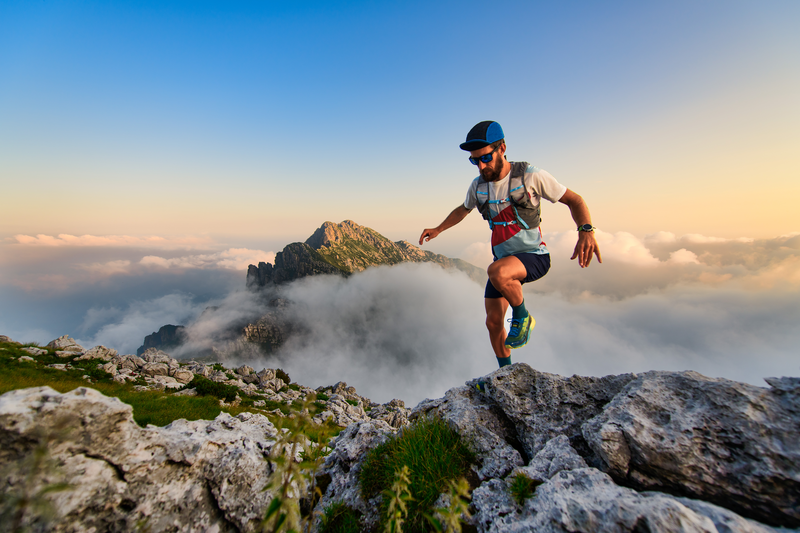
94% of researchers rate our articles as excellent or good
Learn more about the work of our research integrity team to safeguard the quality of each article we publish.
Find out more
ORIGINAL RESEARCH article
Front. Public Health , 08 November 2024
Sec. Public Health and Nutrition
Volume 12 - 2024 | https://doi.org/10.3389/fpubh.2024.1494511
This article is part of the Research Topic Transforming Food Systems: Addressing Malnutrition and Inequality in Low- and Middle-Income Countries View all 12 articles
Objective: To describe antibiotic exposure in children and explore its association with overweight/obesity.
Methods: In June 2022, 328 kindergarten and primary school children were selected from Nantong city in Jiangsu Province. Questionnaires were distributed, and morning urine samples were obtained. Total urinary concentrations of 41 antibiotics were measured using ultra-performance liquid chromatography and tandem mass spectrometry. The rates of antibiotic exposure were expressed as percentages (%), specific percentiles (P95 and P99), and the maximum values were used to describe the concentration of antibiotics. The association between urinary antibiotic creatinine-adjusted and overweight/obesity was analyzed using logistic regression.
Results: A total of 328 children were initially recruited, of which 295 aged 3–8 years met the inclusion criteria and were finally included in the study. The biomonitoring results revealed that 35 antibiotics were detected, with a total detection frequency of 98.31%. Among the included children, 24.75% were classified as overweight/obesity. Multinomial logistic regression analyses revealed significant associations between overweight/obese and exposure to veterinary antibiotics (VAs) and preferred veterinary antibiotics (PVAs). After adjusting for various overweight/obesity-relevant variables, higher exposure to sulfamethoxazole [OR = 2.35, 95% confidence interval (CI):1.17–4.70], norfloxacin (OR = 2.66, 95% CI: 1.01–7.08), and fluoroquinolones (OR = 1.97, 95% CI: 1.02–3.78) were significantly associated with overweight/obesity (p < 0.05). In addition, after stratification by sex and adjustment for confounding variables, sex-specific differences were observed in the association between antibiotic exposure and overweight/obesity. Notably, these associations were predominantly observed among boys.
Conclusion: Children were extensively exposed to antibiotics. Exposure to certain types of veterinary antibiotics and preferred veterinary antibiotic exposure, mainly through food or drinking water, are associated with an increased risk of overweight/obesity in children.
The incidence of childhood obesity is increasing annually and has become one of the most challenging global public health issues (1). Obesity in childhood not only seriously affects growth and development but also increases the risk of chronic obesity-related diseases in adulthood (2). The risk factors that lead to childhood obesity are extremely complex and include unhealthy dietary habits, lifestyle choices, and genetic factors. However, its pathogenesis remains unclear. Studies have shown that antibiotic exposure in early life may lead to intestinal microbiota imbalance and increase the risk of overweight/obesity in children (3, 4). Consequently, the relationship between antibiotic-altered microbiota and adiposity-related metabolic changes may contribute to the obesity in children.
