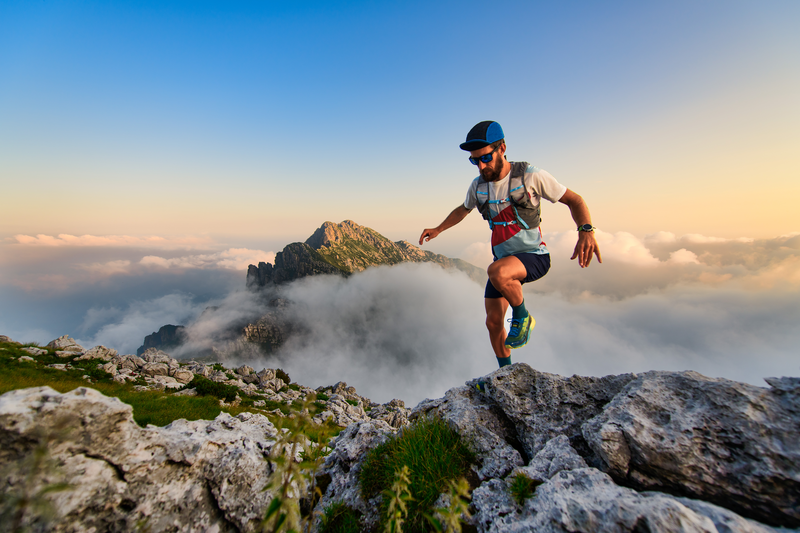
95% of researchers rate our articles as excellent or good
Learn more about the work of our research integrity team to safeguard the quality of each article we publish.
Find out more
MINI REVIEW article
Front. Public Health , 23 October 2024
Sec. Children and Health
Volume 12 - 2024 | https://doi.org/10.3389/fpubh.2024.1455503
This article is part of the Research Topic Preventing Childhood Asthma: the Neglected Impact of Existing Public Health Interventions View all 12 articles
Socioeconomic (SE) disparity and health inequity are closely intertwined and associated with cross-generational increases in the rates of multiple chronic non-communicable diseases (NCDs) in North America and beyond. Coinciding with this social trend is an observed loss of biodiversity within the community of colonizing microbes that live in and on our bodies. Researchers have rightfully pointed to the microbiota as a key modifiable factor with the potential to ease existing health inequities. Although a number of studies have connected the adult microbiome to socioeconomic determinants and health outcomes, few studies have investigated the role of the infant microbiome in perpetuating these outcomes across generations. It is an essential and important question as the infant microbiota is highly sensitive to external forces, and observed shifts during this critical window often portend long-term outcomes of health and disease. While this is often studied in the context of direct modulators, such as delivery mode, family size, antibiotic exposure, and breastfeeding, many of these factors are tied to underlying socioeconomic and/or cross-generational factors. Exploring cross-generational socioeconomic and health inequities through the lens of the infant microbiome may provide valuable avenues to break these intergenerational cycles. In this review, we will focus on the impact of social inequality in infant microbiome development and discuss the benefits of prioritizing and restoring early-life microbiota maturation for reducing intergenerational health disparities.
Socioeconomic disparities are correlated with significant health inequities, as a lower socioeconomic status (SES) increases the likelihood of NCDs, such as chronic respiratory diseases, cardiometabolic diseases, oral diseases, and mental illnesses (1–5). Although researchers have identified environmental mediators, the mechanisms through which SE inequity becomes physiologically embedded remain unclear, especially regarding cross-generational health disparities that can be observed at a very young age (6–8). The observation that children from lower SES families are more likely to experience risk factors for NCDs such as obesity, cardiovascular risk, and behavioral difficulties, is alarming, as many of these diseases are associated with comorbidities that can significantly alter their lifelong health trajectories (1, 8). Thus, research into the biological underpinnings linking lower SES to greater disease risk, with a specific focus on perinatal and early-life risk factors, is increasingly needed.
Our microbiota is a significant factor bridging environmental exposures with host physiology or the microbial community that lives in/on our bodies. In this review, we examine the gut microbiota, which has the greatest bacterial abundance and diversity in the body (9). Immediately after birth, the gut microbiota starts to assemble alongside infant development, eventually forming a stable community. During this early stage, the developing microbiota is highly sensitive to external influences, with changes impacting long-term immune regulation, metabolism, and behavior (10–13). Therefore, infancy is a critical period of microbial and childhood development that has far-reaching impacts on later childhood health and disease.
Interest surrounding the impact of SES on the infant and childhood gut microbiota has grown in recent years, and many factors known to disrupt infant gut microbial communities (e.g., elevated cesarean-sections (C-sections), difficulty maintaining breastfeeding, reduced access to fresh foods, increased antibiotic exposure, and reduced proximity to greenspace) are also encountered by SES-disadvantaged populations and communities (14–19). As such, the role the microbiota plays in observed SES-mediated childhood health inequities is gradually gaining recognition. In this review, we will highlight findings linking SES-associated factors to the infant’s gut microbiota (Table 1). We will also explore accessible strategies for safeguarding and restoring the early-life microbiota in our society, with the goal of reducing health disparities for future generations (Figure 1).
Figure 1. Factors influencing infant microbiome development, focusing on social inequality and potential public interventions to address disparities in child health.
Before exploring the links between social inequity and the infant microbiome, it is important to discuss our current understanding of microbiota development. The primary seeding point for the gut microbiota is at birth, which is significantly influenced by the delivery mode, maternal microbiota, and maternally-derived metabolites and immune components (20–25). Initial colonization is primarily dominated by aerobes and facultative anaerobes, such as Staphylococcus and γ-Proteobacteria, which gradually reduce oxygen to support anaerobic colonizers, such as Bifidobacterium, Bacteroides, and multiple Clostridia genera (26). Within the first few months of life, species diversity is limited and composition is primarily dictated by type of milk diet (27). Later, as infants experience more varied environments and diets, their microbiota diversity increases. As such, prior to reaching a stable community, the microbiota matures in the first 1–3 years through patterned temporal shifts in species abundances that can be commonly detected across studies (11, 28, 29). Supporting the importance of this maturation process, microbiota disruptions during infancy—but not later—are broadly associated with a higher likelihood of allergic disease, obesity, and behavioral disorders (11, 12, 29, 30). Therefore, prioritizing normative microbiota maturation by limiting disruptions or implementing restorative interventions during this sensitive window can yield lifelong health benefits.
The mode of delivery is a primary driver of the initial compositional variance in the infant gut microbiota. However, multiple independent studies within developed nations have shown that women from lower SE backgrounds have a higher likelihood of undergoing C-section deliveries (31–34). Compared to infants born vaginally, infants born via C-sections harbor fewer maternal bacterial strains, display reduced diversity, and tend to have fewer Bacteroides and Bifidobacterium; and these alterations are independent of additional exposures, such as antibiotic use during delivery and a reduced ability to initiate breastfeeding (24, 25, 27, 35–40). However, the impact of this on later health is still unclear. Although a C-section delivery within some studies has been implicated in an elevated risk of childhood obesity, asthma and immune disorders (41–43), others have not observed any correlation (44, 45). This likely underscores a compounding effect of risk factors and implies that the negative outcomes associated with C-section deliveries could be outweighed by subsequent early-life experiences.
Immediately after birth, the diverse array of human milk components is crucial for shaping the infant’s gut microbiota and supporting infant development (36, 39, 46, 47). These include secretory IgA (sIgA) (48), the milk microbiota, which serves as a significant seeding source (25, 49), and metabolites such as human milk oligosaccharides (HMOs), which serves as the third most abundant milk metabolite yet primarily serve to nourish ‘infant-type’ bacteria (50). As a constant force on the infant microbiome, breastfeeding can mitigate disruptions caused by both C-sections and antibiotic exposure (51–53). However, lower-income families often report difficulty initiating breastfeeding and shorter overall breastfeeding durations (54, 55). This has the potential to profoundly impact the infant microbiota, as formula-fed babies experience premature microbiota maturation, reduced abundance of Bifidobacterium, and more opportunistic pathogens compared to their breastfed counterparts (24, 27, 36, 37, 39, 52, 56, 57). These differences are potentially associated with child health consequences, as evidenced by a large, nationally representative longitudinal study in the United States (n = 8,030 participants), which identified infant feeding practices as a significant mediator between SES and early childhood obesity (58). Thus, greater support is needed for mothers and families who would otherwise breastfeed their infants, but are unable to do so.
