- 1The Second Affiliated Hospital of Soochow University, Suzhou, China
- 2Suzhou Medical College, Soochow University, Suzhou, China
- 3State Key Laboratory of Radiation Medicine and Protection, School of Radiation Medicine and Protection, Collaborative Innovation Center of Radiological Medicine of Jiangsu Higher Education Institutions, Soochow University, Suzhou, China
Background: Hypothyroidism is a common sequela after radiotherapy for nasopharyngeal carcinoma (NPC). Magnetic resonance imaging (MRI) has gained prominence in thyroid imaging, leveraging its non-ionizing radiation, high spatial resolution, multiparameter and multidirectional imaging. Few previous studies have investigated the evaluation of radiation-induced thyroid injury by MRI.
Methods: MRI and radiotherapy data of 32 patients who were first diagnosed with nasopharyngeal carcinoma in our hospital from April 2015 to April 2024 and underwent radiotherapy in the radiotherapy department were retrospectively collected. Before, during and after radiotherapy, the thyroid morphology was observed on MR images, and the quantitative parameters of size (width, thickness) were measured on T1-weighted images. The signal intensity (SI) of the thyroid gland was measured on T1-weighted imaging (T1WI), T2-weighted imaging (T2WI) and contrast-enhanced T1-weighted imaging. The differences in thyroid parameters at different time points before and after radiotherapy were compared. The correlation between the MRI quantitative parameters of the thyroid and the radiation dose volume of the thyroid and the radiation dose of the pituitary were analyzed.
Results: The width, thickness and volume of the thyroid decreased gradually before, during and 6 and 12 months after radiotherapy. They were negatively correlated with the mean thyroid dose and V50 (p < 0.05), but were not significantly correlated with the maximum and minimum thyroid doses, V30 and V35 (p > 0.05). The T1WI relative signal intensity (RSI), T2WI RSI, and enhanced T1WI RSI of the thyroid gland gradually decreased from before radiotherapy to during radiotherapy and 6 months and 12 months after radiotherapy. The T1WI RSI, T2WI RSI, and enhanced T1WI RSI during radiotherapy and 6 months and 12 months after radiotherapy were negatively correlated with the mean radiation dose, V40, V45, and V50 of the thyroid gland (p < 0.05), but were not significantly correlated with the maximum radiation dose, minimum radiation dose, V30, and V35 of the thyroid gland or the radiation dose of the pituitary gland (p > 0.05).
Conclusion: Quantitative MRI analysis can non-invasively and effectively show the changes in thyroid shape, size and signal intensity in patients with nasopharyngeal carcinoma before and after radiotherapy, which is crucial for early and accurate assessment of thyroid damage, enabling timely treatment to preserve thyroid function.
Introduction
Nasopharyngeal carcinoma (NPC) is one of the most common malignant tumors in southern China and Southeast Asia; it accounts for 78.29% of head and neck malignant tumors (1). Intensity-modulated radiotherapy (IMRT) has the advantages of high positioning accuracy and radiation accuracy, which can increase the radiation dose for complex tumor targets while reducing the irradiation dose of surrounding normal tissues (2). It has become the first choice of treatment for nasopharyngeal carcinoma (3, 4). However, even in the IMRT era, NPC survivors still face long-term sequelae which negatively affect their quality of life (5). Hypothyroidism (HT), as one of the most common late toxicities, has been reported to occur in 40–50% of patients who were treated with neck irradiation (6–8). Therefore, the prevention and monitoring of radiotherapy-induced hypothyroidism is particularly important in the era of IMRT for nasopharyngeal carcinoma treatment (9).
Laboratory assessment and ultrasonography examination are the main evaluation methods of thyroid function. However, the former is often delayed due to the concealed clinical symptoms and the neglect of doctors and patients, and the disadvantage of the latter is that the accuracy of results is greatly affected by operators. MRI is the preferred routine examination method for patients with nasopharyngeal carcinoma before and after radiotherapy. It has good soft tissue contrast, multiparameter and multidirectional imaging, and the contrast agent is generally gadolinium, which has little effect on thyroid function.
At present, there is no MRI-related literature on radiation injury of the thyroid. The aim of this study is to measure and analyze the changes in thyroid morphology and signal intensity in patients with nasopharyngeal carcinoma before, during and after radiotherapy on 3.0 T MRI and to explore the correlation between the changes and the dose volume of the thyroid and pituitary gland. Thus, this study could provide another valuable evaluation method for the clinical diagnosis and prevention of nasopharyngeal carcinoma.
Materials and methods
The patient
This retrospective study was approved by the medical ethics committee of the hospital. From April 2015 to April 2024, 32 patients (14 women and 18 men; mean age 54.7 ± 12.3 years [range 25–80 years]) who were initially diagnosed with nasopharyngeal carcinoma and received radiotherapy in our hospital were enrolled. Only patients without thyroid, pituitary and hypothalamic diseases confirmed by pathology were included. IMRT was used to complete the intended radiotherapy in all patients. All patients underwent MRI with T1-weighted, T2-weighted, and contrast-enhanced T1-weighted imaging before, during, and 6 months and 12 months after radiotherapy.
Radiotherapy methods and radiation dose volume measurement
Doctors used a treatment planning system (TPS) to design radiotherapy plans, which were established according to the specific condition of patients. Patients received 6 MV X-ray or electron linear accelerator general radiotherapy five times a week, using 200 cGy each time, with a neck cure dose of DT 65–75 Gy and a prevention dose of DT 55–60 Gy. The slice distance and slice thickness of the positioning images were all 3 mm. After scanning, the images were sent to the treatment planning system, and the thyroid contour and other target areas were delineated layer by layer (Figure 1). The dose volume histogram (DVH) was used to calculate the dosimetric parameters of thyroid and pituitary irradiation, including the maximum dose, minimum dose, and average dose of the thyroid and pituitary, and the Vx of the thyroid (the percentage of the thyroid volume irradiated by x Gy dose, x = 30, 35, 40, 45, 50) (Figure 2).
