- 1Department of Environmental Health College of Medicine and Health Sciences, Wollo University, Dessie, Ethiopia
- 2Department of Economic, College of Management and Economics, Wollo University, Dessie, Ethiopia
- 3Department of Epidemiology and Biostatistics, Institute of Public Health, College of Medicine and Health Sciences, University of Gondar, Gondar, Ethiopia
- 4Department of Health System and Management, School of Public Health, College of Medicine and Health Sciences, Wollo University, Dessie, Ethiopia
- 5National Center for Epidemiology and Population Health, The Australia National University, Dessie, Ethiopia
- 6National Center for Epidemiology and Population Health, The Australia National University, Canberra, ACT, Australia
Introduction: Antimicrobial resistance is a major global public health concern, especially in low-resource settings. In low- and middle-income countries, the existing evidence about antimicrobial resistance in drinking water is inconsistence and not comprehensive. Therefore, this study aimed to estimate the pooled prevalence of antimicrobial resistance profiles of Escherichia coli from drinking water in low- and middle-income countries.
Methods: This study was conducted using comprehensive literature searches using various databases such as PubMed, Scientific Direct, HINARI, and Google Scholar. Data extraction was performed using Microsoft Excel and exported to STATA 14/SE software for analysis. We used the Joanna Briggs Institute’s quality appraisal tool to ensure the quality of the included studies. A random effects model was employed to estimate the pooled prevalence. Publication bias was evaluated using funnel plots and Egger’s regression test. Subgroup and sensitivity analysis were also conducted in this study.
Results: The study found that the pooled prevalence of Escherichia coli isolates in drinking water was 37.94% (95% CI: 26.73–49.13). The prevalence of multidrug resistance was 43.65% (95% CI: 31.15–56.15). Regarding specific antimicrobials, the pooled resistance levels of Escherichia coli were 54.65% (95% CI: 41.35–67.96) against contrimoxazole, followed by 48.64% (95% CI: −3.6–101) against amoxicillin and 48% (95% CI: −18.1–114.2) against cefuroxime.
Conclusion: The findings indicated a significant prevalence of antimicrobial resistance of Escherichia coli isolated from drinking water and its multidrug resistance. To address this issue, it recommends focusing on improving basic hygiene and sanitation practices and enhancing water and wastewater treatment systems.
Systematic review registration: Identifier CRD42024533592.
1 Introduction
Antimicrobial Resistance (AMR) occurs when microorganisms such as bacteria, viruses, fungi, and parasites no longer respond to antimicrobials, making infections difficult to treat (1). AMR occurs through genetic changes that occur naturally. This resistance can occur in humans, animals, and the environment (air, water, and soil). The drivers of AMR include misuse and overuse of antimicrobials, lack of access to clean Water, Sanitation and Hygiene (WASH), poor Infection Prevention and Control (IPC) practices, etc. (2, 3).
In our interconnected world, AMR has gained global attention as a significant public health challenge (4). The World Health Organization (WHO) has recognized AMR as one of the top ten global health threats (5). Additionally, AMR has been identified as a significant hurdle and one of the foremost challenges in attaining the Sustainable Development Goals (SDGs) (6–8). Consequently, addressing the escalating threat of AMR necessitates the implementation of a One-Health initiatives (9). One Health is a comprehensive and integrated approach that recognizes the interconnected nature and mutual dependence of the health of humans, animals, and the environment (10, 11).
In 2019, global reports indicated that 1.23 million deaths were attributed directly to AMR, and in addition, 4.95 million deaths were indirectly attributed to AMR (6). Disturbingly, projections suggest that by the year 2050, AMR could potentially lead to up to 10 million deaths per year worldwide (12). The impact of AMR is more severe in Low- and Middle-Income Countries (LMICs) where limited resources and inadequate implementation of WASH measures prevail. Insufficient sanitation facilities in these regions can contribute to water contamination and facilitate the transmission and spread of AMR (2, 13).
As per the United Nations, access to safe water and sanitation is recognized as a basic human right (14). However, AMR has emerged as a concerning contaminant in drinking water. Water plays a main role in the dissemination of AMR within the environment (15). Therefore, it is vital to establish a clean water supply system while improving the use of disinfectant chemicals or exploring effective options. This approach is important for improving water quality at the point of use and reducing the possible transmission of resistance bacteria through water sources (16).
Current treatment technologies used in water and wastewater treatment plants primarily focus on reducing physical and chemical contaminants, but they often provide limited removal of biological contaminants such as AMR (17). Moreover, there is often insufficient monitoring and assessment of AMR following water treatment (18). The water distribution system is recognized as a complex system, posing challenges for the inactivation and treatment of AMR. Despite the availability of advanced water treatment technologies like membrane filtration, activated carbon filtration, and advanced oxidation, these methods may not effectively treat AMR (19, 20). Enteric bacterial pathogens, particularly Escherichia coli (E. coli) isolates originating from drinking water, are a significant public health concern for humans (21, 22). The WHO has identified E. coli as a top priority pathogen and a main contributor to the AMR burden (23).
