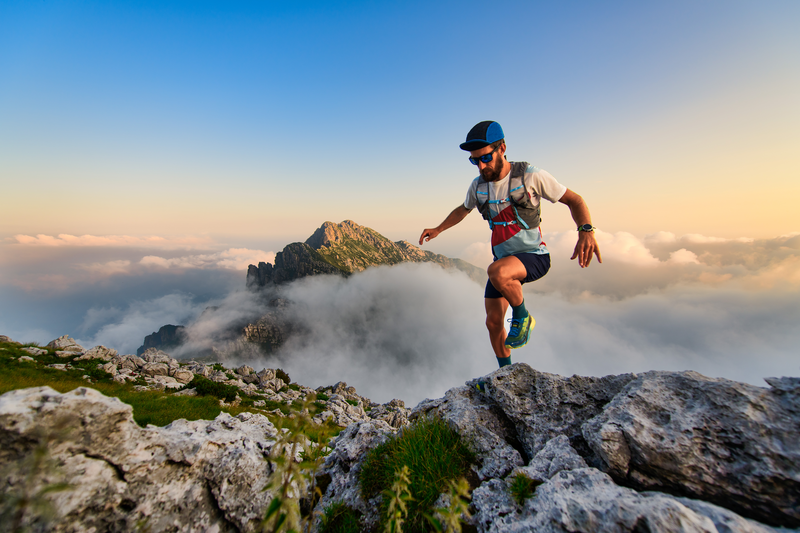
95% of researchers rate our articles as excellent or good
Learn more about the work of our research integrity team to safeguard the quality of each article we publish.
Find out more
SYSTEMATIC REVIEW article
Front. Public Health , 07 January 2025
Sec. Children and Health
Volume 12 - 2024 | https://doi.org/10.3389/fpubh.2024.1489141
Introduction: This study aimed to systematically review the effects of different physical activity programs on the fundamental movement skills of 3 - 7-year-old children.
Methods: For this review, the databases of CNKI, Web of Science, and PubMed were searched to collect relevant literature on the effects of different physical activity program interventions on fundamental movement skills, and a total of 10 articles with 1,121 subjects were included. The Cochrane Risk of Bias Assessment Tool was used to assess the quality of the literature, and meta-analysis was performed using Review Manager 5.4 software.
Results: Physical activity significantly influenced children’s running ability, horizontal jump, dribbling the ball, kicking ability, catching ability, overhand throwing, striking a stationary ball, and dynamic balance. However, the intervention effect was insignificant for the hop and underhand throwing abilities. The intervention effects for running ability, horizontal jump, kicking ability, and catching ability were better at less than 12 weeks than at 12 weeks and above. In addition, an intervention duration of 90 min or more was better than less than 90 min for running ability and horizontal jump.
Conclusion: Future research is recommended to focus on the common factors of the intervention effects of physical activity programs to develop more precise and effective intervention practices to further improve children’s fundamental movement skill levels.
Fundamental Movement Skills (FMS) refer to the ability to coordinate basic human movements (1). They are considered to be the ‘building blocks’ of more complex movement skills required for games or other physical activities (2), including mainly gross motor skills such as running, jumping, etc., object control skills such as grasping and throwing a ball, and stability skills such as balancing and swinging (3). According to Gallahue, a model of movement development was proposed in 2012, broadly divided into four phases: Reflexive Movement Phase, Rudimentary Movement Phase, Fundamental Movement Phase, and Specialized Movement Phase. The Fundamental Movement Phase is from 2 to 7 years old and covers toddlers and early childhood, as children gradually develop more complex movement skills and coordination during this period, including fundamental movement skills such as running, jumping, throwing, and so on (4). As a result, during this stage, children’s coordination and object control abilities improve significantly. Adequate exercise practice and effective instruction are needed to allow children to increase their confidence in exercise and their FMS levels. Children with high levels of FMS will be more able to participate in physical activity (5), improve cardiorespiratory fitness (6), and reduce obesity rates. Children with high levels of FMS will be able to participate in a wide variety of physical activities with high levels of FMS (7).
Physical activity (PA), which encompasses all voluntary bodily movements produced by skeletal muscles that result in energy expenditure (8), is an important factor in promoting healthy child development, and participation in physical activity in early childhood confers considerable benefits, such as improving obesity, promoting cognitive development, and skeletal, psychosocial, and cardiometabolic metabolism (9). The World Health Organization (WHO) recommends that preschool children should engage in at least 60 min of moderate to vigorous physical activity (MVPA) per day (10), yet more than 85% of children and adolescents worldwide do not meet the WHO guidelines. With continuous social and economic development, people’s living standards continue to improve. The increase in sedentary behavior and lack of physical activity has led to an increase in the risk rate of obesity in children year by year (11, 12), and physically insufficient behaviors are easy to form in childhood and continue into adulthood, which ultimately leads to other health problems (13). Therefore, it is essential to understand the underlying mechanisms of physical activity (14).
Stodden et al. noted that PA and FMS have a mutually beneficial relationship, with PA promoting the development of FMS during childhood and FMS promoting PA involvement at a slightly older age (15). One study confirmed the positive correlation between FMS and organized PA (16). Another narrative review found that 8 out of 11 studies on children aged 3–5 years showed a significant relationship between FMS and PA (17). However, longitudinal studies have highlighted significant uncertainty in directly linking physical activity to FMS (18). In a meta-analysis, researchers found that the association between FMS and PA was inconsistent in children (3). Another investigation of studies that used PA as a predictor of FMS found that objective measures of moderate to vigorous physical activity (MVPA) at 3.5 years of age did not predict FMS in 5-year-old Australian children (19). This suggests that our understanding of how physical activity impacts FMS is still incomplete, and further research is necessary.