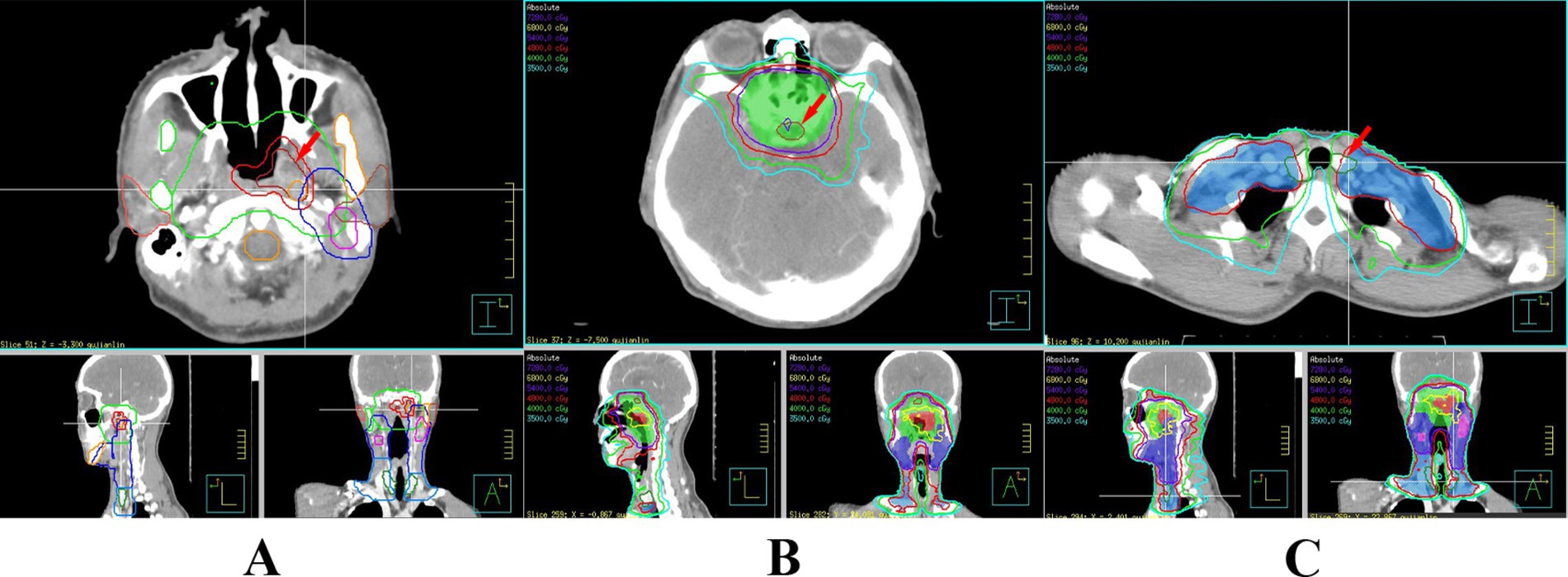
Figure 1. Delineation of the patient’s primary tumor (A), pituitary gland (B), and thyroid gland (C).
MRI technique
A Philips Achieva 3.0 T superconducting MR machine and a head and neck combined phased array coil were used. The scanning scope included the whole thyroid gland. T1WI and enhanced T1WI scans were performed with a spin echo sequence (SE) (TR 650–710 ms, TE 15 ms, FOV 180 mm, matrix 256 × 512, excitation times 1, slice thickness 6 mm, interval 1 mm). Contrast-enhanced scanning was performed with Gd-DTPA injection through a high-pressure autoinjector (XD 2000 CT/MRI, Ulrich GMBH, Ulrich, Germany, Germany) by bolus injection into the forearm vein at a rate of 2 mL/s at a dose of 0.1 mmol/kg. T2WI scans were performed with a turbo echo sequence (TSE) (TR 2800–4,900 ms, TE 80–120 ms, FOV 180 mm, matrix 256 × 512, excitation times 1 time, slice thickness 6 mm, interval 1 mm). The scanning parameters were consistent before and after radiotherapy.
Analysis of images
Philips Extended MR WorkSpace software was used to measure the following data. The transverse and anteroposterior diameters were measured on the axial T1WI images. Then, the outline of the thyroid gland was manually drawn as the region of interest (ROI) on each layer of the image, and the thyroid volume was calculated automatically by software. The percentage of thyroid volume (PTV) before and after radiotherapy was calculated [PTV = (postradiotherapy thyroid volume/preradiotherapy thyroid volume) × 100%]. More than 100% was considered an increase, and less than 100% was considered a decrease. The signal intensity (SI) of the bilateral lobe of the thyroid and surrounding fat on T1WI, T2WI, and enhanced T1WI axial images was measured by drawing the ROI. The SI of both lobes was averaged and used as the mean SI of the thyroid gland. The relative signal intensity (RSI) of the thyroid in each sequence scan was calculated by the formula:
T1WI RSI = T1WI SI thyroid/T1WI SI fat.
T2WI RSI = T2WI SI thyroid/T2WI SI fat.
Enhanced T1WI RSI = enhanced T1WI SI thyroid/T1WI SI fat.
Results
The demographic and clinical characteristics of the patients are listed in Table 1. Table 2 shows the dose to the thyroid gland and the dose to the pituitary gland.
Morphology
The transverse and anteroposterior diameters of each lobe and the volume of the thyroid gland gradually decreased from those before radiotherapy to those during radiotherapy and those 6 months and 12 months after radiotherapy (Table 3 and Figure 3). The PTV during radiotherapy, 6 months after radiotherapy and 12 months after radiotherapy were (87.34 ± 8.90)%, (66.47 ± 8.68)% and (50.87 ± 8.39)% of those before radiotherapy, respectively, and these values were negatively correlated with the mean dose and V50 of the thyroid (p < 0.05). There was a significant negative correlation between PTV and V40 and V45 of the thyroid during and 12 months after radiotherapy. There was a weak negative correlation between PTV and V35 of the thyroid at 12 months after radiotherapy. There was no significant correlation between PTV and V45, V40 or V35 at 6 months after radiotherapy. There was no significant correlation between PTV and V35 during radiotherapy. There was no significant correlation between PTV and the maximum radiation dose, the minimum radiation dose and the V30 of the thyroid and the irradiation dose of the pituitary at each time point after radiotherapy (p > 0.05) (Table 4).