The identification of E. coli in drinking water samples in LMICs has gained increasing importance due to its potential risks to public health. Mahmud et al. (24) in Bangladesh reported that drinking water samples are sources of pathogenic E.coli. Many studies have revealed the occurrence of E. coli in drinking water samples in LMICs, with isolation rates ranging from 5.3% in Egypt (25) to 79.6% in Ghana (26). E. coli isolates from drinking water have shown resistance to antimicrobial agents such as cotrimoxazole, amoxicillin, ampicillin, and tetracycline (27–30). Multidrug resistance (MDR) has been observed among E. coli isolates from drinking water, with prevalence ranging from 19.7% in Peru (27) to 80% in Ethiopia (31).
The emergence and widespread prevalence of AMR present a significant challenge, especially in LMICs (32, 33). Although various countries worldwide have implemented antimicrobial stewardship programs to tackle the issue of AMR (17), and the WHO has launched a global AMR surveillance system (34). There are multiple studies conducted about AMR in health care settings, but there is limited evidence about the general environment, like drinking water, particularly in LMICs (35). Despite limited and inconsistent evidence, to the best of our literature search, no systematic review and meta-analysis has been conducted from a One Health perspective to examine the AMR profiles of E. coli isolates from drinking water in LMICs.
Therefore, the aim of this study is to estimate the overall prevalence of E.coli isolated from drinking water in LMICs. Through this review, the study aims to generate comprehensive evidence regarding the AMR profiles of E. coli isolates from drinking water in LMICs. This research will contribute to the One Health approach and support the attainment of the SDGs. Additionally, the findings of this study can inform decision-making processes and increase awareness among stakeholders and policymakers. Ultimately, the study has the potential to drive action and facilitate necessary interventions to tackle AMR in drinking water systems.
2 Methods
2.1 Study setting and protocol of registration
The guidelines for updated Preferred Reporting Items for Systematic Reviews and Meta-Analysis (PRISMA) were used for this study (36) (Figure 1). The review protocol for this study was registered in the International Prospective Register of Systematic Reviews (PROSPERO) with the record id CRD42024533592. This study was conducted in LMICs, following the list of World Bank data (37).
2.2 Sources of information and search strategies
A systematic literature search was undertaken using a database of PubMed, Scientific Direct, HINARI, and Google Scholar. For example, for the PubMed search, key terms were used in combination with the Boolean operators “AND” and “OR.” Apart from conducting an electronic database search, additional articles were obtained by searching the gray literature through direct Google searches and by examining the bibliographies of the included articles.
2.3 Eligibility criteria
Inclusion criteria: this review included studies that fulfilled the following criteria.
a. Population: articles conducted specifically on drinking water.
b. Outcomes: articles reported the quantitative outcome of E.coli isolates (%), selected antimicrobial agents (%), and MDR of E.coli (%) in samples of drinking water.
c. Study design: any observational studies (cross-sectional, case control, and cohort).
d. Study setting: studies conducted in LMICs.
e. Time frame: all studies reported from January, 02, 2000 to April, 30, 2024.
f. Language of published studies: articles written in English.
g. Publication issue: both published and unpublished studies.
Exclusion criteria: studies that were not fully accessible despite three personal email contacts with the primary and corresponding authors, and studies that did not clearly show us the outcome interest of the study were excluded. Furthermore, certain types of research articles, including letters to editors, qualitative studies, systematic reviews, short communications, and commentaries, were not considered.
2.4 Study selection
Two investigators, BD and YT, conducted independent screenings of articles based on their titles, abstracts, and full texts to identify eligible articles. They followed pre-established criteria during this process. The screened studies were then combined by the two investigators, and any disagreements that arise during data abstraction and selection were resolved by discussions and the involvement of a third investigator (CD).
2.5 Data extraction and management
The format of data extraction for this study included author name, year of publication, country of the study, number of samples, number of E.coli isolates, % of E.coli isolates, % MDR of E.coli isolates, and risk of bias, these details were organized in a table format (Table 1). To collect articles and remove duplicate studies, Zotero Reference Manager was used. Additionally, the updated PRISMA checklist was employed to effectively summarize the study conditions (36) (Supplementary file 1).
