- 1Laboratório das Interações Vírus-Hospedeiros - LIVH, Instituto Oswaldo Cruz/Fiocruz, Rio de Janeiro, Brazil
- 2Programa de Computação Científica, Fundação Oswaldo Cruz - PROCC, Fundação Oswaldo Cruz (Fiocruz), Rio de Janeiro, RJ, Brazil
- 3Instituto de Tecnologia em Fármacos, Fundação Oswaldo Cruz, Rio de Janeiro, Brazil
- 4Florida Medical Entomology Laboratory-FMEL, University of Florida, Vero Beach, FL, United States
- 5Núcleo Operacional Sentinela de Mosquitos Vetores-Nosmove/Fiocruz, Rio de Janeiro, Brazil
Chikungunya virus (CHIKV) is mainly transmitted by the invasive mosquito Aedes (Stegomyia) aegypti in tropical and subtropical regions worldwide. However, genetic adaptations of the virus to the peri domestic mosquito vector Aedes (Stegomyia) albopictus has resulted in enhanced vector competence and associated epidemics and may contribute to further geographic expansion of CHIKV. However, evidence-based data on the relative role of Ae. albopictus in CHIKV transmission dynamics are scarce, especially in regions where Ae. aegypti is the main vector, such as in Brazil. Here, we review the CHIKV genotypes circulating in Brazil, spatial and temporal distribution of Chikungunya cases in Brazil, and susceptibility to infection and transmission (i.e., vector competence) of Ae. albopictus for CHIKV to better understand its relative contribution to the virus transmission dynamics.
Introduction
The dramatic emergence and spread of arboviral diseases in the past 50 years has highlighted the urgent need to review surveillance and control strategies. Only by application of integrated management approaches, emergent epidemic arboviral diseases, such as those recently observed after the emergence of Zika virus (ZIKV) in the Americas, Yellow Fever (YFV) in Angola and Brazil, West Nile virus (WNV) in the Americas and chikungunya virus (CHIKV) worldwide will be able to be prevented and controlled (1, 2).
In the sylvatic cycle, CHIKV usually circulates between non-human primates, other mammalian reservoir hosts and Aedes mosquitoes, but in the urban cycle, the virus is transmitted to humans through infectious bites by invasive mosquitoes, mainly Aedes (Stegomyia) aegypti (Linnaeus 1762) (3, 4).
Chikungunya fever is mainly characterized by fever, rash, and incapacitating arthralgia, with symptoms apparent in approximately 80% of the patients (5–7). High attack rates have been observed during outbreaks and more than 30% of the infected individuals develop chronic disease (e.g., debilitating arthralgia and arthritis) that can persist for years (8, 9). Chronic arthralgia often causes significant disability, hindering daily activities and leading to physical and mental distress. Patients frequently report symptoms like appetite loss, poor sleep, mood swings, and depression (10, 11). These symptoms can result in missed work or school, potentially leading to job loss or academic withdrawal, and a reduced quality of life (11–13).
Animal model studies suggest that chronic CHIKV disease may be the result of induced autoimmunity or viral persistence in joint-associated tissues (14). In rare instances, CHIKV infections have also been associated with neurological manifestations (15, 16).
A brief history of chikungunya
Chikungunya virus (CHIKV), an arbovirus transmitted by mosquito vectors, was first isolated from a febrile patient during an outbreak on the Makonde plateau in southern Tanzania in 1952 and, in 1953, the virus was first isolated from Ae. aegypti mosquitoes. Based on the disabling and debilitating symptoms presented, the disease was named chikungunya, derived from the Kimakonde language, and meaning “that which bends up” (17–19).
In 1958 and following years, cases of chikungunya were reported in Uganda and in other sub-Saharan African countries. The existence of a wild cycle was indicated by the isolation of CHIKV from a pool of the forest mosquito Ae. africanus collected in the Zika Forest and subsequent infection studies using mice and rhesus monkeys (20). The presence of anti-CHIKV antibodies in experimentally infected vervet monkeys (Chlorocebus pygerythrus) was evidence for the possible role of non-human primates and consideration of the involvement of non-human primates in a sylvatic transmission cycle (21).
Since its discovery in Tanzania in the 1950s, CHIKV has been responsible for emerging and reemerging epidemics in several temperate and tropical regions of the world, particularly in geographical areas inhabited by Ae. aegypti and Ae. albopictus mosquitoes (Skuse 1894) (22–24). The first CHIKV record outside Africa occurred in Thailand, associated with Ae. aegypti, in 1958, and India and Cambodia (25). Between the 1970s and 1990s, enzootic outbreaks occurred in and around Senegal (26, 27). Subsequently, CHIKV has emerged and spread across five continents at an unprecedented rate causing millions of cases, mainly in the tropical and subtropical regions worldwide (28, 29). In 2004 an intense epidemic reached the islands of the Indian Ocean. On La Reunión Island more than 300,000 cases were reported in 2006 (30, 31). During the outbreak on islands in the Indian Ocean and Asia, there were reported imported cases of chikungunya in Europe and the Americas (32, 33).
Due to the global epidemiological situation, the Pan American Health Organization (PAHO) began containment planning for a possible introduction of CHIKV in the Americas since 2010 (34, 35) and in that year, CHIKV imported cases were reported in Brazil. However, local transmission of CHIKV in the Americas was first reported in the Caribbean in 2013, on the island of Saint Martin (36). In the following year, nine islands in the Caribbean already recorded more than 15,000 suspected cases and by August 2014, there were more than 1,000 suspected cases of the chikungunya fever in Colombia (37). In April 2015, more than 1 million suspected cases and 191 deaths had already been reported in the Americas (2) and autochthonous transmission had been confirmed in more than 50 territories in the region (37, 38).
Chikungunya virus genotypes and its relation to emergence and spread
CHIKV belongs to the Togaviridae family, genus Alphavirus and is a small spherical enveloped virus (60–70 nm diameter) with a genome comprised of a positive single-strand RNA of approximately 11.8 Kb. The genome consists of two open-reading frames (ORFs) that encodes four conserved nonstructural proteins (nsP 1–4), a capsid protein (C), two envelope glycoproteins (E1 and E2) and two cleavage products (E3 and 6 K) (39, 40). NsP1, nsP2, and nsP4 are involved in RNA capping, helicase/protease activity and polymerase activity, respectively (41). NsP3 plays a role in viral replication (42). E1 and E2 proteins are present at high levels in humans during the acute phase of the disease (43, 44). Both 5′ and 3′ untranslated regions are present in the genome with the latter exhibiting stem-loop structures and repeats probably associated with virus adaptation to mosquitoes (45).
Chikungunya virus has a single serotype with four distinct genotypes: West African, East-Central-South-African (ECSA), Asian, and Indian Ocean Lineage (IOL). The geographic expansion of the different CHIKV genotypes was facilitated by the intense circulation of viremic travelers between countries, including temperate regions in Europe and the United States (46). Evolutionary studies provided evidence that the West African genotype originated in Africa, and subsequently spread into Asia, where it evolved into a distinct variant - the Asian genotype. The CHIKV strains from the Reunion Island epidemic in 2005, evolved and were characterized as the distinct ECSA genotype. The ECSA strains reemerged from the mainland in East Africa during an outbreak in Kenya and spread to Indian Ocean islands and India (47). This led the virus to reach La Reunion island, when its geographic range expanded rapidly to include several countries in Europe (32, 48), Americas (49) and Asia (50). The new Indian Ocean Lineage (IOL) evolved independently, as an ECSA monophyletic group (26, 27, 46, 51, 52). Both Asian and ECSA genotypes are those most frequently detected following the virus spread worldwide (53).
The CHIKV ECSA genotype identified during the Indian Ocean epidemic was a mutant, with a substitution from alanine to valine at position 226 of the E1 envelope glycoprotein (E1-A226V) and was described as the IOL (54, 55). The E1-A226V mutation enhanced infectivity (i.e., lower oral infection dose of 50% of mosquitoes tested, OID50), viral dissemination efficiency to secondary organs, and transmission by Ae. albopictus to mice, favoring it as the main vector in the region (31, 55, 56). The high density of Ae. albopictus in Réunion Island and lower viremia necessary to infect the local Ae. albopictus population (i.e., lower viremic thresholds occur sooner and are sustained for longer periods in human hosts) contributed to viral spread in this region. During this time, 255,000 cases of CHIKV were reported from March 2005 to April 2006, with the IOL strain identified in 90% of the isolates from human cases (54, 57, 58). The extensive geographic distribution of Ae. albopictus, coupled with mutations that improve fitness and infectivity of CHIKV in Ae. albopictus, may allow for expansion of CHIKV into temperate ecosystems (59), as observed with small outbreaks in France and Italy (32).
Tsetsarkin et al. (55) demonstrated that the E1-A226V mutation results in a ECSA genotype being 100-fold more infectious for Ae. albopictus. However, this mutation is associated with a reduction in infectivity of CHIKV in Ae. aegypti midguts, which is considered the main vector of CHIKV, but not in Réunion Island. This CHIKV strain has spread across Asia, although there are also circulating CHIKVs in the region that do not carry the mutation (60).
A new classification for the ECSA genotype was proposed by Schneider et al. (61), given its wide expansion and identification of distinct strains/genotypes. According to the authors, ECSA genotype could be divided into 3 different genotypes, one for each region of the African continent indicated in its name (61).
Spatial–temporal distribution of chikungunya in Brazil
In Brazil, CHIKV autochthonous transmission occurred after the simultaneous introduction of the Asian and ECSA genotypes in 2014 in the municipality of Oiapoque, Amapá, North region and Feira de Santana, Bahia, Northeast region, respectively (37, 52). In the following years, the ECSA genotype spread to other Brazilian states, and CHIKV outbreaks were registered in the Northeast [Bahia (62–64); Alagoas (65, 66), Piauí (67) Sergipe (68, 69), Maranhão (70)], North [Roraima (71)], Southeast [Rio de Janeiro (72–75) and Minas Gerais, (76)], and Midwest regions (77). Despite reports on the south region, most cases in 2014 and 2015 were imported from the other Brazilian regions (78). Since 2016, Brazil has been the epicenter of CHIKV epidemics in the Americas with reports of annual outbreaks each year and more than 1.6 million cases to date (79).
A previous study reported that the ECSA genotype was likely introduced to Rio de Janeiro early in 2014 through a single event, after primary circulation in Bahia in the previous year (80). On the other hand, Fabri et al. (75) suggests that two independent introductions of the ECSA genotype occurred in Rio de Janeiro between 2016 and 2019, both from the Northeast region (75), showing the complexity of tracing an entry path of CHIKV in Brazil. In fact, a recent study analyzing the epidemiological patterns of the designated ECSA American sub-lineage in Rio de Janeiro revealed two distinct clades introduced from the Northeast region mid-2015 and mid-2017 (81). Despite the detection of both Asian and ECSA genotypes in Brazil, the latter was more frequently associated with symptomatic cases in the country (71, 82, 83). Moreover, the newly emergent ECSA American sub-lineage has become prevalent throughout Brazil (79). Previous studies on lineage replacement among dengue viruses (DENV) supports the notion that differences in underlying viral fitness, as measured by viraemia levels in humans and relative infectivity in mosquitoes, is the main driver of evolutionary events where lineage turnover has occurred, most notably with Southeast Asian genotypes of dengue virus displacing American genotypes (84–89).
Epidemics caused by CHIKV present a cyclical pattern, which can be characterized by periods of epidemiological silence, rotated with periods of intense viral circulation. Both Asian and ECSA genotypes could spread and co-circulate in Brazil, considering suitability of Ae. aegypti and Ae. albopictus vectors. The rapid spread and establishment of CHIKV was facilitated by the high density of its main vector Ae. aegypti, favorable climate, unplanned urbanization, wide availability of reservoirs, human behavior, and a large population of susceptible human hosts (22, 54, 57, 58, 90–96).
Additionally, the simultaneous circulation of CHIKV, DENV, Zika virus (ZIKV), and other arboviruses of medical importance [e.g., Mayaro (MAYV) and Oropouche viruses (OROV)], represents a serious public health challenge for Brazil, because of overlapping clinical signs and symptoms, unavailability of specific and reliable tests for differential diagnosis for health professionals, as well as highlighting the need for active and efficient arbovirus surveillance (37, 97–99).