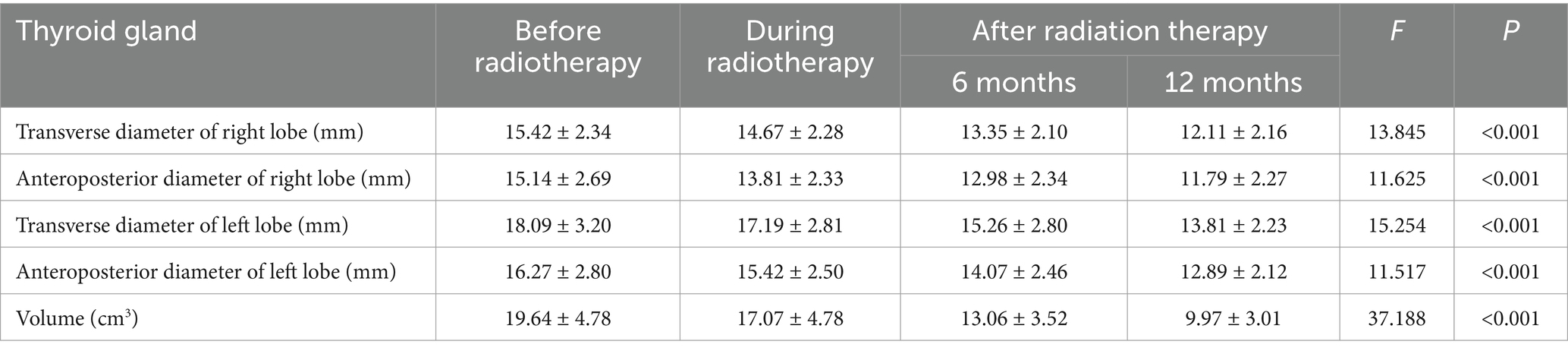
Table 3. Thyroid diameter and volume at different time points before and after radiotherapy (mean ± SD).
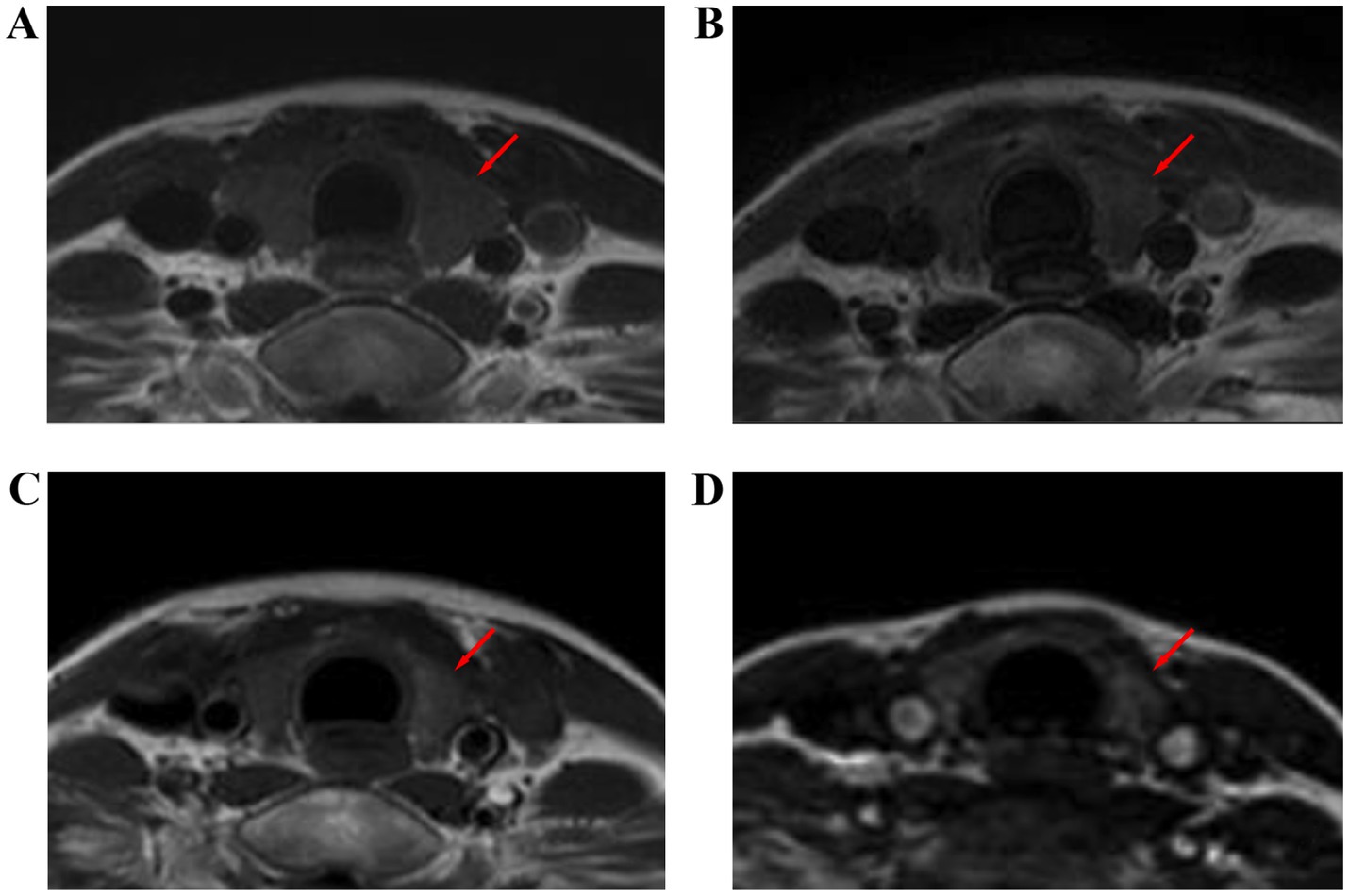
Figure 3. Thyroid size at different time points before and after radiotherapy for nasopharyngeal carcinoma: T1WI images before radiotherapy (A), during radiotherapy (B), 6 months after radiotherapy (C), and 12 months after radiotherapy (D).
Relative signal intensity
The mean T1WI RSI, T2WI RSI, and contrast-enhanced T1WI RSI of the thyroid gland gradually decreased from before radiotherapy to during radiotherapy and at 6 months and 12 months after radiotherapy (Table 5 and Figures 4–6). Tables 6–8 show the correlation between the MRI signal intensity of the thyroid after radiotherapy and the irradiated dose volume. The T1WI RSI, T2WI RSI and enhanced T1WI RSI of the thyroid during radiotherapy, 6 months and 12 months after radiotherapy were negatively correlated with the mean radiation dose, V50, V30, V35, V40, and V45 of the thyroid, respectively (p < 0.05), but were not significantly correlated with the maximum radiation dose, minimum radiation dose of the thyroid gland and the radiation dose of the pituitary gland (p > 0.05).

Table 5. MRI parameters of thyroid at different time points before and after radiotherapy (mean ± SD).