Antibiotics are a potent medical intervention that reduces or depletes pathogenic microbes and has considerably reduced human mortality since their discovery. However, antibiotics, especially broad-spectrum ones, commonly target both pathogenic and beneficial commensal bacteria indiscriminately; and extensive evidence exists for their off-target disruption of infant gut microbiota homeostasis. The detrimental effects include a reduction in species diversity, an enrichment of antimicrobial resistance genes (ARGs), the loss of beneficial bystanders such as Bifidobacterium and Clostridia members, and elevated γ-Proteobacteria during recovery (35, 37, 38, 52, 59–61). In addition, a number of animal studies provide evidence of the causal impact of early-life antibiotic-induced microbiota disruptions on long-term host health (62, 63). Infancy is particularly sensitive to the disruptions of early-life antibiotic exposure, which is widely associated with subsequent risk of inflammatory bowel disease, atopy, asthma, and obesity (61, 64–68). Despite being intrinsically linked to medical care access, lower SES in wealthy countries is paradoxically associated with higher early-life antibiotic exposure (69–71). Indeed, a large US study found that children from lower SES families, particularly in high-poverty areas, received more antibiotics in the first month of life despite receiving fewer antibiotic prescriptions over their lifetime (72). More alarming trends are seen from children in low- and middle-income countries, who are prescribed an average of 25 antibiotic prescriptions during their first 5 years of life. This is a remarkable amount considering that two antibiotic prescriptions per year are considered excessive in many high-income countries (73). Antibiotic stewardship initiatives, which primarily aim at reducing the spread of antimicrobial resistance, may therefore possess the additional benefit of preserving the healthy infant microbiota (60, 71, 74, 75).
Malnutrition can be characterized by the over- or under-bioavailability of both macro- and micronutrients, and is commonly observed in low SES and minoritized populations (2, 76, 77). Due to its capacity to regulate nutritional harvest, the gut microbiota is an important nexus between diet and health outcomes (30). This is evidenced by a longitudinal study of Malawian twins, which found that poorly matured gut microbiota was associated with malnutrition and the causal relationship between microbiota and malnutrition is supported by multiple independent mouse studies (78–80). Malnutrition is associated with reduced obligate anaerobic species and an increase in potentially pathogenic microbes in the infant gut microbiome (78, 81–84). Furthermore, this phenomenon is widespread, as multiple studies in low-SES countries have consistently demonstrated decreased bacterial diversity in malnourished children, reduced beneficial microbes and increased pathogen enrichment (82, 84, 85). Recent randomized clinical trials have established that microbiota-directed foods, but not caloric intake alone, successfully support growth recovery in malnourished children (86, 87). These findings confirm the microbiota’s role in malnutrition and emphasize the need for microbiota-informed interventions in its management.
The environment to which humans are exposed throughout their lives is a complex and important determinant of microbiota composition, with broad implications for NCDs (88–92). The infant microbiota is particularly reflective of its surroundings, with exposures from air pollution, older siblings, pets, and farms all linked to differences in composition (27, 37, 56, 93–96). Furthermore, these have enduring impacts on both the microbiota community and our health. For instance, a comprehensive Dutch study found that childhood living environments were significantly associated with adult microbiota composition despite only a weak association between childhood and adult urbanicity (97). Similarly, research has shown that children who lived on farms or near farm-like environments during the perinatal period had a lower risk of asthma later in life (98, 99). Given that an increase in SES in urban environments is positively linked to surrounding greenspace and biodiversity, and that SES-disadvantaged neighborhoods exhibit measurably reduced microbiota diversity (92), efforts to ‘rewild’ our cities and expanding public access to greenspaces could be incredibly effective ways to facilitate these vital health-promoting microbial exposures.
The quality of the maternal diet is heavily influenced by the resources afforded by SES (100–102). Not only do mothers’ dietary patterns influence their own microbiota, they are also determinants of health outcomes during and after pregnancy (102, 103). As such, maternal diet can significantly influence the infant microbiota via impacting breastfeeding outcomes, altering breastmilk components, or influencing maternal microbes that seed the infant (40, 104). Importantly, these effects can persist or even compound over generations (105). The exact impacts that maternal diet has on health outcomes of offspring are best measured in well controlled animal models. For instance, after researchers in China found that maternal obesity affected child neurodevelopment, they were able to mirror the phenomenon in mice by maternal fecal microbial transplantation (FMT) and reverse the phenotype by feeding the dams high-fiber diets (106). Similarly, a maternal low-fiber diet in mice predisposed offspring to severe lower respiratory tract infections and asthma, and milk microbes from dams fed a high-fiber diet were able to rescue this effect (107). Therefore, prioritizing access to nutritiously complete maternal diets may mitigate detrimental cross-generational impacts of low SES on infant and child health.
The relationship between SES and prenatal antibiotic use remains unclear, with results being largely dependent on the specific SES measures, study cohort demographics, and economic status of the origin country (108–110). However, we mention it because, despite birth being a crucial seeding point for the microbiota, the rates of antibiotic prescription during pregnancy and birth are markedly high. Indeed, in Western countries, between 30 and 40% of women receive antibiotics either prenatally or during birth, with the majority prescribed for prophylactic reasons (111–113). This has real consequences on the neonatal microbiota. Infants with mothers exposed to antibiotics during pregnancy are reported to have reduced microbial diversity, less abundant Bacteriodetes and Bifidobacteria, and expanded γ-Proteobacteria (114, 115). Importantly, while the risks associated with antibiotic disruptions can be mitigated with breastfeeding, this may be less available to mothers of lower SES, particularly in countries with reduced access to paid maternity leave (52, 116).
The strong link between lower SES and prenatal distress is associated with several adverse health outcomes in offspring, such as preterm birth, low birth weight, and negative neurodevelopmental outcomes (117–119). Various changes have been reported between infant microbiota and different indicators of prenatal stress (e.g., subjective distress, cortisol level, precarity, prenatal anxiety, depression, and perceived stress), the most consistent being enrichment of γ-Proteobacteria and reduction in Bifidobacterium. Although reductions in species diversity have been reported, they have not been as consistent and appear to vary depending on the measure and timing of distress, as well as the age of the offspring (120, 121). These effects may be partially explained by altering gut microbial composition during pregnancy and transmission of disrupted vaginal microbiome at birth based on mouse models (122, 123).
Low SES is a risk factor for smoking, and maternal smoking during pregnancy is associated with increased incidence of premature birth, childhood obesity, developmental delays, respiratory disease, and long-term morbidity among offspring (124–127). Evidence connecting maternal smoke exposure to the infant gut microbiota is limited, but consistent. A review of three studies found that infants exposed to maternal smoke had an increased Firmicutes richness, which was associated with an elevated risk of childhood overweight and obesity (128). Similar findings were independently observed in a recent study using the cohort data from the Canadian Healthy Infant Longitudinal Development (CHILD) study, Canada, which revealed that maternal smoking during pregnancy increases the risk of being overweight at 1 and 3 years, and this was mediated by an increase in Firmicutes diversity (129). Interestingly, although smoking cessation during pregnancy does not reduce the risk of offspring being overweight, exclusive breastfeeding does.
Given the impact SES-linked factors have on the infant microbiota and long-term health, prioritizing healthy microbiota development during infancy may alleviate health inequities for future generations. Considerable attention has been given in other reviews to highlight specific avenues that reduce disruptions, reinforce health-promoting microbial interactions, and replenish important species when they are lost (10, 130–132). We will highlight methods that can be broadly integrated into public health efforts to inclusively bolster infant microbiota development throughout our society.
An increasing number of antibiotic stewardship programs (ASPs) have been successfully implemented worldwide, leading to reduced antibiotic use, improved clinical and microbiological outcomes and economic benefits, indicating the effectiveness of this public health intervention (133–136). While the public focus on antibiotic stewardship has been aimed at limiting antimicrobial resistance, an unintended benefit may be protecting vital microbial species within the infant microbiota, which reduce instances of childhood NCDs. Supporting this, our own findings linked recent declines in antibiotic prescriptions within British Columbia, Canada to lower rates of pediatric asthma, with the infant microbiota demonstrated to be the likely mediator of this relationship (60). As additional public health ASPs are launched, it will be interesting to observe if these trends are recapitulated across communities.
Despite the clear benefits of breastfeeding and recommendations to initiate within the first hour of life and exclusively breastfeed for the first 6 months, SES-disadvantaged families often have lower breastfeeding initiation and duration rates (137). This can be attributed to barriers such as a lack of breastfeeding educational resources, an absence of breastfeeding experience within previous generations, and employment and financial limitations (132, 138, 139). Some of these can be addressed at the individual or community level, as evidenced by randomized controlled trials that have successfully increased breastfeeding motivation and success (140, 141). However, societal-level policy and fiscal support, such as generous, universally paid parental leave, are needed to decrease the chasm between low- and high-SES families (132, 142). Furthermore, when breastfeeding is just not an option, regardless of support, standard care that includes access to donor breast milk or prioritized research into formulas that mimic human milk is needed to support the infant microbiota (143, 144). All of these in parallel are necessary to improve long-lasting cross-generational health outcomes and reduce health disparity.
