- 1Center for Food Animal Health, The Ohio State University, Columbus, OH, United States
- 2Global One Health Initiative, The Ohio State University, Addis Ababa, Ethiopia
- 3Haramaya University, Dire Dawa, Ethiopia
- 4University of Florida, Gainesville, FL, United States
- 5Emory University, Atlanta, GA, United States
- 6University of Massachusetts, Amherst, MA, United States
- 7College of Veterinary Medicine, University of Illinois, Champaign, IL, United States
Introduction: Enteric pathogens are a leading causes of diarrheal deaths in low-and middle-income countries. The Exposure Assessment of Campylobacter Infections in Rural Ethiopia (EXCAM) project, aims to identify potential sources of bacteria in the genus Campylobacter and, more generally, fecal contamination of infants during the first 1.5 years of life using Escherichia coli as indicator.
Methods: A total of 1,310 samples (i.e., hand rinses from the infant, sibling and mother, drinking and bathing water, food and fomite provided to or touched by the infants, areola swabs, breast milk and soil) were collected from 76 households between May 2021 and June 2022. Samples were assigned to two groups by infant age: TP1 (time point 1), infants between 4 and 8 months of age, and TP2, infants between 11 and 15 months of age. Fluorometric and semi-selective colorimetric approaches were used to quantify E. coli in the field samples.
Results: Overall, E. coli was ubiquitous within selected households (56.8% across the study). E. coli was more frequently detected than average (>53%) with high concentration (>2-log CFU) in soil (g) and per pair of hand, while the opposite trend (<33%; <1.5-log CFU) was observed in food provided to the infants (g or mL), per areola, and breast milk (mL; p < 0.01). E. coli was frequently detected in fomites touched by the infants, drinking and bathing water (>51%), but at low concentration (<1.5-log CFU). Correlation analysis between E. coli concentration in different sample types suggested that the mother’s hands and fomites might play a key role in the transmission of E. coli to the infants (p < 0.01; r2 > 0.3).
Discussion: Using E. coli as surrogate, our study identified mother (hands and areola) as reservoirs likely to be involved in frequent transmission of fecal contaminants to infants within rural Ethiopian households.
Introduction
Many infectious diseases can affect the human gastrointestinal tract, with enteric pathogens posing a significant health risk. Enteric infections often impair the gut’s intestinal integrity and function, leading to immediate adverse health consequences (e.g., diarrhea) and long-term chronic gastrointestinal issues (e.g., irritable bowel syndrome [IBS], inflammatory bowel disease [IBD], and chronic diarrhea) (1). When combined with nutritional deficits during infancy, chronic exposure to enteric pathogens is linked to prolonged detrimental health conditions such as Environmental Enteric Dysfunction (EED) and stunting (2). Conditions caused by EED are largely irreversible and lead to poor long-term health outcomes (e.g., diminished cognitive and physical development, weakened immune systems, and an increased risk of degenerative diseases later in life), especially when detected in young infants (<2-year-old) (3). Enteric infections are also the primary causes of diarrhea, which is a leading cause of mortality and morbidity among children under five years old worldwide. These infections are particularly prevalent in resource-limited settings, due to limited access to clean water, inadequate sanitation, and poor personal hygiene practices. In 2017, diarrheal diseases were responsible for 1.6 million deaths globally, with the highest mortality rates primarily in Sub-Saharan Africa (4, 5). Despite developmental efforts in these regions, access to clean water and proper sanitation management/infrastructure is precarious. Yet the evidence between enteropathogenic infections and related gastrointestinal disease is alarming, warranting global health interventions. This concern ultimately led the United Nations to include Clean Water and Sanitation as a Sustainable Development Goals (SDG-6) (6).
Climate change, food security, poor infrastructures, and inadequate water, sanitation, and hygiene (WASH) practices, contribute to a high disease burden in low and-middle-income countries (LMIC). Open defecation is a practice commonly performed by Ethiopian households and can lead to fecal-oral diseases such as diarrhea (7–9). Global studies such as Etiology, Risk Factors, and Interactions of Enteric Infections and Malnutrition and the Consequences for Child Health and Development (MAL-ED) (10), WASH Benefits (11), and Sanitation Hygiene Infant Nutrition Efficacy (SHINE) (12) studies have highlighted the intricate interplay between these factors and their impact on health outcomes. These complex relationships present a significant challenge for low and-middle-income countries (LMIC) due to limited resources and inherent vulnerabilities to adapt to dramatic climatic shifts. Similar trends were observed in 2018 when our team conducted a cross-sectional study in rural Eastern Ethiopia as part of the CAGED project (Campylobacter Genomics and Environmental Enteric Dysfunction), during which WASH-oriented questionnaires revealed that only 23% of households used improved sanitation facilities shared between households and roughly 80% of households practiced open defecation (13). In addition, most of the study population did not have access to clean water. Only 2% reported having access to a safely managed drinking water source on premises, available when needed, and not contaminated by fecal and chemical pollutants (13). Diarrhea is often the most common symptom associated with a gastrointestinal infection, frequently characterized by a concentration of enteric pathogens disrupting the normal flora within the gut. Enteropathogenic microorganisms stem across different microbial groups, including bacteria (enteropathogenic or enterotoxigenic E. coli, Shigella spp., Salmonella spp., and Vibrio cholerae), parasites (such as Cryptosporidium spp. Giardia, and Entamoeba histolytica) and viruses (such as rotavirus, norovirus, astrovirus, and adenovirus). However, pinpointing the enteric pathogens in the environment can be complex due to their presence in low concentration. Therefore, surrogate indicators like coliforms and Escherichia coli are commonly used to monitor fecal contamination in water and environmental samples (14).
Escherichia coli, a member of the Enterobacteriaceae family, is a crucial fecal indicator organism widely used for its reliability in signaling potential fecal contamination. Its significance lies in its ubiquity in the intestines of warm-blooded animals, making it an accurate proxy for fecal matter (15, 16). Moreover, detection methods for E. coli are relatively quick, cost-effective, and straightforward, making it an indispensable tool for routine water quality and safety assessments, especially in resource-limited settings where enteric infections are common (17, 18). By focusing on E. coli, one can efficiently assess fecal contamination levels, and thus, identify potential fecal exposure pathways.
In this study, we hypothesized that the detection and quantification of E. coli in household environments serve as a reliable indicator of fecal contamination levels and potential fecal transmission pathways of enteric pathogens. More precisely, the detection and quantification of E. coli was conducted in a broad range of human (i.e., hand rinse, areola swabs, and breast milk) and environmental samples (i.e., drinking water, bathing water, food given to the infants, fomites touched by the infants and soil within the households). This approach enabled to assess the degree of fecal contamination in the infants’ surrounding and pinpoint potential fecal transmission pathways. This study is part of the Exposure Assessment of Campylobacter Infections in Rural Ethiopia (EXCAM) project, which aims to systematically evaluate infants’ exposure to Campylobacter and fecal contamination in rural districts of Haramaya, Eastern Ethiopia. These environments often lack the infrastructure for direct testing of Campylobacter, making E. coli an efficient and convenient marker for evaluating broader public health risks. Thus, by focusing on E. coli, we not only assess the extent of fecal contamination, but also, build a foundation for future study to understand the transmission dynamics of Campylobacter, providing actionable insights into improving infant health outcomes.”