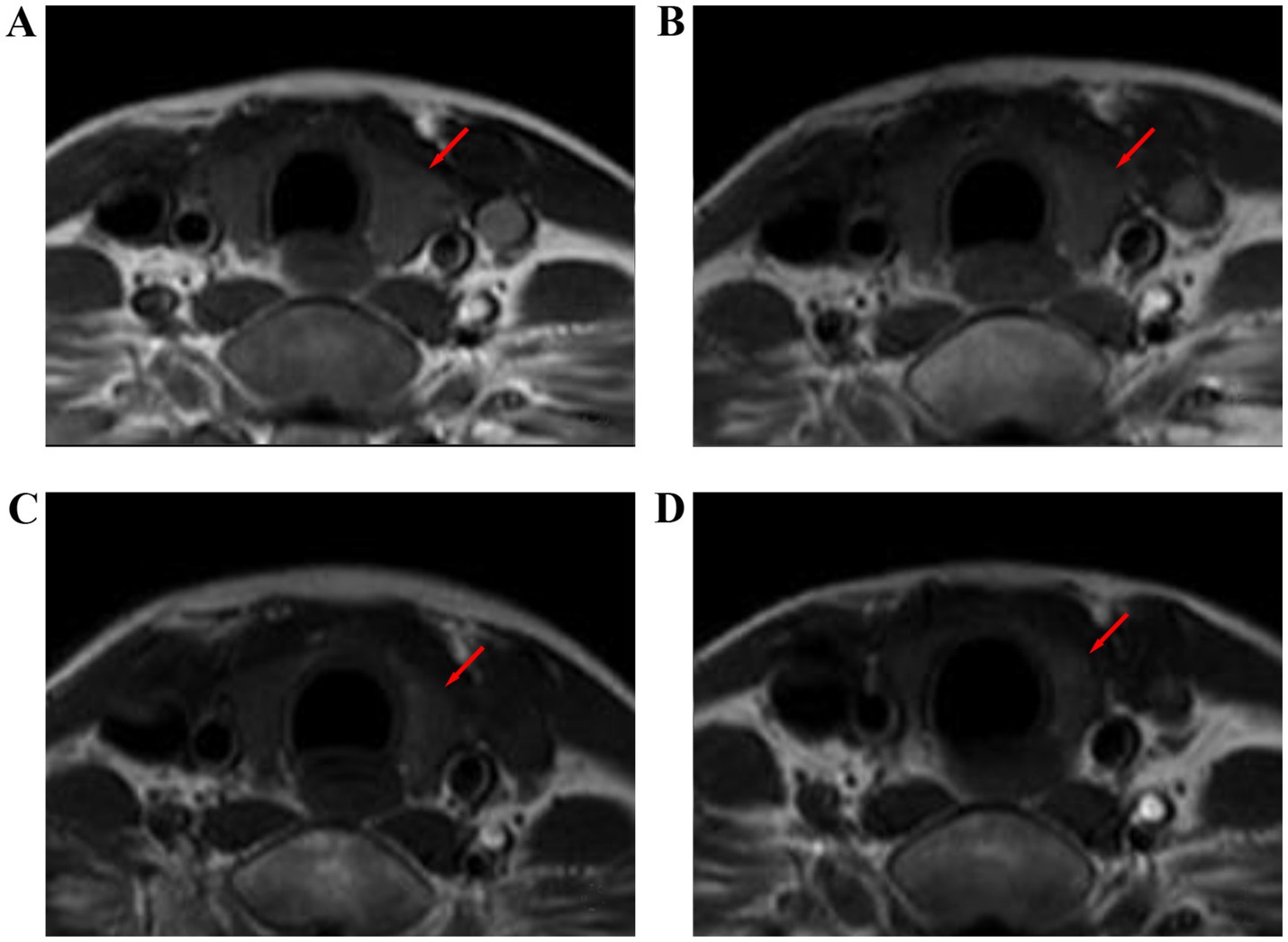
Figure 4. T1-weighted images of the thyroid gland of a 32-year-old female with nasopharyngeal carcinoma before and after radiotherapy. (A) Before radiotherapy, (B) during radiotherapy, (C) 6 months after radiotherapy, and (D) 12 months after radiotherapy.
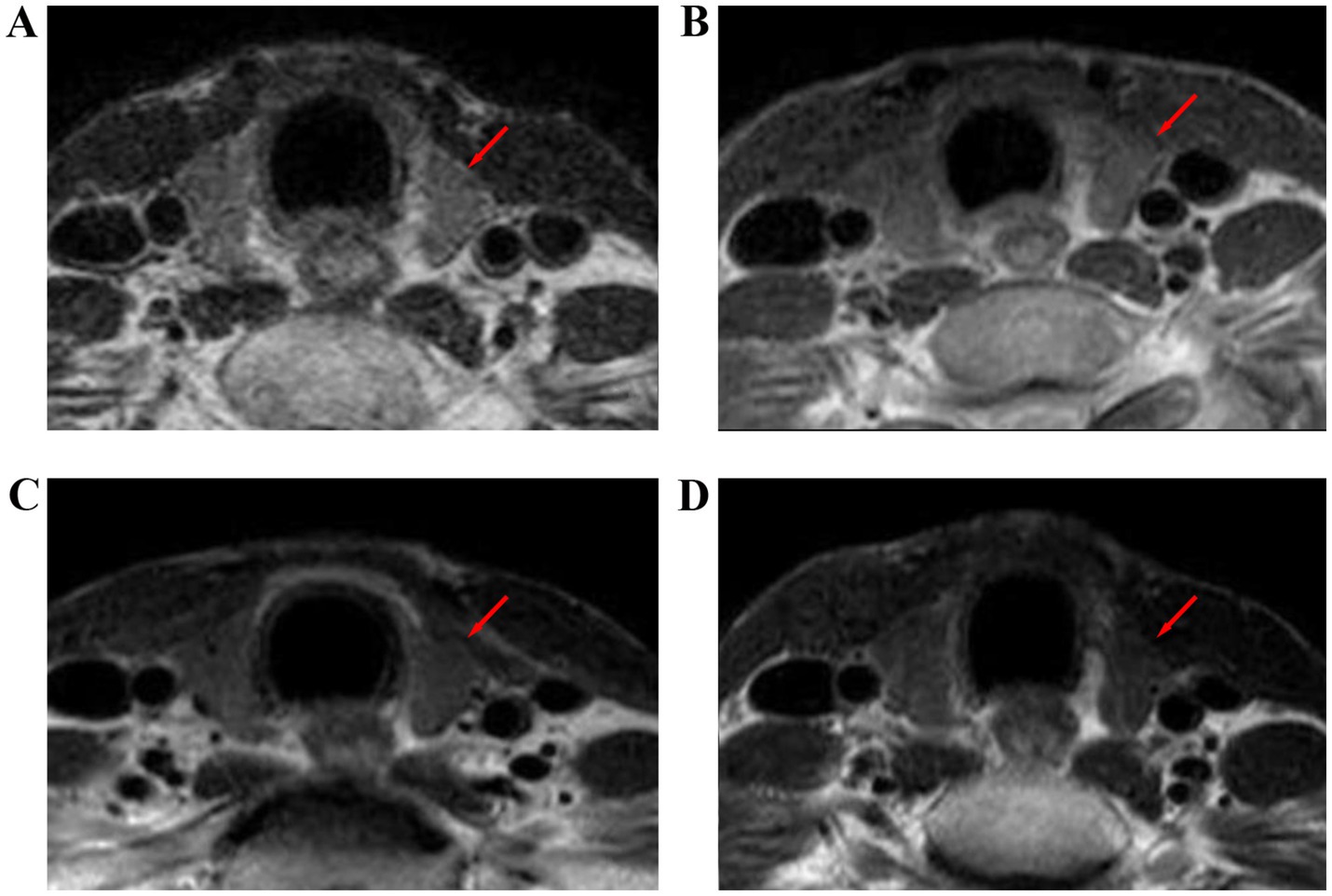
Figure 5. T2-weighted images of the thyroid gland before and after radiotherapy for nasopharyngeal carcinoma. (A) Before radiotherapy, (B) during radiotherapy, (C) 6 months after radiotherapy, and (D) 12 months after radiotherapy.