Notably, children’s FMS does not develop naturally over time (20) but requires intentional cultivation through various interventions, such as ongoing guidance, feedback, and practice (21). Consequently, a range of physical activity programs tailored to children’s physical and mental developmental stages has been created, aiming to enhance FMS. Current research has indicated that jumping classes (22, 23), rhythmic classes (24), active play (25), core movement instruction (26), strength training classes (27), motor intervention classes (28, 29), and ball games (30, 31) are all beneficial in developing children’s FMS. These programs promote the comprehensive and balanced development of children’s FMS by integrating diverse sports elements and employing open-ended physical education games that emphasize enjoyment and variety (32, 33). The main purpose of this study is to explore the effects of different types of physical activity programs on improving children’s FMS levels. The study focuses on running ability, jumping ability, object control ability, and balance ability as key indicators. Running and jumping directly reflect children’s coordination and lower limb strength, which are core skills in daily life and many physical activities. Object control skills include throwing and catching, reflecting children’s upper body coordination and fine motor skills. Balance skills help children maintain stability in both static and dynamic situations, reduce the risk of falling, and improve overall body coordination. By using meta-analysis to assess the impact of physical activity on children’s FMS, the study aimed to elucidate a precise perspective on how physical activity interventions can be more effective in improving children’s levels of fundamental movement skills, laying the groundwork for more effective promotion of lifelong physical education in the future.
This study was guided by the Preferred Reporting Items for Systematic Evaluation and Meta-Analysis (PRISMA) (34), registered in the PROSPERO database under CRD42024548531. By searching the databases of CNKI, PubMed, and Web of Science, the search formula in Chinese and English was (Fundamental movement skill OR gross motor movement) AND (Physical activity OR exercise) AND (children OR kid) AND (RCT OR Randomized controlled experiment); the search formula in English was (Fundamental movement skill OR gross motor skill) AND (physical activity OR Physical exercise) AND (children OR kid) AND (RCT OR Randomized controlled experiment).
(1) Randomized controlled trials of different physical activity programs that intervene in children’s basic movement skills and language restricted to Chinese and English. (2) Children aged 3–7 years with normal physical development. (3) Intervention period of at least six weeks.
(1) Literature not meeting inclusion criteria (2) Children with cognitive and behavioral disorders (3) Experimental and control groups with missing basic information (4) Duplicated literature (5) Lack of full-text literature (6) TGMD-2 not used as a measurement tool.
The experimental group was supervised by different types of physical activity programs. The duration of the intervention ranged from six weeks to two years. The control group participated in physical activity as usual, but the frequency and intensity of the exercises were unlimited. The experimental and control subjects were similar in terms of age, sex ratio, and level of physical activity before the intervention.
(1) running ability, using running as a test method; (2) jumping ability, using standing long jump and one-legged jump as test methods; (3) object control ability, using TGMD-2 as a test method, mainly including kicking, catching, striking a stationary ball, overhand throwing, and underhand throwing (because most studies in the retrieved literature used TGMD as the measure, object control under TGMD was selected as the measure in this study); and (4) stability, measured by walking on a balance beam.
The article’s quality assessment and data extraction were mainly carried out by two researchers, with the third researcher participating in the quality assessment and data extraction in case of disagreement. After extraction, the difference between the mean and standard deviation of the experimental and control groups before and after the intervention was calculated. The mean was calculated by subtracting the pre-test from the post-test, and the standard deviation was calculated using the formula “SD difference2 = SD baseline2 + SD final2–2*R*SD baseline * SD final, R = 0.5” (35).
The included literature was scored using the criteria of the Cochrane Handbook of Systematic Evaluation (36) risk of bias assessment scale, which were (1) random sequence generation; (2) allocation concealment; (3) blinding of participants and personnel; (4) blinding of outcome assessment; (5) incompleteness of outcome data;(6) selective reporting; (7) other bias. Each criterion has three options: low bias, high bias, and unclear. When the number of low biases in the literature is ≥4, it is assigned to category A; when the number of low biases in the literature is ≥2 and < 4, it is assigned to category B; and when the number of low biases in the literature is <2, it is assigned to category C. In total, five articles were assigned to Category A (22, 26, 28, 29, 31), and five articles were assigned to Category B (23–25, 27, 30), and the overall quality of the literature was high (see Figures 1, 2).
Meta-analysis was conducted using Review Manager 5.4 software (37). The chi-square test was used to determine the heterogeneity between studies, supplemented by I2 for quantitative evaluation. An I2 value greater than 50% indicated heterogeneity, prompting the use of a random-effects model, whereas an I2 value less than 50% signified homogeneity, leading to the selection of a fixed-effects model. Effect sizes were categorized based on the absolute value of the standardized mean difference (SMD): 0–0.4 indicated a small effect, 0.4–0.8 a medium effect, and values greater than 0.8 a large effect (38).
Through the search of Chinese and English literature, a total of 2,500 references were retrieved; 744 references were excluded as duplicates, 1,708 references that did not meet the inclusion criteria were excluded after reading the title and abstract, 29 references were excluded after reading the full text, and 10 references were finally excluded, of which a total of 6 references were in English (22–25, 28, 29, 31) and a total of 4 references were in Chinese (26, 27, 30, 31) (see Figure 3).
A total of 1,121 children aged 3–7 years were included: 580 children in the experimental group and 541 children in the control group. The interventions were different types of physical activity programs: two differentiated between genders (23, 27), two (25, 27) illustrated the intensity of the interventions, two interventions were conducted in different age groups (24, 26), and four studies had a follow-up survey after the intervention (22, 23, 28, 31) (see Tables 1, 2).