Many researchers now believe that our society’s intergenerational loss of microbiota biodiversity has paralleled the rise in NCDs (145, 146). This indicates that reinforcements, such as breastfeeding, alone may be insufficient and will need to be complemented by reintroducing key species to infants. Despite the efficacy of full microbiota replacement through FMT for severe diseases such as Clostridioides difficile in older populations, it is unlikely to serve as a preventative measure in infants (147). Even randomized controlled trials that effectively swabbed C-section born neonates with maternal vaginal microbes faced significant opposition from the medical community (148, 149). More feasible are live biotherapeutic products (LBPs) that contain rigorously controlled and tested microbial species to replenish key missing microbes. One excellent example is B. infantis, a crucial HMO-utilizing microbe that has declined in North America (95, 150–152). To combat this, community-based stool testing during checkups could identify infants who would benefit from a B. infantis LBP, which has been shown to successfully colonize infants to reduce gut dysbiosis and shape immune development (151, 153–155). Beyond B. infantis, Bifidobacterium and Lactobacillus contain a number of secure and effective species that can colonize both breastmilk and neonate gut microbiota when administered to mothers during pregnancy or lactation (156, 157). However, studies supporting their utility for long-term colonization and health benefits are still lacking (158, 159). In addition to targeted microbial restoration, a broader “rewilding” of urban environments could provide widespread and lasting benefits to counter biodiversity loss. Indeed, the soil microbiota found in revegetated urban spaces mimics that of natural vegetation, supporting the feasibility of reintroducing wild microbes into urban environments. Naturalized greenspaces can boost microbial biodiversity in young children, with measurable impacts benefitting tolerant immune responses (160, 161). In addition, there is more work to be done in public health policy to mitigate SES-associated microbiota and health inequity, such as making healthcare more affordable, providing education to the general public (i.e., hygiene hypothesis), and increasing access to fresh foods. Collectively, public health initiatives may be able to equitably restore necessary early-life microbiota interactions at the community level, thereby reducing or preventing microbiota-associated SES disparities and ensuring equitable health outcomes.
Over the past several decades, growing evidence has demonstrated the impact of early-life and cross-generational factors on infant microbiota development. These factors include delivery mode, breastfeeding, maternal stress, diet, and urbanization, all of which are commonly associated with SES. Since microbiota composition is modifiable and associated with infant immune development and long-term health, it represents an important opportunity to mitigate the impact of SES inequity on health disparity. In this context, broadly integrated biomedical and policy interventions aimed at reducing, reinforcing, and replenishing the disrupted microbiota should be adopted and provided at equalizing access. In addition, further studies aimed at understanding SES-associated microbiota diversity, composition, and functions in large-scale, diverse, and longitudinal settings are needed to better understand the variations in gut microbiota across diverse geographies, ethnicities, lifestyles, and ages.
DD: Conceptualization, Visualization, Writing – original draft, Writing – review & editing. CP: Conceptualization, Supervision, Visualization, Writing – review & editing. ST: Conceptualization, Supervision, Writing – review & editing, Funding acquisition, Project administration, Resources.
The author(s) declare that financial support was received for the research, authorship, and/or publication of this article. S.E.T. holds a Tier 1 Canada Research Chair in Pediatric Precision Health and the Aubrey J. Tingle Professor of Pediatric Immunology. For this research, support was provided by the Canadian Institutes of Health Research (CIHR; EC1-144621), the Allergy, Genes and Environment Network of Centres of Excellence (AllerGen NCE) (12CHILD) and Genome Canada/Genome BC (274CHI). D.L.Y.D. is supported by a Canadian Institute of Health Research Frederick Banting and Charles Best Canada Graduate Scholarship Doctoral Award (CIHR CGS-D) and the University of British Columbia Four Year Doctoral Fellowship (4YF).
The authors acknowledge the value of ongoing conversations with members of the Turvey lab and the CHILD study in refining the concepts developed in this review. The authors acknowledge the support of Courtney Hoskinson in finalizing the article.
The authors declare that the research was conducted in the absence of any commercial or financial relationships that could be construed as a potential conflict of interest.
The author(s) declared that they were an editorial board member of Frontiers, at the time of submission. This had no impact on the peer review process and the final decision.
All claims expressed in this article are solely those of the authors and do not necessarily represent those of their affiliated organizations, or those of the publisher, the editors and the reviewers. Any product that may be evaluated in this article, or claim that may be made by its manufacturer, is not guaranteed or endorsed by the publisher.
1. Braveman, PA, Cubbin, C, Egerter, S, Williams, DR, and Pamuk, E. Socioeconomic disparities in health in the United States: what the patterns tell us. Am J Public Health. (2010) 100 Suppl 1:S186–96. doi: 10.2105/AJPH.2009.166082
2. Ismail, SU, Asamane, EA, Osei-Kwasi, HA, and Boateng, D. Socioeconomic determinants of cardiovascular diseases, obesity, and diabetes among migrants in the United Kingdom: a systematic review. Int J Environ Res Public Health. (2022) 19:3070. doi: 10.3390/ijerph19053070
3. Reiss, F, Meyrose, AK, Otto, C, Lampert, T, Klasen, F, and Ravens-Sieberer, U. Socioeconomic status, stressful life situations and mental health problems in children and adolescents: results of the German BELLA cohort-study. PLoS One. (2019) 14:e0213700. doi: 10.1371/journal.pone.0213700
4. Ellison-Loschmann, L, Sunyer, J, Plana, E, Pearce, N, Zock, JP, Jarvis, D, et al. Socioeconomic status, asthma and chronic bronchitis in a large community-based study. Eur Respir J. (2007) 29:897–905. doi: 10.1183/09031936.00101606
5. de Lucena, EHG, da Silva, RO, Barbosa, ML, de Araújo, ECF, Pereira, AC, and Cavalcanti, YW. Influence of socioeconomic status on oral disease burden: a population-based study. BMC Oral Health. (2021) 21:608. doi: 10.1186/s12903-021-01970-w
6. Moor, I, Spallek, J, and Richter, M. Explaining socioeconomic inequalities in self-rated health: a systematic review of the relative contribution of material, psychosocial and behavioural factors. J Epidemiol Community Health. (2017) 71:565–75. doi: 10.1136/jech-2016-207589
7. Bradley, RH, and Corwyn, RF. Socioeconomic status and child development. Annu Rev Psychol. (2002) 53:371–99. doi: 10.1146/annurev.psych.53.100901.135233
8. Poulain, T, Vogel, M, and Kiess, W. Review on the role of socioeconomic status in child health and development. Curr Opin Pediatr. (2020) 32:308–14. doi: 10.1097/MOP.0000000000000876
9. Deo, PN, and Deshmukh, R. Oral microbiome: unveiling the fundamentals. J Oral Maxillofac Pathol. (2019) 23:122–8. doi: 10.4103/jomfp.JOMFP_304_18