Temporal analysis of CHIKV
Figure 1 shows the time series of probable chikungunya cases in Brazil (top grid) and in each of the country’s five regions (Midwest, Northeast, North, Southeast and South). The y-axes are set at different ranges (i.e., not standardized) which facilitates observing peaks of cases in each region. Despite several peaks of probable cases in the country occurring in the first half of 2016, 2017, 2019, and 2022, it is possible to see that each region sustained different temporal epidemiological patterns. The Northeast region was the most affected of all five regions both in total number of cases (502,761) but also by being the first to display an increase in cases in 2016 followed by a peak of cases in 2017 (with 11,087 cases in epiweek 18–2017). The increase of cases in 2016 in the Northeast region was followed by a peak of cases in the same region during 2017. Similarly, a peak of cases was observed in the North region (801 cases in epiweek 5–2017, out of a total of 32,485 cases in the whole period) and the first of three consecutive growing peaks of cases in the Southeast region (the most densely populated region in Brazil). The Midwest region exhibited the peak of cases in 2018 (1,263 in epiweek 4–2018, out of 24,038 during the whole period), and a new peak of cases in the Southeast region. The year 2019 was the last year exhibiting an increase of cases, with a peak observed in the Southeast region after two consecutive years of growing number of cases (peak of 5,794 in epiweek 19–2019, out of 191,670 cases during the whole period). After 2 years of relatively low number of cases, 2022 saw an increase of cases in the Midwest, Northeast, and North regions of the country. Finally, it is possible to notice the quick increment of cases by the end of 2022 in the Southeast region. The South region (1,285 total number of cases), historically with low numbers of urban arbovirus cases in Brazil, exhibits a noisy pattern of cases, being the only region to exhibit a peak in 2021, but also a substantial number of cases in 2016. With the observed pattern of peaks in each region differing between the years, it is possible to infer that different epidemiological and/or ecological determinants might be regulating such patterns. Since Brazil is a continental country encompassing several different latitudes and biomes, climate and vector ecology might be impacting the transmission patterns. Further studies are needed to provide insight into the heterogenous patterns of CHIKV epidemics between geographic regions. Vector competence, human population immunity, case detection, virus introduction and dispersal, among others, might help elucidate such patterns.
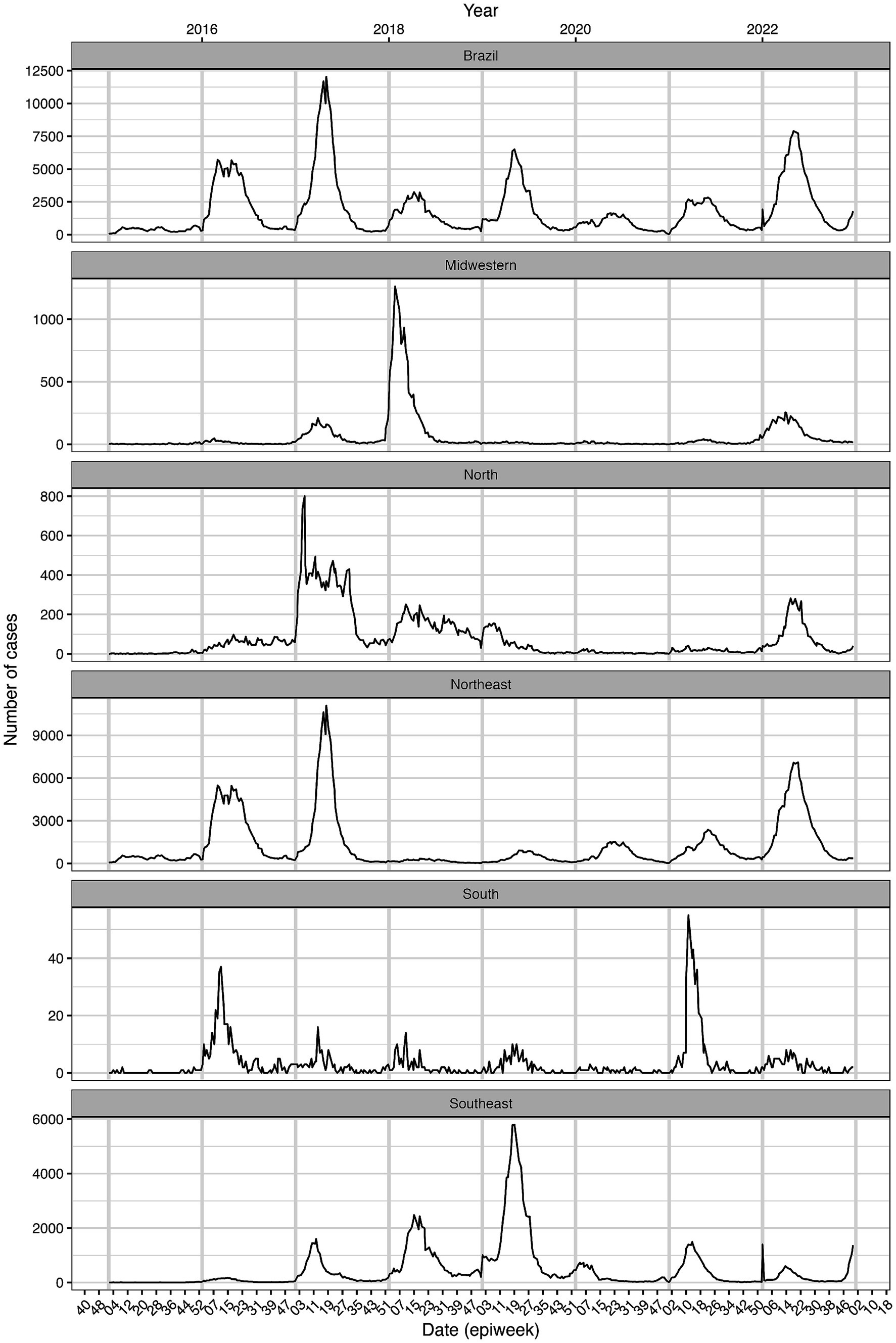
Figure 1. Time series of probable chikungunya cases in Brazil and in each of the five regions of the country: Midwest, Northeast, North, Southeast and South. Notice that the y-axis is not standardized. Source: The Brazilian national disease notification system (SINAN).
Spatial analysis of CHIKV
Figure 2 shows the spatial analysis of probable chikungunya cases in Brazil. The epidemiological elements of chikungunya fever can be discerned from the analysis of disease dissemination patterns over time. The initial occurrence of chikungunya in 2015 in Bahia and Amapá states represents a relevant epidemiological milestone. In the subsequent years, a notable concentration was observed in states within the Northeast region, specifically in 2016, with a focus on Bahia and Ceará, and in 2017, when the concentration shifted primarily to Ceará, along with the emergence of an additional focus in the Northern region, encompassing Tocantins, Southeast Pará, and Roraima (Figure 2).
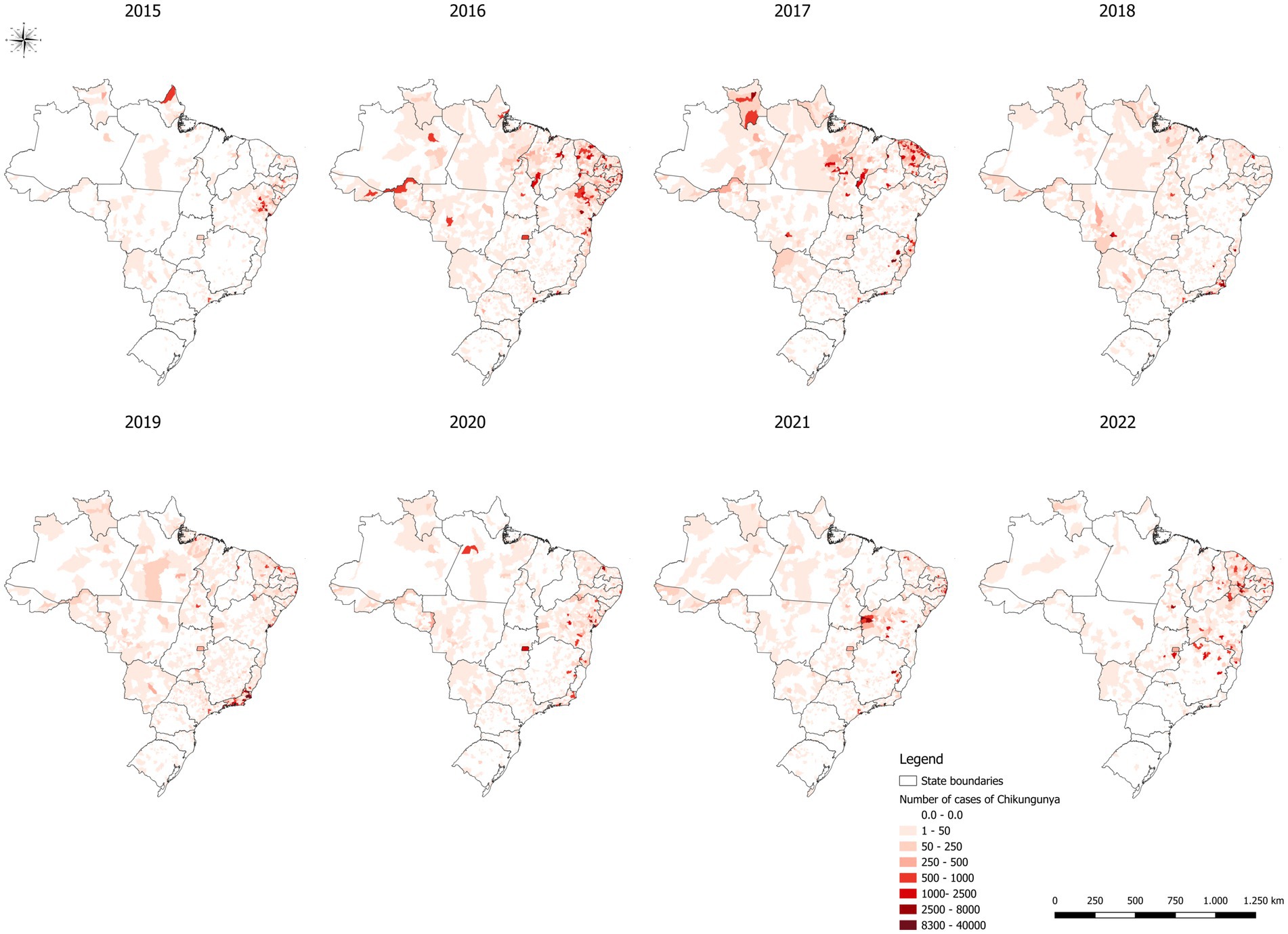
Figure 2. Spatial distribution of probable chikungunya cases in Brazil, 2015–2022. Source: The Brazilian national disease notification system (SINAN).
In 2018 and 2019 the distribution of the phenomenon predominantly centered around the state of Rio de Janeiro, with a notable decrease in its occurrence in other regions of the country. In 2020 and 2021 a resurgence of the phenomenon was observed, but with a distinct geographical distribution. It concentrated in the central and western portions of Bahia, in contrast to the patterns observed in 2016 and 2017. In 2022, the last year analyzed, a new resurgence of the phenomenon was noted in the state of Ceará and in the hinterlands of Pernambuco, as well as an expansion toward the state of Minas Gerais, specifically in its Northern region.
The spatial and temporal analysis revealed complex patterns in the dissemination of the studied phenomenon. Its initial occurrence in Bahia and Amapá in 2015 was followed by periods of concentration in different states, with significant changes in geographical distribution over the years. These variations may be related to a range of factors, including climatic, socioeconomic, and environmental factors.
Aedes albopictus
Like Ae. aegypti, Ae. albopictus is an important vector for arbovirus transmission to humans and acts as a primary vector of dengue virus in several countries, where the main vector (Ae. aegypti) is absent or rare (100–102). Aedes albopictus exhibits eclectic feeding behavior, taking blood meals from diverse animal taxa. Both Ae. albopictus and Ae. aegypti use artificial container habitats for breeding and lay eggs that can withstand dry conditions, allowing them to survive during unfavorable times of the season and facilitating geographic expansion (103–106). Evidence from field surveys (107–109) and experimental infection studies (110, 111), demonstrate vertical transmission of CHIKV from Ae. albopictus adult females to offspring, allowing for the possibility of viral maintenance during adverse environmental conditions such as drought. Also, gonotrophic discordance exhibited by Ae. albopictus (and Ae. aegypti) is predicted to strongly influence vectorial capacity (112, 113). In fact, Ae. albopictus has been shown to be an important and competent vector of more than 20 arboviruses, such as CHIKV, Mayaro, Japanese encephalitis, Rift Valley, West Nile and Sindbis viruses, having the ability to become infected with those viruses, although Ae. albopictus is not considered primary vectors of these arboviruses (101, 114–118). Table 1 highlights key biological traits of Ae. albopictus that may contribute to the role of this mosquito species as a potential vector of CHIKV.