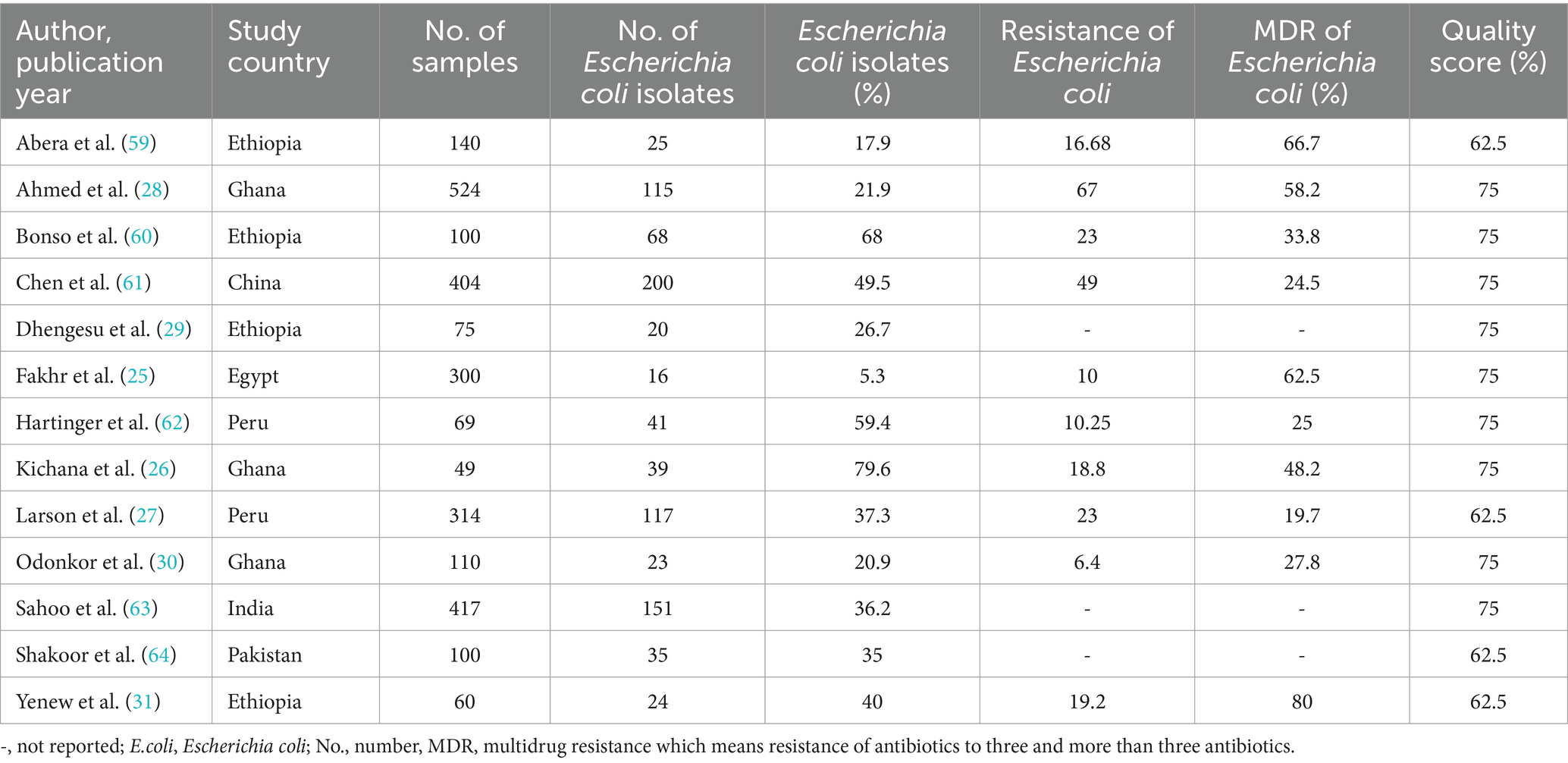
Table 1. Summary of the included articles in AMR profiles of Escherichia coli isolates from drinking water in LMICs, 2024.
2.6 Quality assessment of the studies
The quality appraisal tools of Joanna Briggs Institute (JBI) for analytical cross-sectional studies were used to assess the quality of the included studies (38). The quality of the articles was independently assessed by the two reviewers (BD and YT). Eight criteria were used to assess the quality of each article. The assessment options were categorized as yes, no, unclear, or not applicable. The risk of bias was classified as low (total score between 6 to 8), moderate (total score between 3 to 5), and high (total score between 0 to 2). Finally, articles scored more than 50% were considered in this study (39, 40), detailed assessment in Supplementary file 2.
2.7 Outcome of interest
This study has three main outcomes:
• The pooled prevalence of E.coli isolates in drinking water in LMICs, expressed as (%).
• The pooled prevalence of selected antimicrobial agents against E.coli in drinking water in LMICs, expressed as (%).
• The pooled prevalence of MDR of E.coli isolates in drinking water in LMICs, expressed as (%)
2.8 Statistical methods and data analysis
Microsoft Excel was used to extract data and transported to STATA version-14 for analysis. Index of heterogeneity (I2 statistics) was used to assess heterogeneity among the included articles, where values of 25–50%, 50–75%, and > 75% indicated low, moderate, and high heterogeneity, respectively (41). The metaprop command in STATA was used to estimate the pooled prevalence. Subgroup analysis to explore potential variations in the pooled prevalence in this study was conducted using study countries, sample size, and study year. In this study, the effect of each study on the estimated pooled results was assessed using sensitivity analysis. A funnel plot test and Egger’s regression test with a significance level of p < 0.05 as the cut point were used to ensure the presence of publication bias. To identify a possible heterogeneity source, a univariate meta-regression was employed. Finally, the findings of this study were presented using tables, figures, a forest plot, and descriptive text.
3 Results
3.1 Overview of search process
Using a database and other methods of search, a total of 22,244 studies were identified. After duplicate records were removed, 3,676 records were screened for this review. According to the records, only 1,101 reports were sought for retrieval. After being identified for retrieval, 343 reports were evaluated for eligibility. Following eligibility, a total of 332 studies were excluded due to differences in outcome interest and population differences. Ultimately, a total of 11 studies were included in this review from database sources. In addition to the database sources, 2 studies were included in this review from other sources. Finally, a total of 13 articles were included in this study, as presented in the PRISMA flowchart (Figure 1).
3.2 Characteristics of the eligible studies
All the included articles were cross-sectional studies. In this review, a total of 2,662 drinking water samples were included. Most of the studies were conducted in Ethiopia (n = 4) and Ghana (n = 3). The included studies were conducted between 2012 and 2023. In this study, the number of E.coli isolates was (n = 874), and the number of MDR of E.coli isolates was (n = 243.33). The E.coli isolates in this review ranged from 5.3% in Egypt (25) to 79.6% in Ghana (26), and the MDR of E.coli was found between 19.7% in Peru (27) and 80% in Ethiopia (31). All the included articles were categorized under moderate levels of risk of bias (Table 1).