A total of six pieces of literature have studied running ability in this study. Figure 4 shows the forest plot of running ability between the experimental and control groups after the intervention, χ2 = 445.82, df = 9 (p < 0.00001), and I2 = 98% between the experimental and control groups. It can be assumed that there is heterogeneity between the two groups, and meta-analysis was conducted using the random effects model. The results showed SMD = 2.51, 95% CI [1.22. 3.79], p = 0.0001, which is statistically significant when combined, and the diamond is to the right of the null line, indicating that the difference between the groups is statistically significant, suggesting that physical activity improves children’s running ability. Figure 5 shows that χ2 = 92.93, df = 9 (p < 0.00001), I2 = 90%, SMD = −1.09, 95% CI [−1.61, −0.58], p < 0.00001, indicating that there is a significant change in running ability before and after the intervention in the experimental group.
Figure 5. Forest plot of the running ability of the experimental group before and after the intervention.
Jumping ability was mainly measured by horizontal jump and hop ability. Figure 6 shows the forest plot of the horizontal jump ability of the experimental group and the control group after the intervention, χ2 = 143.32, df = 9 (p < 0.00001), I2 = 94% between the experimental group and the control group. It can be assumed that there is heterogeneity between the two groups, and meta-analysis was performed using the random effects model. SMD = 1.29, 95% CI [0.63, 1.96], p = 0.0001, the diamond is to the right of the null line, with a high degree of effect, indicating that the difference between the groups is statistically significant, suggesting that the different types of physical activity programs have an effect on improving children’s jumping ability. As can be seen in Figure 7, the horizontal jump χ2 = 55.63, df = 9 (p < 0.00001), I2 = 84%, SMD = −0.88, 95% CI [−1.04, −0.73], p < 0.00001 in the experimental group, indicating that there was a significant change in the horizontal jump ability of the experimental group before and after the intervention.
Figure 6. Forest plot comparing horizontal jump of the experimental and control groups after the intervention.
Figure 7. Forest plot comparing the horizontal jump of the experimental group before and after the intervention.
Figure 8 shows the forest plot of hop ability between the experimental and control groups after the intervention. χ2 = 79.93, df = 7 (p < 0.00001), I2 = 91% between the experimental group and the control group, it can be considered that there is heterogeneity between the two groups, and meta-analysis was carried out using the random-effects model. SMD = 0.37, 95% CI [−0.22, 0.95], p = 0.22, and the rhombus intersects the null line, indicating that the difference between the groups was not statistically significant and that different kinds of physical activity programs were not able to promote children’s one-legged jumping ability. As can be seen from Figure 9, the χ2 = 42.33, df = 7 (p < 0.00001), I2 = 83%, SMD = −0.90, 95% CI [−1.33, −0.46], p < 0.0001, indicating that there was a significant change in hop ability before and after the intervention in the experimental group.
Figure 8. Forest plot comparing the hop ability of the experimental and control groups after the intervention.
Figure 9. Forest plot comparing the hop ability of the experimental group before and after intervention.
Figure 10 shows the forest plot of locomotion skills between the experimental group and the control group after the intervention. χ2 = 380.78, df = 11 (p < 0.00001), I2 = 97% between the experimental group and the control group, it can be considered that there is heterogeneity between the two groups, and meta-analysis was carried out using the random effects model. SMD = 1.87, 95% CI [0.92, 2.81], p = 0.0001, the diamond is located on the right side of the null line with a high effect, indicating that different types of physical activity programs have a better-facilitating benefit on children’s overall displacement ability. Figure 11 shows that the experimental group χ2 = 235.27, df = 10 (p < 0.00001), I2 = 96%, SMD = −2.07, 95% CI [−2.84, −1.30], p = 0.0001, and the rhombus is located on the left side of the null line, suggesting that there is a significant change in overall displacement ability before and after the experimental group.
Figure 10. Forest plot of locomotion skill of the experimental and control groups after the intervention.
Figure 11. Forest plot of locomotion skill of the experimental group before and after the intervention.
As can be seen in Figure 12, the heterogeneity of the effect of the physical activity program on children’s object control ability was χ2 = 1029.58, df = 44 (p < 0.00001), I2 = 96%, and therefore, meta-analysis was conducted using a random effects model with SMD = 1.21, 95% CI [0.83, 1.60], p < 0.00001, and SMD > 0.8, which indicated that the physical activity program intervention promoted children’s object control better and there were significant differences between subgroups.
Figure 12. Forest plot comparing object control in the experimental and control groups after the intervention.
Heterogeneity in dribbling the ball was observed (χ2 = 34.53, df = 6, p < 0.00001, I2 = 83%), indicating substantial heterogeneity. A random-effects model was applied, yielding an SMD of 0.97 (95% CI [0.50, 1.44], p < 0.00001). The diamond-shaped squares were positioned to the right of the null line, suggesting that the physical activity program significantly enhanced the promotion of in-situ racket skills.
Heterogeneity in kicking the ball was observed (χ2 = 307.26, df = 8, p < 0.00001, I2 = 97%), indicating high heterogeneity. A random-effects model was applied, resulting in an SMD of 1.67 (95% CI [0.52, 2.81], p = 0.004). The diamond-shaped squares were positioned to the right of the null line, indicating that the physical activity sessions significantly improved the ability to kick the ball.
Heterogeneity in catching the ball was observed (χ2 = 110.76, df = 8, p < 0.00001, I2 = 93%), indicating substantial heterogeneity. A random-effects model was applied, yielding an SMD of 0.90 (95% CI [0.31, 1.49], p = 0.003). The diamond-shaped squares were positioned to the right of the null line, suggesting that the physical activity sessions significantly improved two-handed catching ability.
Heterogeneity in striking a stationary ball was noted (χ2 = 173.32, df = 6, p < 0.00001, I2 = 97%), indicating high heterogeneity. A random-effects model was used, resulting in an SMD of 1.51 (95% CI [0.33, 2.69], p = 0.01). The diamond-shaped squares were located to the right of the null line, indicating that physical activity sessions significantly enhanced the ability to strike a stationary ball.