In recent years, antibiotics have been increasingly used as growth promoters and disease control agents in animal husbandry and aquaculture (5, 6). Owing to the overuse of these antibiotics, an increasing number of antibiotic residues have been detected in soil, water, and animal-derived foods (chicken, beef, and milk) (7, 8). Humans can be exposed to antibiotics in two ways: through clinical or self-medication, leading to short-term high-dose exposure to human antibiotics, and through ingestion of contaminated food or drinking water, leading to long-term low-dose exposure to veterinary antibiotics (9, 10). Detecting the levels of antibiotics and their metabolites in urine can effectively reflect the level of human antibiotic exposure and provide a convenient and reliable method for assessing the body burden of antibiotics in the population (11). Previous research has shown that various antibiotics, especially veterinary antibiotics, are present in children’s urine (12, 13). This indicates that children are exposed to antibiotics extensively and for long durations, and compared to adults, children are more susceptible to the effects of antibiotics.
Epidemiological evidence suggests that antibiotic exposure is associated with childhood obesity (14–16). However, several studies have focused on school-age children, with fewer studies on preschoolers. In addition, these studies did not examine the relationship between antibiotic exposure from the daily diet or drinking water and childhood obesity. Therefore, this study aimed to monitor antibiotic exposure in children in Jiangsu, China and further evaluate the association between foodborne antibiotic exposure and childhood overweight/obesity.
Between June and July 2022, two economically and geographically distinct areas in Nantong City were selected as study areas: Chongchuan (municipal district) and Rugao (prefecture-level city). Using a stratified sampling method, we randomly selected one kindergarten and one primary school from each area, followed by a random selection of small classes from each kindergarten and two classes in the first grade from each primary school. At least 75 children were selected from each of the four schools, resulting in 328 children. The inclusion criteria were as follows: (1) residing in the area for >6 months, (2) not in an acute state of morbidity, and (3) no antibiotic use in the past 30 days. Ultimately, we included 295 children aged 3–8 years (161 boys and 134 girls) who met the inclusion criteria. The study was approved by the Institutional Ethics Committee of the Nantong Center for Disease Control and Prevention, and consent forms were signed by the parents or guardians of the enrolled children.
Potential confounding variables were assessed using a self-designed questionnaire completed by the guardians or parents of the children. Basic information collected included age, sex, school stage, location, duration of physical activity, and annual family income. The frequency of food consumption over the past 3 months was investigated using the Food Frequency Questionnaire (FFQ), which included vegetables, fruits, livestock meat products, poultry meat products, animal viscera, freshwater products, seafood, milk and dairy products, puffed food, and honey.
Morning urine samples were collected from the children using 30 mL polypropylene centrifuge tube. These samples were transported to the laboratory in an incubator containing ice packs and were stored at −80°C in the dark until analysis.
Body weight was measured to the nearest 0.1 kg and height to the nearest 0.1 cm using calibrated instruments and standard procedures by trained technicians. Body mass index (BMI) was calculated as weight in kilograms divided by height in square meters (kg/m2). Participants were classified as overweight/obese according to the criteria for children aged 2–18 in China as proposed by the Working Group on Obesity in China (WGOC) (17).
Forty-one representative antibiotics were selected and classified into eight categories based on their mechanism of action: nine Sulfonamides, six Macrolides, eight β-Lactams, four Tetracyclines, nine fluoroquinolones, one Quinoxalines, one Lincosamides and three Chloramphenicols. These antibiotics were further grouped into four categories according to their usage: six human antibiotics (HAs), 14 veterinary antibiotics (VAs), five preferred human antibiotics (PHAs), and 16 preferred veterinary antibiotics (PVAs). Supplementary Table S1 shows the usage, chemical formula, molecular weight, and CAS number for each antibiotic.
Urinary antibiotic levels were measured using ultra-performance liquid chromatography and tandem mass spectrometry (18). The detection limit (3S/N) of the method obtained using the LC-30A ultra-high-performance liquid chromatograph and the AB SCIEXQTRAP 4500 tandem mass spectrometer in the positive and negative ion modes was 0.01–1.25 μg·L−1. The recoveries of all analytes ranged from 61.3 to 128%, with the relative standard deviations (RDSs) (n = 5) of 1.2–14%.