Materials and methods
Study area
The EXCAM study, nested within the CAGED project in the Eastern rural districts of Ethiopia (13, 19–21), recruited newborns from 79 households across 10 kebeles. The EXCAM study was based on similar methodology used in the SaniPath study (22). Additional details about the study area are previously described (13, 19–21).
Sample collection (time points and sample size)
Samples were collected during two distinct time windows: first, when infants were between 4 and 8 months of age (Time Point [TP] 1, between May 2021 and December 2021), and second, when they were between 11 and 15 months of age (TP 2, between December 2021 and June 2022). This approach allowed for the analysis of how behavior-mediated exposures may change with age during critical developmental stages. Initially, 79 infants were included in TP1, decreasing to 76 in TP2 due to dropouts. Among the 76 infants who completed this study, 41 (54%) were male and 35 (46%) were female.
A total of 1,310 samples were collected across surveyed households between May 2021 and June 2022. During TP1, 586; samples were collected from infants under 8 months of age, while in TP2, 724 samples were collected from infants when they reached 11 to 15 months. Between 101 and 265 samples were collected by sample type during this study, with an average of 17 samples per household and 131 samples per kebele. Breast milk samples were mostly collected at TP1 because most mothers stopped giving breast milk when the infants were above 11 months of age. Soil samples collected inside the households were only collected at TP2 (n = 57) because the infants motility increase after 11 months of age, and thus, allowing them to move around. Across the two periods, a total of 429 hand rinses, 117 breast milk samples, 149 areola swabs from the mother, 148 drinking water, 44 bathing water, 101 foods consumed by the infants, 265 fomites and 155 soil samples were collected. A total of 916 and 394 samples were collected during the dry season (between October and May) and rainy season (between June and September), respectively.
Collection of human-related samples
Three hand rinse samples (infant, sibling, and mother) per household per time point were collected by washing the hands of the surveyed individuals into a 1 L plastic bag prefilled with 200 mL of sterile distilled water. Hands were washed using normal hand-to-hand frictions for 30 s inside the bag.
Areola swabs were collected using cotton swabs (Sterile Cotton Tipped Applicators, Puritan Medical Products; Guilford, ME) humified in sterile water. The humified swab was applied by the mother directly to the skin, rotating it in a circular pattern on both areolas. The inoculated swab was transferred to a 15 mL tube prefilled with 4 mL Letheen broth (Difco BD; Becton, NY).
Once the areola swab was collected, the areolas were cleaned with 70% ethanol to remove potential surface contaminants before collecting the breast milk. The breast milk samples were manually collected by the mother by applying pressure with her hand. The breast milk (at least 1 mL per mother per time point) was collected into a sterile 60 mL specimen collection cup (Starplex Scientific; Etobicoke, Canada). It should be noted that the mothers might not always have properly sanitized their hands before milk collection, potentially introducing contaminants into the milk.
Collection of environmental samples
Foods (at least 10 g per household per time point) consumed by the surveyed infants was collected in a sterile 60 mL screw-capped container (Starplex Scientific; Etobicoke, Canada) using the utensil (e.g., spoon, fingers) commonly used to manipulate the food and feed the infant.
Surfaces (aka. fomites) the most frequently touched by the surveyed infants were swabbed using a Speci-sponge (Nasco WHIRL-PAK; Boston, MA) pre-humified with 10 mL of sterile water. The selection of three mostly touched fomites by the infant of the designated households was based on the total area touched by the infant (e.g., floor, carpet, or phone). Behavioral data related to these fomites for the surveyed infants are detailed in a companion paper (39, in preparation). The designated area was swabbed by moving the humected Speci-sponge back and forth at least five times on both sides. The inoculated sponge was transferred back into its original collection bag.
The detailed procedure used for the collection of drinking and bathing water and soil samples is described in our previously published studies (21). All samples described above were stored and transported on ice to the lab for processing. Phosphate buffered saline solution or equivalent could not be used to collect the field samples (i.e., hand rinse, areola swab and fomites) instead of sterile water because the surveyed households did not believe the buffer was healthy to be touched, especially for the infant.
Sample processing
Hand rinse, drinking, and bathing water samples were designated as large, diluted samples, and thus, concentrated using 500 mL Disposable 0.22 μm Nylon Vacuum Filter (Corning; Corning, NY). The membrane was aseptically removed and cut into 5 mm2 pieces using sterile blade and forceps. The cut membrane was transferred into a 5 mL tube pre-filled with 4 mL of 1X peptone water (pH 7) and twenty 3-mm diameter glass beads. The sample was manually homogenized for at least 1 min to release cells from the nylon membrane. The supernatant was transferred into another 5 mL tube and centrifuged for 10 min at 4,500 rpm. The pellets were concentrated into 1 mL of 1X peptone water (pH 7).
Soil samples (boot socks), fomites, and areola swabs were identified as dry samples, and thus, resuspended into 25, 10, and 3 mL of 1X peptone water (pH 7), respectively. Resuspended samples were homogenized for 1 min by hand for bags or using a vortex for tubes. The liquid obtained from the homogenization was transferred into 50 mL tubes and centrifuged for 10 min at 4,500 rpm. The pellets were carefully concentrated into 1 mL of 1X peptone water (pH 7). Liquid food samples (e.g., cow milk and mango juice) and breast milk samples were used as is. One gram of solid food samples (e.g., biscuit, rice, spaghetti, macaroni and injera) was manually homogenized for 30 s into a 100 mL Whirl-Pak bag (Seattle, WA) filled with 9 mL of 1X peptone water (pH 7). The homogenized samples were centrifuged for 10 min at 4,500 rpm and the pellet were resuspended into 1X peptone water (pH 7; 5 mL final volume).
