawathagedara, M, Bjerregaard, AA, Tornqvist, M, Brantsaeter, AL, et al. A review of dietary intake of acrylamide in humans. Toxics. (2021) 9:155. doi: 10.3390/toxics9070155
6. Sumner, SC, Williams, CC, Snyder, RW, Krol, WL, Asgharian, B, and Fennell, TR. Acrylamide: a comparison of metabolism and hemoglobin adducts in rodents following dermal, intraperitoneal, oral, or inhalation exposure. Toxicol Sci. (2003) 75:260–70. doi: 10.1093/toxsci/kfg191
7. Vesper, HW, Caudill, SP, Osterloh, JD, Meyers, T, Scott, D, and Myers, GL. Exposure of the U.S. population to acrylamide in the National Health and nutrition examination survey 2003-2004. Environ Health Perspect. (2010) 118:278–83. doi: 10.1289/ehp.0901021
8. Filippini, T, Halldorsson, TI, Capitão, C, Martins, R, Giannakou, K, Hogervorst, J, et al. Dietary acrylamide exposure and risk of site-specific cancer: a systematic review and dose-response Meta-analysis of epidemiological studies. Front Nutr. (2022) 9:875607. doi: 10.3389/fnut.2022.875607
9. Pelucchi, C, Bosetti, C, Galeone, C, and La Vecchia, C. Dietary acrylamide and cancer risk: an updated meta-analysis. Int J Cancer. (2015) 136:2912–22. doi: 10.1002/ijc.29339
10. Wang, B, Wang, X, Yu, L, Liu, W, Song, J, Fan, L, et al. Acrylamide exposure increases cardiovascular risk of general adult population probably by inducing oxidative stress, inflammation, and TGF-beta1: a prospective cohort study. Environ Int. (2022) 164:107261. doi: 10.1016/j.envint.2022.107261
11. Liu, S, Ben, X, Liang, H, Fei, Q, Guo, X, Weng, X, et al. Association of acrylamide hemoglobin biomarkers with chronic obstructive pulmonary disease in the general population in the US: NHANES 2013-2016. Food Funct. (2021) 12:12765–73. doi: 10.1039/D1FO02612G
12. Yin, G, Liao, S, Gong, D, and Qiu, H. Association of acrylamide and glycidamide haemoglobin adduct levels with diabetes mellitus in the general population. Environ Pollut. (2021) 277:116816. doi: 10.1016/j.envpol.2021.116816
13. Li, Z, Sun, J, and Zhang, D. Association between acrylamide hemoglobin adduct levels and depressive symptoms in US adults: NHANES 2013-2016. J Agric Food Chem. (2021) 69:13762–71. doi: 10.1021/acs.jafc.1c04647
14. Wang, A, Wan, X, Zhuang, P, Jia, W, Ao, Y, Liu, X, et al. (2023) high fried food consumption impacts anxiety and depression due to lipid metabolism disturbance and neuroinflammation. Proc Natl Acad Sci USA. (2023) 120:e2221097120. doi: 10.1073/pnas.2221097120
15. Alturfan, AA, Tozan-Beceren, A, Sehirli, AO, Demiralp, E, Sener, G, and Omurtag, GZ. Resveratrol ameliorates oxidative DNA damage and protects against acrylamide-induced oxidative stress in rats. Mol Biol Rep. (2012) 39:4589–96. doi: 10.1007/s11033-011-1249-5
16. Pan, X, Wu, X, Yan, D, Peng, C, Rao, C, and Yan, H. Acrylamide-induced oxidative stress and inflammatory response are alleviated by N-acetylcysteine in PC12 cells: involvement of the crosstalk between Nrf2 and NF-κB pathways regulated by MAPKs. Toxicol Lett. (2018) 288:55–64. doi: 10.1016/j.toxlet.2018.02.002
17. Luo, S, Liu, Z, Jiao, R, Li, W, Sun, J, Ma, S, et al. The associations of two novel inflammation indexes, systemic immune-inflammation index (SII) and system inflammation response index (SIRI), with periodontitis: evidence from NHANES 2009-2014. Clin Oral Investig. (2024) 28:129. doi: 10.1007/s00784-024-05529-1