Known as the Asian tiger mosquito, Ae. albopictus was first described in Calcutta, India, and is native to Southeast Asia and islands of the Western Pacific and Indian Ocean (119). Aedes albopictus is an exotic species that spread throughout the tropics from its native home range by human trade developments (e.g., international tire trade) (120). Aedes albopictus is one of the most commonly recognized black-and-white mosquitos, characterized by white bands on its legs, a median longitudinal stripe of silvery scales on the mesonotum and unscaled clypeus (121, 122). It is considered one of the most invasive species worldwide (119, 123, 124). Aedes albopictus can be more commonly found in areas with higher vegetation coverage and more scattered human populations, but it was also described in transitional environments with relatively low vegetation cover and frequently coexisting with Ae. aegypti (123, 125–128). It has strong plasticity, adapting to several habitats including human environments like urban and suburban areas. Despite being an opportunistic and zoophilic mosquito, when given the opportunity and choice, Ae. albopictus shows a predilection for feeding on humans over other animals (107, 129). The predilection of Ae. albopictus to feed on humans elevates its potential role as a CHIKV vector, especially in urban areas with high numbers of people and where Ae. aegypti is absent.
Blood meal analysis of Ae. albopictus at 10 distinct urban-forest interfaces in Brazil showed most blood meals were derived from mammalian hosts, with humans being disproportionately fed upon (130). Similarly, a feeding index measurement showed that Ae. albopictus commonly fed on humans and cattle in a 2-year survey in Tremembé County, State of São Paulo, Brazil (131). Patterns of host use where a majority of bloodmeals are derived from humans suggest Ae. albopictus has the potential to play a substantial role in CHIKV epidemiology. This anthropophilic trait (i.e., feeding on humans) enhances its invasiveness into human-dominated environments and is an important parameter in vectorial capacity and in determining risk of transmission for arboviruses to humans (132). Moreover, Ae. albopictus exhibits gonotrophic discordance, whereby a mosquito engages in multiple feedings during a single gonotrophic cycle, which acts to increase vectorial capacity. Beside feeding on humans, Ae. albopictus has a wide variety of hosts, including other mammalians, birds and reptiles which is considered a serious public health threat as it may be a bridge vector for many zoonotic pathogens to humans in Brazil (130, 133, 134). Moreover, Ae. albopictus mosquitoes have successfully established populations in temperate climates (101, 135), and climate change may further impact its geographic range (103, 136).
Aedes albopictus was introduced in Brazil in 1986 in the states of Rio de Janeiro, Minas Gerais, and São Paulo, located in the Southeast region of country (122, 137, 138). Subsequently, this invasive mosquito rapidly spread and expanded to other regions of the country. In 1996, Ae. albopictus was recorded for the first time in the Southern and Northern regions of Brazil in Paraná and Amazonas state, respectively (139, 140). One year later, the mosquito was identified in the state of Mato Grosso do Sul in the Midwest region of Brazil (141).
In the Northeast region of Brazil, Ae. albopictus was confirmed in Pernambuco in 1999 (142). By this time, it was estimated Ae. albopictus was already present in 14 Brazilian states (143). Before 2002, only seven states did not describe the presence of this mosquito species: Acre, Amapá, Roraima, Tocantins, Piauí, Ceará and Sergipe (144), which 10 years later changed this status to three states with no record of occurrence (100). Currently, Ae. albopictus can be found in all the 27 Brazilian states (121, 145).
Vectorial capacity and vector competence
Aiming to measure the rate of disease transmission by bloodsucking insects, previous studies proposed a mathematical model originally created for malaria vectors but later applied to other diseases, that would formulate epidemiological predictions and assess the impact of vector control strategies (146–149). Vectorial capacity, established by Garret-Jones in 1964 (150), is defined by the number of infections that a population of a given vector may distribute per day and considers several entomological parameters, such as, vector abundance and mortality, blood feeding behavior, extrinsic incubation period of the pathogen in the vector, and vector competence of the vector for the pathogen.
Vector competence is the innate capacity of a vector to acquire a pathogen, replication of the pathogen, and transmission after exposure (151). Vectorial capacity is an index controlled by genetic characteristics of the vector and pathogen and environmental conditions (152–154). For efficient transmission to occur, several factors such as arthropod and vertebrate hosts, arbovirus, and environmental conditions must converge (155).
Aedes aegypti and Ae. albopictus competencies to CHIKV may vary depending on their geographical origin and viral genotype involved in the infection. Regardless, some reports point out Ae. albopictus as more competent than Ae. aegypti (146, 152, 156–161). The demographic history of Ae. albopictus populations is the result of historical lineage diversification and divergence that associates with this species vector competence for CHIKV (162). The vector competence of Ae. albopictus populations are a key parameter in assessing the transmission and spread of a disease like chikungunya. Although the ECSA genotype of Brazilian CHIKV does not have the mutation related to adaptability to Ae. albopictus (163), which will be further addressed below, other mutations may be present and deserve further investigation.
The spread of the ECSA genotype in Brazil, which apparently replaced the Asian genotype in the Roraima state (Amazon region), suggests a greater potential for transmission of the ECSA genotype (71, 72, 164). In contrast, the CHIKV-Asian genotype has been geographically restricted mostly in the state of Amapá, in the North region (165). According to de Oliveira Ribeiro et al. (165), the pattern of CHIKV strain replacements in the Amazon region could be probably affected by different factors, including the ecological community, human behavior, and the genetics of the virus. An analysis of salivary glands and saliva from Ae. albopictus and Ae. aegypti infected with CHIKV strains R99659 (Asian genotype) and LR2006 OPY1 (IOL from ECSA genotype) (166) showed that, despite similar and high midgut infection and disseminated infection of these genotypes in both species, transmission efficiency was consistently lower for the Asian genotype in multiple strains of Ae. albopictus and Ae. aegypti, suggesting a salivary gland exit/escape barrier to the Asian genotype (166). However, both the Asian genotype and the ECSA genotype can spread and co-circulate in the country, considering the suitability of the vector species which includes widespread distribution of both Ae. aegypti and Ae. albopictus (52, 167).
Regarding Ae. albopictus vector competence for CHIKV, Vega-Rúa et al. (115) tested American and Brazilian populations of Ae. aegypti and Ae. albopictus mosquitoes for their susceptibility to three CHIKV genotypes. All populations were found to be susceptible to infection. However, CHIKV transmission efficiency varied significantly across populations, ranging from 11.1 to 96.7%. Some populations of Ae. albopictus from Rio de Janeiro for example were particularly efficient, transmitting infectious viral particles as early as 2 days post-infection. These observations suggest that efficacy of the salivary gland barrier(s) is heterogeneous for Ae. albopictus and associates with geographic origin.
In a 2018 study by Honório et al. (152), Brazilian Ae. albopictus populations were assessed for their viral dissemination rates after exposure to the CHIKV Asian genotype (GenBank accession: KJ451624) at 2-, 5-, and 13-days post-infection. The results indicated that a significant proportion (exceeding 80%) of individuals from both Aedes species developed a disseminated infection as early as 2 days post-exposure. For Ae. albopictus, Brazilian populations from Manguinhos, Rio de Janeiro demonstrated a disseminated infection rate of 82.7 ± 7.1% on the second day. Transmission rates were observed to vary across populations, with Brazilian Ae. albopictus exhibiting the highest percentage of individuals (82%) capable of transmitting CHIKV by bite (i.e., infected saliva) within 2 days post-infection.
From 2015 to 2020, the range of Ae. albopictus in Brazil expanded significantly. In 2020, it was present in 24.8% of the 2,937 surveyed municipalities (728 municipalities). Currently, this mosquito species has been detected in all Brazilian states (145) and natural infection by other arboviruses of medical and veterinary importance has been reported, such as DENV and ZIKV during an outbreak in a rural area in Brazil (168, 169). While its spread seems to be stabilizing, ongoing monitoring programs are crucial to accurately assess its distribution. In fact, authorities should implement control strategies also for Ae. albopictus, with regular surveys conducted in all Brazilian municipalities (170).
Chikungunya virus mutations impacting vector competence
Although CHIKV is generally transmitted by Ae. aegypti mosquitoes, the outbreak occurred in La Réunion island was caused by Ae. albopictus, which acted as the main vector (171, 172) due to the ECSA CHIKV genotype adaptation to this vector, as the E1-A226V, resulted in a dramatic increase in infectivity.
As a result of the increased viral fitness and vector competence, the virus transmission spread to temperate areas and caused epidemics in regions that lack the typical vector, Ae. aegypti (55, 173). In fact, the emergence of the E1-A226V mutated CHIKV during the La Reunion outbreak has been considered a well-characterized example on how a single nucleotide mutation in a virus may lead to a new epidemiological scenario given specific ecological conditions (174). Furthermore, the E1-A226V was associated with enhanced viral dissemination and higher viral loads in Ae. albopictus (56), but did not affect viral replication in Ae. aegypti (55, 173). However, when the mutation G60D in the E2 gene is in the presence of either alanine or valine at position 226 in E1 in the ECSA genotype, an increased CHIKV infectivity is observed in both Ae. albopictus and Ae. aegypti. The mutation I211T on E2 also increases viral infectivity in Ae. albopictus, but only when associated with E1-A226V (175). Interestingly, despite the long history of circulation in native areas of Ae. albopictus, CHIKV Asian genotypes have not shown the E1-A226V mutation yet (27). Lineage-specific epistatic interactions between substitutions of amino acids in positions 226 and 98 of the E1 envelope glycoprotein have largely contributed to an adaptive constraint of the Asian genotype in Ae. albopictus (176), underscoring the importance of how different adaptive landscapes can profoundly influence evolution and the emergence of closely related viral genotypes.
It has been shown that the E1-A226V mutation is impacted by the presence of both E1-98T and E2-211I residues found in most ECSA strains, including those circulating introduced in Brazil, thus impacting the potential emergence of CHIKV via Ae. albopictus transmission in parts of Africa and the Americas (175). However, it is not yet clear if ECSA strains circulating in Brazil and introduced from Angola in 2014, will select for E2-I211T, as did early IOL strains in Kenya in 2004 (47) and therefore, enabling subsequent adaptive evolution to Ae. albopictus (177), posing a major public health challenge.
The E1-A226V evolutionary adaptation illustrates a favorable viral strategy to improve its transmissibility by the vector (174), but other viral mutations are believed to be part of that strategy. It has been shown that mutations on the CHIKV envelope genes from the IOL strains, such as K252Q, K233E, L210Q in the E3 gene and R198Q/S18F in the E3/E3 may impact the initial infection of the Ae. albopictus midgut cells (12, 29, 163, 173), and by extension, viral dissemination to secondary organs and transmission. In fact, the substitutions L210Q and K252Q on E2, are associated with a higher CHIKV dissemination in Ae. albopictus (163, 173), however, the substitution K211E in the E1 gene, and V264A, in E2 resulted in an increased CHIKV dissemination and transmission in Ae. aegypti, but not in Ae. albopictus (178).
The 3’UTR plasticity of alphaviruses may also be associated to enhanced transmission and epidemic potential of viral strains. Although it has been reported that the 3’UTR enhances viral replication in a mosquito-specific manner in vitro (179), information on the role of this region on virus transmission by mosquito vectors are still scarce. A study on the 3’UTR from strains of the CHIKV Asian genotype showed that mutations occurring at that region could also contribute to vector adaptability (180). For example, infection studies with the Asian genotype of CHIKV in Ae. aegypti showed that duplication of repeated RNA elements in the 3’UTR contribute to replication kinetics and subsequent disseminated infection and transmission (181). The authors of this study suggested that mutant viruses with slower replication were less able to cause systemic infections.
Finally, the CHIKV adaptation to Ae. albopictus has been consistently associated with the spread of the disease to new areas of the world (177, 182–184), but to date, CHIKV ECSA genotypes circulating in Brazil and leading to adaptive changes in Ae. albopictus, such as E1-A226V and E2-L210Q have not been reported yet (12, 72, 164). On the other hand, CHIKV ECSA genotypes circulating in the country presented exclusive amino acids substitutions—V156A and K211T (72), and using a mosquito-to-mouse transmission model, it has been suggested that those mutations may play an important role in CHIKV biology, such as modulation of virus attachment and fusion (185). As RNA viruses present high mutation rates leading to genetically and potentially emergent viral genotypes, and the interaction with the host as well distinct ecological conditions may shape how those will evolve and impact its transmission, studies of molecular characterization of viral strains and vector biology are still needed.
Author contributions
MR: Conceptualization, Writing – original draft, Writing – review & editing. DC: Data curation, Formal analysis, Writing – review & editing. FS: Funding acquisition, Writing – review & editing. JS: Data curation, Formal analysis, Writing – review & editing. BA: Conceptualization, Writing – original draft, Writing – review & editing. NH: Conceptualization, Data curation, Formal analysis, Funding acquisition, Writing – original draft, Writing – review & editing.