3.3 The pooled prevalence of Escherichia coli isolates from drinking water in LMICs
The pooled prevalence of E.coli isolates using a random-effect model was estimated at 37.94% (95% CI: 26.75–49.13), with high heterogeneity (I2 = 98%, p-value < 0.001) (Figure 2). A sub-group analysis based on study countries, year of publication, and sample size was performed to assess the heterogeneity sources, which were presented in Table 2. The highest pooled prevalence of 49.5% (95% CI: 44.63–54.38) was observed in China, and the lowest pooled prevalence was estimated at 5.33% (95% CI: 2.79–7.88) in Egypt. Heterogeneity was highest among studies conducted in Ghana (I2 = 97.9%), followed by Ethiopia (I2 = 96.3%). Based on the year of publication, a study conducted in above 2018 was estimated at 43.87% (95% CI: 30–57.75) with (I2 = 96.4%, p-value < 0.001) and 28.72% (95% CI: 9.50–47.94) with (I2 = 98.8%, p-value < 0.001) for a study conducted in 2018 and below. In addition, a study conducted with a sample size of ≤ 200 found 43.22% (95% CI: 27.12–59.32) with (I2 = 95.9%, p-value < 0.001) and sample size of >200 found 29.97% (95% CI: 13.42–46.53) with (I2 = 98.9%, p-value < 0.001).
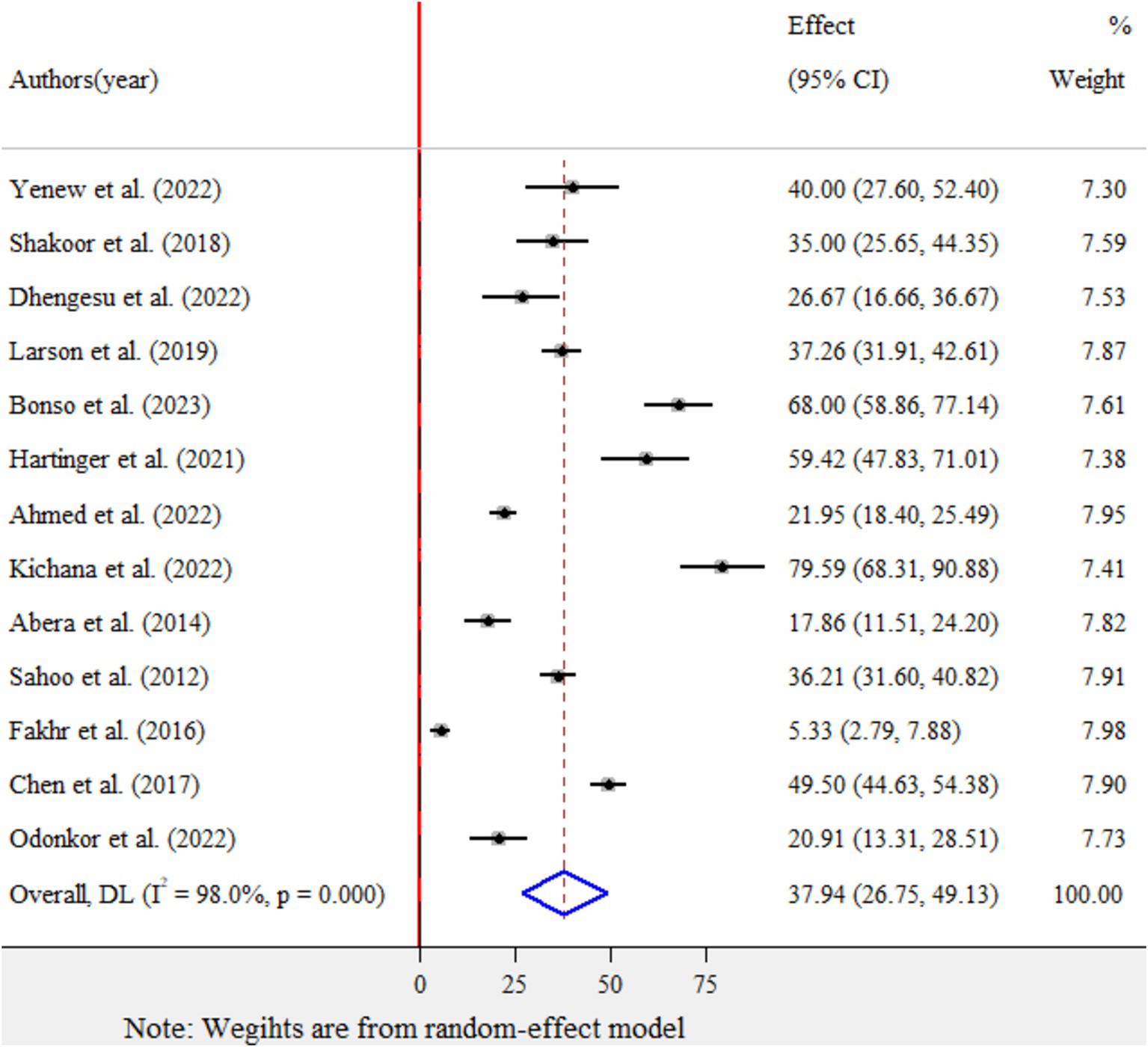
Figure 2. Forest plot for the pooled prevalence of Escherichia coli isolates from drinking water in LMICs, 2024.