Detection and quantification of Escherichia coli in clear and translucent field samples using EC-MUG fluorometric approach
The goal of this study was to develop an easy, low-cost, and high-throughput tests that could be applied on clear/translucent samples (e.g., drinking and bathing water, hand rinse, fomites touched by the infants and areola swabs) or opaque samples (e.g., food provided to the infants, milk and soil collected from the households). The prevalence and concentration of E. coli reported in this study were determined based on standardized selective colorimetric Chromocult plating (23, 24) and fluorometric EC-MUG approaches (25, 26). Thereby, the results indicated the total E. coli population but do not differentiate between commensal and pathogenic E. coli. Further, we acknowledge that the prevalence of E. coli in the drinking water might have been underestimated due to the limited sample volume processed in this study. The E. coli concentration in clear (e.g., drinking and bathing water) and translucent (e.g., hand rinse and fomites) samples was estimated using the modified MPN 96-well plate assay combined with EC-MUG fluorometric approach. Based on the total volume available per sample’s type, a total of 15.84 mL of sample (n = 88 reactions; 180 μL of the designated sample per well = one reaction) was tested from hand rinse, drinking and bathing water samples (also called “large liquid samples”), while 0.9 mL of concentrated samples (n = 5 reactions; 180 μL of the designated sample per well = one reaction) were tested from fomite, areola swab and food samples (also called “small liquid samples”). For both “large and small liquid samples,” 1.62 mL of EC-MUG broth was added into each well of a 96-deep well plate (VWR; Radnor, PA). In addition, four wells were inoculated with 180 μL of sterile 1X peptone water (pH 7; negative controls) or four wells were inoculated with 180 μL of an overnight E. coli culture known to produce fluorescence (positive controls). The inoculated plate was sealed using adhesive plate seal (Thermo Scientific; Waltham, MA) and incubated at 37°C for 48 h. After incubation, 200 μL of incubated product was transferred into a transparent 96-well plate (VWR; Radnor, PA) and expose to UV light to enumerate number of fluorescence wells (indicating the presence of E. coli). In parallel, the field samples were serially 10-fold diluted up to 8 times in EC-MUG broth in case if the E. coli concentration was too high to be quantified using the first approach. For the analysis of the data, we hypothesized that one positive well harbors at least one viable E. coli CFU.
Detection and quantification of Escherichia coli population in turbid/opaque field samples using Chromocult colorimetric approach
Opaque samples (Breast milk, soil and opaque food samples) were 10-fold serial diluted in 1X peptone water (pH 7). Two hundred microliters of undiluted and diluted samples were plated on Chromocult® Coliform agar (Sigma Aldrich Millipore; Saint-Louis, MO). The plates were incubated at 37°C for up to 48 h. Colonies with purple color (characteristic of E. coli) were recorded.
Statistical analysis
Escherichia coli concentration data were log-transformed. Statistical analyses were performed using JMP PRO 16 software (SAS Institute; Cary, NC, USA) and R v4.2.1.1 The normality of the E. coli concentration data was assessed using Goodness of fit combined with Shapiro test. Chi2 and Wilcoxson tests were used to identify differences in E. coli prevalence and concentration, respectively based on the recorded metadata (e.g., kebeles, infant sex, samples types, and collection dates). A p-value of 0.05 was used at the cut-off for the above statistical tests. A multivariate analysis (Pearson correlation and linear regression reported for each pairwise comparison axis) was used to identify correlation between the concentration of E. coli and sample types for the designated time points. A p-value of 0.01 was used as the cut-off.
Results
Prevalence of Escherichia coli in the Ethiopian households
Overall, E. coli was detected in 58% (745/1,310) of all the samples, and no significant differences in E. coli prevalence were detected between TP1 (58% [n = 341/586; CI95% = 54–62%]) and TP2 (56% [n = 404/724; CI95% = 52–59%]; p > 0.05; Table 1). No difference in E. coli prevalence was detected across the 10 kebeles surveyed in this study (58% [CI95% = 54–62%] and 56% [CI95% = 52–59%] at TP1 and TP2, respectively; Table 1).
Escherichia coli prevalence was divided into two distinct groups based on the sample types studied (e.g., prevalence below 33% versus higher than 53%; p < 0.001; Figure 1A). Specifically, E. coli was more frequently detected in drinking water, bathing water, fomites touched by the infants, mother hand rinse, sibling hand rinse, infant hand rinse samples, and soil samples collected inside the households for both time points compared to food eaten by the infants, areola swabs and breast milk samples (Figure 1A).
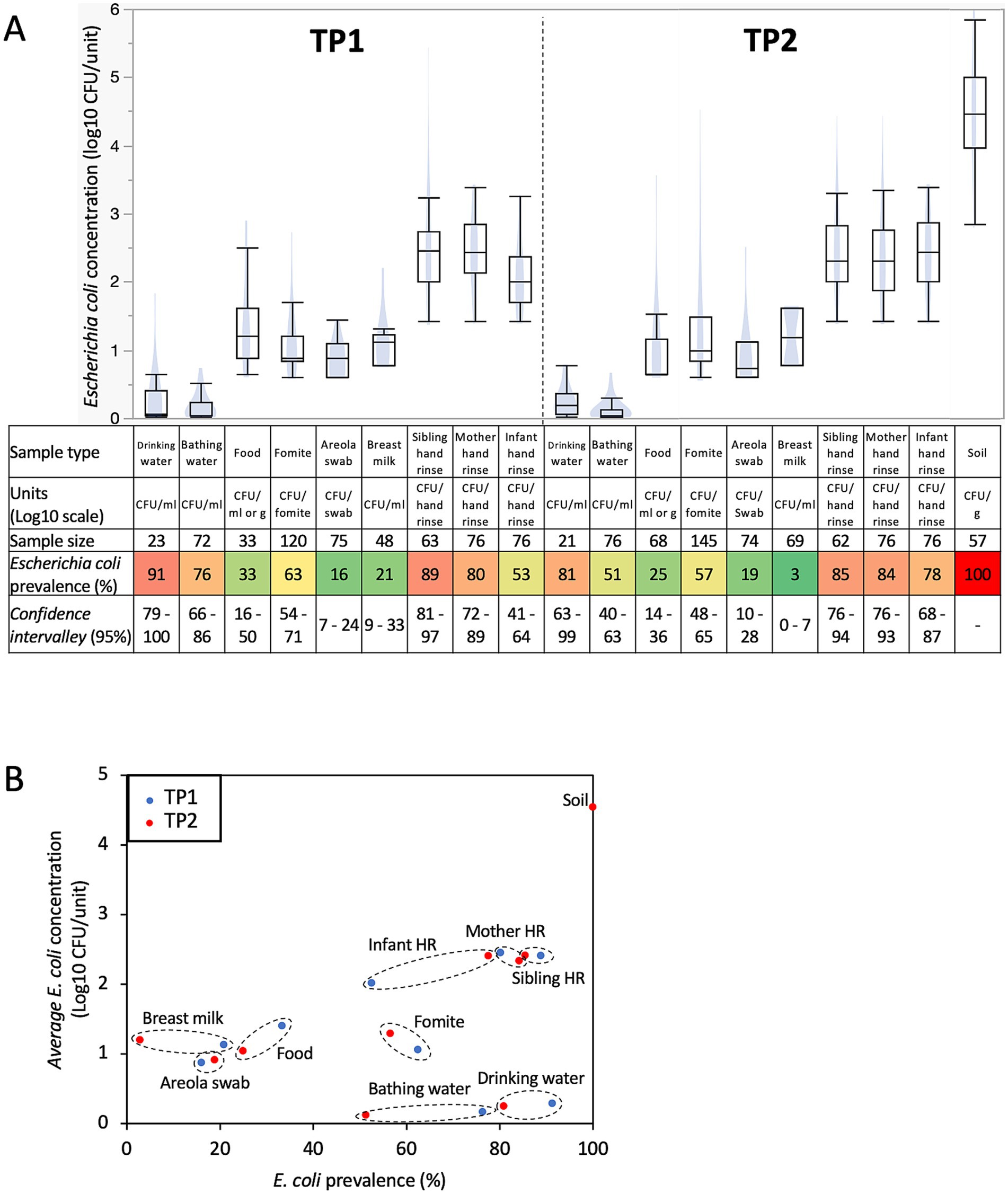
Figure 1. Prevalence and concentration of Escherichia coli in samples collected within 76 Ethiopian households between May 2021 and June 2022. (A) Boxplots of E. coli concentration and table showing E. coli prevalence by sample type and time points. Concentration data were determined only based on the samples positive for E. coli. Blue shadows within the boxplots represents the density ellipse (graphical representation that can show data extent, center of mass, least squares fit line and outliers) obtained based on the E. coli concentration data for the designated sample type and time point. HR, hand rinse. The color of the cells in the table are proportional to the E. coli prevalence data (e.g., red means high prevalence and green means low prevalence). (B) Comparison between E. coli prevalence and concentration across all the samples collected in this study. Samples from TP1 (red dots; n = 586; between May 2021 and December 2021) were collected when the infants were under 8 months of age, while the samples from TP2 (blue dots; n = 724; between December 2021 and June 2022) were collected when the infants between 11 and 15 months of age. Dotted circles cluster data (E. coli prevalence and concentration) obtained for similar sample type at TP1 and TP2.