Funding
The author(s) declare that financial support was received for the research, authorship, and/or publication of this article. This study was supported by the Programa de Pós-Graduação em Medicina Tropical—Instituto Oswaldo Cruz/Fiocruz, Brazil, CNPq and Coordenação de Aperfeiçoamento de Pessoal de Nível Superior (CAPES) - Finance Code 001 for students’ fellowship, Fundação de Amparo à Pesquisa do Estado do Rio de Janeiro (FAPERJ) - grant numbers E-26/202.736/2018 and E-26/201.207/2022 to NAH, grant numbers 303146/2022–2, E-26/202.659/2019, E-26/211.344/2021 and E-26/200.407/2023 to FBdS, the Fundação Oswaldo Cruz (Fiocruz), Conselho Nacional de Desenvolvimento Científico e Tecnológico (CNPq) grant number 303146/2022–2 to FBS. Funders had no role in the study design, data collection, analysis, and decision to publish or preparation of the manuscript.
Conflict of interest
The authors declare that the research was conducted in the absence of any commercial or financial relationships that could be construed as a potential conflict of interest.
Publisher’s note
All claims expressed in this article are solely those of the authors and do not necessarily represent those of their affiliated organizations, or those of the publisher, the editors and the reviewers. Any product that may be evaluated in this article, or claim that may be made by its manufacturer, is not guaranteed or endorsed by the publisher.
References
1. Girard, M, Nelson, CB, Picot, V, and Gubler, DJ. Arboviruses: a global public health threat. Vaccine. (2020) 38:3989–94. doi: 10.1016/j.vaccine.2020.04.011
2. WHO. Chikungunya. (2022). Available at: https://www.who.int/news-room/fact-sheets/detail/chikungunya. (Accessed December 2, 2024).
3. Diallo, M, Thonnon, J, Traore-Lamizana, M, and Fontenille, D. Vectors of chikungunya virus in Senegal: current data and transmission cycles. Am J Trop Med Hyg. (1999) 60:281–6. doi: 10.4269/ajtmh.1999.60.281
4. Honório, NA, Câmara, DC, Calvet, GA, and Brasil, P. Chikungunya: an arbovirus infection in the process of establishment and expansion in Brazil. Cad Saude Publica. (2015) 31:906–8. doi: 10.1590/0102-311XPE020515
5. Staples, JE, Breiman, RF, and Powers, AM. Chikungunya fever: an epidemiological review of a re-emerging infectious disease. Clin Infect Dis. (2009) 49:942–8. doi: 10.1086/605496
6. Thiberville, SD, Boisson, V, Gaudart, J, Simon, F, Flahault, A, and de Lamballerie, X. Chikungunya fever: a clinical and virological investigation of outpatients on Reunion Island, south-West Indian Ocean. PLoS Negl Trop Dis. (2013) 7:e2004. doi: 10.1371/journal.pntd.0002004
7. Nakkhara, P, Chongsuvivatwong, V, and Thammapalo, S. Risk factors for symptomatic and asymptomatic chikungunya infection. Trans R Soc Trop Med Hyg. (2013) 107:789–96. doi: 10.1093/trstmh/trt083
8. Slavov, SN, Otaguiri, KK, Bianquini, ML, Bitencourt, HTO, Chagas, MCM, Guerreiro, DSS, et al. Seroprevalence of chikungunya virus in blood donors from northern and southeastern Brazil. Hematol Transfus Cell Ther. (2018) 40:358–62. doi: 10.1016/j.htct.2018.04.002
9. Rezza, G, and Weaver, SC. Chikungunya as a paradigm for emerging viral diseases: evaluating disease impact and hurdles to vaccine development. PLoS Negl Trop Dis. (2019) 13:e0006919. doi: 10.1371/journal.pntd.0006919
10. Elsinga, J, Grobusch, MP, Tami, A, Gerstenbluth, I, and Bailey, A. Health-related impact on quality of life and coping strategies for chikungunya: a qualitative study in Curaçao. PLoS Negl Trop Dis. (2017) 11:e0005987. doi: 10.1371/journal.pntd.0005987
11. Couzigou, B, Criquet-Hayot, A, Javelle, E, Tignac, S, Mota, E, Rigaud, F, et al. Occurrence of chronic stage chikungunya in the general population of Martinique during the first 2014 epidemic: a prospective epidemiological study. Am J Trop Med Hyg. (2018) 99:182–90. doi: 10.4269/ajtmh.17-0543
12. Cunha, MS, Costa, PAG, Correa, IA, de Souza, MRM, Calil, PT, da Silva, GPD, et al. Chikungunya virus: an emergent arbovirus to the south American continent and a continuous threat to the world. Front Microbiol. (2020) 11:1297. doi: 10.3389/fmicb.2020.01297
13. Dutra, JIS, Souza, MC, Lins, CAA, and Medeiros, ACQ. Impact of chronic illness caused by chikungunya fever on quality of life and functionality. Einstein (São Paulo). (2024) 22:eAO0562. doi: 10.31744/einstein_journal/2024AO0562
14. McCarthy, MK, Davenport, BJJ, and Morrison, TE. Chronic chikungunya virus disease. Curr Top Microbiol Immunol. (2019) 435:55–80. doi: 10.1007/82_2018_147
15. Mehta, R, Soares, CN, Medialdea-Carrera, R, Ellul, M, da Silva, MTT, Rosala-Hallas, A, et al. The spectrum of neurological disease associated with Zika and chikungunya viruses in adults in Rio de Janeiro, Brazil: a case series. PLoS Negl Trop Dis. (2018) 12:e0006212. doi: 10.1371/journal.pntd.0006212
16. Mello, CDS, Cabral-Castro, MJ, Silva de Faria, LC, Peralta, JM, and Puccioni-Sohler, M. Dengue and chikungunya infection in neurologic disorders from endemic areas in Brazil. Neurol Clin Pract. (2020) 10:497–502. doi: 10.1212/CPJ.0000000000000776
17. Robinson, MC. An epidemic of virus disease in Southern Province, Tanganyika territory, in 1952-53. I. Clinical features. Trans R Soc Trop Med Hyg. (1955) 49:28–32. doi: 10.1016/0035-9203(55)90080-8
18. Ross, RW. The Newala epidemic. III. The virus: isolation, pathogenic properties and relationship to the epidemic. J Hyg. (1956) 54:177–91. doi: 10.1017/S0022172400044442
19. Mason, PJ, and Haddow, AJ. An epidemic of virus disease in Southern Province, Tanganyika territory, in 1952-53; an additional note on chikungunya virus isolations and serum antibodies. Trans R Soc Trop Med Hyg. (1957) 51:238–40. doi: 10.1016/0035-9203(57)90022-6
20. Weinbren, MP, Haddow, AJ, and Williams, MC. The occurrence of chikungunya virus in Uganda. I. Isolation from mosquitoes. Trans R Soc Trop Med Hyg. (1958) 52:253–62. doi: 10.1016/0035-9203(58)90084-1
21. Paterson, HE, and Mcintosh, BM. Further studies on the chikungunya outbreak in southern Rhodesia IN 1962. II. Transmission experiments with the Aedes furcifer-taylori group of mosquitoes and with a member of the Anopheles gambiae complex. Ann Trop Med Parasitol. (1964) 58:52–5.
22. Pialoux, G, Gaüzère, BA, Jauréguiberry, S, and Strobel, M. Chikungunya, an epidemic arbovirosis. Lancet Infect Dis. (2007) 7:319–27. doi: 10.1016/S1473-3099(07)70107-X
23. Weaver, SC, and Reisen, WK. Present and future arboviral threats. Antivir Res. (2010) 85:328–45. doi: 10.1016/j.antiviral.2009.10.008
24. Petersen, LR, and Powers, AM. Chikungunya: epidemiology. F1000Res. (2016) 5:5. doi: 10.12688/f1000research.7171.1
25. Hammon, WM, Rudnick, A, and Sather, GE. Viruses associated with epidemic hemorrhagic fevers of the Philippines and Thailand. Science. (1960) 131:1102–3. doi: 10.1126/science.131.3407.1102
26. Powers, AM, Brault, AC, Tesh, RB, and Weaver, SC. Re-emergence of chikungunya and O'nyong-nyong viruses: evidence for distinct geographical lineages and distant evolutionary relationships. J Gen Virol. (2000) 81:471–9. doi: 10.1099/0022-1317-81-2-471
27. Volk, SM, Chen, R, Tsetsarkin, KA, Adams, AP, Garcia, TI, Sall, AA, et al. Genome-scale phylogenetic analyses of chikungunya virus reveal independent emergences of recent epidemics and various evolutionary rates. J Virol. (2010) 84:6497–504. doi: 10.1128/JVI.01603-09
28. Sharp, TM, Roth, NM, Torres, J, Ryff, KR, Pérez Rodríguez, NM, Mercado, C, et al. Chikungunya cases identified through passive surveillance and household investigations--Puerto Rico, May 5-August 12, 2014. MMWR Morb Mortal Wkly Rep. (2014) 63:1121–8.
29. Vairo, F, Haider, N, Kock, R, Ntoumi, F, Ippolito, G, and Zumla, A. Chikungunya: epidemiology, pathogenesis, clinical features, management, and prevention. Infect Dis Clin N Am. (2019) 33:1003–25. doi: 10.1016/j.idc.2019.08.006
30. Schilte, C, Staikowsky, F, Staikovsky, F, Couderc, T, Madec, Y, Carpentier, F, et al. Chikungunya virus-associated long-term arthralgia: a 36-month prospective longitudinal study. PLoS Negl Trop Dis. (2013) 7:e2137. doi: 10.1371/journal.pntd.0002137
31. Weaver, SC, and Forrester, NL. Chikungunya: evolutionary history and recent epidemic spread. Antivir Res. (2015) 120:32–9. doi: 10.1016/j.antiviral.2015.04.016
32. Rezza, G, Nicoletti, L, Angelini, R, Romi, R, Finarelli, AC, Panning, M, et al. Infection with chikungunya virus in Italy: an outbreak in a temperate region. Lancet. (2007) 370:1840–6. doi: 10.1016/S0140-6736(07)61779-6
33. Lanciotti, RS, Kosoy, OL, Laven, JJ, Panella, AJ, Velez, JO, Lambert, AJ, et al. Chikungunya virus in US travelers returning from India, 2006. Emerg Infect Dis. (2007) 13:764–7. doi: 10.3201/eid1305.070015
34. PAHO. Preparedness and response for chikungunya virus introduction in the Americas. (2011). Available at: https://iris.paho.org/handle/10665.2/4009 (Accessed December 2, 2024).
35. Díaz-González, EE, Kautz, TF, Dorantes-Delgado, A, Malo-García, IR, Laguna-Aguilar, M, Langsjoen, RM, et al. First report of Aedes aegypti transmission of chikungunya virus in the Americas. Am J Trop Med Hyg. (2015) 93:1325–9. doi: 10.4269/ajtmh.15-0450
36. Puntasecca, CJ, King, CH, and LaBeaud, AD. Measuring the global burden of chikungunya and Zika viruses: a systematic review. PLoS Negl Trop Dis. (2021) 15:e0009055. doi: 10.1371/journal.pntd.0009055
37. Figueiredo, ML, and Figueiredo, LT. Emerging alphaviruses in the Americas: chikungunya and Mayaro. Rev Soc Bras Med Trop. (2014) 47:677–83. doi: 10.1590/0037-8682-0246-2014
38. PAHO. Number of reported cases of chikungunya in the Americas, by country weekly Washington DC (2023). Available at: https://www3.paho.org/data/index.php/en/mnu-topics/chikv-en/550-chikv-weekly-en.html (Accessed December 2, 2024).