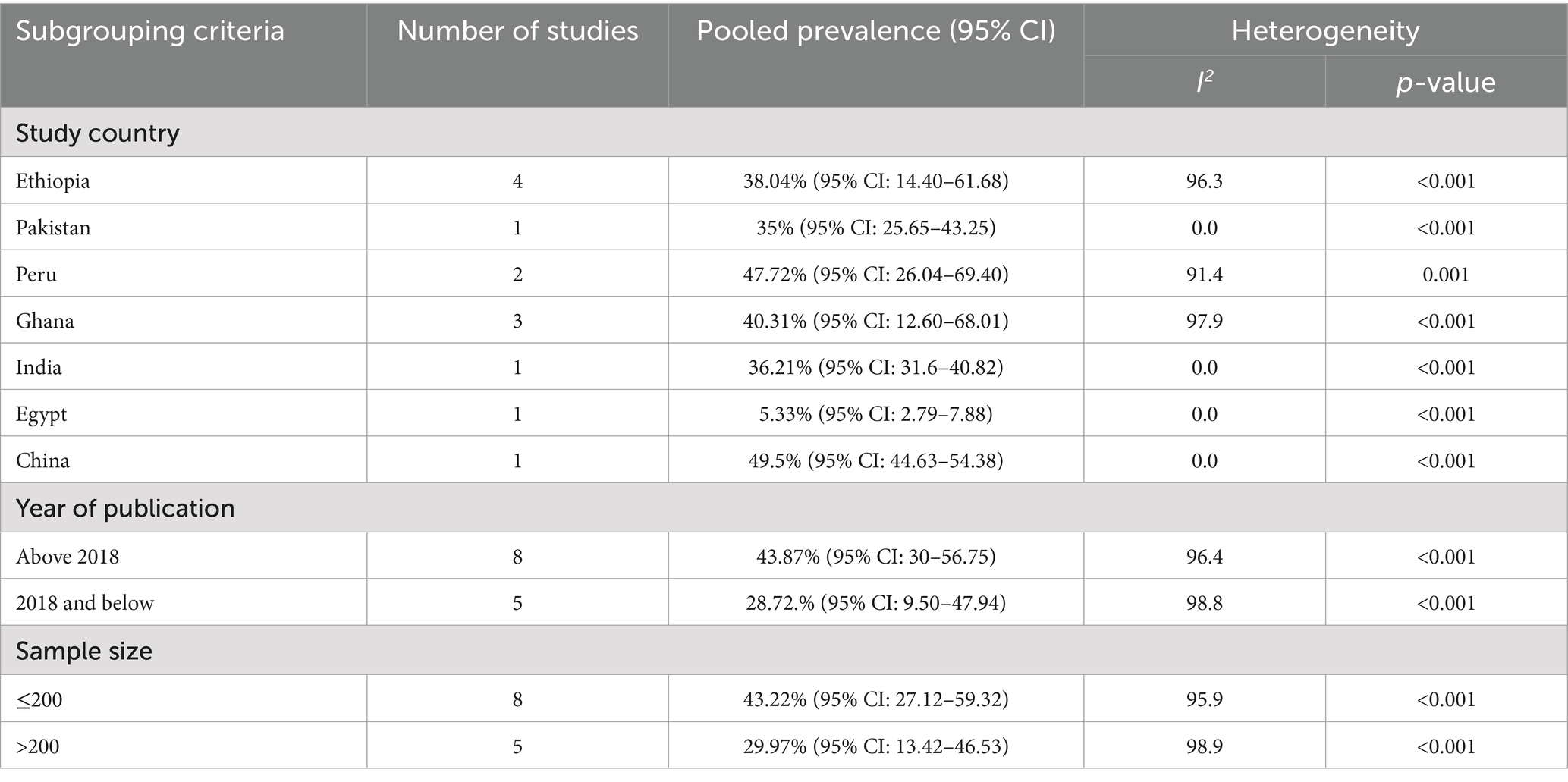
Table 2. Subgroup analysis of the pooled prevalence of Escherichia coli isolates from drinking water in LMICs, 2024.
Furthermore, univariate meta-regression was conducted using study country, sample size, and study year as factors to identify the source of heterogeneity. However, neither of them was found to be statistically significant as sources of heterogeneity (Supplementary file 3). A sensitivity analysis was also conducted to evaluate a single study effect. It was found that 37.94% (95%CI: 23.72–52.46) indicates a slightly broader confidence interval from the pooled prevalence of E.coli isolates, there is no a strong evidence for the effect of a single study (Supplementary file 4). In addition, the funnel plot showed that there was no evidence for publication bias, the included articles were symmetrically distributed (Figure 3). In addition to the funnel plot, the Egger-regression test confirmed that there is no publication bias for this study (p-value = 0.110).