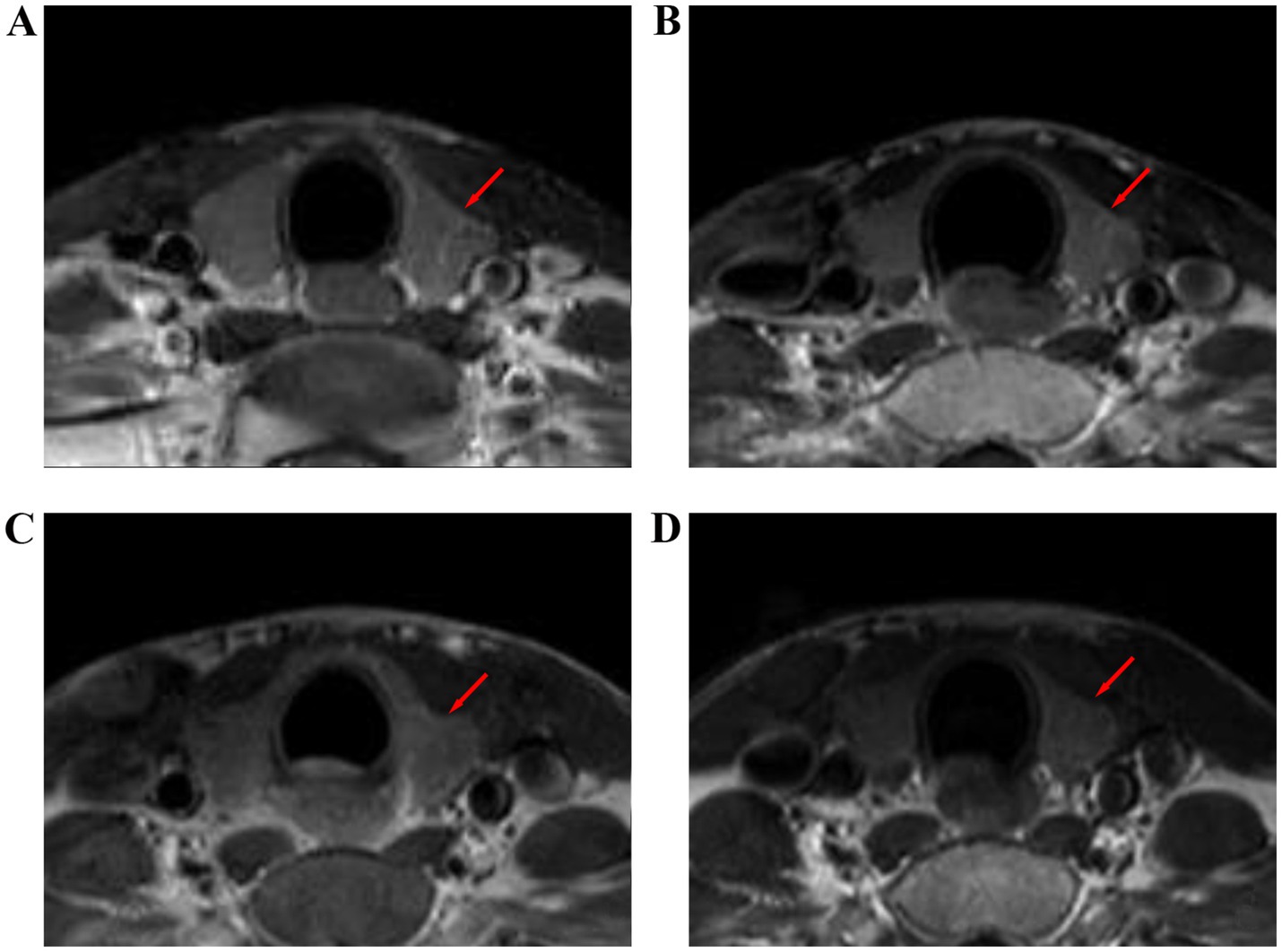
Figure 6. Contrast enhanced T1WI images of the thyroid gland before and after radiotherapy for nasopharyngeal carcinoma. (A) Before radiotherapy, (B) during radiotherapy, (C) 6 months after radiotherapy, and (D) 12 months after radiotherapy.
Discussion
Both X-ray radiotherapy and electron radiotherapy are the main methods of radiotherapy. Although the electron radiotherapy dose distribution is more concentrated, but it has a clear “rapidly decreasing dose” region, so electron radiotherapy is generally used for superficial tumors. X-ray radiotherapy is applied to deep tumors and it is the therapy method for nasopharyngeal carcinoma in clinical. Radiotherapy can cause different degrees of thyroid damage in patients with nasopharyngeal carcinoma, which may cause various histopathological changes in the thyroid. Thyroid complications, such as hypothyroidism, hyperthyroidism, benign adenoma, Graves’ disease and even thyroid cancer, have been reported in the literature; among these complications, hypothyroidism is the most common one (7, 10, 11).