18. Hu, B, Yang, XR, Xu, Y, Sun, YF, Sun, C, Guo, W, et al. Systemic immune-inflammation index predicts prognosis of patients after curative resection for hepatocellular carcinoma. Clin Cancer Res. (2014) 20:6212–22. doi: 10.1158/1078-0432.CCR-14-0442
19. Qi, Q, Zhuang, L, Shen, Y, Geng, Y, Yu, S, Chen, H, et al. A novel systemic inflammation response index (SIRI) for predicting the survival of patients with pancreatic cancer after chemotherapy. Cancer. (2016) 122:2158–67. doi: 10.1002/cncr.30057
20. Wang, P, Guo, X, Zhou, Y, Li, Z, Yu, S, Sun, Y, et al. Monocyte-to-high-density lipoprotein ratio and systemic inflammation response index are associated with the risk of metabolic disorders and cardiovascular diseases in general rural population. Front Endocrinol. (2022) 13:944991. doi: 10.3389/fendo.2022.944991
21. Xia, Y, Xia, C, Wu, L, Li, Z, Li, H, and Zhang, J. Systemic immune inflammation index (SII), system inflammation response index (SIRI) and risk of all-cause mortality and cardiovascular mortality: a 20-year follow-up cohort study of 42,875 US adults. J Clin Med. (2023) 12:1128. doi: 10.3390/jcm12031128
22. Gao, H, Xue, Y, Wu, L, Huo, J, Pang, Y, Chen, J, et al. Protective effect of Lycium ruthenicum polyphenols on oxidative stress against acrylamide induced liver injury in rats. Molecules. (2022) 27:4100. doi: 10.3390/molecules27134100
23. Kuro, OM. The klotho proteins in health and disease. Nat Rev Nephrol. (2019) 15:27–44. doi: 10.1038/s41581-018-0078-3
24. Brooke, M, Emerling 1, FW, Liu, J-L, Mak, TW, and Chandel, NS. PTEN regulates p300-dependent hypoxia-inducible factor 1 transcriptional activity through Forkhead transcription factor 3a (FOXO3a). Proc Natl Acad Sci USA. (2008) 105:2622–7. doi: 10.1073/pnas.0706790105
25. Hsieh, CC, Kuro-o, M, Rosenblatt, KP, Brobey, R, and Papaconstantinou, J. The ASK1-signalosome regulates p38 MAPK activity in response to levels of endogenous oxidative stress in the klotho mouse models of aging. Aging. (2010) 2:597–611. doi: 10.18632/aging.100194
26. Gu, W, Zhang, J, Ren, C, Gao, Y, Zhang, T, Long, Y, et al. The association between biomarkers of acrylamide and cancer mortality in U.S. adult population: evidence from NHANES 2003-2014. Front Oncol. (2022) 12:970021. doi: 10.3389/fonc.2022.970021
27. Naruszewicz, M, Zapolska-Downar, D, Kosmider, A, Nowicka, G, Kozlowska-Wojciechowska, M, Vikstrom, AS, et al. Chronic intake of potato chips in humans increases the production of reactive oxygen radicals by leukocytes and increases plasma C-reactive protein: a pilot study. Am J Clin Nutr. (2009) 89:773–7. doi: 10.3945/ajcn.2008.26647
28. Wang, B, Wang, X, Yang, S, Cheng, M, Zhou, Y, Zhou, M, et al. Acrylamide exposure and pulmonary function reduction in general population: the mediating effect of systemic inflammation. Sci Total Environ. (2021) 778:146304. doi: 10.1016/j.scitotenv.2021.146304
29. Centers for Disease Control and Prevention (CDC). National Health and Nutrition Examination Survey (NHANES). Available online at: https://www.cdc.gov/nchs/nhanes/index.htm (Accessed January 2, 2024).