3.4 Pooled prevalence resistance pattern of Escherichia coli isolates from drinking water in LMICs
Eleven antimicrobial agents were used to assess the resistance pattern of E. coli. The finding revealed a high pooled resistance level of E.coli was 54.65% (95% CI: 41.35–67.96) against contrimoxazole, 48.64% (95% CI: −3.6-101) against amoxicillin and 48% (95% CI: −18.1-114.2) against cefuroxime. However, a low pooled resistance level of E.coli was found at 15% (95% CI: 4.95–25.1) against for gentamicin and 15.73% (95% CI: 7.8–23.7) against ciprofloxacin (Table 3).
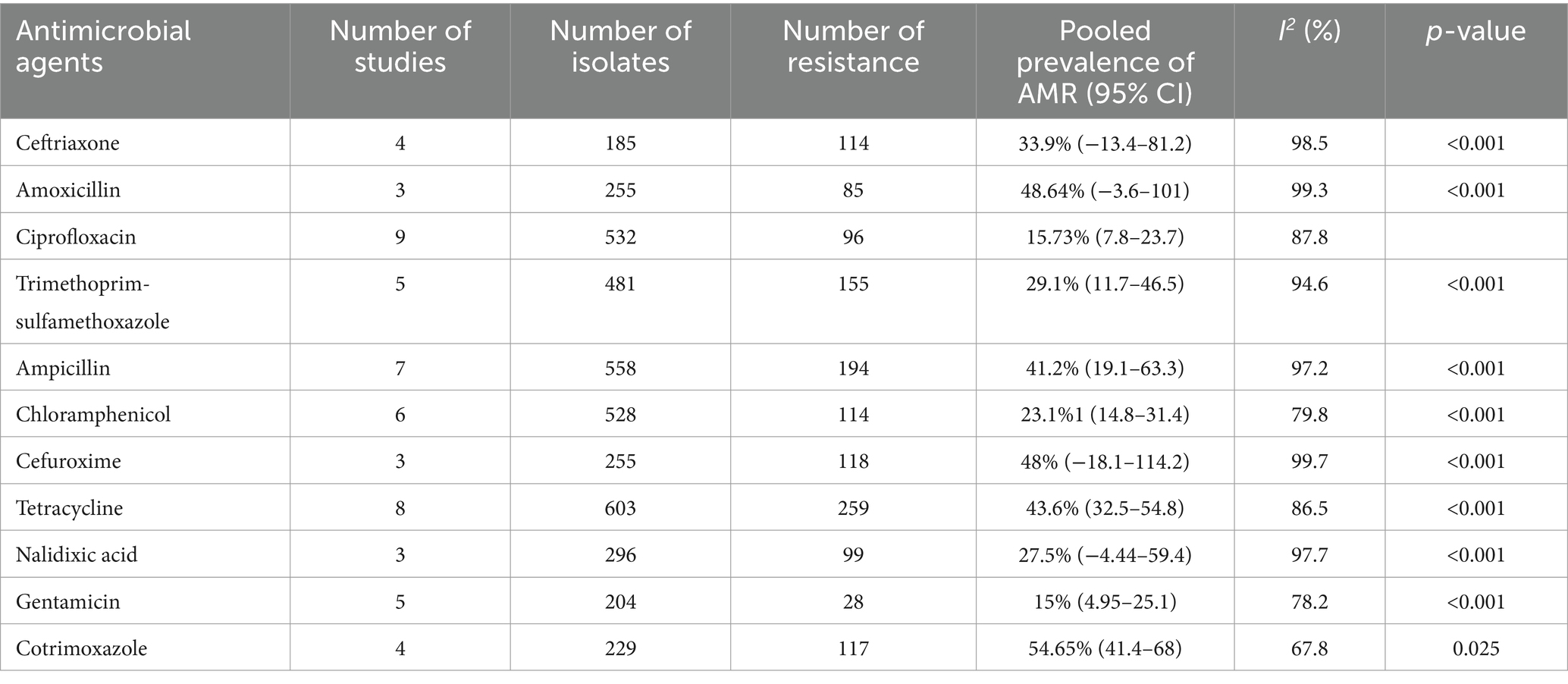
Table 3. Pooled prevalence of AMR patterns of Escherichia coli isolates from drinking water in LMIC, 2024.
3.5 The pooled prevalence of MDR for Escherichia coli isolates from drinking water in LMICs
The pooled prevalence of MDR for E.coli isolates using a random-effects model was estimated at 43.65% (95% CI: 31.15–56.15), with high heterogeneity (I2 = 91.6%, p-value < 0.001) (Figure 4). A sub-group analysis based on study countries, year of publication, and sample size was performed to assess the heterogeneity sources, which were depicted in Table 4. The highest pooled prevalence of 62.50% (95% CI: 38.78–86.22) was observed in Egypt, and the lowest pooled prevalence was estimated 20.88% (95% CI: 14.55–27.20) in Peru. Heterogeneity was highest among studies conducted in Ethiopia (I2 = 91.7%, p-value = <0.001), followed by Ghana (I2 = 77.1%, p-value = 0.013). Based on the year of publication, a study conducted in above 2018 was estimated at 41.58% (95% CI: 25.58–57.78) with (I2 = 92.3%, p-value < 0.001) and 50.06% (95% CI: 17.81–82.30) with (I2 = 92.2%, p-value < 0.001) for a study conducted in 2018 and below. In addition, a study conducted with a sample size of ≤ 100 found 48.53% (95% CI: 32.66–64.40) with (I2 = 85.7%, p-value < 0.001) and a studies conducted with sample size of >100 found 33.92% (95% CI: 13.10–54.74) with (I2 = 95.6%, p-value < 0.001). Furthermore, univariate meta-regression was conducted using study country, sample size, and study year to identify the sources of factor for heterogeneity. However, neither of them was found to be statistically significant for sources of heterogeneity (Supplementary file 5). The findings of the sensitivity analysis indicated that it was 43.65% (95%CI: 28–60.69), only slightly broader in confidence interval from the pooled prevalence, that cannot assured the presence of the effect of a single study (Supplementary file 6). In addition, the funnel plot revealed that there was no evidence of publication bias, the included articles were symmetrically distributed (Figure 5). Moreover, the Egger- regression test confirmed that there is no publication bias for this study (p-value = 0.070).