In this study, the thyroid volume gradually decreased with time after radiotherapy. Previous studies have found that the cause of radiation-induced thyroid injury is mainly related to vascular injury, parenchymal cell injury and autoimmune response (12–14). After radiation, thyroid cells undergo DNA strand damage in acute changes, which leads to mitotic death of thyroid cells (15). Damaged cells can still maintain their basic morphology and function, and due to the slow renewal of thyroid tissue cells, damaged cells can continue to exist and function for a long time. Therefore, radiation-induced thyroid injury often manifests gradually after a long time. The chronic changes occurred mainly months to years after radiotherapy and were characterized by massive death of thyroid cells and inhibition of thyroid cell replication by proliferating fibers (16). In addition, the products of radiation damage may increase thyroid autoantibodies (17, 18) and promote the occurrence of thyroid autoimmunity.
In terms of the rate of decrease, the decrease in thyroid volume was most obvious at 6 months after radiotherapy. The reason for this is that there is acute inflammatory edema of the thyroid gland and the compensatory effect of the thyroid gland during radiotherapy. The fibrous tissue and other tissues increase in the late stage (more than 1 year) after radiotherapy (19). However, the early stage (less than 6 months) after radiotherapy is mainly dominated by cell death (20), so the volume decrease is the fastest.
The thyroid-enhanced T1WI RSI gradually decreased with time, which was considered to be mainly related to delayed vascular damage after radiotherapy. On the one hand, the structure and function of vascular smooth muscle cells and vascular endothelial cells are changed, cell apoptosis is increased, and cell proliferation is inhibited after radiotherapy. On the other hand, various inflammatory mediators and cytokines produced in the irradiated area can mediate the local inflammatory response, and the two interact together to lead to vascular injury (21, 22). Vascular injury can occur in all levels of arteries and veins, but it most commonly occurs in microvessels, especially capillaries and venous sinuses (23, 24). Such injuries lead to vascular fibrosis, vascular diameter reduction, occlusion, and blood supply reduction and then cause thyroid injury. Enhanced T1WI RSI increased in some patients after radiotherapy. The reason for this was considered to be the decrease in thyroid hormone in blood, the increase in thyroid stimulating hormone (TSH) secretion by feedback stimulation, and the increase in thyroid blood flow.
The results of this study showed that there was a significant negative correlation between the mean radiation dose of the thyroid and the volume of bilateral thyroid lobes, including the transverse diameters, anteroposterior diameters and the degree of volume reduction. Colevas et al. (25) suggested that thyroid dysfunction could occur after exposure to more than 30–45 Gy of radiation. Zhai et al. (26) showed a significant correlation between the radiation dose to the thyroid and the incidence of hypothyroidism. The mean radiation dose to the thyroid gland in our study was higher than the above dose, and this study confirmed the above findings from a certain angle. In this study, the transverse diameter, anteroposterior diameter and volume reduction of the thyroid gland were negatively correlated with the V40, V45, and V50 of the thyroid gland. The correlation with V50 was the most significant, which was similar to the results of previous study (27). Alterio et al. (28) also found that V50 was one of the most important predictors of hypothyroidism after radiotherapy by LASSO analysis. One adult-based study (29) stated that volume reductions usually occurred prior to hypothyroidism, which could imply that thyroid hormone substitution therapy should be started early.
The T1WI RSI of the thyroid gland at 12 months after radiotherapy had the highest correlation with the mean radiation dose, V40, V45, and V50 of the thyroid gland, considering the gradual aggravation of tissue fibrosis in radiation-induced thyroid injury with time after radiotherapy. The correlation between T2WI RSI and mean radiation dose, V40, V45, and V50 of the thyroid gland was the highest during radiotherapy, considering the existence of tissue edema at this time. The correlation between enhanced T1WI RSI and mean radiation dose, V40, V45, and V50 of the thyroid gland was higher during radiotherapy and at 12 months after radiotherapy than at 6 months after radiotherapy because the inflammatory edema of the former was the most severe, and the vascular injury of the latter was the most severe. An ultrasound study found a significant increase in all color Doppler ultrasonography (CDU) parameters of the inferior thyroid artery after early radiotherapy. This may indicate that radiation-induced acute thyroiditis, which can lead to subsequent hypothyroidism or hyperthyroidism, is the main damage to the thyroid (30).
Radiation damage to the pituitary and thalamus after radiotherapy can cause central hypothyroidism. It has been reported that radiotherapy can directly inhibit the normal function of the anterior pituitary, leading to a decrease in the secretion of TSH. According to Graffeo et al. (31), when the radiation dose of the pituitary gland exceeds 45 Gy, pituitary dysfunction will begin to appear. Pomeraniec et al. (32) showed that central hypothyroidism has a long latency and may be a late side effect. Darzy and Shalet (33) showed that the pituitary dose threshold can reach 60 Gy, and hypothyroidism generally occurs 2–5 years after radiotherapy. In this study, the thalamus was not exposed to the radiation field. The mean radiation dose to the pituitary gland was 52 Gy, which was within the range of the pituitary control limit of 50–55 Gy. The follow-up time was relatively short, and no obvious pituitary injury was generally observed. There was no significant correlation between these parameters and the radiation dose to the pituitary gland. It is suggested that the changes in thyroid volume and signal intensity after radiotherapy are not related to central hypothyroidism.
Conclusion
Current studies have shown that head and neck radiotherapy can cause hypothyroidism in patients with nasopharyngeal carcinoma, which is characterized by reduced thyroid volume and decreased signal intensity. Magnetic resonance imaging can provide useful additional information in patients with nasopharyngeal carcinoma undergoing radiotherapy.
Data availability statement
The raw data supporting the conclusions of this article will be made available by the authors, without undue reservation.
Ethics statement
The studies involving humans were approved by The Ethics Committee of the Second Affiliated Hospital of Soochow University. The studies were conducted in accordance with the local legislation and institutional requirements. The participants provided their written informed consent to participate in this study. Written informed consent was obtained from the individual(s) for the publication of any potentially identifiable images or data included in this article.