Heterogeneity in overhand throwing was observed (χ2 = 96.15, df = 6, p < 0.00001, I2 = 94%), indicating substantial heterogeneity. A random-effects model was applied, resulting in an SMD of 1.57 (95% CI [0.71, 2.44], p = 0.0004). The diamond-shaped squares were positioned to the right of the null line, suggesting that the physical activity sessions significantly improved overhand throwing ability.
Heterogeneity in underhand throwing was observed (χ2 = 247.54, df = 5, p < 0.00001, I2 = 98%), indicating substantial heterogeneity. A random-effects model was applied, resulting in an SMD of 0.58 (95% CI [−1.14, 2.29], p < 0.00001). The rhombus-shaped squares intersected the null line, suggesting that the physical activity sessions did not significantly improve underhand throwing ability.
A total of two literature tests on stability ability were included in this study, and the balance beam walking method was used to assess children’s dynamic balance ability. Figure 13 shows the forest plot of the dynamic balance ability between the experimental and control groups after the intervention. χ2 = 80.87, df = 3 (p < 0.00001), and I2 = 96% between the experimental group and the control group, which can be regarded as the existence of heterogeneity between the two groups, and the random effect model was used to Meta-analysis was performed. SMD = −2.97, 95% CI [−5.27, −0.67], p = 0.01, the diamond is located on the left side of the null line, which indicates that physical activity has a good benefit in promoting children’s stability ability. As can be seen in Figure 14, χ2 = 3.26, df = 3 (p = 0.35), I2 = 8%, SMD = 0.67, 95% CI [0.38, 0.96], p < 0.00001, indicating that the experimental group’s balancing ability was improved after the intervention.
Figure 13. Forest plot comparing object control in the experimental and control groups after the intervention.
Figure 14. Forest plot of the dynamic balance ability of the experimental group before and after the intervention.
Due to the variation in the content and methodology of the literature cited, sensitivity analysis was conducted to assess the effect of this heterogeneity. In the analysis of heterogeneity for each motor ability, the results showed that the I2 for running ability, horizontal jump, hop ability, locomotion skill, dribbling, catching, kicking, striking, overhand throwing, and underhand throwing ability remained high after excluding the selected literature one by one, but it did not affect the stability of the experimental results. This means that although there were differences between the different studies, their effect on the overall effect size was not significant, suggesting that the results were relatively robust.
In the heterogeneity analysis of balance ability, after excluding Lu’s study (31), the I2 dropped significantly from 96 to 0%, suggesting that this study might be a major source of heterogeneity. To further explore the reasons for heterogeneity differences, we conducted an in-depth analysis from three aspects: the intervention participants, content, and duration. Regarding the intervention participants, Lu’s study (31) involved children aged 5–6, while Hu X’s study included children aged 3–6 (24), dividing them into three age groups for comparison. As for the intervention content, Lu’s study (31) used toddler soccer training, mainly focusing on skills such as dribbling, ball control, trapping, and passing to develop children’s FMS. The course was more geared towards reaction and speed, with balance ability being applied but not as a core focus of the curriculum. As a result, the improvement in balance ability may not have been significant. In contrast, Hu et al.’s study (24) used a novel rhythmic exercise as the intervention method. This new rhythmic exercise was built upon traditional rhythmic movements (such as flexing, vibrating, twisting, and circling, which aid in the development of balance ability) and incorporated exercises for locomotor and object control abilities. This more systematic and comprehensive intervention method likely placed greater emphasis on improving balance ability, which might explain the more significant effects observed in this study. In terms of intervention duration, the duration of Hu et al.’s study (24) was longer than that of Lu’s study (31), giving children more time to participate and practice. A longer intervention period generally provides more opportunities for children to reinforce newly acquired skills. Particularly for balance, which requires repetitive practice and fine motor control, increased time might have had a substantial impact.
As less of the included literature measured balance ability, which was not suitable for subgroup analysis, this study analyzed subgroups in terms of weekly intervention duration and intervention period as well as different age groups. When comparing the performance of children in the two subgroups with weekly intervention durations of 90 min and below and 90 min and above in various basic motor skill tests, the results showed that SMD90min above > SMD90min below in running ability, horizontal jump, and catching ability, suggesting that the effect of the weekly intervention durations of 90 min and above for running ability, horizontal jump, and catching ability is better than that of below 90 min. In kicking ability, although SMD above 90 min = 2.81 is a large effect size, it is not a significant difference, which may be related to the training method or individual differences, and more data or further research is needed to confirm this (see Table 3).
This study divided the intervention cycle into less than 12 weeks, 12 weeks, and more than 12 weeks. The results showed that the intervention effect of less than 12 weeks was better than 12 weeks and more for running ability, horizontal jump, kicking ability, and catching ability; kicking ability was not significant at 12 weeks. There were no between-group differences in catching ability beyond 12 weeks. This study suggests that further observations are needed to draw accurate conclusions about the mastery of object control skills (see Table 4).
This study compares the fundamental movement skills of 3–5 and 5–7 year-olds separately. 3-5-year-olds showed a medium and significant difference in effect sizes for running ability, horizontal jump, and catching, while 5-7-year-olds did not show a significant difference for running ability, horizontal jump, and catching. 3-5-year-olds showed a statistically significant difference in kicking ability with a medium effect size, and 5-7-year-olds were more prominent in this indicator with a significant difference and a large effect size, indicating a significant increase in kicking ability for this age group. Children showed more prominent performance on this indicator with a significant difference and large effect size, indicating that children in this age group showed significant improvement in kicking ability (see Table 5).