39. Powers, AM, Brault, AC, Shirako, Y, Strauss, EG, Kang, W, Strauss, JH, et al. Evolutionary relationships and systematics of the alphaviruses. J Virol. (2001) 75:10118–31. doi: 10.1128/JVI.75.21.10118-10131.2001
40. Burt, FJ, Chen, W, Miner, JJ, Lenschow, DJ, Merits, A, Schnettler, E, et al. Chikungunya virus: an update on the biology and pathogenesis of this emerging pathogen. Lancet Infect Dis. (2017) 17:e107–17. doi: 10.1016/S1473-3099(16)30385-1
41. Neuvonen, M, Kazlauskas, A, Martikainen, M, Hinkkanen, A, Ahola, T, and Saksela, K. SH3 domain-mediated recruitment of host cell amphiphysins by alphavirus nsP3 promotes viral RNA replication. PLoS Pathog. (2011) 7:e1002383. doi: 10.1371/journal.ppat.1002383
42. Wang, YF, Sawicki, SG, and Sawicki, DL. Alphavirus nsP3 functions to form replication complexes transcribing negative-strand RNA. J Virol. (1994) 68:6466–75. doi: 10.1128/jvi.68.10.6466-6475.1994
43. Petitdemange, C, Wauquier, N, and Vieillard, V. Control of immunopathology during chikungunya virus infection. J Allergy Clin Immunol. (2015) 135:846–55. doi: 10.1016/j.jaci.2015.01.039
44. Reddy, A, Bosch, I, Salcedo, N, Herrera, BB, de Puig, H, Narváez, CF, et al. Development and validation of a rapid lateral flow E1/E2-antigen test and ELISA in patients infected with emerging asian strain of chikungunya virus in the Americas. Viruses. (2020) 12:971. doi: 10.3390/v12090971
45. Chen, R, Wang, E, Tsetsarkin, KA, and Weaver, SC. Chikungunya virus 3′ untranslated region: adaptation to mosquitoes and a population bottleneck as major evolutionary forces. PLoS Pathog. (2013) 9:e1003591. doi: 10.1371/journal.ppat.1003591
46. Silva, LA, and Dermody, TS. Chikungunya virus: epidemiology, replication, disease mechanisms, and prospective intervention strategies. J Clin Invest. (2017) 127:737–49. doi: 10.1172/JCI84417
47. Kariuki Njenga, M, Nderitu, L, Ledermann, JP, Ndirangu, A, Logue, CH, Kelly, CH, et al. Tracking epidemic chikungunya virus into the Indian Ocean from East Africa. J Gen Virol. (2008) 89:2754–60. doi: 10.1099/vir.0.2008/005413-0
48. Charrel, RN, and de Lamballerie, X. Chikungunya virus in North-Eastern Italy: a consequence of seasonal synchronicity. Euro Surveill. (2008) 13:3–4. doi: 10.2807/ese.13.01.08003-en
49. Centers for Disease Control and Prevention. Update on emerging infections: news from the Centers for Disease Control and Prevention. Notes from the field: chikungunya virus spreads in the Americas-Caribbean and South America, 2013-2014. Ann Emerg Med. (2014) 64:552–3. doi: 10.1016/j.annemergmed.2014.08.002
50. Pulmanausahakul, R, Roytrakul, S, Auewarakul, P, and Smith, DR. Chikungunya in Southeast Asia: understanding the emergence and finding solutions. Int J Infect Dis. (2011) 15:e671–6. doi: 10.1016/j.ijid.2011.06.002
51. Powers, AM, and Logue, CH. Changing patterns of chikungunya virus: re-emergence of a zoonotic arbovirus. J Gen Virol. (2007) 88:2363–77. doi: 10.1099/vir.0.82858-0
52. Nunes, MR, Faria, NR, de Vasconcelos, JM, Golding, N, Kraemer, MU, de Oliveira, LF, et al. Emergence and potential for spread of chikungunya virus in Brazil. BMC Med. (2015) 13:102. doi: 10.1186/s12916-015-0348-x
53. Wahid, B, Ali, A, Rafique, S, and Idrees, M. Global expansion of chikungunya virus: mapping the 64-year history. Int J Infect Dis. (2017) 58:69–76. doi: 10.1016/j.ijid.2017.03.006
54. Schuffenecker, I, Iteman, I, Michault, A, Murri, S, Frangeul, L, Vaney, MC, et al. Genome microevolution of chikungunya viruses causing the Indian Ocean outbreak. PLoS Med. (2006) 3:e263. doi: 10.1371/journal.pmed.0030263
55. Tsetsarkin, KA, Vanlandingham, DL, McGee, CE, and Higgs, S. A single mutation in chikungunya virus affects vector specificity and epidemic potential. PLoS Pathog. (2007) 3:e201. doi: 10.1371/journal.ppat.0030201
56. Vazeille, M, Moutailler, S, Coudrier, D, Rousseaux, C, Khun, H, Huerre, M, et al. Two chikungunya isolates from the outbreak of La Reunion (Indian Ocean) exhibit different patterns of infection in the mosquito, Aedes albopictus. PLoS One. (2007) 2:e1168. doi: 10.1371/journal.pone.0001168
57. Josseran, L, Paquet, C, Zehgnoun, A, Caillere, N, Le Tertre, A, Solet, JL, et al. Chikungunya disease outbreak, Reunion Island. Emerg Infect Dis. (2006) 12:1994–5. doi: 10.3201/eid1212.060710
58. Silva, JVJ, Ludwig-Begall, LF, Oliveira-Filho, EF, Oliveira, RAS, Durães-Carvalho, R, Lopes, TRR, et al. A scoping review of chikungunya virus infection: epidemiology, clinical characteristics, viral co-circulation complications, and control. Acta Trop. (2018) 188:213–24. doi: 10.1016/j.actatropica.2018.09.003
59. Weaver, SC, Chen, R, and Diallo, M. Chikungunya virus: role of vectors in emergence from enzootic cycles. Annu Rev Entomol. (2020) 65:313–32. doi: 10.1146/annurev-ento-011019-025207
60. Arankalle, VA, Shrivastava, S, Cherian, S, Gunjikar, RS, Walimbe, AM, Jadhav, SM, et al. Genetic divergence of chikungunya viruses in India (1963-2006) with special reference to the 2005-2006 explosive epidemic. J Gen Virol. (2007) 88:1967–76. doi: 10.1099/vir.0.82714-0
61. Schneider, AB, Ochsenreiter, R, Hostager, R, Hofacker, IL, Janies, D, and Wolfinger, MT. Updated phylogeny of chikungunya virus suggests lineage-specific RNA architecture. Viruses. (2019) 11:798. doi: 10.3390/v11090798
62. Teixeira, MG, Costa, MC, Barreto, ML, and Barreto, FR. Epidemiology of dengue in Salvador-Bahia, 1995-1999. Rev Soc Bras Med Trop. (2001) 34:269–74. doi: 10.1590/S0037-86822001000300007
63. Tauro, LB, Cardoso, CW, Souza, RL, Nascimento, LC, Santos, DRD, Campos, GS, et al. A localized outbreak of chikungunya virus in Salvador, Bahia, Brazil. Mem Inst Oswaldo Cruz. (2019) 114:e180597. doi: 10.1590/0074-02760180597
64. Goes de Jesus, J, da Luz, WG, Lima Maia, M, Xavier, J, Oliveira Lima, MA, Fonseca, V, et al. Persistence of chikungunya ECSA genotype and local outbreak in an upper medium class neighborhood in Northeast Brazil. PLoS One. (2020) 15:e0226098. doi: 10.1371/journal.pone.0226098
65. Costa, ALFA, Martins, TGDS, and Martins, DGDS. Third cranial nerve palsy after a chikungunya virus infection. Strabismus. (2017) 25:172–5. doi: 10.1080/09273972.2017.1391851
66. Tanabe, ELL, Tanabe, ISB, Santos, ECD, Marques, JPDS, Borges, AA, Lima, MC, et al. Report of east-central south African chikungunya virus genotype during the 2016 outbreak in the Alagoas state, Brazil. Rev Inst Med Trop Sao Paulo. (2018) 60:e19. doi: 10.1590/s1678-9946201860019
67. Cardoso, FD, Rezende, IM, Barros, ELT, Sacchetto, L, Garcês, TCCS, Silva, NIO, et al. Circulation of chikungunya virus east-Central-South Africa genotype during an outbreak in 2016-17 in Piaui state, Northeast Brazil. Rev Inst Med Trop Sao Paulo. (2019) 61:e57. doi: 10.1590/s1678-9946201961057
68. Costa-da-Silva, AL, Ioshino, RS, Petersen, V, Lima, AF, Cunha, MDP, Wiley, MR, et al. First report of naturally infected Aedes aegypti with chikungunya virus genotype ECSA in the Americas. PLoS Negl Trop Dis. (2017) 11:e0005630. doi: 10.1371/journal.pntd.0005630
69. Jesus, MCS, Chagas, RDO, Santos, CA, Santos, RWF, Barros, GS, La Corte, R, et al. Molecular characterization and phylogenetic analysis of chikungunya virus during the 2016 outbreak in Sergipe, northeastern Brazil. Trans R Soc Trop Med Hyg. (2021) 115:779–84. doi: 10.1093/trstmh/traa123
70. Aragão, CF, Cruz, ACR, Nunes Neto, JP, Monteiro, HAO, da Silva, EVP, da Silva, SP, et al. Circulation of chikungunya virus in Aedes aegypti in Maranhão, Northeast Brazil. Acta Trop. (2018) 186:1–4. doi: 10.1016/j.actatropica.2018.06.022
71. Naveca, FG, Claro, I, Giovanetti, M, de Jesus, JG, Xavier, J, Iani, FCM, et al. Genomic, epidemiological and digital surveillance of chikungunya virus in the Brazilian Amazon. PLoS Negl Trop Dis. (2019) 13:e0007065. doi: 10.1371/journal.pntd.0007065
72. Souza, TM, Azeredo, EL, Badolato-Corrêa, J, Damasco, PV, Santos, C, Petitinga-Paiva, F, et al. First report of the east-central south African genotype of chikungunya virus in Rio de Janeiro, Brazil. PLoS Curr. (2017):9. doi: 10.1371/currents.outbreaks.4200119978d62ccaa454599cd2735727
73. de Souza, TMA, Ribeiro, ED, Corrêa, VCE, Damasco, PV, Santos, CC, de Bruycker-Nogueira, F, et al. Following in the footsteps of the chikungunya virus in Brazil: the first autochthonous cases in Amapá in 2014 and its emergence in Rio de Janeiro during 2016. Viruses. (2018) 10:623. doi: 10.3390/v10110623
74. Xavier, J, Giovanetti, M, Fonseca, V, Thézé, J, Gräf, T, Fabri, A, et al. Circulation of chikungunya virus east/central/south African lineage in Rio de Janeiro, Brazil. PLoS One. (2019) 14:e0217871. doi: 10.1371/journal.pone.0217871
75. Fabri, AA, Rodrigues, CDDS, Santos, CCD, Chalhoub, FLL, Sampaio, SA, Faria, NRDC, et al. Co-circulation of two independent clades and persistence of CHIKV-ECSA genotype during epidemic waves in Rio de Janeiro, Southeast Brazil. Pathogens. (2020) 9:984. doi: 10.3390/pathogens9120984
76. Lessa-Aquino, C, Trinta, KS, Pestana, CP, Ribeiro, MO, Sucupira, MVF, Boia, MN, et al. Detection of east/central/south African genotype chikungunya virus during an outbreak in a southeastern state of Brazil. Epidemiol Infect. (2018) 146:2056–8. doi: 10.1017/S0950268818002467
77. de Souza Costa, MC, Siqueira Maia, LM, Costa de Souza, V, Gonzaga, AM, Correa de Azevedo, V, Ramos Martins, L, et al. Arbovirus investigation in patients from Mato Grosso during Zika and chikungunya virus introdution in Brazil, 2015-2016. Acta Trop. (2019) 190:395–402. doi: 10.1016/j.actatropica.2018.12.019
78. Gregianini, TS, Ranieri, T, Favreto, C, Nunes, ZMA, Tumioto Giannini, GL, Sanberg, ND, et al. Emerging arboviruses in Rio Grande do Sul, Brazil: chikungunya and Zika outbreaks, 2014-2016. Rev Med Virol. (2017) 27:rmv.1943. doi: 10.1002/rmv.1943
79. de Souza, WM, Ribeiro, GS, de Lima, STS, de Jesus, R, Moreira, FRR, Whittaker, C, et al. Chikungunya: a decade of burden in the Americas. Lancet Reg Health Am. (2024) 30:100673. doi: 10.1016/j.lana.2023.100673
80. Souza, TML, Vieira, YR, Delatorre, E, Barbosa-Lima, G, Luiz, RLF, Vizzoni, A, et al. Emergence of the east-central-south-African genotype of chikungunya virus in Brazil and the city of Rio de Janeiro may have occurred years before surveillance detection. Sci Rep. (2019) 9:2760. doi: 10.1038/s41598-019-39406-9
81. Moreira, FRR, Menezes, MT, Salgado-Benvindo, C, Whittaker, C, Cox, V, Chandradeva, N, et al. Epidemiological and genomic investigation of chikungunya virus in Rio de Janeiro state, Brazil, between 2015 and 2018. PLoS Negl Trop Dis. (2023) 17:e0011536. doi: 10.1371/journal.pntd.0011536