Author contributions
KL: Conceptualization, Investigation, Methodology, Writing – original draft. CZ: Investigation, Writing – review & editing. JR: Investigation, Writing – review & editing. JN: Data curation, Writing – review & editing. WY: Data curation, Writing – review & editing. YW: Data curation, Writing – review & editing. DJ: Data curation, Writing – review & editing. JQ: Project administration, Supervision, Writing – review & editing. YZ: Project administration, Supervision, Writing – review & editing. DS: Project administration, Supervision, Writing – review & editing. WH: Funding acquisition, Project administration, Supervision, Writing – review & editing. LX: Conceptualization, Funding acquisition, Methodology, Project administration, Writing – review & editing.
Funding
The author(s) declare that financial support was received for the research, authorship, and/or publication of this article. This research was funded by the Project of State Key Laboratory of Radiation Medicine and Protection, Soochow University (GZK12024050, GZK12024051, and GZK12023041).
Conflict of interest
The authors declare that the research was conducted in the absence of any commercial or financial relationships that could be construed as a potential conflict of interest.
Generative AI statement
The authors declare that no Generative AI was used in the creation of this manuscript.
Publisher’s note
All claims expressed in this article are solely those of the authors and do not necessarily represent those of their affiliated organizations, or those of the publisher, the editors and the reviewers. Any product that may be evaluated in this article, or claim that may be made by its manufacturer, is not guaranteed or endorsed by the publisher.
References
1. Lee, HM, Okuda, KS, Gonzalez, FE, and Patel, V. Current perspectives on nasopharyngeal carcinoma. Adv Exp Med Biol. (2019) 1164:11–34. doi: 10.1007/978-3-030-22254-3_2
2. Su, SF, Han, F, Zhao, C, Chen, CY, Xiao, WW, Li, JX, et al. Long-term outcomes of early-stage nasopharyngeal carcinoma patients treated with intensity-modulated radiotherapy alone. Int J Radiat Oncol Biol Phys. (2012) 82:327–33. doi: 10.1016/j.ijrobp.2010.09.011
3. Tang, LL, Chen, YP, Chen, CB, Chen, MY, Chen, NY, Chen, XZ, et al. The Chinese Society of Clinical Oncology (CSCO) clinical guidelines for the diagnosis and treatment of nasopharyngeal carcinoma. Cancer Commun. (2021) 41:1195–227. doi: 10.1002/cac2.12218
4. Sommat, K, Ong, WS, Hussain, A, Soong, YL, Tan, T, Wee, J, et al. Thyroid V40 predicts primary hypothyroidism after intensity modulated radiation therapy for nasopharyngeal carcinoma. Int J Radiat Oncol Biol Phys. (2017) 98:574–80. doi: 10.1016/j.ijrobp.2017.03.007
5. McDowell, LJ, Rock, K, Xu, W, Chan, B, Waldron, J, Lu, L, et al. Long-term late toxicity, quality of life, and emotional distress in patients with nasopharyngeal carcinoma treated with intensity modulated radiation therapy. Int J Radiat Oncol Biol Phys. (2018) 102:340–52. doi: 10.1016/j.ijrobp.2018.05.060
6. Jia-Mahasap, B, Assavanopakun, K, Chitapanarux, I, Kittidachanan, K, and Sirikul, W. Incidence of radiation-induced hypothyroidism following head and neck irradiation: a single-center analysis. Rep Pract Oncol Radiother. (2022) 27:479–89. doi: 10.5603/RPOR.a2022.0055
7. Vogelius, IR, Bentzen, SM, Maraldo, MV, Petersen, PM, and Specht, L. Risk factors for radiation-induced hypothyroidism: a literature-based meta-analysis. Cancer. (2011) 117:5250–60. doi: 10.1002/cncr.26186
8. Jereczek-Fossa, BA, Alterio, D, Jassem, J, Gibelli, B, Tradati, N, and Orecchia, R. Radiotherapy-induced thyroid disorders. Cancer Treat Rev. (2004) 30:369–84. doi: 10.1016/j.ctrv.2003.12.003
9. Chen, YP, Chan, ATC, Le, QT, Blanchard, P, Sun, Y, and Ma, J. Nasopharyngeal carcinoma. Lancet. (2019) 394:64–80. doi: 10.1016/S0140-6736(19)30956-0
10. Lian, CL, Zhuo, RG, Zhou, R, Yu, YF, Zhou, P, Lin, Q, et al. Risk factors of early thyroid dysfunction after definitive radiotherapy in nasopharyngeal carcinoma patients. Head Neck. (2023) 45:2344–54. doi: 10.1002/hed.27448
11. Boomsma, MJ, Bijl, HP, and Langendijk, JA. Radiation-induced hypothyroidism in head and neck cancer patients: a systematic review. Radiother Oncol. (2011) 99:1–5. doi: 10.1016/j.radonc.2011.03.002
12. Rooney, MK, Andring, LM, Corrigan, KL, Bernard, V, Williamson, TD, Fuller, CD, et al. Hypothyroidism following radiotherapy for head and neck Cancer: a systematic review of the literature and opportunities to improve the therapeutic ratio. Cancers. (2023) 15:4321. doi: 10.3390/cancers15174321
13. Miller, MC. Agrawal, a., hypothyroidism in postradiation head and neck cancer patients: incidence, complications, and management. Curr Opin Otolaryngol Head Neck Surg. (2009) 17:111–5. doi: 10.1097/moo.0b013e328325a538
14. Lin, Z, Yang, Z, He, B, Wang, D, Gao, X, Tam, SY, et al. Pattern of radiation-induced thyroid gland changes in nasopharyngeal carcinoma patients in 48 months after radiotherapy. PLoS One. (2018) 13:e0200310. doi: 10.1371/journal.pone.0200310
15. Durante, M, and Formenti, SC. Radiation-induced chromosomal aberrations and immunotherapy: micronuclei, cytosolic DNA, and interferon-production pathway. Front Oncol. (2018) 8:192. doi: 10.3389/fonc.2018.00192
16. Kamal, M, Peeler, CR, Yepes, P, Mohamed, ASR, Blanchard, P, Frank, S, et al. Radiation-induced hypothyroidism after radical intensity modulated radiation therapy for oropharyngeal carcinoma. Adv Radiat Oncol. (2020) 5:111–9. doi: 10.1016/j.adro.2019.08.006