Some studies have confirmed that physical activity programs can improve FMS levels. Ali et al. (23) found significant improvements in children’s FMS levels through a ten-week physical activity program intervention. Jones et al. (22) made a similar observation in 2011 through a twenty-week instrumental intervention. However, there was a bias in children’s jumping ability, which could be attributed to the different focuses of different physical activity programs and the different physical fitness of the children involved in the experiment, resulting in a bias in results. This may be due to the program’s different focuses and the physical qualities of the children involved in the experiment. Magistro et al. (29) proposed in their study “classroom-based physical activity,” a new type of physical activity program that combines math lessons with physical activity by starting each lesson with a 10-min running walk and other physical activities. At the beginning of each lesson, the teacher warms up with 10 min of physical activity such as running and walking. Then, in the explanation section, the teacher explains the mathematical concepts for 15 min, demonstrates the physical activity learning task, guides the children to apply the mathematical knowledge explained in the explanation section through the physical activity learning task, and then concludes the lesson. Through the integrated teaching of education and physical activity, children’s potential to improve their knowledge and skills is fully realized.
Different types of physical activity programs have a positive effect on the improvement of children’s displacement ability, which mainly consists of running ability and jumping ability, and when analyzing the effect of different physical activity programs on running ability, children’s running ability was significantly improved after the intervention, as evidenced by relevant studies (22, 26, 28, 30, 31), mainly because the running ability is one of the earliest abilities to emerge in children, however, Hu et al. (24) found in his study that after a period of intervention, the running ability of the 3-year-old experimental group was lower than the running ability of the control group, probably because some of the children at that time are still in the early stages of development, their coordination and muscle control may not have reached the level of their peers, and some of the children may have already developed better coordination and muscle control, leading to the differences in results.
When analyzing the impact of physical activity on horizontal jump, it was observed that children’s horizontal jump ability significantly improved after the intervention. However, Eyre et al.’s study (28) noted that children in the experimental group exhibited lower horizontal jump ability than those in the control group. This disparity may stem from demographic differences between the two groups. Notably, in Eyre et al.’s study (28), the experimental group was comprised predominantly of South Asian children, while the control group consisted mostly of white children. Such population variances might have influenced the outcomes due to diverse cultural and genetic backgrounds, thereby impacting the children’s athletic performance. Previous research (22, 30) has demonstrated a significant enhancement in hop ability following a training period. However, Hu et al. (24) revealed no significant discrepancy in hop ability between the experimental and control groups at the ages of 3 and 5 years post-intervention. This outcome could be attributed to the inherent demands of hop exercises, which necessitate greater muscle strength, multi-limb coordination, and dynamic balance compared to vertical jumps for proficient execution. Moreover, mastering jumping skills typically requires considerable time and practice. Furthermore, jumping entails a multifaceted task requiring extensive skill development, demanding children to possess adequate strength and coordination between their arms and legs (39).
The physical activity program successfully enhanced children’s object control skills, yet no significant improvement was observed in hand-throwing. This aligns with the findings of Hu et al. (24), where the hand-throwing ability of the 4-year-old group showed no notable change over time, possibly due to irregular movements and a lack of coordination. Regarding catching the ball, Jones et al. (22) discovered that children’s proficiency in two-handed catching did not significantly improve after a specific intervention period. This might be attributed to the study’s emphasis on enhancing children’s displacement ability rather than giving sufficient attention to the development of object control skills, leading to a lack of significant progress in this aspect of performance.
As this study has limited literature regarding balance skills, the analysis of such skills may be less precise, and further validation is necessary in the future.
Most of the studies had no more than 12 weeks of intervention. The duration of the intervention tended to be no more than 90 min per week, so this study argues that a longer duration of intervention does not necessarily lead to better exercise performance, which is in line with the conclusions reached by Li et al. (40). Additionally, a meta-analysis on the effects of physical activity programs on gross motor skill development in preschool children reached a similar conclusion (41). Except for interventions lasting longer than 12 weeks, other interventions with varying durations, frequencies, and cycles significantly promoted the development of gross motor skills in young children. This finding suggests that high-frequency and long-duration interventions may reduce children’s adherence and enthusiasm for physical activities. Furthermore, prolonged interventions might be subject to a “ceiling effect,” where further improvements become minimal once a certain developmental threshold has been reached (6).
In addition to intervention duration, exercise intensity is an important factor influencing the effectiveness of physical activity. However, in the literature surveyed, there are few clear ranges of exercise intensity for children aged 3–7 years, which may be due to the greater challenge of monitoring children’s physical activity. When providing physical activity recommendations, the World Health Organization (WHO) has specifically emphasized that a differentiated approach to instruction should be taken for children aged 3–5 years versus 5–6 years (42), and in analyzing the findings of this study, it was found that the level of basic motor skills of children aged 3–5 years was higher than that of children aged 5–7 years, especially in terms of running ability, horizontal jump, and catching ability, and that the kicking ability of 5-7-year-olds performed better, which is contrary to the results obtained by Gao and Wang (43), probably because at the age of 3–5 years, children are usually in the early stages of growth and development, their bodies may be better suited for fast running, jumping and catching the ball, and their muscle control and coordination may also be relatively good at this age. At the same time, their cognitive abilities are developing rapidly, which makes it easier for them to understand and execute simple technical movements. However, by the age of 5–7 years, their cognitive abilities may be developing further, allowing them to better understand and perform more complex skills, and this difference may also be related to their experience of physical activity: 3–5-year-olds are likely to be more involved in basic physical activity, whereas, by the age of 5–7 years, they may be exposed to more types of sport, like ball games, which require more complex skills, and therefore increase competence in these areas. Thus, kicking a ball may require more skill and coordination relative to running, horizontal jumping, and catching, which makes children aged 5–7 perform better in this area. More literature is needed to confirm this, as the methodological approach varies from experiment to experiment and the physical fitness of the children participating.