82. Charlys da Costa, A, Thézé, J, Komninakis, SCV, Sanz-Duro, RL, Felinto, MRL, Moura, LCC, et al. Spread of chikungunya virus east/central/south African genotype in Northeast Brazil. Emerg Infect Dis. (2017) 23:1742–4. doi: 10.3201/eid2310.170307
83. Xavier, J, Fonseca, V, Bezerra, JF, do Monte Alves, M, Mares-Guia, MA, Claro, IM, et al. Chikungunya virus ECSA lineage reintroduction in the northeasternmost region of Brazil. Int J Infect Dis. (2021) 105:120–3. doi: 10.1016/j.ijid.2021.01.026
84. Rico-Hesse, R, Harrison, LM, Salas, RA, Tovar, D, Nisalak, A, Ramos, C, et al. Origins of dengue type 2 viruses associated with increased pathogenicity in the Americas. Virology. (1997) 230:244–51. doi: 10.1006/viro.1997.8504
85. Armstrong, PM, and Rico-Hesse, R. Differential susceptibility of Aedes aegypti to infection by the American and southeast Asian genotypes of dengue type 2 virus. Vector Borne Zoonotic Dis. (2001) 1:159–68. doi: 10.1089/153036601316977769
86. Rico-Hesse, R. Microevolution and virulence of dengue viruses. Adv Virus Res. (2003) 59:315–41. doi: 10.1016/S0065-3527(03)59009-1
87. Cologna, R, Armstrong, PM, and Rico-Hesse, R. Selection for virulent dengue viruses occurs in humans and mosquitoes. J Virol. (2005) 79:853–9. doi: 10.1128/JVI.79.2.853-859.2005
88. Cologna, R, and Rico-Hesse, R. American genotype structures decrease dengue virus output from human monocytes and dendritic cells. J Virol. (2003) 77:3929–38. doi: 10.1128/JVI.77.7.3929-3938.2003
89. Vu, TT, Holmes, EC, Duong, V, Nguyen, TQ, Tran, TH, Quail, M, et al. Emergence of the Asian 1 genotype of dengue virus serotype 2 in Viet Nam: in vivo fitness advantage and lineage replacement in South-East Asia. PLoS Negl Trop Dis. (2010) 4:e757. doi: 10.1371/journal.pntd.0000757
90. Tsetsarkin, K, Higgs, S, McGee, CE, De Lamballerie, X, Charrel, RN, and Vanlandingham, DL. Infectious clones of chikungunya virus (La Réunion isolate) for vector competence studies. Vector Borne Zoonotic Dis. (2006) 6:325–37. doi: 10.1089/vbz.2006.6.325
91. Simon, F, Savini, H, and Parola, P. Chikungunya: a paradigm of emergence and globalization of vector-borne diseases. Med Clin North Am. (2008) 92:1323–43, ix. doi: 10.1016/j.mcna.2008.07.008
92. Mohan, A, Kiran, DH, Manohar, IC, and Kumar, DP. Epidemiology, clinical manifestations, and diagnosis of chikungunya fever: lessons learned from the re-emerging epidemic. Indian J Dermatol. (2010) 55:54–63. doi: 10.4103/0019-5154.60355
93. Kraemer, MU, Sinka, ME, Duda, KA, Mylne, A, Shearer, FM, Brady, OJ, et al. The global compendium of Aedes aegypti and Ae. albopictus occurrence. Sci Data. (2015) 2:150035. doi: 10.1038/sdata.2015.35
94. Lima-Camara, TN. Emerging arboviruses and public health challenges in Brazil. Rev Saude Publica. (2016) 50:36. doi: 10.1590/S1518-8787.2016050006791
95. Kotsakiozi, P, Gloria-Soria, A, Caccone, A, Evans, B, Schama, R, Martins, AJ, et al. Tracking the return of Aedes aegypti to Brazil, the major vector of the dengue, chikungunya and Zika viruses. PLoS Negl Trop Dis. (2017) 11:e0005653. doi: 10.1371/journal.pntd.0005653
96. Reinhold, JM, Lazzari, CR, and Lahondère, C. Effects of the environmental temperature on Aedes aegypti and Aedes albopictus mosquitoes: a review. Insects. (2018) 9:158. doi: 10.3390/insects9040158
97. Benelli, G, and Mehlhorn, H. Declining malaria, rising of dengue and Zika virus: insights for mosquito vector control. Parasitol Res. (2016) 115:1747–54. doi: 10.1007/s00436-016-4971-z
98. Brasil, P, Calvet, GA, Siqueira, AM, Wakimoto, M, de Sequeira, PC, Nobre, A, et al. Zika virus outbreak in Rio de Janeiro, Brazil: clinical characterization, epidemiological and virological aspects. PLoS Negl Trop Dis. (2016) 10:e0004636. doi: 10.1371/journal.pntd.0004636
99. Silva, JVJ, Lopes, TRR, Oliveira-Filho, EF, Oliveira, RAS, Durães-Carvalho, R, and Gil, LHVG. Current status, challenges and perspectives in the development of vaccines against yellow fever, dengue, Zika and chikungunya viruses. Acta Trop. (2018) 182:257–63. doi: 10.1016/j.actatropica.2018.03.009
100. Pancetti, FG, Honório, NA, Urbinatti, PR, and Lima-Camara, TN. Twenty-eight years of Aedes albopictus in Brazil: a rationale to maintain active entomological and epidemiological surveillance. Rev Soc Bras Med Trop. (2015) 48:87–9. doi: 10.1590/0037-8682-0155-2014
101. Gratz, NG. Critical review of the vector status of Aedes albopictus. Med Vet Entomol. (2004) 18:215–27. doi: 10.1111/j.0269-283X.2004.00513.x
103. Alto, BW, and Juliano, SA. Temperature effects on the dynamics of Aedes albopictus (Diptera: Culicidae) populations in the laboratory. J Med Entomol. (2001) 38:548–56. doi: 10.1603/0022-2585-38.4.548
104. Juliano, SA, and Lounibos, LP. Ecology of invasive mosquitoes: effects on resident species and on human health. Ecol Lett. (2005) 8:558–74. doi: 10.1111/j.1461-0248.2005.00755.x
105. Honório, NA, Nogueira, RM, Codeço, CT, Carvalho, MS, Cruz, OG, MeA, M, et al. Spatial evaluation and modeling of dengue seroprevalence and vector density in Rio de Janeiro, Brazil. PLoS Negl Trop Dis. (2009) 3:e545. doi: 10.1371/journal.pntd.0000545
106. Scott, TW, and Takken, W. Feeding strategies of anthropophilic mosquitoes result in increased risk of pathogen transmission. Trends Parasitol. (2012) 28:114–21. doi: 10.1016/j.pt.2012.01.001
107. Delatte, H, Dehecq, JS, Thiria, J, Domerg, C, Paupy, C, and Fontenille, D. Geographic distribution and developmental sites of Aedes albopictus (Diptera: Culicidae) during a chikungunya epidemic event. Vector Borne Zoonotic Dis. (2008) 8:25–34. doi: 10.1089/vbz.2007.0649
108. Jain, J, Kushwah, RBS, Singh, SS, Sharma, A, Adak, T, Singh, OP, et al. Evidence for natural vertical transmission of chikungunya viruses in field populations of Aedes aegypti in Delhi and Haryana states in India-a preliminary report. Acta Trop. (2016) 162:46–55. doi: 10.1016/j.actatropica.2016.06.004
109. Niyas, KP, Abraham, R, Unnikrishnan, RN, Mathew, T, Nair, S, Manakkadan, A, et al. Molecular characterization of chikungunya virus isolates from clinical samples and adult Aedes albopictus mosquitoes emerged from larvae from Kerala, South India. Virol J. (2010) 7:189. doi: 10.1186/1743-422X-7-189
110. Chompoosri, J, Thavara, U, Tawatsin, A, Boonserm, R, Phumee, A, Sangkitporn, S, et al. Vertical transmission of Indian Ocean lineage of chikungunya virus in Aedes aegypti and Aedes albopictus mosquitoes. Parasit Vectors. (2016) 9:227. doi: 10.1186/s13071-016-1505-6
111. Honório, NA, Wiggins, K, Eastmond, B, Câmara, DCP, and Alto, BW. Experimental vertical transmission of chikungunya virus by Brazilian and Florida Aedes albopictus populations. Viruses. (2019) 11:353. doi: 10.3390/v11040353
112. Fernández, Z, and Forattini, OP. Sobrevivência de populações de Aedes albopictus: idade fisiológica e história reprodutiva. Rev Saude Publica. (2003) 37:285–91. doi: 10.1590/S0034-89102003000300004
113. Dos Santos, AP, Urbinatti, PR, da Rocha, CR, de Sá Almeida, RMM, Ribeiro, SS, and de Lima-Camara, TN. Parity and gonotrophic discordance of females of Aedes aegypti and Aedes albopictus (Diptera: Culicidae) in the city of São Paulo, SP, Brazil. J Vector Ecol. (2019) 44:233–40. doi: 10.1111/jvec.12354
114. Paupy, C, Delatte, H, Bagny, L, Corbel, V, and Fontenille, D. Aedes albopictus, an arbovirus vector: from the darkness to the light. Microbes Infect. (2009) 11:1177–85. doi: 10.1016/j.micinf.2009.05.005
115. Vega-Rúa, A, Zouache, K, Girod, R, Failloux, AB, and Lourenço-de-Oliveira, R. High level of vector competence of Aedes aegypti and Aedes albopictus from ten American countries as a crucial factor in the spread of chikungunya virus. J Virol. (2014) 88:6294–306. doi: 10.1128/JVI.00370-14
116. Medlock, JM, Hansford, KM, Versteirt, V, Cull, B, Kampen, H, Fontenille, D, et al. An entomological review of invasive mosquitoes in Europe. Bull Entomol Res. (2015) 105:637–63. doi: 10.1017/S0007485315000103
117. Wiggins, K, Eastmond, B, and Alto, BW. Transmission potential of Mayaro virus in Florida Aedes aegypti and Aedes albopictus mosquitoes. Med Vet Entomol. (2018) 32:436–42. doi: 10.1111/mve.12322
118. Lwande, OW, Obanda, V, Lindström, A, Ahlm, C, Evander, M, Näslund, J, et al. Globe-trotting Aedes aegypti and Aedes albopictus: Risk factors for arbovirus pandemics. Vector Borne Zoonotic Dis. (2020) 20:71–81. doi: 10.1089/vbz.2019.2486
119. Bonizzoni, M, Gasperi, G, Chen, X, and James, AA. The invasive mosquito species Aedes albopictus: current knowledge and future perspectives. Trends Parasitol. (2013) 29:460–8. doi: 10.1016/j.pt.2013.07.003
120. Tatem, AJ, Hay, SI, and Rogers, DJ. Global traffic and disease vector dispersal. Proc Natl Acad Sci USA. (2006) 103:6242–7. doi: 10.1073/pnas.0508391103
121. Ferreira-de-Lima, VH, Câmara, DCP, Honório, NA, and Lima-Camara, TN. The Asian tiger mosquito in Brazil: observations on biology and ecological interactions since its first detection in 1986. Acta Trop. (2020) 205:105386. doi: 10.1016/j.actatropica.2020.105386
122. Consoli, R, and Lourenço-de-Oliveira, R. Principais mosquitos de importância sanitária do Brasil. Rio de Janeiro: Fiocruz (1994).
123. Lounibos, LP. Invasions by insect vectors of human disease. Annu Rev Entomol. (2002) 47:233–66. doi: 10.1146/annurev.ento.47.091201.145206
124. Database GIS. Species profile: Aedes albopictus. (2009). Available at: http://www.iucngisd.org/gisd/search.php. (Accessed December 2, 2024).
125. Braks, MA, Honório, NA, Lourençqo-De-Oliveira, R, Juliano, SA, and Lounibos, LP. Convergent habitat segregation of Aedes aegypti and Aedes albopictus (Diptera: Culicidae) in southeastern Brazil and Florida. J Med Entomol. (2003) 40:785–94. doi: 10.1603/0022-2585-40.6.785
126. Honório, NA, Codeço, CT, Alves, FC, Magalhães, MA, and Lourenço-De-Oliveira, R. Temporal distribution of Aedes aegypti in different districts of Rio de Janeiro, Brazil, measured by two types of traps. J Med Entomol. (2009) 46:1001–14. doi: 10.1603/033.046.0505
127. Câmara, DCP, Pinel, CDS, Rocha, GP, Codeço, CT, and Honório, NA. Diversity of mosquito (Diptera: Culicidae) vectors in a heterogeneous landscape endemic for arboviruses. Acta Trop. (2020) 212:105715. doi: 10.1016/j.actatropica.2020.105715
128. Câmara, DCP, Codeço, CT, Ayllón, T, Nobre, AA, Azevedo, RC, Ferreira, DF, et al. Entomological surveillance of Aedes mosquitoes: comparison of different collection methods in an endemic area in Rio de Janeiro, Brazil. Trop Med Infect Dis. (2022) 7:114. doi: 10.3390/tropicalmed7070114
129. Niebylski, ML, and Craig, GB Jr. Dispersal and survival of Aedes albopictus at a scrap tire yard in Missouri. J Am Mosq Control Assoc. (1994) 10:339–43.