10. Petersen, C, and Turvey, SE. Can we prevent allergic disease? Understanding the links between the early life microbiome and allergic diseases of childhood. Curr Opin Pediatr. (2020) 32:790–7. doi: 10.1097/mop.0000000000000956
11. Donald, K, and Finlay, BB. Early-life interactions between the microbiota and immune system: impact on immune system development and atopic disease. Nat Rev Immunol. (2023) 23:735–48. doi: 10.1038/s41577-023-00874-w
12. Dominguez-Bello, MG, Godoy-Vitorino, F, Knight, R, and Blaser, MJ. Role of the microbiome in human development. Gut. (2019) 68:1108–14. doi: 10.1136/gutjnl-2018-317503
13. Arrieta, MC, Stiemsma, LT, Dimitriu, PA, Thorson, L, Russell, S, Yurist-Doutsch, S, et al. Investigators: early infancy microbial and metabolic alterations affect risk of childhood asthma. Sci Transl Med. (2015) 7:307ra152. doi: 10.1126/scitranslmed.aab2271
14. Amaruddin, AI, Hamid, F, Koopman, JPR, Muhammad, M, Brienen, EA, van Lieshout, L, et al. The bacterial gut microbiota of schoolchildren from high and low socioeconomic status: a study in an urban area of Makassar, Indonesia. Microorganisms. (2020) 8:961. doi: 10.3390/microorganisms8060961
15. Lewis, CR, Bonham, KS, McCann, SH, Volpe, AR, D'Sa, V, Naymik, M, et al. Family SES is associated with the gut microbiome in infants and children. Microorganisms. (2021) 9:1608. doi: 10.3390/microorganisms9081608
16. Lapidot, Y, Reshef, L, Maya, M, Cohen, D, Gophna, U, and Muhsen, K. Socioeconomic disparities and household crowding in association with the fecal microbiome of school-age children. NPJ Biofilms Microbiomes. (2022) 8:10. doi: 10.1038/s41522-022-00271-6
17. Gschwendtner, S, Kang, H, Thiering, E, Kublik, S, Fösel, B, Schulz, H, et al. Early life determinants induce sustainable changes in the gut microbiome of six-year-old children. Sci Rep. (2019) 9:12675. doi: 10.1038/s41598-019-49160-7
18. Francis, J, Mildon, A, Stewart, S, Underhill, B, Tarasuk, V, Di Ruggiero, E, et al. Vulnerable mothers' experiences breastfeeding with an enhanced community lactation support program. Matern Child Nutr. (2020) 16:e12957. doi: 10.1111/mcn.12957
19. Christian, VJ, Miller, KR, and Martindale, RG. Food insecurity, malnutrition, and the microbiome. Curr Nutr Rep. (2020) 9:356–60. doi: 10.1007/s13668-020-00342-0
20. Rackaityte, E, Halkias, J, Fukui, EM, Mendoza, VF, Hayzelden, C, Crawford, ED, et al. Viable bacterial colonization is highly limited in the human intestine in utero. Nat Med. (2020) 26:599–607. doi: 10.1038/s41591-020-0761-3
21. de Goffau, MC, Lager, S, Sovio, U, Gaccioli, F, Cook, E, Peacock, SJ, et al. Human placenta has no microbiome but can contain potential pathogens. Nature. (2019) 572:329–34. doi: 10.1038/s41586-019-1451-5
22. Mishra, A, Lai, GC, Yao, LJ, Aung, TT, Shental, N, Rotter-Maskowitz, A, et al. Microbial exposure during early human development primes fetal immune cells. Cell. (2021) 184:3394–3409.e20. doi: 10.1016/j.cell.2021.04.039
23. Kennedy, KM, de Goffau, MC, Perez-Muñoz, ME, Arrieta, MC, Bäckhed, F, Bork, P, et al. Questioning the fetal microbiome illustrates pitfalls of low-biomass microbial studies. Nature. (2023) 613:639–49. doi: 10.1038/s41586-022-05546-8
24. Bäckhed, F, Roswall, J, Peng, Y, Feng, Q, Jia, H, Kovatcheva-Datchary, P, et al. Dynamics and stabilization of the human gut microbiome during the first year of life. Cell Host Microbe. (2015) 17:690–703. doi: 10.1016/j.chom.2015.04.004
25. Bogaert, D, van Beveren, GJ, de Koff, EM, Lusarreta Parga, P, Balcazar Lopez, CE, Koppensteiner, L, et al. Mother-to-infant microbiota transmission and infant microbiota development across multiple body sites. Cell Host Microbe. (2023) 31:447–460.e6. doi: 10.1016/j.chom.2023.01.018
26. Robertson, RC, Manges, AR, Finlay, BB, and Prendergast, AJ. The human microbiome and child growth - first 1000 days and beyond. Trends Microbiol. (2019) 27:131–47. doi: 10.1016/j.tim.2018.09.008
27. Stewart, CJ, Ajami, NJ, O'Brien, JL, Hutchinson, DS, Smith, DP, Wong, MC, et al. Temporal development of the gut microbiome in early childhood from the TEDDY study. Nature. (2018) 562:583–8. doi: 10.1038/s41586-018-0617-x
28. Petersen, C, Dai, DLY, Boutin, RCT, Sbihi, H, Sears, MR, Moraes, TJ, et al. A rich meconium metabolome in human infants is associated with early-life gut microbiota composition and reduced allergic sensitization. Cell Rep Med. (2021) 2:100260. doi: 10.1016/j.xcrm.2021.100260
29. Hoskinson, C, Dai, DLY, Del Bel, KL, Becker, AB, Moraes, TJ, Mandhane, PJ, et al. Delayed gut microbiota maturation in the first year of life is a hallmark of pediatric allergic disease. Nat Commun. (2023) 14:4785. doi: 10.1038/s41467-023-40336-4
30. Reyna, ME, Petersen, C, Dai, DLY, Dai, R, Becker, AB, Azad, MB, et al. Longitudinal body mass index trajectories at preschool age: children with rapid growth have differential composition of the gut microbiota in the first year of life. Int J Obes. (2022) 46:1351–8. doi: 10.1038/s41366-022-01117-z
31. Kottwitz, A . Mode of birth and social inequalities in health: the effect of maternal education and access to hospital care on cesarean delivery. Health Place. (2014) 27:9–21. doi: 10.1016/j.healthplace.2014.01.005
32. Linton, A, Peterson, MR, and Williams, TV. Effects of maternal characteristics on cesarean delivery rates among U.S. Department of Defense healthcare beneficiaries, 1996-2002. Birth. (2004) 31:3–11. doi: 10.1111/j.0730-7659.2004.0268.x
33. Joseph, KS, Dodds, L, Allen, AC, Jones, DV, Monterrosa, L, Robinson, H, et al. Socioeconomic status and receipt of obstetric services in Canada. Obstet Gynecol. (2006) 107:641–50. doi: 10.1097/01.AOG.0000201977.45284.3c
34. Milcent, C, and Zbiri, S. Prenatal care and socioeconomic status: effect on cesarean delivery. Health Econ Rev. (2018) 8:7. doi: 10.1186/s13561-018-0190-x
35. Bokulich, NA, Chung, J, Battaglia, T, Henderson, N, Jay, M, Li, H, et al. Antibiotics, birth mode, and diet shape microbiome maturation during early life. Sci Transl Med. (2016) 8:343ra82. doi: 10.1126/scitranslmed.aad7121
36. Fehr, K, Moossavi, S, Sbihi, H, Boutin, RCT, Bode, L, Robertson, B, et al. Breastmilk feeding practices are associated with the co-occurrence of Bacteria in Mothers' Milk and the infant gut: the CHILD cohort study. Cell Host Microbe. (2020) 28:285–297.e4. doi: 10.1016/j.chom.2020.06.009
37. Penders, J, Thijs, C, Vink, C, Stelma, FF, Snijders, B, Kummeling, I, et al. Factors influencing the composition of the intestinal microbiota in early infancy. Pediatrics. (2006) 118:511–21. doi: 10.1542/peds.2005-2824
38. Yassour, M, Vatanen, T, Siljander, H, Hämäläinen, AM, Härkönen, T, Ryhänen, SJ, et al. Natural history of the infant gut microbiome and impact of antibiotic treatment on bacterial strain diversity and stability. Sci Transl Med. (2016) 8:343ra81. doi: 10.1126/scitranslmed.aad0917
39. Reyman, M, van Houten, MA, van Baarle, D, Bosch, AATM, Man, WH, Chu, MLJN, et al. Impact of delivery mode-associated gut microbiota dynamics on health in the first year of life. Nat Commun. (2019) 10:4997. doi: 10.1038/s41467-019-13014-7
40. Lundgren, SN, Madan, JC, Emond, JA, Morrison, HG, Christensen, BC, Karagas, MR, et al. Maternal diet during pregnancy is related with the infant stool microbiome in a delivery mode-dependent manner. Microbiome. (2018) 6:109. doi: 10.1186/s40168-018-0490-8
41. Sevelsted, A, Stokholm, J, Bønnelykke, K, and Bisgaard, H. Cesarean section and chronic immune disorders. Pediatrics. (2015) 135:e92–8. doi: 10.1542/peds.2014-0596
42. Darmasseelane, K, Hyde, MJ, Santhakumaran, S, Gale, C, and Modi, N. Mode of delivery and offspring body mass index, overweight and obesity in adult life: a systematic review and meta-analysis. PLoS One. (2014) 9:e87896. doi: 10.1371/journal.pone.0087896