Based on the findings of this study, future research should prioritize school and community-based efforts. Schools are particularly well-suited for fostering PA and developing FMS, as children spend approximately 50% of their time in school. Building a strong foundation for FMS during childhood is essential (21), as this is a period when children acquire skills more quickly and easily, without the self-consciousness or shame associated with imperfect motor performance. A strong foundation not only breaks down a “proficiency barrier” (44) that exists prior to the different skill acquisition stages of children between the ages of 3 and 7 and 7 and 12 but also allows for the transition of children’s motor skill levels to a more specialized stage in an organized PA program. Schools need to ensure that they provide rich and varied physical activity content that includes a variety of movements such as running, jumping, throwing, and catching and that the activities are fun and interactive to cater to different children’s interests and abilities. Secondly, physical education teachers should receive professional training to effectively instruct and motivate children to promote the development of their motor skills. To ensure effective implementation of the curriculum, schools and communities should also work closely with parents to develop and implement family activity programs to further promote children’s motor development in the family environment. Schools and communities are required to provide children with continuous assessment and monitoring in light of their physical and mental development to provide more useful values for the development of fundamental movement skills by establishing the optimal time, frequency, and period of intervention.
The limited number of literature included in this study and the small number of balance tests may have resulted in an insufficient sample size, limiting to some extent the overall understanding of children’s fundamental movement skills, which could lead to the occurrence of a chance error. Future studies should expand the literature search to include more databases and keywords to increase the number of literature included. The absence of some of the key rubrics in the text had an impact on the credibility of the results, so future articles should be selected to ensure that all important rubrics are included and reported. Meanwhile, sensitivity analyses should be used in literature screening and data extraction to assess the possible impact on the results of studies with missing key rubrics. This further increases the possibility of analytical error due to the differences in experimental populations, interventions, study designs, and outcome assessments across studies. Therefore, multi-group subgroup analyses or sensitivity analyses are conducted to explore the impact of different study characteristics on the results. In terms of literature quality assessment, the assessment methods used may lack sufficient objectivity and systematicity, which may also affect the judgment of literature quality, and multiple risk assessment tools may be used in the future to improve the objectivity and consistency of the assessment. In addition, subgroup analyses of gender differences were not conducted in the study, and in future studies, gender-specific data should be collected and reported, and gender subgroup analyses should be conducted to explore the effects of gender on children’s fundamental movement skills.
In summary, different types of physical activity programs had a positive impact on promoting children’s FMS in seven main areas: running ability, standing long jump, tapping a ball in place, kicking a ball, catching a ball with both hands, hitting a stationary ball, and overhand throwing, with no significant improvement in one-legged jumping and underhand throwing, and with fewer tests of balance in the included literature, the results obtained need to be further validated. Meanwhile, the subgroup analysis revealed that interventions exceeding 90 min per week significantly positively impacted children’s running ability, horizontal jumping, and catching skills. Additionally, interventions lasting less than 12 weeks showed greater effectiveness than those of 12 weeks or longer in improving running, horizontal jump, kicking, and catching abilities. Children aged 3–5 years performed better in running, horizontal jumping, and catching, while those aged 5–7 years showed better improvements in kicking ability. This suggests that future research should focus more on the duration of interventions and the careful planning of intervention periods to ensure that children’s motor skills are maximized. It also highlights the importance of selecting appropriate physical activity programs tailored to the growth and developmental characteristics of different age groups for the comprehensive development of children. Furthermore, as a critical factor, the intensity of interventions should be explored in greater depth in future studies.
The original contributions presented in the study are included in the article/Supplementary material, further inquiries can be directed to the corresponding author.
YY: Writing – original draft. XM: Writing – review & editing. WL: Writing – review & editing. BW: Writing – review & editing. LF: Writing – review & editing.
The author(s) declare financial support was received for the research, authorship, and/or publication of this article. This research was supported by the National Ministry of Education Humanities and Social Sciences Fund General Project (grant no. 21YJAZH01). The Jinan Huaiyin District Education and Sports Bureau Educational Research Project titled “Construction of an Intelligent Evaluation System for Children’s Physical Literacy under the Implementation of the ‘Double Reduction’ Policy”.
The authors declare that the research was conducted in the absence of any commercial or financial relationships that could be construed as a potential conflict of interest.
All claims expressed in this article are solely those of the authors and do not necessarily represent those of their affiliated organizations, or those of the publisher, the editors and the reviewers. Any product that may be evaluated in this article, or claim that may be made by its manufacturer, is not guaranteed or endorsed by the publisher.
The Supplementary material for this article can be found online at: https://www.frontiersin.org/articles/10.3389/fpubh.2024.1489141/full#supplementary-material
1. Clark, JE. Motor Development In: VS Ramachandran, editor. Encyclopedia of human behavior. New York: Academic Press (1994). 250.
2. Logan, SW, Ross, SM, Chee, K, Stodden, DF, and Robinson, LE. Fundamental motor skills: a systematic review of terminology. J Sports Sci. (2018) 36:781–96. doi: 10.1080/02640414.2017.1340660
3. Barnett, LM, Lai, SK, Veldman, SLC, Hardy, LL, Cliff, DP, Morgan, PJ, et al. Correlates of gross motor competence in children and adolescents: a systematic review and meta-analysis. Sports Med. (2016) 46:1663–88. doi: 10.1007/s40279-016-0495-z
4. Gallahue David, L. “Understanding motor development: Infants, children, adolescents, adults, 7th ed.” New York, NY: McGraw - Hill. (2012).