Escherichia coli prevalence decreased across most sample types at TP2 compared to TP1 (c.a., 12% ±8 decrease; especially in bathing and drinking water, and breast milk; p < 0.05; Figure 1A), while the opposite trend was observed with the infant hand rinse (c.a., 25% increase; p < 0.002). Furthermore, variations in E. coli prevalence were also observed based on the date of sample collection and the age of the infants when the samples were collected. The prevalence of E. coli in the infant hand rinse significantly increased based on the infants’ age at sampling (r2 = 0.2; p = 0.006). These results were not affected by the sex of the infant (86 and 88% of the hand rinse samples collected from male and female infants were positive for E. coli, respectively). Other sample types also displayed equivalent E. coli prevalence between sex (p > 0.05). On the other hand, the opposite trend was observed with drinking water and breast milk samples. The prevalence of E. coli in the drinking water and breast milk significantly decreased over time (r2 = −0.2 and-0.3, respectively; p < 0.004) and significantly decreased based on the age of the infant when the samples were collected (r2 = −0.2 and − 0.3, respectively; p < 0.006).
No differences in E. coli prevalence were detected between the food types provided to the infants (e.g., biscuit soaked in water, cow milk, injera, Fanta soda, macaroni, sliced mango, and boiled rice and corn) and the fomites touched by the infants (e.g., bottle used to feed the infant, mobile phone, plastic bag, and teacups). However, it is worth noting that the sample size of the mentioned food items was small, which affected the power of the statistical analysis. E. coli was the most frequently detected in food items consumed by the infants (i.e., n = 3/6 cooked rice, 2/5 macaroni, 4/9 cow milk and 10/45 injera) and fomites touched by the infants (i.e., n = 5/7 mobile phone and 6/15 tea cup).
Concentration of Escherichia coli in the Ethiopian households
The concentration of E. coli discussed in this section is based only on the E. coli positive samples. Overall, E. coli was detected in 745 samples processed in this study (n = 341 and 404 positive samples at TP1 and TP2, respectively). No difference in E. coli concentration was detected between kebeles and households for the sample types studied. On the other hand, the concentration of E. coli was separated into three and four distinct groups across the sample type at TP1 and TP2, respectively (Figure 1A). Drinking and bathing water harbored low level of E. coli (<0.3-log CFU/mL), followed by foods eaten by the infants, fomites touched by the infants, areola swabs and breast milk with a concentration of E. coli between 0.9-and 1.2-log CFU/mL, g or sample (i.e., areola swabs and fomites touched by the infants; Figure 1A). Interestingly, the hand rinse samples collected from the mother, sibling and infant harbored an average concentration in E. coli between 2.2- and 2.4-log CFU/pair of hands (Figure 1A). Finally, E. coli was the most abundant in the soil samples (4.5-Log CFU/g ± 0.1). As observed with the prevalence data, the E. coli population from the hand rinse collected from the infants was positively correlated with the date of collection (r2 = 0.3; p = 0.002) and the age of the infant when the samples were collected (r2 = 0.3; p = 0.001). Therefore, E. coli was significantly higher in the hand rinse collected from the infant at TP2 (2.4-log CFU/fomite ±0.1) compared to the ones collected at TP1 (2-log CFU/fomite ±0.1; p = 0.001; Figure 1A). These results were not affected by the sex of the infant. Overall, 2.4-log E. coli CFU/hand rinse were detected from both male and female infants hand rinse samples, respectively. Other sample types also displayed equivalent E. coli prevalence between sex (p > 0.05). E. coli concentration was also significantly higher in fomites touched by the infants collected at TP2 (1.3-log CFU/fomite ±0.1) compared to the ones collected at TP1 (1.1-log CFU/fomite ±0.1; p = 0.03; Figure 1A).
The concentration of E. coli was not always associated with the prevalence in the designated sample type (Figure 1B). High prevalence (>53%) and concentration of E. coli (>2-log CFU/sample) were detected in soil (gram) collected inside the households and the pair of hands. Low prevalence (<33%) and concentration of E. coli (<1.5-log CFU/sample) were detected in the foods (g or mL) eaten by the infants, areola and breast milk (mL; Figure 1B). On the other hand, E. coli was frequently detected in fomites touched by the infants, drinking and bathing water (mL; >51%), but at low concentration (<1.5-log CFU/sample; Figure 1B).
Correlations between Escherichia coli concentration in reservoirs in the Ethiopian households
Distinct correlations in E. coli concentration were observed between sample types at the two time points, TP1 (Figure 2A) and TP2 (Figure 2B). At TP1, when the infants were under 8 months of age, strong positive correlations in E. coli concentration were detected between the hand rinse from the mother and her children (infant hand rinse; r2 = 0.5; p = 0.0001; sibling hand rinse; r2 = 0.4; p = 0.002; green area in Figure 2A). The concentration of E. coli detected in the mothers’ and the infants’ hand rinses were positively correlated with concentration of E. coli in the areola swabs (r2 = 0.3 and 0.4, respectively; p < 0.009; pink area in Figure 2A).