43. Kristensen, K, and Henriksen, L. Cesarean section and disease associated with immune function. J Allergy Clin Immunol. (2016) 137:587–90. doi: 10.1016/j.jaci.2015.07.040
44. Pyrhönen, K, Näyhä, S, Hiltunen, L, and Läärä, E. Caesarean section and allergic manifestations: insufficient evidence of association found in population-based study of children aged 1 to 4 years. Acta Paediatr. (2013) 102:982–9. doi: 10.1111/apa.12342
45. Ahlqvist, VH, Persson, M, Magnusson, C, and Berglind, D. Elective and nonelective cesarean section and obesity among young adult male offspring: a Swedish population-based cohort study. PLoS Med. (2019) 16:e1002996. doi: 10.1371/journal.pmed.1002996
46. Yang, R, Gao, R, Cui, S, Zhong, H, Zhang, X, Chen, Y, et al. Dynamic signatures of gut microbiota and influences of delivery and feeding modes during the first 6 months of life. Physiol Genomics. (2019) 51:368–78. doi: 10.1152/physiolgenomics.00026.2019
47. Ballard, O, and Morrow, AL. Human milk composition: nutrients and bioactive factors. Pediatr Clin N Am. (2013) 60:49–74. doi: 10.1016/j.pcl.2012.10.002
48. Donald, K, Petersen, C, Turvey, SE, Finlay, BB, and Azad, MB. Secretory IgA: linking microbes, maternal health, and infant health through human milk. Cell Host Microbe. (2022) 30:650–9. doi: 10.1016/j.chom.2022.02.005
49. Yassour, M, Jason, E, Hogstrom, LJ, Arthur, TD, Tripathi, S, Siljander, H, et al. Strain-level analysis of mother-to-child bacterial transmission during the first few months of life. Cell Host Microbe. (2018) 24:146–154.e4. doi: 10.1016/j.chom.2018.06.007
50. Lin, C, Lin, Y, Zhang, H, Wang, G, Zhao, J, and Chen, W. Intestinal 'Infant-Type' Bifidobacteria mediate immune system development in the first 1000 days of life. Nutrients. (2022) 14:1498. doi: 10.3390/nu14071498
51. Korpela, K, Salonen, A, Hickman, B, Kunz, C, Sprenger, N, Kukkonen, K, et al. Fucosylated oligosaccharides in mother's milk alleviate the effects of caesarean birth on infant gut microbiota. Sci Rep. (2018) 8:13757. doi: 10.1038/s41598-018-32037-6
52. Dai, DLY, Petersen, C, Hoskinson, C, Del Bel, KL, Becker, AB, Moraes, TJ, et al. Breastfeeding enrichment of B. longum subsp. infantis mitigates the effect of antibiotics on the microbiota and childhood asthma risk. Med. (2023) 4:92–112.e5. doi: 10.1016/j.medj.2022.12.002
53. Pärnänen, KMM, Hultman, J, Markkanen, M, Satokari, R, Rautava, S, Lamendella, R, et al. Early-life formula feeding is associated with infant gut microbiota alterations and an increased antibiotic resistance load. Am J Clin Nutr. (2022) 115:407–21. doi: 10.1093/ajcn/nqab353
54. Foster, SF, Vazquez, C, Cubbin, C, Nichols, AR, Rickman, RR, and Widen, EM. Breastfeeding, socioeconomic status, and long-term postpartum weight retention. Int Breastfeed J. (2023) 18:1. doi: 10.1186/s13006-022-00534-0
55. Carpay, NC, Kakaroukas, A, Embleton, ND, and van Elburg, RM. Barriers and facilitators to breastfeeding in moderate and late preterm infants: a systematic review. Breastfeed Med. (2021) 16:370–84. doi: 10.1089/bfm.2020.0379
56. Ma, J, Li, Z, Zhang, W, Zhang, C, Zhang, Y, Mei, H, et al. Comparison of gut microbiota in exclusively breast-fed and formula-fed babies: a study of 91 term infants. Sci Rep. (2020) 10:15792. doi: 10.1038/s41598-020-72635-x
57. Depner, M, Taft, DH, Kirjavainen, PV, Kalanetra, KM, Karvonen, AM, Peschel, S, et al. Maturation of the gut microbiome during the first year of life contributes to the protective farm effect on childhood asthma. Nat Med. (2020) 26:1766–75. doi: 10.1038/s41591-020-1095-x
58. Gibbs, BG, and Forste, R. Socioeconomic status, infant feeding practices and early childhood obesity. Pediatr Obes. (2014) 9:135–46. doi: 10.1111/j.2047-6310.2013.00155.x
59. Reyman, M, van Houten, MA, Watson, RL, Chu, MLJN, Arp, K, de Waal, WJ, et al. Effects of early-life antibiotics on the developing infant gut microbiome and resistome: a randomized trial. Nat Commun. (2022) 13:893. doi: 10.1038/s41467-022-28525-z
60. Patrick, DM, Sbihi, H, Dai, DLY, Al Mamun, A, Rasali, D, Rose, C, et al. Decreasing antibiotic use, the gut microbiota, and asthma incidence in children: evidence from population-based and prospective cohort studies. Lancet Respir Med. (2020) 8:1094–105. doi: 10.1016/S2213-2600(20)30052-7
61. Hoskinson, C, Medeleanu, MV, Reyna, ME, Dai, DLY, Chowdhury, B, Moraes, TJ, et al. Antibiotics taken within the first year of life are linked to infant gut microbiome disruption and elevated atopic dermatitis risk. J Allergy Clin Immunol. (2024) 154:131–42. doi: 10.1016/j.jaci.2024.03.025
62. Cho, I, Yamanishi, S, Cox, L, Methé, BA, Zavadil, J, Li, K, et al. Antibiotics in early life alter the murine colonic microbiome and adiposity. Nature. (2012) 488:621–6. doi: 10.1038/nature11400
63. Russell, SL, Gold, MJ, Reynolds, LA, Willing, BP, Dimitriu, P, Thorson, L, et al. Perinatal antibiotic-induced shifts in gut microbiota have differential effects on inflammatory lung diseases. J Allergy Clin Immunol. (2015) 135:100–109.e5. doi: 10.1016/j.jaci.2014.06.027
64. Zhang, Z, Wang, J, Wang, H, Li, Y, Jia, Y, Yi, M, et al. Association of infant antibiotic exposure and risk of childhood asthma: a meta-analysis. World Allergy Organ J. (2021) 14:100607. doi: 10.1016/j.waojou.2021.100607
65. Virta, L, Auvinen, A, Helenius, H, Huovinen, P, and Kolho, KL. Association of repeated exposure to antibiotics with the development of pediatric Crohn's disease--a nationwide, register-based Finnish case-control study. Am J Epidemiol. (2012) 175:775–84. doi: 10.1093/aje/kwr400
66. Korpela, K, Salonen, A, Virta, LJ, Kekkonen, RA, Forslund, K, Bork, P, et al. Intestinal microbiome is related to lifetime antibiotic use in Finnish pre-school children. Nat Commun. (2016) 7:10410. doi: 10.1038/ncomms10410
67. Ahmadizar, F, Vijverberg, SJH, Arets, HGM, de Boer, A, Lang, JE, Garssen, J, et al. Early-life antibiotic exposure increases the risk of developing allergic symptoms later in life: a meta-analysis. Allergy. (2018) 73:971–86. doi: 10.1111/all.13332
68. Miller, SA, Wu, RKS, and Oremus, M. The association between antibiotic use in infancy and childhood overweight or obesity: a systematic review and meta-analysis. Obes Rev. (2018) 19:1463–75. doi: 10.1111/obr.12717
69. Mangrio, E, Wremp, A, Moghaddassi, M, Merlo, J, Bramhagen, AC, and Rosvall, M. Antibiotic use among 8-month-old children in Malmö, Sweden--in relation to child characteristics and parental sociodemographic, psychosocial and lifestyle factors. BMC Pediatr. (2009) 9:31. doi: 10.1186/1471-2431-9-31
70. Jensen, JN, Bjerrum, L, Boel, J, Jarløv, JO, and Arpi, M. Parents' socioeconomic factors related to high antibiotic prescribing in primary health care among children aged 0-6 years in the Capital Region of Denmark. Scand J Prim Health Care. (2016) 34:274–81. doi: 10.1080/02813432.2016.1207145
71. Larsen, SB, Jensen, MLV, Bjerrum, L, Siersma, V, Bang, CW, and Jensen, JN. Trend in antibiotic prescription to children aged 0-6 years old in the capital region of Denmark between 2009 and 2018: differences between municipalities and association with socioeconomic composition. Eur J Gen Pract. (2021) 27:257–63. doi: 10.1080/13814788.2021.1965121
72. McGurn, A, Watchmaker, B, Adam, K, Ni, J, Babinski, P, Friedman, H, et al. Socioeconomic status and determinants of pediatric antibiotic use. Clin Pediatr (Phila). (2021) 60:32–41. doi: 10.1177/0009922820941629