30. Vesper, HWSN, Hallmans, G, Tjonneland, A, Agudo, A, and Benetou, V. Cross-sectional study on acrylamide hemoglobin adducts in subpopulations from the European prospective investigation into Cancer and nutrition (EPIC) study. J Agric Food Chem. (2008) 56:6046–53. doi: 10.1021/jf703750t
31. Yamazaki, Y, Urakawa, AI, Shimada, T, Murakami, J, Aono, Y, Hasegawa, H, et al. Establishment of a sandwich ELISA for soluble alpha-klotho measurements: age-dependent change of soluble alpha-klotho levels in healthy subjects. Biochem Biophys Res Comm. (2017) 398:513–8. doi: 10.1016/j.bbrc.2010.06.110
32. Dong, JX, Jiang, LL, Liu, YP, and Zheng, AX. Association between composite dietary antioxidant index and metabolic dysfunction-associated fatty liver disease: a cross-sectional study from NHANES. BMC Gastroenterol. (2024) 24:465. doi: 10.1186/s12876-024-03556-6
33. Kenwood, BM, Zhu, W, Zhang, L, Bhandari, D, and Blount, BC. Cigarette smoking is associated with acrylamide exposure among the U.S. population: NHANES 2011-2016. Environ Res. (2022) 209:112774. doi: 10.1016/j.envres.2022.112774
34. Zhang, L, Wang, E, Chen, F, Yan, H, and Yuan, Y. Potential protective effects of oral administration of allicin on acrylamide-induced toxicity in male mice. Food Funct. (2013) 4:1229–36. doi: 10.1039/c3fo60057b
35. Kim, D, Lee, S, Choi, JY, Lee, J, Lee, HJ, Min, JY, et al. Association of alpha-klotho and lead and cadmium: a cross-sectional study. Sci Total Environ. (2022) 843:156938. doi: 10.1016/j.scitotenv.2022.156938
36. Li, M, Ma, Y, Cheng, W, Zhang, L, Zhou, C, Zhang, W, et al. Association between perfluoroalkyl and polyfluoroalkyl internal exposure and serum alpha-klotho levels in middle-old aged participants. Front Public Health. (2023) 11:1136454. doi: 10.3389/fpubh.2023.1136454
37. Chen, YY, and Chen, WL. The relationship between polycyclic aromatic hydrocarbons exposure and serum klotho among adult population. BMC Geriatr. (2022) 22:198. doi: 10.1186/s12877-022-02924-9
38. Wang, B, Cheng, M, Yang, S, Qiu, W, Li, W, Zhou, Y, et al. Exposure to acrylamide and reduced heart rate variability: the mediating role of transforming growth factor-β. J Hazard Mater. (2020) 395:122677. doi: 10.1016/j.jhazmat.2020.122677
39. Zhang, Y, Huang, M, Zhuang, P, Jiao, J, Chen, X, Wang, J, et al. Exposure to acrylamide and the risk of cardiovascular diseases in the National Health and nutrition examination survey 2003-2006. Environ Int. (2018) 117:154–63. doi: 10.1016/j.envint.2018.04.047
40. Johannsen, A, Susin, C, and Gustafsson, A. Smoking and inflammation: evidence for a synergistic role in chronic disease. Periodontol. (2014) 64:111–26. doi: 10.1111/j.1600-0757.2012.00456.x
41. Du, R, Tang, X, Jiang, M, Qian, S, Yang, L, Tong, X, et al. Association between cigarette smoking and serum alpha klotho levels among US adults over 40-years-old: a cross-sectional study. Sci Rep. (2023) 13:19519. doi: 10.1038/s41598-023-46698-5
Keywords: acrylamide, glycidamide, systemic immune-inflammation index, system inflammation response index, α-klotho
Citation: Gan L, Wang J, Qu K, Jiang W, Lei Y and Dong M (2025) Association of acrylamide exposure with markers of systemic inflammation and serum alpha-klotho concentrations in middle-late adulthood. Front. Public Health. 13:1457630. doi: 10.3389/fpubh.2025.1457630
Received: 01 July 2024; Accepted: 10 March 2025;
Published: 19 March 2025.
Edited by:
Pu Xia, University of Birmingham, United KingdomReviewed by:
Janneke Hogervorst, University of Hasselt, BelgiumCopyright © 2025 Gan, Wang, Qu, Jiang, Lei and Dong. This is an open-access article distributed under the terms of the Creative Commons Attribution License (CC BY). The use, distribution or reproduction in other forums is permitted, provided the original author(s) and the copyright owner(s) are credited and that the original publication in this journal is cited, in accordance with accepted academic practice. No use, distribution or reproduction is permitted which does not comply with these terms.
*Correspondence: Ming Dong, ZG9uZ2dlQGpsdS5lZHUuY24=
Disclaimer: All claims expressed in this article are solely those of the authors and do not necessarily represent those of their affiliated organizations, or those of the publisher, the editors and the reviewers. Any product that may be evaluated in this article or claim that may be made by its manufacturer is not guaranteed or endorsed by the publisher.
Research integrity at Frontiers
Learn more about the work of our research integrity team to safeguard the quality of each article we publish.