5. Burns, RD, Bai, Y, Byun, W, Colotti, TE, Pfledderer, CD, Kwon, S, et al. Bidirectional relationships of physical activity and gross motor skills before and after summer break: application of a cross-lagged panel model. Sport Health Sci. (2022) 11:244–51. doi: 10.1016/j.jshs.2020.07.001
6. Cattuzzo, MT, dos Santos, HR, Ré, AHN, de Oliveira, IS, Melo, BM, deSousa, MM, et al. Motor competence and health related physical fitness in youth: a systematic review. J Sci Med Sport. (2016) 19:123–9. doi: 10.1016/j.jsams.2014.12.004
7. Robinson, LE, Stodden, DF, Barnett, LM, Lopes, VP, Logan, SW, Rodrigues, LP, et al. Motor competence and its effect on positive development a trajectories of health. Sports Med. (2015) 45:1273–84. doi: 10.1007/s40279-015-0351-6
8. Caspersen, CJ, and Christenson, PGM. Physical activity, exercise, and physical fitness: definitions and distinctions for health-related research. Public Health Rep. (1974), 1985) 100:126–31. doi: 10.2307/20056429
9. Carson, V, Lee, EY, Hewitt, L, Jennings, C, Hunter, S, Kuzik, N, et al. Systematic review of the relationships between physical activity and health indicators in the early years (0-4 years). BMC Public Health. (2017) 17:854. doi: 10.1186/s12889-017-4860-0
10. Guthold, R, Stevens, GA, Riley, LM, and Bull, FC. Global trends in insufficient physical activity among adolescents: a pooled analysis of 298 population-based surveys with 1·6 million participants. Lancet Child Adoles Health. (2020) 4:23–35. doi: 10.1016/S2352-4642(19)30323-2
11. Paterson, DC, Ramage, K, Moore, SA, Riazi, N, Tremblay, MS, and Faulkner, G. Exploring the impact of COVID-19 on the movement behaviors of children and youth: a scoping review of evidence after the first year. Sport Health Sci. (2021) 10:675–89. doi: 10.1016/j.jshs.2021.07.001
12. Dunton, GF, Do, B, and Wang, SD. Early effects of the COVID-19 pandemic on physical activity and sedentary behavior in children living in the U.S. BMC Public Health. (2020) 20:1351. doi: 10.1186/s12889-020-09429-3
13. Morrow Jr, JR, Jackson, AW, and Payne, VG. Physical activity promotion and school physical education In: President’s Council on Physical Fitness and Sports Research Digest (1999)
14. Jones, D, Innerd, A, Giles, EL, and Azevedo, LB. Association between fundamental motor skills and physical activity in the early years: a systematic review and meta-analysis. J Sport Health Sci. (2020) 9:542–52. doi: 10.1016/j.jshs.2020.03.001
15. Stodden, DF, Goodway, JD, Langendorfer, SJ, Roberton, MA, Rudisill, ME, Garcia, C, et al. A developmental perspective on the role of motor skill competence in physical activity: An emergent relationship. Quest. (2008) 60:290–306. doi: 10.1080/00336297.2008.10483582
16. Holfelder, B, and Schott, N. Relationship of fundamental movement skills and physical activity in children and adolescents: a systematic review. Psychol Sport Exerc. (2014) 15:382–91. doi: 10.1016/j.psychsport.2014.03.005
17. Figueroa, R, and An, R. Motor skill competence and physical activity in preschoolers: a review. Matern Child Health J. (2017) 21:136–46. doi: 10.1007/s10995-016-2102-1
18. Barnett, L, Webster, E, Hulteen, R, De Meester, AD, Valentini, N, Lenoir, M, et al. Correction to: through the looking glass: A systematic review of longitudinal evidence, providing new insight for motor competence and Health. Sports Med (Auckland, N.Z.). (2021) 52:921. doi: 10.1007/s40279-021-01563-1
19. Barnett, LM, Salmon, J, and Hesketh, KD. More active pre-school children have better motor competence at school starting age: an observational cohort study. BMC Public Health. (2016) 16:1068. doi: 10.1186/s12889-016-3742-1
20. Wick, K, Leeger-Aschmann, CS, Monn, ND, Radtke, T, Ott, LV, Rebholz, CE, et al. Interventions to promote fundamental movement skills in childcare and kindergarten: a systematic review and Meta-analysis. Sports Med. (2017) 47:2045–68. doi: 10.1007/s40279-017-0723-1
21. Ma, R, and Song, H. Effects of fundamental movement skill development on physical activity and health of children. Sports Science (in Chinese). (2017) 37:54–61+97. doi: 10.16469/j.css.201704007
22. Jones, RA, Riethmuller, A, Hesketh, K, et al. Promoting movement skill development and physical activity in early childhood settings: a pilot randomised controlled trial. Obes Res Clin Pract. (2010) 4:S17–7. doi: 10.1016/j.orcp.2010.09.034
23. Ali, A, Mclachlan, C, Mugridge, O, et al. The effect of a 10-week physical activity Programme on fundamental movement skills in 3–4-year-old children within early childhood education Centres. Children. (2021) 8:440. doi: 10.3390/children8060440
24. Hu, X, Jiang, GP, Ji, ZQ, Pang, B, and Liu, J. Effect of novel rhythmic physical activities on fundamental movement skills in 3- to 5-year-old children. Biomed Res Int. (2020) 2020:1–10. doi: 10.1155/2020/8861379
25. Moghaddaszadeh, A, and Belcastro, AN. Guided active play promotes physical activity and improves fundamental motor skills for school-aged children. J Sports Sci Med. (2021) 20:86–93. doi: 10.52082/jssm.2021.86
26. Yang, Q. Strategies of movement education for the test of gross motor development in preschool children. J Xi’an Inst Phys Educ. (2017) 34:341–7. doi: 10.16063/j.cnki.issn1001-747x.2017.03.014
27. Zhang, Q. Experimental study on the effects of moderate and high intensity physical activity at different time on basic motor skills and physical health level of U6-7 Gannan Normal University (2021). doi: 10.27685/d.cnki.ggnsf.2021.000349