To process the sample, 1.00 mL of urine was pipetted and mixed with 10 μL of mixed internal standard intermediate solution (1.0 μg/mL), followed by the addition of 0.30 mL of Na2 EDTA-Mcllvaine buffer (pH = 3.5). The mixture was vortexed for 30 s, and oscillated at 14,000 r/min for 5 min, and the supernatant was passed through the column. Methanol (3 mL) was used to wash the column, and the supernatant was collected after centrifugation and passed through the column again. The Oasis HLB solid-phase extraction column was sequentially activated with 3 mL of methanol, water (3 mL), and 3 mL of Na2 EDTA-Mcllvaine buffer. The supernatant was transferred throught the activated solid-phase extraction column at a flow rate of 1.0 mL/min. After evacuation using a vacuum pump, it was eluted with 3 mL of methanol at a rate of 1.0 mL/min. The eluate was collected, evaporated to near dryness under nitrogen, reconstituted to 0.5 mL with 10% methanol aqueous solution, vortexed to mix, passed through a 0.22 μm organic filter, and analyzed. For chromatographic analysis, a Kinetex® F5 100A chromatographic column (50 × 3.0 mm, 2.6 μm) was used. The mobile phase consisted of 0.1% formic acid in water and 0.1% formic acid in acetonitrile, using gradient elution. Electrospray ionization was used with simultaneous scanning of positive and negative ions, and multiple reaction monitoring mode was employed for analysis. To correct the effect of urine dilution, we used the urine creatinine concentration to adjust the volume mass fraction of antibiotics. The corrected antibiotic creatinine concentration (μg/g creatinine) was calculated as the antibiotic concentration (ng/mL)/[urine creatinine concentration (mol/L) × 113.12 (g/mol)]. Urinary creatinine concentrations were measured using an automated biochemical analyzer [COBAS-8900, Roche Diagnostics (Shanghai) Co., Ltd., Shanghai, China].
The antibiotic concentrations below the limit of detection (LODs) were replaced with LOD s/2 to calculate the total detected concentration. The rates of antibiotic exposure were expressed as percentages (%), specific percentiles (P95 and P99), and the maximum values were used to describe the concentration of antibiotics. Children’s ages being skewed were represented by M (P25, P75). Comparison of rates between the two groups was performed using Pearson’s chi-square test. According to LODs and urinary creatinine-corrected concentrations of antibiotics, antibiotics are divided into three groups: <LODs (negative detection group), <median (low concentration group), ≥median (high concentration group). A total of 16 individual antibiotics were detected and > 10% of the samples were selected for further analysis. The association between urinary antibiotic creatinine-adjusted concentrations and overweight/obesity was analyzed using logistic regression. After stratification by sex, the association between different antibiotic exposure levels and overweight/obesity in children of different sexes was analyzed. All statistical analyses were conducted using SPSS software (version 21.0, IBM). A two-sided p < 0.05 was considered statistically significant.
Among the 295 children aged 3–8 years, 73 were overweight/obese (39 were overweight and 34 were obese), resulting in a rate of overweight/obesity of 24.75%. The median age was 6.50 years (interquartile range: 4.00, 7.50). No statistically significant differences were observed in sex, school stage, location, physical activity time, annual family income, and consumption of vegetables, fruits, and livestock products between the normal-weight and overweight/obese groups. However, children with overweight/obesity showed a higher consumption of puffed food compared to children with normal weight (p < 0.05), while the consumption of meat products, poultry meat products, animal viscera, freshwater products, seafood, milk and dairy products, and honey were comparable between the two groups (Table 1).
All 35 antibiotics were detected in urine, with a total detection frequency of 98.31%. Regarding antibiotic categories, sulfonamides exhibited the highest detection rates (81.69%), followed by fluoroquinolones (64.07%), macrolides (48.47%), β-lactams (43.39%), tetracyclines (25.42%), lincosamides (8.81%), chloramphenicols (3.73%), quinoxalines (0.00%). The detection rates of individual antibiotics ranged from 0.00 to 49.49%. Among them, 16 antibiotics, including sulfameter (49.49%), sulfaclozine (29.49%), sulfamerazine (38.98%), sulfamonomethoxine (28.81%), erythromycin (38.31%), azithromycin (21.02%), cefquinome (15.25%), amoxicillin (23.73%), oxytetracycline (16.27%), pefloxacin (18.98%), lomefloxacin (16.61%), danofloxacine (13.22%), sarafloxacin (15.25%), ofloxacin (12.88%), ciprofloxacin (10.17%), norfloxacin (12.20%), were detected in more than 10% of the participants. HAs, VAs, PHAs, and PVAs were detected in 26.10, 72.20, 55.25, and 90.17% of the urine samples, respectively. Moreover, two or more antibiotics were simultaneously detected in 93.90% of urine samples. Urinary concentrations ranged from <0 ng/mL to 14.9 μg/mL. The urinary concentrations of sulfaclozine and norfloxacin in children who were overweight/obese were significantly higher than those in normal-weight group (p < 0.05). Conversely, the urinary concentrations of sulfamerazine in children who were overweight/obese were significantly lower than those in normal-weight group (p < 0.05) (Table 2).
Table 2. Detection frequency and urinary concentration (ng/mL) distribution of included antibiotics in normal weight and overweight/obesity.
A total of 16 individual antibiotics detected in >10% of the samples were selected for further analysis. Univariate conditional logistic regression analysis revealed that high urinary concentrations of ulfaclozine, norfloxacin, and fluoroquinolones were significantly associated with an increased risk of childhood overweight/obesity (p < 0.05). After adjusting for all covariates, we observed that the association between high exposure levels of sulfaclozine (OR = 2.35; 95% CI: 1.17–4.70), norfloxacin (OR = 2.66; 95% CI: 1.01–7.08), fluoroquinolones (OR = 1.97; 95% CI: 1.02–3.78) and an increased risk of childhood overweight/obesity remained significant (p < 0.05) (Table 3).