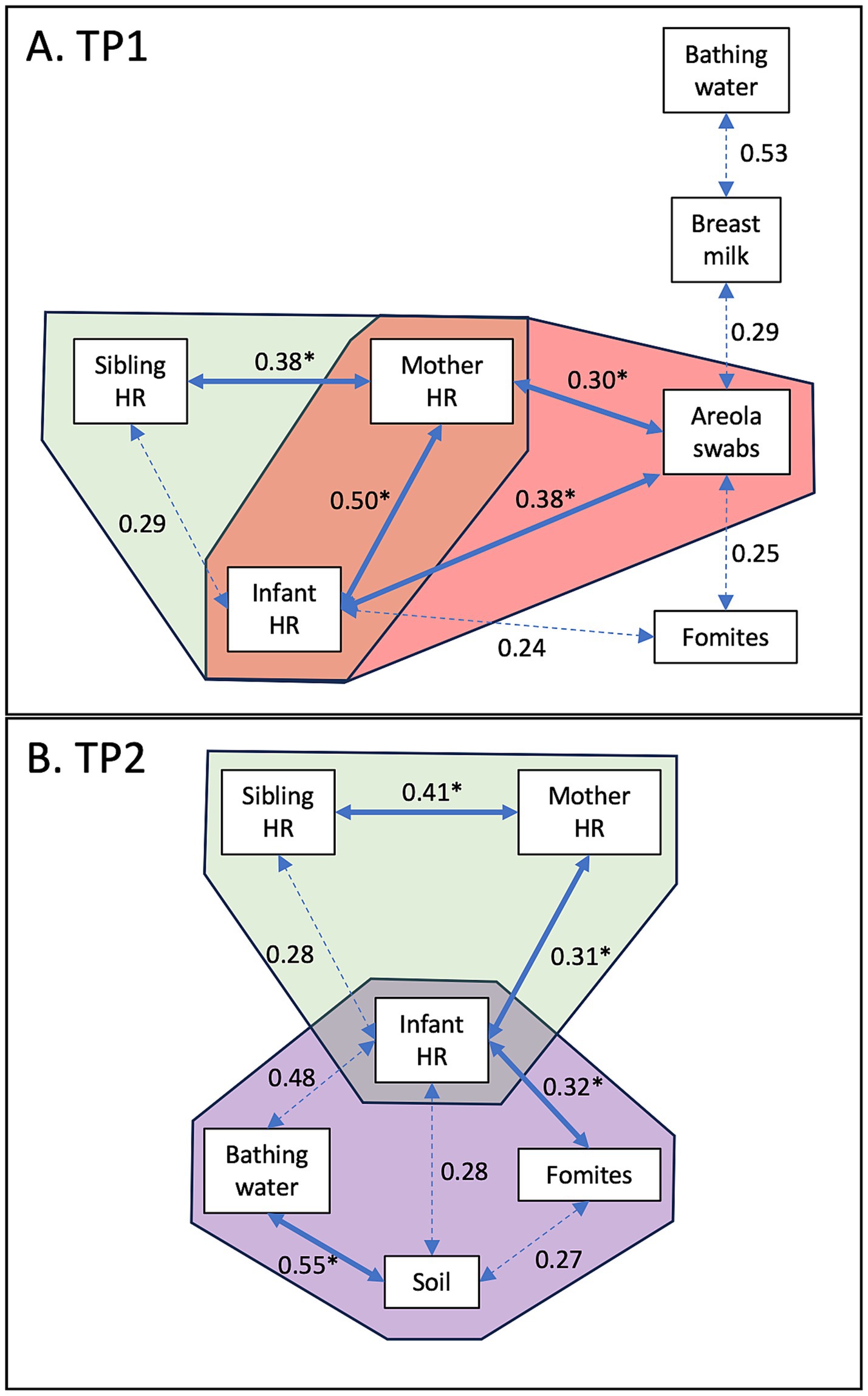
Figure 2. Correlation of E. coli concentrations between sample types. (A) Correlation detected at TP1 when the infants were under 8 months of age. (B) Correlation detected at TP2 when the infants were between 11 and 15 months of age. Values indicate the r2 values. The full arrows combined with the symbol “*” indicates that the p-value was lower than 0.01. The dotted arrows indicates that the p-value was lower than 0.05.
At TP2, when the infants were between 11 and 15 months of age, strong positive correlations in E. coli concentration were still detected between the hand rinse from the mother and her children (infant hand rinse; r2 = 0.3; p = 0.005; sibling hand rinse; r2 = 0.4; p = 0.008; green area in Figure 2A). The concentration of E. coli in the infants’ hand rinse was also positively correlated with the concentration of E. coli from the fomites touched by the infants (r2 = 0.3; p = 0.004; purple area in Figure 2B). The E. coli concentration in the soil samples collected inside the households was positively correlated with the bathing water (r2 = 0.5; p = 0.01; purple area in Figure 2B).
Discussion
The transmission of fecal contaminants within rural households poses a significant health concern (27, 28). Poor sanitation and inadequate hygiene practices can increase exposure to fecal pathogens, like diarrheagenic E. coli, Salmonella, and Campylobacter, leading to diarrheal diseases and other health complications, especially among vulnerable infants (29). This study analyzed commensal E. coli as an indicator of fecal contamination in human-related and environmental samples collected between May 2021 and June 2022 in 76 households of infants in rural Ethiopia. Overall, E. coli was detected in 56.8% of samples collected in this study, which is concordant with other studies conducted in developing countries (E. coli prevalence between 47–83%) (5, 30, 31). E. coli was detected in all sample types, especially in fomites and hand rinse samples collected from the household members (i.e., mother, sibling, and infant). These findings are in alignment with previous studies reporting that hands are a recurrent carrier of fecal contaminant due to improper sanitation after defecation or contact with a contaminated environment (32–35). E. coli was also frequently detected, albeit in lower concentration on fomites, drinking water and bathing water. E. coli concentration obtained in this study are equivalent to data reported from LMIC (e.g., Bangladesh, Uganda and Cambodia) (33). On the other hand, breast milk, areola swabs and food given to the infants were less likely to have E. coli.
The presence of E. coli within specific reservoirs and its potential movement between sample types significantly varied with age. E. coli was less frequently detected when the infants were under 8 months of age (53%) compared to the hand rinse samples collected when the infants were between 11 and 15 months of age (78%). This increase in E. coli prevalence may be linked to the infants’ increased autonomy and their greater interaction with their environment. In Ethiopia, it is customary for mothers and infants to stay indoors together for extended periods post-birth, which may facilitate transfer of E. coli as the mothers’ hand contamination was most correlated with E. coli levels in the infant hands during early age. Thereby, the daily routine that the mothers are engaging with the infants could lead to repeated exposures of the infant to fecal contaminants, especially during breast feeding and handling the infant, as reported by previous studies (36, 37). We previously showed that the enteric pathogen Campylobacter was highly prevalent in mothers’ stools (83.1%) collected from the same households (21). It was also found that the Campylobacter concentration in the mothers’ stools correlated with the Campylobacter concentration in the infants’ stools (21), which supports the transfer of fecal contaminants between mother and infant.
Marked changes were observed in potential E. coli transfer within the households between the first period (TP1) and the second period (TP2) of this study. As mentioned above, initially interactions observed in TP1 predominantly involved mothers (i.e., hand rinse and areola swabs) and the infants. When the infants were between 11 and 15 months of age, they are likely to maintain frequent contact with their mothers’ hands while increasingly interacting with other environmental reservoirs within the household (i.e., fomites, bathing water, and soil). This behavior aligns with the observed E. coli correlations detected between different sample types at TP2.