28. Eyre, ELJ, Clark, CCT, Tallis, J, Hodson, D, Lowton-Smith, S, Nelson, C, et al. The effects of combined movement and storytelling intervention on motor skills in south Asian and white children aged 5–6 years living in the United Kingdom. Int J Environ Res Public Health. (2020) 17:3391. doi: 10.3390/ijerph17103391
29. Magistro, D, Cooper, SB, Carlevaro, F, Marchetti, I, Magno, F, Bardaglio, G, et al. Two years of physically active mathematics lessons enhance cognitive function and gross motor skills in primary school children. Psychol Sport Exerc. (2022) 63:102254. doi: 10.1016/j.psychsport.2022.102254
30. Xiao, K. An empirical study on the effect of 8-week exercise intervention on the development of fundamental motor skills of 3 to 6 years old children (in Chinese). Nanjing Sport Institute (2022). doi: 10.27247/d.cnki.gnjtc.2022.000094
31. Lu, W. An experimental study on the effect of infant football on positive mental quality and physical health of preschool children from 5 to 6Years old. East China Normal University, (2022). doi: 10.27149/d.cnki.ghdsu.2022.001367
32. Martin, R, and Murtagh, EM. An intervention to improve the physical activity levels of children: design and rationale of the ‘Active Classrooms’ cluster randomised controlled trial. Contemp Clin Trials. (2015) 41:180–91. doi: 10.1016/j.cct.2015.01.019
33. Routen, AC, Biddle, SJ, Bodicoat, DH, Cale, L, Clemes, S, Edwardson, CL, et al. Study design and protocol for a mixed methods evaluation of an intervention to reduce and break up sitting time in primary school classrooms in the UK: the CLASS PAL (physically active learning) Programme. BMJ Open. (2017) 7:e019428. doi: 10.1136//bmjopen-2017-019428
34. Page, MJ, McKenzie, JE, Bossuyt, PM, Boutron, I, Hoffmann, TC, Mulrow, CD, et al. The PRISMA 2020 statement: an updated guideline for reporting systematic reviews. Br Med J. (2021) 372:n71. doi: 10.1136/bmj.n71
35. Higgins, JPT, Li, T, and Deeks, JJ. Chapter 6: choosing effect measures and computing estimates of effect In: JPT Higgins, J Thomas, J Chandler, M Cumpston, T Li, and MJ Page, editors. Cochrane handbook for systematic reviews of interventions version 6.1 (updated September 2020). Bristol UK: Cochrane (2020). 44–59.
36. Higgins, P, and Green, S. Cochrane handbook for systematic reviews on interventions 5.3.3 user guide. (2014). Available at: www.cochrane-handbook.org
37. The Nordic Cochrane Centre. Review manager; cochrane collaboration. London, UK: The Nordic Cochrane Centre (2014). 1–43.
38. Deeks, JJ, Higgins, J, and Altman, DG. Analysing data and undertaking meta-analyses. Cochrane handbook for systematic reviews of interventions: Cochrane book series. USA: John Wiley & sons 20080:243–96.20.
39. Yajnik, CS, Lubree, HG, Rege, SS, Naik, SS, Deshpande, JA, Deshpande, SS, et al. Adiposity and hyperinsulinemia in Indians are present at birth. J Clin Endocrinol Metab. (2002) 87:5575–80. doi: 10.1210/jc.2002-020434
40. Li, B, Liu, J, and Ying, B. Physical education interventions improve the fundamental movement skills in kindergarten: a systematic review and meta-analysis. Food Sci Technol. (2021) 42:e46721. doi: 10.1590/fst.46721
41. Yuxin, Y, Xiaofen, L, and Wanxu, L. Effects of early childhood physical activity program on gross motor development in preschool children: a meta-analysis. Chin J Evid Based Med. (2023) 23:319–26.
42. He, L, and Sheng, Q. Physical Activity, Sedentary behaviour and sleep advice for the children of less than 5 years old. Digit Educ (in Chinese). (2020) 6:84–92.
43. Gao, W, and Wang, H. Meta analysis of the effect of physical activity intervention on physical fitness in Chinese children aged 3-6 years. China School Health. (2021) 42:1311–1317+1322. doi: 10.16835/j.cnki.1000-9817.2021.09.009
Keywords: children, fundamental movement skills, physical activity programs, locomotion skill, object control ability, stability, meta-analysis
Citation: Yang Y, Mao X, Li W, Wang B and Fan L (2025) A meta-analysis of the effect of physical activity programs on fundamental movement skills in 3–7-year-old children. Front. Public Health. 12:1489141. doi: 10.3389/fpubh.2024.1489141
Received: 31 August 2024; Accepted: 17 December 2024;
Published: 07 January 2025.
Edited by:
Ana Filipa Silva, Instituto Politécnico de Viana do Castelo, PortugalReviewed by:
Adela Badau, Transilvania University of Brașov, RomaniaCopyright © 2025 Yang, Mao, Li, Wang and Fan. This is an open-access article distributed under the terms of the Creative Commons Attribution License (CC BY). The use, distribution or reproduction in other forums is permitted, provided the original author(s) and the copyright owner(s) are credited and that the original publication in this journal is cited, in accordance with accepted academic practice. No use, distribution or reproduction is permitted which does not comply with these terms.
*Correspondence: Lixia Fan, ZmFubGl4aWExODlAMTYzLmNvbQ==
Disclaimer: All claims expressed in this article are solely those of the authors and do not necessarily represent those of their affiliated organizations, or those of the publisher, the editors and the reviewers. Any product that may be evaluated in this article or claim that may be made by its manufacturer is not guaranteed or endorsed by the publisher.
Research integrity at Frontiers
Learn more about the work of our research integrity team to safeguard the quality of each article we publish.