17. Ling, S, Bhatt, AD, Brown, NV, Nguyen, P, Sipos, JA, Chakravarti, A, et al. Correlative study of dose to thyroid and incidence of subsequent dysfunction after head and neck radiation. Head Neck. (2017) 39:548–54. doi: 10.1002/hed.24643
18. Shen, G, Peng, Y, Li, J, Wu, H, Zhang, G, Zhao, C, et al. Deng, X., multivariate NTCP model of hypothyroidism after intensity-modulated radiotherapy for nasopharyngeal carcinoma. Front Oncol. (2021) 11:714536. doi: 10.3389/fonc.2021.714536
19. Roberson, J, Huang, H, Noldner, C, Hou, W, Mani, K, Valentine, E, et al. Thyroid volume changes following adjuvant radiation therapy for breast cancer. Clin Transl Radiat Oncol. (2023) 39:100566. doi: 10.1016/j.ctro.2022.100566c
20. Ran, JT, Song, BF, Liu, ZQ, Gao, LY, and Wang, XH. Comparison of dose distribution in thyroid gland during intensity modulated radiotherapy for nasopharyngeal carcinoma. Chin J Cancer Prevent Treat. (2015) 22:951–3. doi: 10.16073/j.cnki.cjcpt.2015.12.010
21. Possenti, L, Mecchi, L, Rossoni, A, Sangalli, V, Bersini, S, Cicchetti, A, et al. Radiobiological studies of microvascular damage through in vitro models: a methodological perspective. Cancers. (2021) 13:13051182. doi: 10.3390/cancers
22. Zhang, SY, Park, KW, Oh, S, Cho, HJ, Cho, HJ, Park, JS, et al. NF-kappa B decoy potentiates the effects of radiation on vascular smooth muscle cells by enhancing apoptosis. Exp Mol Med. (2005) 37:18–26. doi: 10.1038/emm.2005.3
23. Fajardo, LF. The pathology of ionizing radiation as defined by morphologic patterns. Acta Oncol. (2005) 44:13–22. doi: 10.1080/02841860510007440
24. Jahng, JWS, Little, MP, No, HJ, Loo, BW Jr, and Wu, JC. Consequences of ionizing radiation exposure to the cardiovascular system. Nat Rev Cardiol. (2024) 21:880–98. doi: 10.1038/s41569-024-01056-4
25. Colevas, AD, Read, R, Thornhill, J, Adak, S, Tishler, R, Busse, P, et al. Hypothyroidism incidence after multimodality treatment for stage III and IV squamous cell carcinomas of the head and neck. Int J Radiat Oncol Biol Phys. (2001) 51:599–604. doi: 10.1016/s0360-3016(01)01688-1
26. Zhai, R, Lyu, Y, Ni, M, Kong, F, Du, C, Hu, C, et al. Predictors of radiation-induced hypothyroidism in nasopharyngeal carcinoma survivors after intensity-modulated radiotherapy. Radiat Oncol. (2022) 17:57. doi: 10.1186/s13014-022-02028-z
27. Sachdev, S, Refaat, T, Bacchus, ID, Sathiaseelan, V, and Mittal, BB. Thyroid V50 highly predictive of hypothyroidism in head-and-neck Cancer patients treated with intensity-modulated radiotherapy (IMRT). Am J Clin Oncol. (2017) 40:413–7. doi: 10.1097/COC.0000000000000165
28. Alterio, D, Jereczek-Fossa, BA, Franchi, B, D’Onofrio, A, Piazzi, V, Rondi, E, et al. Thyroid disorders in patients treated with radiotherapy for head-and-neck cancer: a retrospective analysis of seventy-three patients. Int J Radiat Oncol Biol Phys. (2007) 67:144–50. doi: 10.1016/j.ijrobp.2006.08.051
29. Lin, Z, Wu, VW, Lin, J, Feng, H, and Chen, L. A longitudinal study on the radiation-induced thyroid gland changes after external beam radiotherapy of nasopharyngeal carcinoma. Thyroid. (2011) 21:19–23. doi: 10.1089/thy.2010.0229
30. Bakhshandeh, M, Hashemi, B, Mahdavi, SR, Nikoofar, A, Edraki, HR, and Kazemnejad, A. Evaluation of thyroid disorders during head-and-neck radiotherapy by using functional analysis and ultrasonography. Int J Radiat Oncol Biol Phys. (2012) 83:198–203. doi: 10.1016/j.ijrobp.2011.05.064
31. Graffeo, CS, Perry, A, Link, MJ, Brown, PD, Young, WF, and Pollock, BE. Biological effective dose as a predictor of hypopituitarism after single-fraction pituitary adenoma radiosurgery: dosimetric analysis and cohort study of patients treated using contemporary techniques. Neurosurgery. (2021) 88:E330–5. doi: 10.1093/neuros/nyaa555
32. Pomeraniec, IJ, Xu, Z, Lee, CC, Yang, HC, Chytka, T, Liscak, R, et al. Dose to neuroanatomical structures surrounding pituitary adenomas and the effect of stereotactic radiosurgery on neuroendocrine function: an international multicenter study. J Neurosurg. (2022) 136:813–21. doi: 10.3171/2021.3.JNS203812
Keywords: thyroid, magnetic resonance imaging (MRI), nasopharyngeal carcinoma, radiation therapy, dose
Citation: Lu K, Zhou C, Ren J, Ni J, Yang W, Wang Y, Jin D, Qian J, Zhu Y, Shi D, Hu W and Xu L (2025) Preliminary study on the correlation between thyroid magnetic resonance parameters and radiation dose after radiotherapy for nasopharyngeal carcinoma. Front. Public Health. 12:1526147. doi: 10.3389/fpubh.2024.1526147
Edited by:
Fei Tuo, Chinese Center for Disease Control and Prevention, ChinaReviewed by:
Burong Hu, Wenzhou Medical University, ChinaZhen Wang, Gunma University Heavy Ion Medical Center, Japan
Copyright © 2025 Lu, Zhou, Ren, Ni, Yang, Wang, Jin, Qian, Zhu, Shi, Hu and Xu. This is an open-access article distributed under the terms of the Creative Commons Attribution License (CC BY). The use, distribution or reproduction in other forums is permitted, provided the original author(s) and the copyright owner(s) are credited and that the original publication in this journal is cited, in accordance with accepted academic practice. No use, distribution or reproduction is permitted which does not comply with these terms.
*Correspondence: Dai Shi, c2hpZGFpMzE4QDE2My5jb20=; Wentao Hu, d3RodUBzdWRhLmVkdS5jbg==; Liang Xu, eGw3NDE1QDE2My5jb20=
†These authors share first authorship