130. Pereira Dos Santos, T, Roiz, D, Santos de Abreu, FV, Luz, SLB, Santalucia, M, Jiolle, D, et al. Potential of Aedes albopictus as a bridge vector for enzootic pathogens at the urban-forest interface in Brazil. Emerg Microbes Infect. (2018) 7:1–8. doi: 10.1038/s41426-018-0194-y
131. Gomes, AC, Silva, NN, Marques, GR, and Brito, M. Host-feeding patterns of potential human disease vectors in the Paraíba Valley region, state of Säo Paulo, Brazil. J Vector Ecol. (2003) 28:74–8.
132. Victor, TJ, Malathi, M, Gurusamy, D, Desai, A, Ravi, V, Narayanasamy, G, et al. Dengue fever outbreaks in two villages of Dharmapuri district in Tamil Nadu. Indian J Med Res. (2002) 116:133–9.
133. Benedict, M, Levine, R, Hawley, W, and Lounibos, L. Spread of the tiger: global risk of invasion by the mosquito Aedes albopictus. Vector Borne Zoonotic Dis. (2007) 7:76–85. doi: 10.1089/vbz.2006.0562
134. Stenn, T, Peck, KJ, Rocha Pereira, G, and Burkett-Cadena, ND. Vertebrate hosts of Aedes aegypti, Aedes albopictus, and Culex quinquefasciatus (Diptera: Culicidae) as potential vectors of Zika virus in Florida. J Med Entomol. (2019) 56:10–7. doi: 10.1093/jme/tjy148
135. Medlock, JM, Avenell, D, Barrass, I, and Leach, S. Analysis of the potential for survival and seasonal activity of Aedes albopictus (Diptera: Culicidae) in the United Kingdom. J Vector Ecol. (2006) 31:292–304. doi: 10.3376/1081-1710(2006)31[292:AOTPFS]2.0.CO;2
136. Mascarenhas, M, Garasia, S, Berthiaume, P, Corrin, T, Greig, J, Ng, V, et al. A scoping review of published literature on chikungunya virus. PLoS One. (2018) 13:e0207554. doi: 10.1371/journal.pone.0207554
137. Forattini, OP. Identification of Aedes (Stegomyia) albopictus (Skuse) in Brazil. Rev Saude Publica. (1986) 20:244–5. doi: 10.1590/S0034-89101986000300009
138. Glasser, CM, and AeC, GA. Climate and the superimposed distribution of Aedes aegypti and Aedes albopictus on infestation of São Paulo state, Brazil. Rev Saude Publica. (2002) 36:166–72. doi: 10.1590/S0034-89102002000200008
139. Sant’Ana, AL, editor First recorded occurrence of Aedes (Stegomyia) albopictus (Skuse) in the south-eastern region of Brazil. Rev Saude Publica. (1996). 30:392–3. doi: 10.1590/s0034-89101996000400013
140. Fé, NF, Barbosa, MGV, Alecrim, WD, and Guerra, MVF. Registration of the occurrence of Aedes albopictus in an urban zone in Manaus, Amazonas, Brazil. Rev Saude Publica. (2003) 37:674–5. doi: 10.1590/S0034-89102003000500020
141. dos Santos, SO, and do Nascimento, JC. First register of Aedes (Stegomyia) albopictus (Skuse) presence in Mato Grosso do Sul, Brazil. Rev Saude Publica. (1998) 32:486. doi: 10.1590/S0034-89101998000500013
142. de Albuquerque, CM, Melo-Santos, MA, Bezerra, MA, Barbosa, RM, Silva, DF, and da Silva, E. First report of Aedes albopictus in areas of Mata Atlantica, Recife, PE, Brazil. Rev Saude Publica. (2000) 34:314–5. doi: 10.1590/S0034-89102000000300017
143. Gomes, AC, Bitencourt, MD, Natal, D, Pinto, PL, Mucci, LF, de Paula, MB, et al. Aedes albopictus in rural zone of Brazil and its implication in the wild yellow fever transmission. Rev Saude Publica. (1999) 33:95–7.
144. Corte, L, and dos Santos, R. Updating of the distribution of Aedes albopictus in Brazil (1997-2002). Rev Saude Publica. (2003) 37:671–3. doi: 10.1590/S0034-89102003000500019
145. Rocha, RDC, Cardoso, ADS, Souza, JL, Pereira, EDS, Amorim, MF, Souza, MSM, et al. First official record of Aedes (Stegomyia) albopictus (Diptera: Culicidae) in the Acre state, northern Brazil. Rev Inst Med Trop Sao Paulo. (2023) 65:e20. doi: 10.1590/s1678-9946202365020
146. Alto, BW, Wiggins, K, Eastmond, B, Velez, D, Lounibos, LP, and Lord, CC. Transmission risk of two chikungunya lineages by invasive mosquito vectors from Florida and the Dominican Republic. PLoS Negl Trop Dis. (2017) 11:e0005724. doi: 10.1371/journal.pntd.0005724
147. Macdonald, G. Epidemiologic models in studies of vector-borne diseases. Public Health Rep. (1961) 76:753–64. doi: 10.2307/4591271
148. Dye, C, and Hasibeder, G. Population dynamics of mosquito-borne disease: effects of flies which bite some people more frequently than others. Trans R Soc Trop Med Hyg. (1986) 80:69–77. doi: 10.1016/0035-9203(86)90199-9
149. Smith, DL, Battle, KE, Hay, SI, Barker, CM, Scott, TW, and McKenzie, FE. Ross, Macdonald, and a theory for the dynamics and control of mosquito-transmitted pathogens. PLoS Pathog. (2012) 8:e1002588. doi: 10.1371/journal.ppat.1002588
150. Garrett-Jones, C. Prognosis for interruption of malaria transmission through assessment of the mosquito's vectorial capacity. Nature. (1964) 204:1173–5. doi: 10.1038/2041173a0
151. Clements, AN. The biology of mosquitoes, vol. 3. Viral and bacterial pathogens and bacterial symbionts: CABI (2012).
152. Honório, NA, Wiggins, K, Câmara, DCP, Eastmond, B, and Alto, BW. Chikungunya virus vector competency of Brazilian and Florida mosquito vectors. PLoS Negl Trop Dis. (2018) 12:e0006521. doi: 10.1371/journal.pntd.0006521
153. Black, WC, Bennett, KE, Gorrochótegui-Escalante, N, Barillas-Mury, CV, Fernández-Salas, I, de Lourdes, MM, et al. Flavivirus susceptibility in Aedes aegypti. Arch Med Res. (2002) 33:379–88. doi: 10.1016/S0188-4409(02)00373-9
154. Tabachnick, WJ. Nature, nurture and evolution of intra-species variation in mosquito arbovirus transmission competence. Int J Environ Res Public Health. (2013) 10:249–77. doi: 10.3390/ijerph10010249
155. Lambrechts, L, and Failloux, AB. Vector biology prospects in dengue research. Mem Inst Oswaldo Cruz. (2012) 107:1080–2. doi: 10.1590/S0074-02762012000800022
156. Mangiafico, JA. Chikungunya virus infection and transmission in five species of mosquito. Am J Trop Med Hyg. (1971) 20:642–5.
157. Tesh, RB, Gubler, DJ, and Rosen, L. Variation among geographic strains of Aedes albopictus in susceptibility to infection with chikungunya virus. Am J Trop Med Hyg. (1976) 25:326–35. doi: 10.4269/ajtmh.1976.25.326
158. Banerjee, K, Mourja, DT, and Malunjkar, AS. Susceptibility and transmissibility of different geographical strains of Aedes aegypti mosquitoes to chikungunya virus. Indian J Med Res. (1988) 87:134–8.
159. Mourya, DT, Malunjkar, AS, and Banerjee, K. Susceptibility and transmissibility of Aedes aegypti to four strains of chikungunya virus. Indian J Med Res. (1987) 86:185–90.
160. Turell, MJ, Beaman, JR, and Tammariello, RF. Susceptibility of selected strains of Aedes aegypti and Aedes albopictus (Diptera: Culicidae) to chikungunya virus. J Med Entomol. (1992) 29:49–53. doi: 10.1093/jmedent/29.1.49
161. Weaver, SC, Charlier, C, Vasilakis, N, and Lecuit, M. Zika, chikungunya, and other emerging vector-borne viral diseases. Annu Rev Med. (2018) 69:395–408. doi: 10.1146/annurev-med-050715-105122
162. Vega-Rúa, A, Marconcini, M, Madec, Y, Manni, M, Carraretto, D, Gomulski, LM, et al. Vector competence of Aedes albopictus populations for chikungunya virus is shaped by their demographic history. Commun Biol. (2020) 3:326. doi: 10.1038/s42003-020-1046-6
163. Tsetsarkin, KA, and Weaver, SC. Sequential adaptive mutations enhance efficient vector switching by chikungunya virus and its epidemic emergence. PLoS Pathog. (2011) 7:e1002412. doi: 10.1371/journal.ppat.1002412
164. Cunha, MS, Cruz, NVG, Schnellrath, LC, Medaglia, MLG, Casotto, ME, Albano, RM, et al. Autochthonous transmission of east/central/south African genotype chikungunya virus, Brazil. Emerg Infect Dis. (2017) 23:1737–9. doi: 10.3201/eid2310.161855
165. de Oliveira Ribeiro, G, Gill, DE, do Socorro Foro Ramos, E, Villanova, F, Soares D'Athaide Ribeiro, E, Monteiro, FJC, et al. Chikungunya virus Asian lineage infection in the Amazon region is maintained by asiatic and caribbean-introduced variants. Viruses. (2022) 14:1445. doi: 10.3390/v14071445
166. Sanchez-Vargas, I, Harrington, LC, Black, WC, and Olson, KE. Analysis of salivary glands and saliva from Aedes albopictus and Aedes aegypti infected with chikungunya viruses. Insects. (2019) 10:39. doi: 10.3390/insects10020039
167. Ribeiro Cruz, AC, Pinto Nunes Neto, J, Patroca da Silva, S, Pinto, V, da Silva, E, Juscely Galvão Pereira, G, et al. Chikungunya virus detection in Aedes aegypti and Culex quinquefasciatus during an outbreak in the Amazon region. Viruses. (2020) 12:853. doi: 10.3390/v12080853
168. Garcia-Rejon, JE, Navarro, J-C, Cigarroa-Toledo, N, and Baak-Baak, CM. An updated review of the invasive Aedes albopictus in the Americas; geographical distribution, host feeding patterns, arbovirus infection, and the potential for vertical transmission of dengue virus. Insects. (2021) 12:967. doi: 10.3390/insects12110967
169. Ricas Rezende, H, Malta Romano, C, Morales Claro, I, Santos Caleiro, G, Cerdeira Sabino, E, Felix, AC, et al. First report of Aedes albopictus infected by dengue and Zika virus in a rural outbreak in Brazil. PLoS One. (2020) 15:e0229847. doi: 10.1371/journal.pone.0229847
170. Variza, PF, Lorenz, C, de Oliveira, JG, Fernandes, M, Netto, SA, and Prophiro, JS. Updated spatio-temporal distribution of Aedes (Stegomyia) albopictus in Brazil. Acta Trop. (2022) 232:106511. doi: 10.1016/j.actatropica.2022.106511
171. Zeller, H, Van Bortel, W, and Sudre, B. Chikungunya: its history in Africa and Asia and its spread to new regions in 2013-2014. J Infect Dis. (2016) 214:S436–40. doi: 10.1093/infdis/jiw391
172. Morrison, TE. Reemergence of chikungunya virus. J Virol. (2014) 88:11644–7. doi: 10.1128/JVI.01432-14
173. Tsetsarkin, KA, Chen, R, Yun, R, Rossi, SL, Plante, KS, Guerbois, M, et al. Multi-peaked adaptive landscape for chikungunya virus evolution predicts continued fitness optimization in Aedes albopictus mosquitoes. Nat Commun. (2014) 5:4084. doi: 10.1038/ncomms5084
174. Vignuzzi, M, and Higgs, S. The bridges and blockades to evolutionary convergence on the road to predicting chikungunya virus evolution. Annu Rev Virol. (2017) 4:181–200. doi: 10.1146/annurev-virology-101416-041757
175. Tsetsarkin, KA, McGee, CE, Volk, SM, Vanlandingham, DL, Weaver, SC, and Higgs, S. Epistatic roles of E2 glycoprotein mutations in adaption of chikungunya virus to Aedes albopictus and Ae. aegypti mosquitoes. PLoS One. (2009) 4:e6835. doi: 10.1371/journal.pone.0006835
176. Tsetsarkin, KA, Chen, R, Leal, G, Forrester, N, Higgs, S, Huang, J, et al. Chikungunya virus emergence is constrained in Asia by lineage-specific adaptive landscapes. Proc Natl Acad Sci USA. (2011) 108:7872–7. doi: 10.1073/pnas.1018344108
177. Tsetsarkin, KA, Chen, R, and Weaver, SC. Interspecies transmission and chikungunya virus emergence. Curr Opin Virol. (2016) 16:143–50. doi: 10.1016/j.coviro.2016.02.007
178. Agarwal, A, Sharma, AK, Sukumaran, D, Parida, M, and Dash, PK. Two novel epistatic mutations (E1:K211E and E2:V264A) in structural proteins of chikungunya virus enhance fitness in Aedes aegypti. Virology. (2016) 497:59–68. doi: 10.1016/j.virol.2016.06.025
179. Filomatori, CV, Bardossy, ES, Merwaiss, F, Suzuki, Y, Henrion, A, Saleh, MC, et al. RNA recombination at chikungunya virus 3'UTR as an evolutionary mechanism that provides adaptability. PLoS Pathog. (2019) 15:e1007706. doi: 10.1371/journal.ppat.1007706
180. Stapleford, KA, Moratorio, G, Henningsson, R, Chen, R, Matheus, S, Enfissi, A, et al. Whole-genome sequencing analysis from the chikungunya virus caribbean outbreak reveals novel evolutionary genomic elements. PLoS Negl Trop Dis. (2016) 10:e0004402. doi: 10.1371/journal.pntd.0004402
181. Merwaiss, F, Filomatori, CV, Susuki, Y, Bardossy, ES, Alvarez, DE, and Saleh, MC. Chikungunya virus replication rate determines the capacity of crossing tissue barriers in mosquitoes. J Virol. (2021) 95:e01956-20. doi: 10.1128/JVI.01956-20
182. de Lamballerie, X, Leroy, E, Charrel, RN, Ttsetsarkin, K, Higgs, S, and Gould, EA. Chikungunya virus adapts to tiger mosquito via evolutionary convergence: a sign of things to come? Virol J. (2008) 5:33. doi: 10.1186/1743-422X-5-33
183. Dubrulle, M, Mousson, L, Moutailler, S, Vazeille, M, and Failloux, AB. Chikungunya virus and Aedes mosquitoes: saliva is infectious as soon as two days after oral infection. PLoS One. (2009) 4:e5895. doi: 10.1371/journal.pone.0005895
184. Severini, F, Boccolini, D, Fortuna, C, Di Luca, M, Toma, L, Amendola, A, et al. Vector competence of Italian Aedes albopictus populations for the chikungunya virus (E1-226V). PLoS Negl Trop Dis. (2018) 12:e0006435. doi: 10.1371/journal.pntd.0006435
185. Rangel, MV, McAllister, N, Dancel-Manning, K, Noval, MG, Silva, LA, and Stapleford, KA. Emerging chikungunya virus variants at the E1-E1 interglycoprotein spike interface impact virus attachment and inflammation. J Virol. (2022) 96:e0158621. doi: 10.1128/jvi.01586-21
186. Niebylski, ML, Savage, HM, Nasci, RS, and Craig, GB Jr. Blood hosts of Aedes albopictus in the United States. J Am Mosq Control Assoc. (1994) 10:447–50.