73. Fink, G, D'Acremont, V, Leslie, HH, and Cohen, J. Antibiotic exposure among children younger than 5 years in low-income and middle-income countries: a cross-sectional study of nationally representative facility-based and household-based surveys. Lancet Infect Dis. (2020) 20:179–87. doi: 10.1016/S1473-3099(19)30572-9
74. Lee, GC, Reveles, KR, Attridge, RT, Lawson, KA, Mansi, IA, Lewis, JS, et al. Outpatient antibiotic prescribing in the United States: 2000 to 2010. BMC Med. (2014) 12:96. doi: 10.1186/1741-7015-12-96
75. King, LM, Bartoces, M, Fleming-Dutra, KE, Roberts, RM, and Hicks, LA. Changes in US outpatient antibiotic prescriptions from 2011-2016. Clin Infect Dis. (2020) 70:370–7. doi: 10.1093/cid/ciz225
76. Ekholuenetale, M, Tudeme, G, Onikan, A, and Ekholuenetale, CE. Socioeconomic inequalities in hidden hunger, undernutrition, and overweight among under-five children in 35 sub-Saharan Africa countries. J Egypt Public Health Assoc. (2020) 95:9. doi: 10.1186/s42506-019-0034-5
77. Barazzoni, R, and Gortan Cappellari, G. Double burden of malnutrition in persons with obesity. Rev Endocr Metab Disord. (2020) 21:307–13. doi: 10.1007/s11154-020-09578-1
78. Smith, MI, Yatsunenko, T, Manary, MJ, Trehan, I, Mkakosya, R, Cheng, J, et al. Gut microbiomes of Malawian twin pairs discordant for kwashiorkor. Science. (2013) 339:548–54. doi: 10.1126/science.1229000
79. Brown, EM, Wlodarska, M, Willing, BP, Vonaesch, P, Han, J, Reynolds, LA, et al. Diet and specific microbial exposure trigger features of environmental enteropathy in a novel murine model. Nat Commun. (2015) 6:7806. doi: 10.1038/ncomms8806
80. Littlejohn, PT, Metcalfe-Roach, A, Cardenas Poire, E, Holani, R, Bar-Yoseph, H, Fan, YM, et al. Multiple micronutrient deficiencies in early life cause multi-kingdom alterations in the gut microbiome and intrinsic antibiotic resistance genes in mice. Nat Microbiol. (2023) 8:2392–405. doi: 10.1038/s41564-023-01519-3
81. Camara, A, Konate, S, Tidjani Alou, M, Kodio, A, Togo, AH, Cortaredona, S, et al. Clinical evidence of the role of Methanobrevibacter smithii in severe acute malnutrition. Sci Rep. (2021) 11:5426. doi: 10.1038/s41598-021-84641-8
82. Tidjani Alou, M, Million, M, Traore, SI, Mouelhi, D, Khelaifia, S, Bachar, D, et al. Gut bacteria missing in severe acute malnutrition, can we identify potential probiotics by Culturomics? Front Microbiol. (2017) 8:899. doi: 10.3389/fmicb.2017.00899
83. Dinh, DM, Ramadass, B, Kattula, D, Sarkar, R, Braunstein, P, Tai, A, et al. Longitudinal analysis of the intestinal microbiota in persistently stunted young children in South India. PLoS One. (2016) 11:e0155405. doi: 10.1371/journal.pone.0155405
84. Subramanian, S, Huq, S, Yatsunenko, T, Haque, R, Mahfuz, M, Alam, MA, et al. Persistent gut microbiota immaturity in malnourished Bangladeshi children. Nature. (2014) 510:417–21. doi: 10.1038/nature13421
85. Raman, AS, Gehrig, JL, Venkatesh, S, Chang, HW, Hibberd, MC, Subramanian, S, et al. A sparse covarying unit that describes healthy and impaired human gut microbiota development. Science. (2019) 365:eaau4735. doi: 10.1126/science.aau4735
86. Gehrig, JL, Venkatesh, S, Chang, HW, Hibberd, MC, Kung, VL, Cheng, J, et al. Effects of microbiota-directed foods in gnotobiotic animals and undernourished children. Science. (2019) 365:eaau4732. doi: 10.1126/science.aau4732
87. Chen, RY, Mostafa, I, Hibberd, MC, Das, S, Mahfuz, M, Naila, NN, et al. A microbiota-directed food intervention for undernourished children. N Engl J Med. (2021) 384:1517–28. doi: 10.1056/NEJMoa2023294
88. Mills, JG, Brookes, JD, Gellie, NJC, Liddicoat, C, Lowe, AJ, Sydnor, HR, et al. Relating urban biodiversity to human health with the 'Holobiont' concept. Front Microbiol. (2019) 10:550. doi: 10.3389/fmicb.2019.00550
89. McCall, LI, Callewaert, C, Zhu, Q, Song, SJ, Bouslimani, A, Minich, JJ, et al. Home chemical and microbial transitions across urbanization. Nat Microbiol. (2020) 5:108–15. doi: 10.1038/s41564-019-0593-4
90. Rook, GA . Regulation of the immune system by biodiversity from the natural environment: an ecosystem service essential to health. Proc Natl Acad Sci USA. (2013) 110:18360–7. doi: 10.1073/pnas.1313731110
91. Strachan, DP . Hay fever, hygiene, and household size. BMJ. (1989) 299:1259–60. doi: 10.1136/bmj.299.6710.1259
92. Zuniga-Chaves, I, Eggers, S, Kates, AE, Safdar, N, Suen, G, and Malecki, KMC. Neighborhood socioeconomic status is associated with low diversity gut microbiomes and multi-drug resistant microorganism colonization. NPJ Biofilms Microbiomes. (2023) 9:61. doi: 10.1038/s41522-023-00430-3
93. Christensen, ED, Hjelmsø, MH, Thorsen, J, Shah, S, Redgwell, T, Poulsen, CE, et al. The developing airway and gut microbiota in early life is influenced by age of older siblings. Microbiome. (2022) 10:106. doi: 10.1186/s40168-022-01305-z
94. Tun, HM, Konya, T, Takaro, TK, Brook, JR, Chari, R, Field, CJ, et al. Exposure to household furry pets influences the gut microbiota of infant at 3-4 months following various birth scenarios. Microbiome. (2017) 5:40. doi: 10.1186/s40168-017-0254-x
95. Seppo, AE, Bu, K, Jumabaeva, M, Thakar, J, Choudhury, RA, Yonemitsu, C, et al. Infant gut microbiome is enriched with Bifidobacterium longum ssp. infantis in old order Mennonites with traditional farming lifestyle. Allergy. (2021) 76:3489–503. doi: 10.1111/all.14877
96. Bailey, MJ, Holzhausen, EA, Morgan, ZEM, Naik, N, Shaffer, JP, Liang, D, et al. Postnatal exposure to ambient air pollutants is associated with the composition of the infant gut microbiota at 6-months of age. Gut Microbes. (2022) 14:2105096. doi: 10.1080/19490976.2022.2105096
97. Gacesa, R, Kurilshikov, A, Vich Vila, A, Sinha, T, Klaassen, MAY, Bolte, LA, et al. Environmental factors shaping the gut microbiome in a Dutch population. Nature. (2022) 604:732–9. doi: 10.1038/s41586-022-04567-7
98. Ege, MJ, Mayer, M, Normand, AC, Genuneit, J, Cookson, WO, Braun-Fahrländer, C, et al. Exposure to environmental microorganisms and childhood asthma. N Engl J Med. (2011) 364:701–9. doi: 10.1056/NEJMoa1007302
99. Kirjavainen, PV, Karvonen, AM, Adams, RI, Täubel, M, Roponen, M, Tuoresmäki, P, et al. Farm-like indoor microbiota in non-farm homes protects children from asthma development. Nat Med. (2019) 25:1089–95. doi: 10.1038/s41591-019-0469-4
100. Spielau, U, Vogel, M, Körner, A, Kiess, W, and Poulain, T. Composition and culture of eating (CoCu) pregnancy: a new short questionnaire to evaluate diet composition and culture of eating during pregnancy. Public Health Nutr. (2021) 24:6227–35. doi: 10.1017/S1368980021002445
101. Savard, C, Lemieux, S, Carbonneau, É, Provencher, V, Gagnon, C, Robitaille, J, et al. Trimester-specific assessment of diet quality in a sample of Canadian pregnant women. Int J Environ Res Public Health. (2019) 16:311. doi: 10.3390/ijerph16030311
102. Li, M, Grewal, J, Hinkle, SN, Yisahak, SF, Grobman, WA, Newman, RB, et al. Healthy dietary patterns and common pregnancy complications: a prospective and longitudinal study. Am J Clin Nutr. (2021) 114:1229–37. doi: 10.1093/ajcn/nqab145
103. Tian, M, Li, Q, Zheng, T, Yang, S, Chen, F, Guan, W, et al. Maternal microbe-specific modulation of the offspring microbiome and development during pregnancy and lactation. Gut Microbes. (2023) 15:2206505. doi: 10.1080/19490976.2023.2206505
104. Chu, DM, Antony, KM, Ma, J, Prince, AL, Showalter, L, Moller, M, et al. The early infant gut microbiome varies in association with a maternal high-fat diet. Genome Med. (2016) 8:77. doi: 10.1186/s13073-016-0330-z
105. Sonnenburg, ED, Smits, SA, Tikhonov, M, Higginbottom, SK, Wingreen, NS, and Sonnenburg, JL. Diet-induced extinctions in the gut microbiota compound over generations. Nature. (2016) 529:212–5. doi: 10.1038/nature16504