Table 3. Logistic regression analysis of association between antibiotic exposure levels and overweight/obesity in children (N = 295).
Sex-stratified analysis revealed that high exposure levels of sulfaclozine (OR = 3.97; 95% CI: 1.63–9.62), norfloxacin (OR = 5.40; 95% CI: 1.37–21.22), fluoroquinolones (OR = 2.60; 95% CI: 1.10–6.13) and low exposure levels of oxytetracycline (OR = 4.77; 95%CI:1.26–18.06), tetracyclines (OR = 4.49; 95 CI:1.53–13.17) were significantly associated with the risk of BMI-based overweight/obesity in boys (p < 0.05). In addition, the sex-stratified analysis revealed a significant positive association between high exposure levels of β-lactams (OR = 2.99; 95% CI: 1.08–8.32) exposure and BMI-based overweight/obesity risk in girls (p < 0.05) (Table 4).
Table 4. Logistic regression analysis of association between antibiotic exposure levels and overweight/obesity in different gender Children (N = 295).
In this population-based cross-sectional study conducted in the eastern Jiangsu Province, we monitored urinary exposure to 41 antibiotics in children and investigated the relationship between urinary antibiotic levels and the risk of overweight/obesity. Our findings revealed that children who were overweight/obese had higher exposure to VAs and PVAs (such as sulfaclozine, norfloxacin, and fluoroquinolones) compared to children with normal weight. However, no significant association was observed between HAs and PHAs antibiotic exposure and overweight/obesity risk in children. After stratifying by sex and adjusting for confounding variables, we identified sex-specific differences in the association between antibiotic exposure and overweight/obesity. In recent years, VAs and PVAs have been widely used as growth promoters in animal husbandry and aquaculture, resulting in antibiotic residues in animal-derived foods becoming the primary source of foodborne antibiotics for human consumption (19, 20). The respondents included in this study were healthy and had not used antibiotics clinically or self-medicated in the past month. Therefore, children are exposed to VAs and PVAs in their daily lives, mainly through contaminated food or drinking water. When the dietary habits and living environment contributing to antibiotic exposure in children remain unchanged, the concentration of antibiotics detected in a single urine test can represent the level of exposure to foodborne antibiotics in children over a certain period.
Our study included 41 antibiotics, with a detection rate of 98.31%. This finding suggests that children are being extensively exposed to antibiotics. Among the eight major categories of antibiotics, sulfonamides and fluoroquinolones showed the highest detection rates. However, these antibiotics are not commonly prescribed for children in clinical practice. Therefore, their high detection in urine samples is more likely due to residues of veterinary drugs in food or environment exposure to antibiotics (21). Compared to previous studies, our study revealed differences in antibiotic detection rates. For example, one study in Jiangsu found a 38.6% detection rate for seven antibiotics in children’s urine (22), while another study in Shanghai identified a detection rate of 56.0% for 15 antibiotics and two metabolites in children’s urine (23). The variation in detection rate can be attributed to the different antibiotics monitored in the study. This study monitored 41 antibiotics, which may have led to a higher detection rate than that reported in other studies. In addition, the detection rates of florfenicol and trimethoprim in urine samples from children in Shanghai (13), Shandong Province, and Guangdong Province (16) were higher than those reported in our study. These results suggest regional disparities in antibiotic exposure.
Current research suggests that the biological mechanism by which antibiotics promote lipogenesis is through changes in the composition of the intestinal microbiota, resulting in bacterial imbalance. Broad-spectrum antibiotics such as fluoroquinolones can reduce intestinal biodiversity by affecting the composition of the intestinal microbiota, and even have an impact on intestinal homeostasis for a long period of time after exposure to antibiotics. Animal study confirmed that the proportion of Firmicutes and Bacteroidetes phyla/species in obese mice was higher than that in non-obese mice (24). A high value of evidence indicated that changes in Firmicutes and Bacteroidetes phyla/species levels might be significant indicators/factors for obesity in children (25). Antibiotic exposure may affect the production of short-chain fatty acids (SCFAs) by altering bacterial abundance. SCFAs serve as energy substrates to provide energy to the host and as signal regulators and participate in the regulation of lipids (26). In addition, the intestinal microbiota can influence host energy metabolism by affecting bile acid metabolism and its signaling pathways (27). Animal experiments have confirmed that continued exposure to low-dose antibiotics can significantly increase body fat content in mice (28). As early as the 1950s, antibiotics were found to promote weight gain in aquatic animals such as livestock and poultry. Therefore, low-dose antibiotics have long been used as feed additives to enhance animal growth. Studies have indicated that late application of antibiotics, compared to earlier applications, can increase animal body weight (29), providing further evidence for the growth-promoting effects of VAs and PVAs.