187. Scholte, E-J. Waiting for the tiger: Establishment and spread of the Aedes albopictus mosquito in Europe In: Emerging pests and vector-borne diseases in Europe. Wageningen: Wageningen Academic (2007). 241–60.
188. Richards, SL, Ponnusamy, L, Unnasch, TR, Hassan, HK, and Apperson, CS. Host-feeding patterns of Aedes albopictus (Diptera: Culicidae) in relation to availability of human and domestic animals in suburban landscapes of Central North Carolina. J Med Entomol. (2006) 43:543–51. doi: 10.1093/jmedent/43.3.543
189. Faraji, A, Egizi, A, Fonseca, DM, Unlu, I, Crepeau, T, Healy, SP, et al. Comparative host feeding patterns of the Asian tiger mosquito, Aedes albopictus, in urban and suburban northeastern USA and implications for disease transmission. PLoS Negl Trop Dis. (2014) 8:e3037. doi: 10.1371/journal.pntd.0003037
190. Pereira-dos-Santos, T, Roiz, D, Lourenço-de-Oliveira, R, and Paupy, C. A systematic review: is Aedes albopictus an efficient bridge vector for zoonotic arboviruses? Pathogens. (2020) 9:266. doi: 10.3390/pathogens9040266
191. Moore, CG, and Fisher, BR. Competition in mosquitoes. Density and species ratio effects on growth, mortality, fecundity, and production of growth retardant. Ann Entomol Soc Am. (1969) 62:1325–31. doi: 10.1093/aesa/62.6.1325
192. Barrera, R. Competition and resistance to starvation in larvae of container-inhabiting Aedes mosquitoes. Ecol Entomol. (1996) 21:117–27. doi: 10.1111/j.1365-2311.1996.tb01178.x
193. Juliano, SA, Lounibos, LP, and O’Meara, GF. A field test for competitive effects of Aedes albopictus on A. aegypti in South Florida: differences between sites of coexistence and exclusion? Oecologia. (2004) 139:583–93. doi: 10.1007/s00442-004-1532-4
194. Braks, M, Honório, N, Lounibos, L, Lourenço-de-Oliveira, R, and Juliano, S. Interspecific competition between two invasive species of container mosquitoes, Aedes aegypti and Aedes albopictus (Diptera: Culicidae), in Brazil. Ann Entomol Soc Am. (2004) 97:130–9. doi: 10.1603/0013-8746(2004)097[0130:ICBTIS]2.0.CO;2
195. Camara, DC, Codeço, CT, Juliano, SA, Lounibos, LP, Riback, TI, Pereira, GR, et al. Seasonal differences in density but similar competitive impact of Aedes albopictus (Skuse) on Aedes aegypti (L.) in Rio de Janeiro, Brazil. PLoS One. (2016) 11:e0157120. doi: 10.1371/journal.pone.0157120
196. Zouache, K, Fontaine, A, Vega-Rua, A, Mousson, L, Thiberge, JM, Lourenco-De-Oliveira, R, et al. Three-way interactions between mosquito population, viral strain and temperature underlying chikungunya virus transmission potential. Proc Biol Sci. (2014) 281:20141078. doi: 10.1098/rspb.2014.1078
197. Wimalasiri-Yapa, BR, Stassen, L, Hu, W, Yakob, L, McGraw, EA, Pyke, AT, et al. Chikungunya virus transmission at low temperature by Aedes albopictus mosquitoes. Pathogens. (2019) 8:149. doi: 10.3390/pathogens8030149
198. Heitmann, A, Jansen, S, Lühken, R, Helms, M, Pluskota, B, Becker, N, et al. Experimental risk assessment for chikungunya virus transmission based on vector competence, distribution and temperature suitability in Europe, 2018. Euro Surveill. (2018) 23:1800033. doi: 10.2807/1560-7917.ES.2018.23.29.1800033
199. Honório, NA, Silva, WC, Leite, PJ, Gonçalves, JM, Lounibos, LP, and Lourenço-de-Oliveira, R. Dispersal of Aedes aegypti and Aedes albopictus (Diptera: Culicidae) in an urban endemic dengue area in the state of Rio de Janeiro, Brazil. Mem Inst Oswaldo Cruz. (2003) 98:191–8. doi: 10.1590/S0074-02762003000200005
200. Maciel-de-Freitas, R, Neto, RB, Gonçalves, JM, Codeço, CT, and Lourenço-de-Oliveira, R. Movement of dengue vectors between the human modified environment and an urban forest in Rio de Janeiro. J Med Entomol. (2006) 43:1112–20. doi: 10.1093/jmedent/43.6.1112
201. Eritja, R, Palmer, JR, Roiz, D, Sanpera-Calbet, I, and Bartumeus, F. Direct evidence of adult Aedes albopictus dispersal by car. Sci Rep. (2017) 7:14399. doi: 10.1038/s41598-017-12652-5
202. Ayllón, T, Câmara, DCP, Morone, FC, Gonçalves, LS, Monteiro, S, de Barros, F, et al. Dispersion and oviposition of Aedes albopictus in a Brazilian slum: initial evidence of Asian tiger mosquito domiciliation in urban environments. PLoS One. (2018) 13:e0195014. doi: 10.1371/journal.pone.0195014
203. Vavassori, L, Saddler, A, and Müller, P. Active dispersal of Aedes albopictus: a mark-release-recapture study using self-marking units. Parasit Vectors. (2019) 12:1–14. doi: 10.1186/s13071-019-3837-5
204. Lima-Camara, TN, Codeço, CT, Honório, NA, Bruno, RV, Peixoto, AA, and Lounibos, LP. Male accessory gland substances from Aedes albopictus affect the locomotor activity of Aedes aegypti females. Mem Inst Oswaldo Cruz. (2013) 108:18–25. doi: 10.1590/0074-0276130381
205. Honório, NA, Carrasquilla, MC, Bargielowski, IE, Nishimura, N, Swan, T, and Lounibos, LP. Male origin determines satyrization potential of Aedes aegypti by invasive Aedes albopictus. Biol Invasions. (2018) 20:653–64. doi: 10.1007/s10530-017-1565-3
206. Feitoza, TS, Ferreira-de-Lima, VH, Câmara, DCP, Honório, NA, Lounibos, LP, and Lima-Camara, TN. Interspecific mating effects on locomotor activity rhythms and refractoriness of Aedes albopictus (Diptera: Culicidae) females. Insects. (2020) 11:874. doi: 10.3390/insects11120874
207. Serufo, JC, de Oca, HM, Tavares, VA, Souza, AM, Rosa, RV, Jamal, MC, et al. Isolation of dengue virus type 1 from larvae of Aedes albopictus in Campos Altos city, state of Minas Gerais, Brazil. Mem Inst Oswaldo Cruz. (1993) 88:503–4. doi: 10.1590/S0074-02761993000300025
208. Ferreira-de-Lima, VH, and Lima-Camara, TN. Natural vertical transmission of dengue virus in Aedes aegypti and Aedes albopictus: a systematic review. Parasit Vectors. (2018) 11:77. doi: 10.1186/s13071-018-2643-9
209. Maia, LMS, Bezerra, MCF, Costa, MCS, Souza, EM, Oliveira, MEB, Ribeiro, ALM, et al. Natural vertical infection by dengue virus serotype 4, Zika virus and Mayaro virus in Aedes (Stegomyia) aegypti and Aedes (Stegomyia) albopictus. Med Vet Entomol. (2019) 33:437–42. doi: 10.1111/mve.12369
Keywords: chikungunya, virus diversity and genotypes, Aedes albopictus , vector competence, environmental/ecological determinants
Citation: Resck MEB, Câmara DCP, dos Santos FB, dos Santos JPC, Alto BW and Honório NA (2024) Spatial–temporal distribution of chikungunya virus in Brazil: a review on the circulating viral genotypes and Aedes (Stegomyia) albopictus as a potential vector. Front. Public Health. 12:1496021. doi: 10.3389/fpubh.2024.1496021
Edited by:
Benjamin Cull, University of Minnesota Twin Cities, United StatesReviewed by:
Camila Malta Romano, University of São Paulo, BrazilSandra V. Mayer, Walter Reed Army Institute of Research, United States
Henry Puerta-Guardo, Universidad Autónoma de Yucatán, Mexico
Copyright © 2024 Resck, Câmara, dos Santos, dos Santos, Alto and Honório. This is an open-access article distributed under the terms of the Creative Commons Attribution License (CC BY). The use, distribution or reproduction in other forums is permitted, provided the original author(s) and the copyright owner(s) are credited and that the original publication in this journal is cited, in accordance with accepted academic practice. No use, distribution or reproduction is permitted which does not comply with these terms.
*Correspondence: Maria Eduarda Barreto Resck, ZHVkYXJlc2NrQGhvdG1haWwuY29t; Nildimar Alves Honório, bmlsZGltYXIuaG9ub3Jpb0Bpb2MuZmlvY3J1ei5icg==; Barry Wilmer Alto, YndhbHRvQHVmbC5lZHU=
†These authors have contributed equally to this work and share last authorship