Similar observations were reported in our previous study conducted in the same region in eastern Ethiopia, where the prevalence and concentration of Campylobacter in the infants were closely correlated with the concentration of Campylobacter in the soil collected inside the households and presence of specific livestock (e.g., goat and sheep) (21). It should be noted that when the infants reach 11 months of age, breast feeding becomes less frequent and with increasing exposure to other foods (e.g., injera, rice and dry biscuit), which are less likely to have E. coli. On the other hand, given all the households selected in this study owned livestock (i.e., chicken, cow, goat and/or sheep), the infants were more likely to encounter particles rich in fecal contaminants (38).
As previously described in our recent published studies (20, 21), this study faced several limitations during its execution and sample collection, which significantly influenced the methodology used to detect and quantify E. coli. Due to limited resources and cultural norms, only a small volume of drinking water (i.e., 1 L per household per time point) could be collected from the selected households. Further, hesitation from some mothers to provide samples was encountered due to stigma, rumors, and cultural norms. Due to the sensitive nature of the operation, areola swabs and breast milk collections were performed privately by the mother. Thereby, the quality and number of samples collected was likely to fluctuate between households. Given the mothers’ hands harbored high level of E. coli, our study might have overestimated the prevalence of E. coli in the areola swabs and breast milk samples due to potential cross-contamination with the hands during the sample collection.
In conclusion, our study revealed a high prevalence and concentration of E. coli in the different reservoirs surrounding infants, indicating significant fecal contamination and potential for transmission of associated enteric pathogens (e.g., Campylobacter spp., pathogenic E. coli, rotavirus, and Salmonella enterica) within rural Ethiopian households. Future studies will assess whether the prevalence and concentration of E. coli could be used as bioindicator for other enteric pathogens (i.e., Campylobacter) in these low-income resource settings. Furthermore, the interconnections detected between E. coli reservoirs highlighted different routes that could lead to transmission of fecal contaminants within the households, and thus, could potentially lead to early infection of the infants with enteric pathogens. Statistical models incorporating the E. coli data with the behavioral data during this study will be developed to determine whether reservoirs less likely to harbor E. coli or at low concentration still have a predominant risk for the transmission of E. coli and other fecal contaminants to the infants (Wang et al., in preparation).
Data availability statement
The raw data supporting the conclusions of this article will be made available by the authors, without undue reservation.
Ethics statement
The studies involving humans were approved by the University of Florida Internal Review Board (IRB201903141); the Haramaya University Institutional Health Research Ethics Committee (COHMS/1010/3796/20) and the Ethiopia National Research Ethics Review Committee (SM/14.1/1059/20). Written informed consent is obtained from all participating households (husband and wife) using a form in the local language (Afaan Oromo). Research findings will be disseminated to community stakeholders, including participants, through the existing CAB. Findings will be disseminated to scientific, academic, policy and development stakeholders through conferences and peer-reviewed journals and through the Feed the Future Innovation Lab for Livestock Systems. The Bill and Melinda Gates Foundation, the funder of this trial, requires an open access data policy. Therefore, all manuscripts from this funded work will be open access with the data underlying the published research results available in a public repository. The website https://www.gatesfoundation.org/how-we-work/generalinformation/open-access-policy provides more information on this policy. A Community Advisory Board including a representative of the community, religious leaders (imam), woreda and kebele administration, woreda women and children affairs, woreda bureau of health and agriculture, kebele health, and agricultural extension workers was established to guide the research team for better understanding of local context and entry to the community and is regularly engaged in the research (20). Only the project manager at Haramaya University and the data manager at the University of Florida have access to personally identifiable information in the REDCap database. Any data shared among researchers within the project was identified and blinded. Materials and Data Transfer Agreements assure confidentiality of data when exchanged with international partners and others. The studies were conducted in accordance with the local legislation and institutional requirements. Written informed consent for participation in this study was provided by the participants’ legal guardians/next of kin. Written informed consent was obtained from the minor(s)’ legal guardian/next of kin for the publication of any potentially identifiable images or data included in this article.
Author contributions
LD: Conceptualization, Data curation, Formal analysis, Methodology, Supervision, Validation, Visualization, Writing – original draft, Writing – review & editing. BA: Data curation, Methodology, Validation, Visualization, Writing – original draft, Writing – review & editing. AO: Data curation, Formal analysis, Methodology, Supervision, Visualization, Writing – original draft, Writing – review & editing. BM: Data curation, Supervision, Validation, Writing – review & editing. YW: Formal analysis, Methodology, Writing – review & editing. YM: Methodology, Validation, Writing – review & editing. YD: Data curation, Methodology, Writing – review & editing. KH: Data curation, Methodology, Writing – review & editing. MB: Data curation, Methodology, Writing – review & editing. SM: Conceptualization, Funding acquisition, Investigation, Methodology, Supervision, Writing – review & editing. AH: Conceptualization, Funding acquisition, Investigation, Methodology, Project administration, Resources, Supervision, Writing – review & editing. SL: Conceptualization, Funding acquisition, Investigation, Methodology, Project administration, Resources, Supervision, Writing – review & editing. GR: Conceptualization, Funding acquisition, Investigation, Methodology, Project administration, Resources, Supervision, Validation, Visualization, Writing – review & editing.
Funding
The author(s) declare that financial support was received for the research, authorship, and/or publication of this article. This project was funded by the United States Agency for International Development Bureau for Food Security under Agreement #AID-OAA-L-15-00003 as part of Feed the Future Innovation Lab for Livestock Systems, and by the Bill & Melinda Gates Foundation OPP#1175487. Under the grant conditions of the Foundation, a Creative Commons Attribution 4.0 Generic License has already been assigned to the Author Accepted Manuscript version that might arise from this submission. Any opinions, findings, conclusions or recommendations expressed here are those of the authors alone. Research reported in this publication was supported by the University of Florida Clinical and Translational Science Institute, which was supported in part by the NIH National Center for Advancing Translational Sciences under award number UL1TR001427.
Acknowledgments
We thank the local community advisory board for facilitating this research in different selected kebeles. The EXCAM project is funded by the Bill & Melinda Gates Foundation to address food insecurity issues in Ethiopia and Burkina Faso through the project Equip - Strengthening Smallholder Livestock Systems for the Future (grant number OPP11755487). These funds are administered by the Feed the Future Innovation Lab for Livestock Systems, which was established by funding from the United States Agency for International Development (USAID) and is co-led by the University of Florida’s Institute of Food and Agricultural Sciences and the International Livestock Research Institute. Support for the Feed the Future Innovation Lab for Livestock Systems is made possible by the generous support of the American people through USAID. The contents are the responsibility of the authors and do not necessarily reflect the views of USAID or the United States Government. REDCap is hosted at the University of Florida Clinical and Translational Science Institute (CTSI), supported by NIH National Center for Advancing Translational Sciences grant UL1 TR000064.