Our findings revealed that exposure to fluoroquinolones, particularly norfloxacin and tetracyclines was associated with overweight/obesity in boys. Fluoroquinolones are usually used to treat and prevent diseases in animals. They also represent the most commonly detected class of antibiotics in aquatic products, milk, livestock, and poultry, often detected at relatively high concentrations (30–32). Tetracyclines, on the other hand, are the most commonly used antibiotics in animal husbandry, and both fluoroquinolones and tetracyclines are classified as β-diketone antibiotics (33). Previous studies have reported that exposure to β-diketone antibiotics may lead to obesity in the older adult (34). In an animal experiment, zebrafish exposed to high concentrations of β-diketone antibiotics exhibited higher body weight and BMI compared to controls, with male zebrafish displaying higher body weights across all concentrations of β-diketone antibiotics (35). Moreover, animal experiments have shown that the use of fluoroquinolones disrupts the type and quantity of the intestinal microbiota in mice (36).
Notably, our findings revealed that exposure to lower concentrations of oxytetracycline and tetracycline was associated with overweight and obesity in children, whereas exposure to higher concentrations did not. An animal study found that exposure to low doses of penicillin or oxytetracycline caused weight gain in mice, whereas high doses resulted in weight loss (37). This phenomenon may be due to the varied effects of antibiotic exposure on body weight at different doses. Therefore, further research is needed to explore the relationship between different types and concentrations of antibiotic exposure and overweight/obesity.
Our study revealed sex-specific differences in the association between antibiotic exposure and overweight/obesity. Sulfaclozine, oxytetracycline, norfloxacin, tetracyclines, and quinolones were independently and positively associated with the risk of overweight/obesity in boys. Moreover, higher levels of β-lactams were significantly correlated with the risk of overweight/obesity in girls. This sex-specific correlation may be attributed to several factors. First, there are obvious differences in exposure levels to different antibiotics among children of different sexes (16). Second, disparities in dietary and behavioral habits between boys and girls (38) could affect the association between antibiotic exposure and obesity. Finally, the levels of sex hormones are different in boys and girls, and some studies have indicated that sex hormone levels influence the structure and function of intestinal flora (39, 40). Therefore, the sex-specific association between antibiotic exposure and childhood overweight/obesity may be related to the sex-specific gut microbiota.
Furthermore, our findings revealed that exposure to several antibiotics, such as ulfaclozine, oxytetracycline, and norfloxacin, was associated with childhood overweight/obesity. Moreover, a cross-sectional study examining the relationship between antibiotics and obesity in children in Shanghai, China, revealed that exposure to florfenicol and trimethoprim was positively correlated with overweight/obesity in children aged 8–12 years (15). In a case–control study conducted in Shandong and Guangdong provinces in China exposure to florfenicol was found to be associated with overweight/obesity in children aged 6–9 years (16). Another cross-sectional study from northern China indicated that exposure to enrofloxacin was associated with an increased risk of overweight/obesity in children aged 0–15 years (12). Several reasons may account for the observed inconsistent results. First, variations in environmental conditions and dietary habits of populations across different research areas can result in different antibiotic detection rates. In addition, the lower detection rates of some antibiotics (such as florfenicol and trimethoprim) suggest their exclusion from the association analysis.
This study is not without its limitations. Firstly, the study only investigated dietary frequency and not dietary intake. A retrospective collection of children’s dietary consumption data conducted through a questionnaire survey may have introduced recall bias. Secondly, as a cross-sectional study, it did not establish a causal relationship between antibiotic exposure and overweight/obesity. Finally, most antibiotics have short half-lives, which may not adequately represent long-term exposure levels to antibiotics.
Using BMI as an indicator of overweight/obesity and employing a biomonitoring approach, our findings reveal that exposure to foodborne antibiotics (VAs and PVAs) in children increases the risk of overweight/obesity and highlights sex disparities in the association between antibiotic exposure and overweight/obesity. It is necessary to further regulate the use of antibiotics in animal husbandry and aquaculture, enhance the monitoring of antibiotic residues in animal-derived foods, and maintain a focus on children’s exposure to foodborne antibiotics.
The original contributions presented in the study are included in the article/Supplementary material, further inquiries can be directed to the corresponding authors.
The studies involving humans were approved by the Institutional Ethics Committee of the Nantong Center for Disease Control and Prevention. The studies were conducted in accordance with the local legislation and institutional requirements. Written informed consent for participation in this study was provided by the participants’ legal guardians/next of kin.