106. Liu, X, Li, X, Xia, B, Jin, X, Zou, Q, Zeng, Z, et al. High-fiber diet mitigates maternal obesity-induced cognitive and social dysfunction in the offspring via gut-brain axis. Cell Metab. (2021) 33:923–938.e6. doi: 10.1016/j.cmet.2021.02.002
107. Sikder, MAA, Rashid, RB, Ahmed, T, Sebina, I, Howard, DR, Ullah, MA, et al. Maternal diet modulates the infant microbiome and intestinal Flt3L necessary for dendritic cell development and immunity to respiratory infection. Immunity. (2023) 56:1098–1114.e10. doi: 10.1016/j.immuni.2023.03.002
108. Stokholm, J, Schjørring, S, Pedersen, L, Bischoff, AL, Følsgaard, N, Carson, CG, et al. Prevalence and predictors of antibiotic administration during pregnancy and birth. PLoS One. (2013) 8:e82932. doi: 10.1371/journal.pone.0082932
109. Pisa, FE, Casetta, A, Clagnan, E, Michelesio, E, Vecchi Brumatti, L, and Barbone, F. Medication use during pregnancy, gestational age and date of delivery: agreement between maternal self-reports and health database information in a cohort. BMC Pregnancy Childbirth. (2015) 15:310. doi: 10.1186/s12884-015-0745-3
110. Schmiege, D, Evers, M, Kistemann, T, and Falkenberg, T. What drives antibiotic use in the community? A systematic review of determinants in the human outpatient sector. Int J Hyg Environ Health. (2020) 226:113497. doi: 10.1016/j.ijheh.2020.113497
111. Huang, H, Jiang, J, Wang, X, Jiang, K, and Cao, H. Exposure to prescribed medication in early life and impacts on gut microbiota and disease development. EClinicalMedicine. (2024) 68:102428. doi: 10.1016/j.eclinm.2024.102428
112. Gardemeister, S, Skogberg, K, Saisto, T, Salonen, A, de Vos, WM, Korpela, K, et al. Cross-sectional study of the proportion of antibiotic use during childbirth in full-term deliveries in Finland. BMC Pregnancy Childbirth. (2023) 23:50. doi: 10.1186/s12884-023-05368-0
113. Petersen, I, Gilbert, R, Evans, S, Ridolfi, A, and Nazareth, I. Oral antibiotic prescribing during pregnancy in primary care: UK population-based study. J Antimicrob Chemother. (2010) 65:2238–46. doi: 10.1093/jac/dkq307
114. Dierikx, TH, Visser, DH, Benninga, MA, van Kaam, AHLC, de Boer, NKH, de Vries, R, et al. The influence of prenatal and intrapartum antibiotics on intestinal microbiota colonisation in infants: a systematic review. J Infect. (2020) 81:190–204. doi: 10.1016/j.jinf.2020.05.002
115. Nogacka, A, Salazar, N, Suárez, M, Milani, C, Arboleya, S, Solís, G, et al. Impact of intrapartum antimicrobial prophylaxis upon the intestinal microbiota and the prevalence of antibiotic resistance genes in vaginally delivered full-term neonates. Microbiome. (2017) 5:93. doi: 10.1186/s40168-017-0313-3
116. Navarro-Rosenblatt, D, and Garmendia, ML. Maternity leave and its impact on breastfeeding: a review of the literature. Breastfeed Med. (2018) 13:589–97. doi: 10.1089/bfm.2018.0132
117. Katz, J, Crean, HF, Cerulli, C, and Poleshuck, EL. Material hardship and mental health symptoms among a predominantly low income sample of pregnant women seeking prenatal care. Matern Child Health J. (2018) 22:1360–7. doi: 10.1007/s10995-018-2518-x
118. Demers, CH, Bagonis, MM, Al-Ali, K, Garcia, SE, Styner, MA, Gilmore, JH, et al. Exposure to prenatal maternal distress and infant white matter neurodevelopment. Dev Psychopathol. (2021) 33:1526–38. doi: 10.1017/s0954579421000742
119. Van den Bergh, BRH, van den Heuvel, MI, Lahti, M, Braeken, M, de Rooij, SR, Entringer, S, et al. Prenatal developmental origins of behavior and mental health: the influence of maternal stress in pregnancy. Neurosci Biobehav Rev. (2020) 117:26–64. doi: 10.1016/j.neubiorev.2017.07.003
120. Galley, JD, Mashburn-Warren, L, Blalock, LC, Lauber, CL, Carroll, JE, Ross, KM, et al. Maternal anxiety, depression and stress affects offspring gut microbiome diversity and bifidobacterial abundances. Brain Behav Immun. (2023) 107:253–64. doi: 10.1016/j.bbi.2022.10.005
121. Aatsinki, AK, Keskitalo, A, Laitinen, V, Munukka, E, Uusitupa, HM, Lahti, L, et al. Maternal prenatal psychological distress and hair cortisol levels associate with infant fecal microbiota composition at 2.5 months of age. Psychoneuroendocrinology. (2020) 119:104754. doi: 10.1016/j.psyneuen.2020.104754
122. Jašarević, E, Howard, CD, Misic, AM, Beiting, DP, and Bale, TL. Stress during pregnancy alters temporal and spatial dynamics of the maternal and offspring microbiome in a sex-specific manner. Sci Rep. (2017) 7:44182. doi: 10.1038/srep44182
123. Jašarević, E, Howard, CD, Morrison, K, Misic, A, Weinkopff, T, Scott, P, et al. The maternal vaginal microbiome partially mediates the effects of prenatal stress on offspring gut and hypothalamus. Nat Neurosci. (2018) 21:1061–71. doi: 10.1038/s41593-018-0182-5
124. Hiscock, R, Bauld, L, Amos, A, Fidler, JA, and Munafò, M. Socioeconomic status and smoking: a review. Ann N Y Acad Sci. (2012) 1248:107–23. doi: 10.1111/j.1749-6632.2011.06202.x
125. Adibelli, D, and Kirca, N. The relationship between gestational active and passive smoking and early postpartum complications. J Matern Fetal Neonatal Med. (2020) 33:2473–9. doi: 10.1080/14767058.2020.1763294
126. Philips, EM, Santos, S, Trasande, L, Aurrekoetxea, JJ, Barros, H, von Berg, A, et al. Changes in parental smoking during pregnancy and risks of adverse birth outcomes and childhood overweight in Europe and North America: an individual participant data meta-analysis of 229,000 singleton births. PLoS Med. (2020) 17:e1003182. doi: 10.1371/journal.pmed.1003182
127. AM, AS, Tan, PT, Soh, SE, Goh, A, Shek, LP, van Bever, HP, et al. Tobacco smoke exposure and respiratory morbidity in young children. Tob Control. (2016) 25:e75–82. doi: 10.1136/tobaccocontrol-2015-052383
128. McLean, C, Jun, S, and Kozyrskyj, A. Impact of maternal smoking on the infant gut microbiota and its association with child overweight: a scoping review. World J Pediatr. (2019) 15:341–9. doi: 10.1007/s12519-019-00278-8
129. Peng, Y, Tun, HM, Ng, SC, Wai, HK, Zhang, X, Parks, J, et al. Maternal smoking during pregnancy increases the risk of gut microbiome-associated childhood overweight and obesity. Gut Microbes. (2024) 16:2323234. doi: 10.1080/19490976.2024.2323234
130. Dowd, JB, and Renson, A. “Under the skin” and into the gut: social epidemiology of the microbiome. Curr Epidemiol Rep. (2018) 5:432–41. doi: 10.1007/s40471-018-0167-7
131. Ishaq, SL, Rapp, M, Byerly, R, McClellan, LS, O'Boyle, MR, Nykanen, A, et al. Framing the discussion of microorganisms as a facet of social equity in human health. PLoS Biol. (2019) 17:e3000536. doi: 10.1371/journal.pbio.3000536
132. Amato, KR, Arrieta, MC, Azad, MB, Bailey, MT, Broussard, JL, Bruggeling, CE, et al. The human gut microbiome and health inequities. Proc Natl Acad Sci USA. (2021) 118:e2017947118. doi: 10.1073/pnas.2017947118
133. Mendelson, M, and Matsoso, MP. The World Health Organization global action plan for antimicrobial resistance. S Afr Med J. (2015) 105:325. doi: 10.7196/samj.9644
134. Park, M, Deo, D, Tan, SPP, Bahtigur, N, and Lwin, N. The effectiveness of an antimicrobial stewardship program in an Australian rural hospital. Aust J Rural Health. (2023) 31:522–31. doi: 10.1111/ajr.12979
135. Alawi, MM, Tashkandi, WA, Basheikh, MA, Warshan, FM, Ghobara, HA, Ramos, RB, et al. Effectiveness of antimicrobial stewardship program in long-term care: A five-year prospective single-center study. Interdiscip Perspect Infect Dis. (2022) 2022:8140429–12. doi: 10.1155/2022/8140429
136. Harun, MGD, Sumon, SA, Hasan, I, Akther, FM, Islam, MS, and Anwar, MMU. Barriers, facilitators, perceptions and impact of interventions in implementing antimicrobial stewardship programs in hospitals of low-middle and middle countries: a scoping review. Antimicrob Resist Infect Control. (2024) 13:8. doi: 10.1186/s13756-024-01369-6