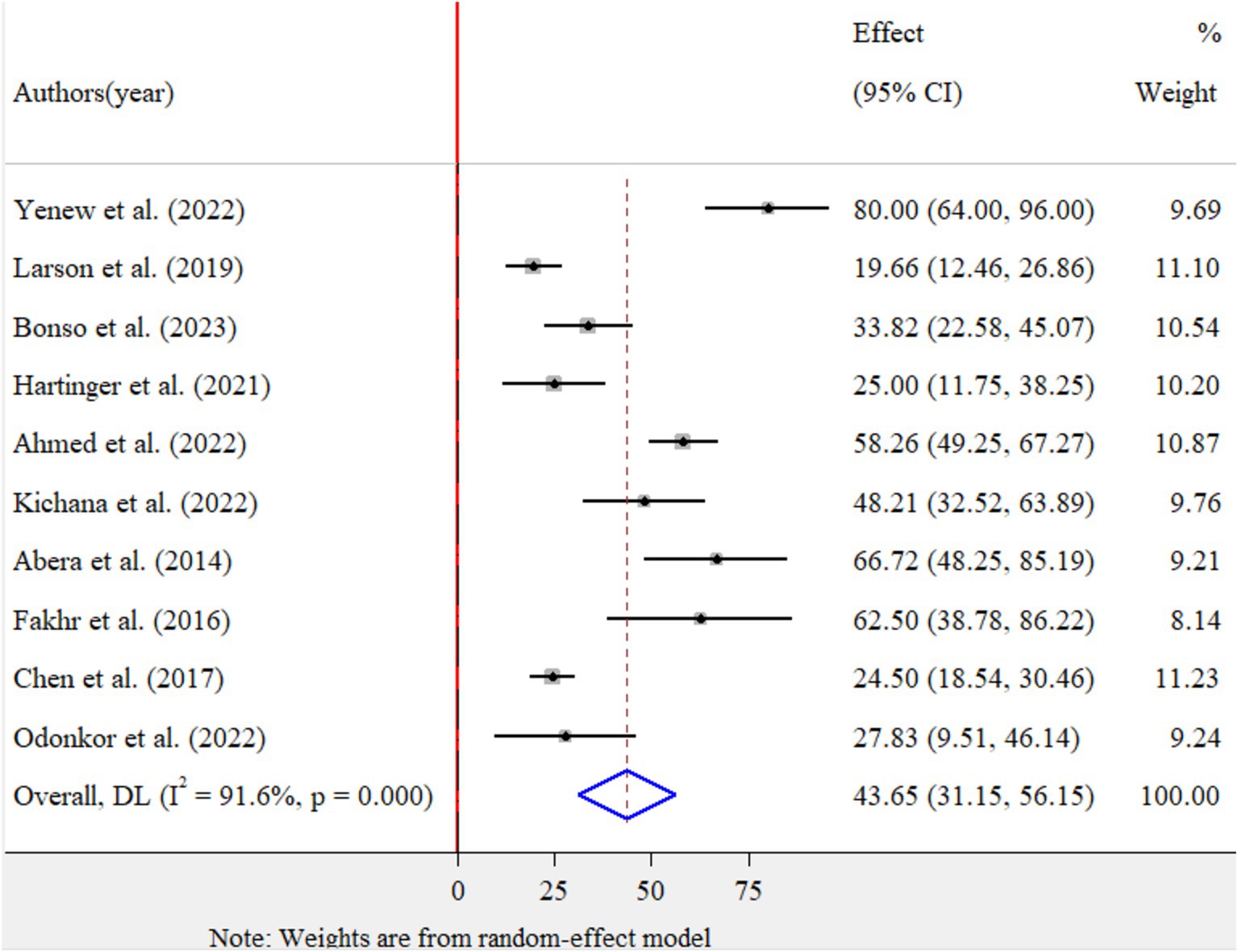
Figure 4. Forest plot for the pooled prevalence of MDR of Escherichia coli isolates from drinking water in LMICs, 2024.