HS: Data curation, Investigation, Software, Validation, Writing – original draft, Writing – review & editing. JH: Writing – review & editing, Methodology, Project administration, Resources. YZ: Writing – review & editing, Methodology, Project administration, Resources. XG: Writing – review & editing, Methodology, Project administration, Resources. MJ: Writing – review & editing, Conceptualization, Investigation, Software. XZ: Writing – review & editing, Conceptualization, Investigation, Software. WT: Writing – review & editing, Validation, Supervision, Visualization. WZ: Writing – review & editing, Supervision, Validation, Visualization.
The author(s) declare financial support was received for the research, authorship, and/or publication of this article. The study was supported by the research project of Jiangsu Provincial Health Commissionthe (Z2023022), Jiangsu Province Preventive Medicine Research Project General Project (Ym2023021), and the Social Livelihood Project of Nantong Science and Technology Bureau (MS2023035).
We would like to thank Editage (www.editage.cn) for English language editing.
The authors declare that the research was conducted in the absence of any commercial or financial relationships that could be construed as a potential conflict of interest.
All claims expressed in this article are solely those of the authors and do not necessarily represent those of their affiliated organizations, or those of the publisher, the editors and the reviewers. Any product that may be evaluated in this article, or claim that may be made by its manufacturer, is not guaranteed or endorsed by the publisher.
The Supplementary material for this article can be found online at: https://www.frontiersin.org/articles/10.3389/fpubh.2024.1494511/full#supplementary-material
1. Ng, M, Fleming, T, Robinson, M, Thomson, B, Graetz, N, Margono, C, et al. Global regional and national prevalence of overweight and obesity in children and adults during 1980-2013: a systematic analysis for the global burden of disease study 2013. Lancet. (2014) 384:766–81. doi: 10.1016/S0140-6736(14)60460-8
2. Llewellyn, A, Simmonds, M, Owen, CG, and Woolacott, N. Childhood obesity as a predictor of morbidity in adulthood: a systematic review and meta-analysis. Obes Rev. (2016) 17:56–67. doi: 10.1111/obr.12316
3. Chelimo, C, Camargo, CA, Morton, SMB, and Grant, CC. Association of repeated antibiotic exposure up to age 4 years with body mass at age 4.5 years. JAMA Netw Open. (2020) 3:e1917577. doi: 10.1001/jamanetworkopen.2019.17577
4. Chen, LW, Xu, J, Soh, SE, Aris, IM, Tint, MT, Gluckman, PD, et al. Implication of gut microbiota in the association between infant antibiotic exposure and childhood obesity and adiposity accumulation. Int J Obes. (2020) 44:1508–20. doi: 10.1038/s41366-020-0572-0
5. Habiba, UE, Khan, A, Mmbaga, EJ, Green, IR, and Asaduzzaman, M. Use of antibiotics in poultry and poultry farmers- a cross-sectional survey in Pakistan. Front Public Health. (2023) 11:1154668. doi: 10.3389/fpubh.2023.1154668
6. Wang, Q, Duan, YJ, Wang, SP, Wang, LT, Hou, ZL, Cui, YX, et al. Occurrence and distribution of clinical and veterinary antibiotics in the faeces of a Chinese population. J Hazard Mater. (2020) 383:121129–9. doi: 10.1016/j.jhazmat.2019.121129
7. Ben, YJ, Hu, M, Zhong, FX, Du, E, Li, Y, Zhang, H, et al. Human daily dietary intakes of antibiotic residues: dominant sources and health risks. Environ Res. (2022) 212:113387. doi: 10.1016/j.envres.2022.113387
8. Liu, Y, Liu, M, Feng, B, Wang, X, Zhao, R, Guo, Y, et al. Distribution and potential risk assessment of antibiotic pollution in the main drinking water sources of Nanjing, China. Environ Sci Pollut Res Int. (2020) 27:21429–41. doi: 10.1007/s11356-020-08516-7
9. Chu, L, Wang, HX, Su, DQ, Zhang, HW, Yimingniyazi, BH, Aili, DL, et al. Urinary antibiotics and dietary determinants in adults in Xinjiang, West China. Nutrients. (2022) 14:4748. doi: 10.3390/nu14224748
10. Köhler-Forsberg, O, Petersen, L, Gasse, C, Mortensen, PB, Dalsgaard, S, Yolken, RH, et al. A Nationwide study in Denmark of the association between treated infections and the subsequent risk of treated mental disorders in children and adolescents. JAMA Psychiatry. (2019) 76:271–9. doi: 10.1001/jamapsychiatry.2018.3428