Conflict of interest
The authors declare that the research was conducted in the absence of any commercial or financial relationships that could be construed as a potential conflict of interest.
Publisher’s note
All claims expressed in this article are solely those of the authors and do not necessarily represent those of their affiliated organizations, or those of the publisher, the editors and the reviewers. Any product that may be evaluated in this article, or claim that may be made by its manufacturer, is not guaranteed or endorsed by the publisher.
Footnotes
References
1. Hentschel, V, Arnold, F, Seufferlein, T, Azoitei, N, Kleger, A, and Müller, M. Enteropathogenic infections: organoids go bacterial. Stem Cells Int. (2021) 2021:e8847804:1–14. doi: 10.1155/2021/8847804
2. Chen, D, Mechlowitz, K, Li, X, Schaefer, N, Havelaar, AH, and McKune, SL. Benefits and risks of smallholder livestock production on child nutrition in low-and middle-income countries. Front Nutr. (2021) 8:751686. doi: 10.3389/fnut.2021.751686
3. Soliman, A, De Sanctis, V, Alaaraj, N, Ahmed, S, Alyafei, F, Hamed, N, et al. Early and long-term consequences of nutritional stunting: from childhood to adulthood. Acta Biomed. (2021) 92:e2021168. doi: 10.23750/abm.v92i1.11346
4. GBD 2017 Diarrhoeal Disease Collaborators. Quantifying risks and interventions that have affected the burden of diarrhoea among children younger than 5 years: an analysis of the global burden of disease study 2017. Lancet Infect Dis. (2020) 20:37–59. doi: 10.1016/S1473-3099(19)30401-3
5. Robert, E, Grippa, M, Nikiema, DE, Kergoat, L, Koudougou, H, Auda, Y, et al. Environmental determinants of E. coli, link with the diarrheal diseases, and indication of vulnerability criteria in tropical West Africa (Kapore, Burkina Faso). PLoS Negl Trop Dis. (2021) 15:e0009634. doi: 10.1371/journal.pntd.0009634
6. Martin. Water and sanitation. United Nations sustainable development. Available online at: https://www.un.org/sustainabledevelopment/water-and-sanitation/ (Accessed February 29, 2024)
7. Abebe, TA, and Tucho, GT. Open defecation-free slippage and its associated factors in Ethiopia: a systematic review. Syst Rev. (2020) 9:252. doi: 10.1186/s13643-020-01511-6
8. Alemu, ZA, Adugna, EA, Kidane, AW, Girmay, AM, Weldegebriel, MG, Likasa, BW, et al. Prevalence of open defecation practice and associated factors among households in Geshiyaro project implementation sites in Ethiopia: a cross-sectional study. Environ Health Insights. (2024) 18:11786302241252732. doi: 10.1177/11786302241252732
9. Ismail, AM, Ibrahim, MA, Abdi, MM, Geremew, A, Mulugeta, Y, Ayele, DM, et al. Open defecation practice among households with latrines in rural communities of Ararso District, Somali region, Eastern Ethiopia. Front Public Health. (2024) 12:1394351. doi: 10.3389/fpubh.2024.1394351
10. The MAL-ED Network Investigators. The MAL-ED study: a multinational and multidisciplinary approach to understand the relationship between enteric pathogens, malnutrition, gut physiology, physical growth, cognitive development, and immune responses in infants and children up to 2 years of age in resource-poor environments. Clin Infect Dis. (2014) 59:S193–206. doi: 10.1093/cid/ciu653
11. Arnold, BF, Null, C, Luby, SP, Unicomb, L, Stewart, CP, Dewey, KG, et al. Cluster-randomised controlled trials of individual and combined water, sanitation, hygiene and nutritional interventions in rural Bangladesh and Kenya: the WASH benefits study design and rationale. BMJ Open. (2013) 3:e003476. doi: 10.1136/bmjopen-2013-003476
12. The Sanitation Hygiene Infant Nutrition Efficacy (SHINE) Trial Team. The sanitation hygiene infant nutrition efficacy (SHINE) trial: rationale, design, and methods. Clin Infect Dis. (2015) 61:S685–702. doi: 10.1093/cid/civ844
13. Chen, D, McKune, SL, Singh, N, Yousuf Hassen, J, Gebreyes, W, Manary, MJ, et al. Campylobacter colonization, environmental enteric dysfunction, stunting, and associated risk factors among young children in rural Ethiopia: a cross-sectional study from the Campylobacter genomics and environmental enteric dysfunction (CAGED) project. Front Public Health. (2021) 8:615793. doi: 10.3389/fpubh.2020.615793
14. McLellan, SL, and Eren, AM. Discovering new indicators of fecal pollution. Trends Microbiol. (2014) 22:697–706. doi: 10.1016/j.tim.2014.08.002
15. Devane, ML, Moriarty, E, Weaver, L, Cookson, A, and Gilpin, B. Fecal indicator bacteria from environmental sources; strategies for identification to improve water quality monitoring. Water Res. (2020) 185:116204. doi: 10.1016/j.watres.2020.116204
16. Nowicki, S, de Laurent, ZR, de Villiers, EP, Githinji, G, and Charles, KJ. The utility of Escherichia coli as a contamination indicator for rural drinking water: evidence from whole genome sequencing. PLoS One. (2021) 16:e0245910. doi: 10.1371/journal.pone.0245910
17. Brown, J, Bir, A, and Bain, RES. Novel methods for global water safety monitoring: comparative analysis of low-cost, field-ready E. coli assays. NPJ Clean Water. (2020) 3:1–6. doi: 10.1038/s41545-020-0056-8
18. Hariri, S. Detection of Escherichia coli in food samples using culture and polymerase chain reaction methods. Cureus. (2022) 14:e32808. doi: 10.7759/cureus.32808
19. Terefe, Y, Deblais, L, Ghanem, M, Helmy, YA, Mummed, B, Chen, D, et al. Co-occurrence of Campylobacter species in children from eastern Ethiopia, and their association with environmental enteric dysfunction, diarrhea, and host microbiome. Front Public Health. (2020) 8:99. doi: 10.3389/fpubh.2020.00099
20. Havelaar, AH, Brhane, M, Ahmed, IA, Kedir, J, Chen, D, Deblais, L, et al. Unravelling the reservoirs for colonisation of infants with Campylobacter spp. in rural Ethiopia: protocol for a longitudinal study during a global pandemic and political tensions. BMJ Open. (2022) 12:e061311. doi: 10.1136/bmjopen-2022-061311
21. Deblais, L, Ojeda, A, Brhane, M, Mummed, B, Hassen, KA, Ahmedo, BU, et al. Prevalence and load of the Campylobacter genus in infants and associated household contacts in rural eastern Ethiopia: a longitudinal study from the Campylobacter genomics and environmental enteric dysfunction (CAGED) project. Appl Environ Microbiol. (2023) 89:e0042423. doi: 10.1128/aem.00424-23