137. Nixarlidou, E, Margioula-Siarkou, C, Almperis, A, Vavoulidis, E, Laganà, AS, Dinas, K, et al. Clinical significance and main parameters promoting the breast-feeding strategy (review). Med Int (Lond). (2024) 4:14. doi: 10.3892/mi.2024.138
138. Kopp, SJ, Kelly, EA, and DeFranco, EA. Influence of social determinants of health on breastfeeding intent in the United States. Birth. (2023) 50:858–67. doi: 10.1111/birt.12740
139. Wagner, S, Kersuzan, C, Gojard, S, Tichit, C, Nicklaus, S, Thierry, X, et al. Breastfeeding initiation and duration in France: the importance of intergenerational and previous maternal breastfeeding experiences - results from the nationwide ELFE study. Midwifery. (2019) 69:67–75. doi: 10.1016/j.midw.2018.10.020
140. Çerçer, Z, and Nazik, E. The effects of the breastfeeding problems management model on breastfeeding problems, breastfeeding motivation and breastfeeding success: a randomized controlled trial. J Pediatr Nurs. (2023) 73:e116–24. doi: 10.1016/j.pedn.2023.07.021
141. Gonzalez-Darias, A, Diaz-Gomez, NM, Rodriguez-Martin, S, Hernandez-Perez, C, and Aguirre-Jaime, A. ‘Supporting a first-time mother’: assessment of success of a breastfeeding promotion programme. Midwifery. (2020) 85:102687. doi: 10.1016/j.midw.2020.102687
142. Brown, A . Breastfeeding as a public health responsibility: a review of the evidence. J Hum Nutr Diet. (2017) 30:759–70. doi: 10.1111/jhn.12496
143. Borewicz, K, Suarez-Diez, M, Hechler, C, Beijers, R, de Weerth, C, Arts, I, et al. The effect of prebiotic fortified infant formulas on microbiota composition and dynamics in early life. Sci Rep. (2019) 9:2434. doi: 10.1038/s41598-018-38268-x
144. Estorninos, E, Lawenko, RB, Palestroque, E, Sprenger, N, Benyacoub, J, Kortman, GAM, et al. Term infant formula supplemented with milk-derived oligosaccharides shifts the gut microbiota closer to that of human milk-fed infants and improves intestinal immune defense: a randomized controlled trial. Am J Clin Nutr. (2022) 115:142–53. doi: 10.1093/ajcn/nqab336
145. Vangay, P, Johnson, AJ, Ward, TL, Al-Ghalith, GA, Shields-Cutler, RR, Hillmann, BM, et al. US immigration westernizes the human gut microbiome. Cell. (2018) 175:962–972.e10. doi: 10.1016/j.cell.2018.10.029
146. Prescott, SL . A butterfly flaps its wings: extinction of biological experience and the origins of allergy. Ann Allergy Asthma Immunol. (2020) 125:528–34. doi: 10.1016/j.anai.2020.05.025
147. Chen, CC, and Chiu, CH. Current and future applications of fecal microbiota transplantation for children. Biom J. (2022) 45:11–8. doi: 10.1016/j.bj.2021.11.004
148. Korpela, K, Helve, O, Kolho, KL, Saisto, T, Skogberg, K, Dikareva, E, et al. Maternal fecal microbiota transplantation in cesarean-born infants rapidly restores Normal gut microbial development: a proof-of-concept study. Cell. (2020) 183:324–334.e5. doi: 10.1016/j.cell.2020.08.047
149. Wharton, KR, and Birsner, ML. Committee opinion no. 725 summary: vaginal seeding. Obstet Gynecol. (2017) 130:1178–9. doi: 10.1097/AOG.0000000000002396
150. Casaburi, G, Duar, RM, Brown, H, Mitchell, RD, Kazi, S, Chew, S, et al. Metagenomic insights of the infant microbiome community structure and function across multiple sites in the United States. Sci Rep. (2021) 11:1472. doi: 10.1038/s41598-020-80583-9
151. Chichlowski, M, Shah, N, Wampler, JL, Wu, SS, and Vanderhoof, JA. Bifidobacterium longum subspecies infantis (B. infantis) in pediatric nutrition: current state of knowledge. Nutrients. (2020) 12:6. doi: 10.3390/nu12061581
152. Sakanaka, M, Hansen, ME, Gotoh, A, Katoh, T, Yoshida, K, Odamaki, T, et al. Evolutionary adaptation in fucosyllactose uptake systems supports bifidobacteria-infant symbiosis. Sci Adv. (2019) 5:eaaw7696. doi: 10.1126/sciadv.aaw7696
153. Batta, VK, Rao, SC, and Patole, SK. Bifidobacterium infantis as a probiotic in preterm infants: a systematic review and meta-analysis. Pediatr Res. (2023) 94:1887–905. doi: 10.1038/s41390-023-02716-w
154. Mills, J, Bissett, A, Gellie, N, Lowe, A, Selway, C, Thomas, T, et al. Revegetation of urban green space rewilds soil microbiotas with implications for human health and urban design. Restor Ecol. (2020) 28:S322–S334. doi: 10.1111/rec.13175
155. Henrick, BM, Rodriguez, L, Lakshmikanth, T, Pou, C, Henckel, E, Arzoomand, A, et al. Bifidobacteria-mediated immune system imprinting early in life. Cell. (2021) 184:3884–3898.e11. doi: 10.1016/j.cell.2021.05.030
156. Zaidi, AZ, Moore, SE, and Okala, SG. Impact of maternal nutritional supplementation during pregnancy and lactation on the infant gut or breastmilk microbiota: a systematic review. Nutrients. (2021) 13:1137. doi: 10.3390/nu13041137
157. Murphy, R, Morgan, XC, Wang, XY, Wickens, K, Purdie, G, Fitzharris, P, et al. Eczema-protective probiotic alters infant gut microbiome functional capacity but not composition: sub-sample analysis from a RCT. Benef Microbes. (2019) 10:5–17. doi: 10.3920/BM2017.0191
158. Dotterud, CK, Avershina, E, Sekelja, M, Simpson, MR, Rudi, K, Storrø, O, et al. Does maternal perinatal probiotic supplementation Alter the intestinal microbiota of mother and child? J Pediatr Gastroenterol Nutr. (2015) 61:200–7. doi: 10.1097/MPG.0000000000000781
159. Singh, A, Sarangi, AN, Goel, A, Srivastava, R, Bhargava, R, Gaur, P, et al. Effect of administration of a probiotic preparation on gut microbiota and immune response in healthy women in India: an open-label, single-arm pilot study. BMC Gastroenterol. (2018) 18:85. doi: 10.1186/s12876-018-0819-6
160. Roslund, MI, Parajuli, A, Hui, N, Puhakka, R, Grönroos, M, Soininen, L, et al. A placebo-controlled double-blinded test of the biodiversity hypothesis of immune-mediated diseases: environmental microbial diversity elicits changes in cytokines and increase in T regulatory cells in young children. Ecotoxicol Environ Saf. (2022) 242:113900. doi: 10.1016/j.ecoenv.2022.113900
Keywords: socioeconomic status, health disparity, SES inequity, early-life exposures, intergenerational factors, microbiota
Citation: Dai DLY, Petersen C and Turvey SE (2024) Reduce, reinforce, and replenish: safeguarding the early-life microbiota to reduce intergenerational health disparities. Front. Public Health. 12:1455503. doi: 10.3389/fpubh.2024.1455503
Received: 27 June 2024; Accepted: 02 October 2024;
Published: 23 October 2024.
Edited by:
Jaime Díaz-Zúñiga, University of Chile, ChileReviewed by:
Laura Weyrich, The Pennsylvania State University (PSU), United StatesCopyright © 2024 Dai, Petersen and Turvey. This is an open-access article distributed under the terms of the Creative Commons Attribution License (CC BY). The use, distribution or reproduction in other forums is permitted, provided the original author(s) and the copyright owner(s) are credited and that the original publication in this journal is cited, in accordance with accepted academic practice. No use, distribution or reproduction is permitted which does not comply with these terms.
*Correspondence: Stuart E. Turvey, c3R1cnZleUBiY2Noci5jYQ==
Disclaimer: All claims expressed in this article are solely those of the authors and do not necessarily represent those of their affiliated organizations, or those of the publisher, the editors and the reviewers. Any product that may be evaluated in this article or claim that may be made by its manufacturer is not guaranteed or endorsed by the publisher.
Research integrity at Frontiers
Learn more about the work of our research integrity team to safeguard the quality of each article we publish.