11. Wang, H, Wang, B, Zhou, Y, and Jiang, QW. Rapid and sensitive screening and selective quantification of antibiotics in human urine by two-dimensional ultraperformance liquid chromatography coupled with quadrupole time-of-flight mass spectrometry. Anal Bioanal Chem. (2014) 406:8049–58. doi: 10.1007/s00216-014-8197-6
12. Shan, LX, Gao, M, Pan, XH, Li, WJ, Wang, JJ, Li, HX, et al. Association between fluoroquinolone exposure and children's growth and development: a multisite biomonitoring-based study in northern China. Environ Res. (2022) 214:113924. doi: 10.1016/j.envres.2022.113924
13. Zhang, Y, Tang, WF, Wang, YQ, Nian, M, Jiang, F, Zhang, J, et al. Environmental antibiotics exposure in school-age children in Shanghai and health risk assessment: a population-based representative investigation. Sci Total Environ. (2022) 824:153859. doi: 10.1016/j.scitotenv.2022.153859
14. Li, N, Ho, KWK, Ying, GG, and Deng, WJ. Veterinary antibiotics in food, drinking water, and the urine of preschool children in Hong Kong. Environ Int. (2017) 108:246–52. doi: 10.1016/j.envint.2017.08.014
15. Wang, HX, Wang, N, Wang, B, Fang, H, Fu, CW, Tang, CX, et al. Antibiotics detected in urines and adipogenesis in school children. Environ Int. (2016) 89-90:204–11. doi: 10.1016/j.envint.2016.02.005
16. Li, J, Wang, B, Liu, SJ, Zhang, Y, Chen, C, Jin, YH, et al. Antibiotic exposure and risk of overweight/obesity in school children: a multicenter, case-control study from China. Ecotoxicol Environ Saf. (2022) 240:113702. doi: 10.1016/j.ecoenv.2022.113702
17. Li, H, Zong, XN, Ji, CY, and Mi, J. Body mass index cut-offs for overweight and obesity in Chinese children and adolescents aged 2-18years. Chin J Epidemiol. (2010) 31:616–20.
18. Xu, X, Sun, WF, Xu, J, Huo, X, and Liu, XP. Simultaneous determination of residues of 40 antibiotics in human urine by ultra-high performance liquid chromatography-tandem mass spectrometry with solid phase extraction. Phys Test and Chem Anal Part B:Chem Anal. (2023) 59:591–8.
19. Zhao, L, Dong, YH, and Wang, H. Residues of veterinary antibiotics in manures from feedlot livestock in eight provinces of China. Sci Total Environ. (2010) 408:1069–75. doi: 10.1016/j.scitotenv.2009.11.014
20. Bu, Q, Wang, B, Huang, J, Deng, SB, and Yu, G. Pharmaceuticals and personal care products in the aquatic environment in China: a review. J Hazard Mater. (2013) 262:189–211. doi: 10.1016/j.jhazmat.2013.08.040
21. Zhang, JS, Liu, G, Zhang, WS, Shi, HY, Lu, G, Zhao, CA, et al. Antibiotic usage in Chinese children: a point prevalence survey. World J Pediatr. (2018) 14:335–43. doi: 10.1007/s12519-018-0176-0
22. Zhou, YJ, Zhu, F, Zheng, DY, Gao, MM, Guo, BF, Zhang, N, et al. Detection of antibiotics in the urine of children and pregnant women in Jiangsu, China. Environ Res. (2021) 196:110945. doi: 10.1016/j.envres.2021.110945
23. Wang, H, Tang, C, Yang, J, Wang, N, Jiang, F, Xia, Q, et al. Predictors of urinary antibiotics in children of Shanghai and health risk assessment. Environ Int. (2018) 121:507–14. doi: 10.1016/j.envint.2018.09.032
24. Sittipo, P, Lobionda, S, Lee, YK, and Maynard, CL. Intestinal microbiota and the immune system in metabolic diseases. J Microbiol. (2018) 56:154–62. doi: 10.1007/s12275-018-7548-y
25. Indiani, CMDSP, Rizzardi, KF, Castelo, PM, Ferraz, LFC, Darrieux, M, and Parisotto, TM. Childhood obesity and Firmicutes/Bacteroidetes ratio in the gut microbiota: a systematic review. Child Obes. (2018) 14:501–9. doi: 10.1089/chi.2018.0040
26. Vliex, LMM, Penders, J, Nauta, A, Zoetendal, EG, and Blaak, EE. The individual response to antibiotics and diet-insights into gut microbial resilience and host metabolism. Nat Rev Endocrinol. (2024) 20:387–98. doi: 10.1038/s41574-024-00966-0
27. Chen, R, Wu, W, Panyod, S, Liu, P, Chuang, H, Chen, Y, et al. Dietary exposure to antibiotic residues facilitates metabolic disorder by altering the gut microbiota and bile acid composition. mSystems. (2022) 7:e0017222. doi: 10.1128/msystems.00172-22