22. Wang, Y, Moe, CL, Null, C, Raj, SJ, Baker, KK, Robb, KA, et al. Multipathway quantitative assessment of exposure to fecal contamination for young children in low-income urban environments in Accra, Ghana: the SaniPath analytical approach. Am J Trop Med Hyg. (2017) 97:1009–19. doi: 10.4269/ajtmh.16-0408
23. Romão, NF, Pereira, NAC, Funes-Huacca, ME, and Brito, LG. Portable diagnostic platform for detection of microorganisms coliforms and E. coli. Adv Microbiol. (2020) 10:224–37. doi: 10.4236/aim.2020.10518
24. Rodoplu, D, Chang, C, Kao, C, and Hsu, C. A micro-pupil device for point-of-care testing of viable Escherichia coli in tap water. Microchem J. (2022) 178:107390. doi: 10.1016/j.microc.2022.107390
25. Ahmed, W, Bivins, A, Payyappat, S, Cassidy, M, Harrison, N, and Besley, C. Distribution of human fecal marker genes and their association with pathogenic viruses in untreated wastewater determined using quantitative PCR. Water Res. (2022) 226:119093. doi: 10.1016/j.watres.2022.119093
26. Diner, RE, Zimmer-Faust, A, Cooksey, E, Allard, S, Kodera, SM, Kunselman, E, et al. Host and water microbiota are differentially linked to potential human pathogen accumulation in oysters. Appl Environ Microbiol. (2023) 89:e0031823–3. doi: 10.1128/aem.00318-23
27. Penakalapati, G, Swarthout, J, Delahoy, MJ, McAliley, L, Wodnik, B, Levy, K, et al. Exposure to animal feces and human health: a systematic review and proposed research priorities. Environ Sci Technol. (2017) 51:11537–52. doi: 10.1021/acs.est.7b02811
28. Mills, M, Lee, S, Piperata, BA, Garabed, R, Choi, B, and Lee, J. Household environment and animal fecal contamination are critical modifiers of the gut microbiome and resistome in young children from rural Nicaragua. Microbiome. (2023) 11:207. doi: 10.1186/s40168-023-01636-5
29. Baker, KK, O’Reilly, CE, Levine, MM, Kotloff, KL, Nataro, JP, Ayers, TL, et al. Sanitation and hygiene-specific risk factors for moderate-to-severe diarrhea in young children in the global enteric multicenter study, 2007–2011: case-control study. PLoS Med. (2016) 13:e1002010. doi: 10.1371/journal.pmed.1002010
30. Rakhalaru, P, Munzhedzi, L, Abia, ALK, Kabue, JP, Potgieter, N, and Traore, AN. Prevalence and antimicrobial resistance profile of Diarrheagenic Escherichia coli from fomites in rural households in South Africa. Antibiotics. (2023) 12:1345. doi: 10.3390/antibiotics12081345
31. Ramatla, T, Ramaili, T, Lekota, KE, Ndou, R, Mphuti, N, Bezuidenhout, C, et al. A systematic review and meta-analysis on prevalence and antimicrobial resistance profile of Escherichia coli isolated from water in africa (2000–2021). Heliyon. (2023) 9:e16123. doi: 10.1016/j.heliyon.2023.e16123
32. Fuhrmeister, ER, Ercumen, A, Grembi, JA, Islam, M, Pickering, AJ, and Nelson, KL. Shared bacterial communities between soil, stored drinking water, and hands in rural Bangladeshi households. Water Res X. (2020) 9:100056. doi: 10.1016/j.wroa.2020.100056
33. Wang, Y, Mairinger, W, Raj, SJ, Yakubu, H, Siesel, C, Green, J, et al. Quantitative assessment of exposure to fecal contamination in urban environment across nine cities in low-income and lower-middle-income countries and a city in the United States. Sci Total Environ. (2022) 806:151273. doi: 10.1016/j.scitotenv.2021.151273
34. Cantrell, ME, Sylvestre, É, Wharton, H, Scheidegger, R, Curchod, L, Gute, DM, et al. Hands are frequently contaminated with fecal bacteria and enteric pathogens globally: A systematic review and meta-analysis. Washington, DC: American Chemical Society (2023).
35. Rifqi, MA, Hamidah, U, Sintawardani, N, Harada, H, Nyambe, S, Sai, A, et al. Effect of handwashing on the reduction of Escherichia coli on children’s hands in an urban slum Indonesia. J Water Health. (2023) 21:1651–62. doi: 10.2166/wh.2023.121
36. Ferretti, P, Pasolli, E, Tett, A, Asnicar, F, Gorfer, V, Fedi, S, et al. Mother-to-infant microbial transmission from different body sites shapes the developing infant gut microbiome. Cell Host Microbe. (2018) 24:133–145.e5. doi: 10.1016/j.chom.2018.06.005
37. Fernández, L, Pannaraj, PS, Rautava, S, and Rodríguez, JM. The microbiota of the human mammary ecosystem. Front Cell Infect Microbiol. (2020) 10:586667. doi: 10.3389/fcimb.2020.586667
38. Navab-Daneshmand, T, Friedrich, MND, Gächter, M, Montealegre, MC, Mlambo, LS, Nhiwatiwa, T, et al. Escherichia coli contamination across multiple environmental compartments (soil, hands, drinking water, and handwashing water) in urban Harare: correlations and risk factors. Am J Trop Med Hyg. (2018) 98:803–13. doi: 10.4269/ajtmh.17-0521
Keywords: Escherichia coli , fecal contamination, eastern Ethiopia, environmental sampling, Chromocult colorimetric medium, EC-MUG fluorometric broth
Citation: Deblais L, Ahmedo BU, Ojeda A, Mummed B, Wang Y, Mekonnen YT, Demisie Weldesenbet Y, Hassen KA, Brhane M, McKune S, Havelaar AH, Liang S and Rajashekara G (2025) Assessing fecal contamination from human and environmental sources using Escherichia coli as an indicator in rural eastern Ethiopian households—a cross-sectional study from the EXCAM project. Front. Public Health. 12:1484808. doi: 10.3389/fpubh.2024.1484808
Edited by:
Juarez Antonio Simões Quaresma, Federal University of Pará, BrazilReviewed by:
Youngbeom Ahn, National Center for Toxicological Research (FDA), United StatesEustachio Cuscianna, University of Bari Aldo Moro, Italy
Copyright © 2025 Deblais, Ahmedo, Ojeda, Mummed, Wang, Mekonnen, Demisie Weldesenbet, Hassen, Brhane, McKune, Havelaar, Liang and Rajashekara. This is an open-access article distributed under the terms of the Creative Commons Attribution License (CC BY). The use, distribution or reproduction in other forums is permitted, provided the original author(s) and the copyright owner(s) are credited and that the original publication in this journal is cited, in accordance with accepted academic practice. No use, distribution or reproduction is permitted which does not comply with these terms.
*Correspondence: Gireesh Rajashekara, Z2lyZWVzaDJAaWxsaW5vaXMuZWR1
†These authors have contributed equally to this work