28. Cox, LM, Yamanishi, S, Sohn, J, Alekseyenko, AV, Leung, JM, Cho, I, et al. Altering the intestinal microbiota during a critical developmental window has lasting metabolic consequences. Cell. (2014) 158:705–21. doi: 10.1016/j.cell.2014.05.052
29. Cho, I, Yamanishi, S, Cox, L, Methé, BA, Zavadil, J, Li, K, et al. Antibiotics in early life alter the murine colonic microbiome and adiposity. Nature. (2012) 488:621–6. doi: 10.1038/nature11400
30. Liu, X, Steele, JC, and Meng, XZ. Usage, residue, and human health risk of antibiotics in Chinese aquaculture: a review. Environ Pollut. (2017) 223:161–9. doi: 10.1016/j.envpol.2017.01.003
31. Kang, HS, Lee, SB, Shinc, D, Jeong, J, Hong, JH, and Rhee, GS. Occurrence of veterinary drug residues in farmed fishery products in South Korea. Food Control. (2017) 85:57–65. doi: 10.1016/j.foodcont.2017.09.019
32. Fang, LX, Huang, ZY, Fan, LM, Hu, G, Qiu, L, and Song, C. Health risks associated with sulfonamide and quinolone residues in cultured Chinese mitten crab (Eriocheir sinensis) in China. Mar Pollut Bull. (2021) 165:112184. doi: 10.1016/j.marpolbul.2021.112184
33. Qu, S, Wang, X, Tong, C, and Wu, J. Metal ion mediated molecularly imprinted polymer for selective capturing antibiotics containing beta-diketone structure. J Chromatogr A. (2010) 1217:8205–11. doi: 10.1016/j.chroma.2010.10.097
34. Sang, YR, Zhang, JJ, Liu, KY, Wang, QN, Wang, SF, Sheng, J, et al. Antibiotics biomonitored in urine and obesogenic risk in a community-dwelling elderly population. Ecotoxicol Environ Saf. (2021) 210:111863. doi: 10.1016/j.ecoenv.2020.111863
35. Li, J, Liu, J, Zhang, Y, Wang, X, Li, W, Zhang, H, et al. Screening on the differentially expressed mirnas in zebrafish (danio rerio)exposed to trace β-diketone antibiotics and their related functions. Aquat Toxicol. (2016) 178:27–38. doi: 10.1016/j.aquatox.2016.07.009
36. Gu, SL, Gong, Y, Zhang, J, Chen, Y, Wu, Z, Xu, Q, et al. Effect of the short-term use of fluoroquinolone and β-lactam antibiotics on mouse gut microbiota. Infect Drug Resist. (2020) 13:4547–58. doi: 10.2147/IDR.S281274
37. Dubos, R, Schaedler, RW, and Costello, RL. The effect of antibacterial drugs on the weight of mice. J Exp Med. (1963) 117:245–57. doi: 10.1084/jem.117.2.245
38. Wen, J, Geng, S, Zhu, L, Yao, X, Zhou, Y, and Shen, F. Urinary antibiotic concentrations in preschool children from eastern China and health risk assessment. Environ Sci Pollut Res Int. (2023) 30:82547–59. doi: 10.1007/s11356-023-28209-1
39. Gavin, KM, and Bessesen, DH. Sex differences in adipose tissue function. Endocrinol Metab Clin N Am. (2020) 49:215–28. doi: 10.1016/j.ecl.2020.02.008
Keywords: antibiotics, urine, overweight, obesity, children
Citation: Sun H, Huang J, Zhou Y, Guo X, Jiao M, Zhu X, Tan W and Zhang W (2024) Antibiotic exposure and risk of overweight/obesity in children: a biomonitoring-based study from eastern Jiangsu, China. Front. Public Health. 12:1494511. doi: 10.3389/fpubh.2024.1494511
Received: 11 September 2024; Accepted: 21 October 2024;
Published: 08 November 2024.
Edited by:
Catarine Santos Da Silva, Universidade Federal do Rio Grande do Norte, BrazilReviewed by:
Muhammad Akbar Shahid, Bahauddin Zakariya University, PakistanCopyright © 2024 Sun, Huang, Zhou, Guo, Jiao, Zhu, Tan and Zhang. This is an open-access article distributed under the terms of the Creative Commons Attribution License (CC BY). The use, distribution or reproduction in other forums is permitted, provided the original author(s) and the copyright owner(s) are credited and that the original publication in this journal is cited, in accordance with accepted academic practice. No use, distribution or reproduction is permitted which does not comply with these terms.
*Correspondence: Weiwei Tan, dHd3MDUxM0AxNjMuY29t; Weibing Zhang, bnR6d2I3MUAxNjMuY29t
Disclaimer: All claims expressed in this article are solely those of the authors and do not necessarily represent those of their affiliated organizations, or those of the publisher, the editors and the reviewers. Any product that may be evaluated in this article or claim that may be made by its manufacturer is not guaranteed or endorsed by the publisher.
Research integrity at Frontiers
Learn more about the work of our research integrity team to safeguard the quality of each article we publish.