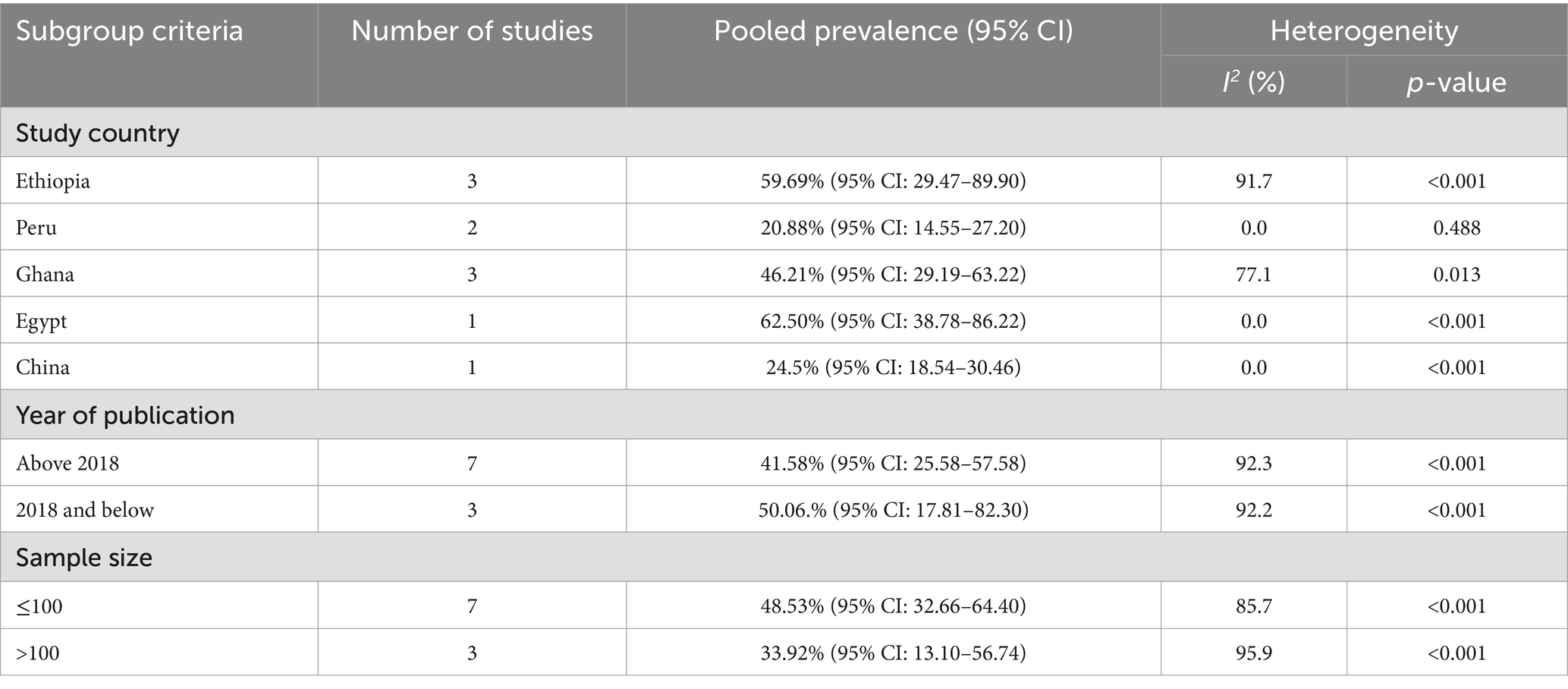
Table 4. Subgroup analysis of the pooled prevalence of MDR of Escherichia coli isolates from drinking water in LMICs, 2024.
4 Discussion
AMR has emerged as a significant worldwide public health problem in the 21st century. It causes high rates of illness and death, particularly in LMICs (42). The presence of AMR exacerbates the high burden of bacterial infections in such areas, where access to adequate diagnostic tools is limited, antimicrobials are often misused or overused, and environmental conditions are poor (43, 44). The presence of AMR in drinking water increases the risk to human health. In this study, we estimate the prevalence and AMR patterns of E.coli isolates from drinking water in LMICs.
In this study, the pooled prevalence estimate of E.coli isolates from drinking water in LMICs was found to be 37.94% (95% CI: 26.75–49.13). This prevalence is lower than the 61.9% reported in a study of systematic reviews and meta-analysis conducted in Africa (45). The differences in prevalence could be attributed to variations in sample characteristics and the effectiveness of water treatment systems in place. The present findings might be explained by the lack of adequate water treatment and poor hygiene and sanitation conditions in those resource-constrained settings (44). However, WHO recommends drinking water be free from E.coli (46). Therefore, it is crucial to strengthen AMR stewardship efforts and improve hygiene and sanitation conditions to address this issue effectively.
In this study, E. coli demonstrated a high level of resistance to several antimicrobials. The highest resistance rates were observed for cotrimoxazole (54.65%), amoxicillin (48.64%), cefuroxime (48%), tetracycline (43.6%), and ampicillin (41.2%). On the other hand, the lowest resistance rates were found for gentamicin (15%), ciprofloxacin (15.73%), and chloramphenicol (23.1%). The current result is supported by many studies showing that E.coli is resistant to many antimicrobials. For example, studies have shown E.coli resistance rates of 69.4 and 77% for ampicillin (45, 47). Another study documented the resistance of E. coli to amoxicillin (24.5%), ampicillin (23.5%), chloramphenicol (12.3%), and trimethoprim-sulfamethoxazole (22.5%) (42). In addition, studies reported lower resistance rates for E.coli rates for ciprofloxacin, ranging from 3 to 13.1% (45, 47). The observed variations in the reported resistance rates could be due to geographical locations and the nature of the study samples. Different countries may have varying levels of antimicrobial use, healthcare practices, surveillance, and sanitation systems, which can influence the resistance level of E.coli to each antimicrobial. Additionally, the condition of the samples analyzed, the source of the sample, and the setting conditions can also contribute to the differences in resistance rates (45, 47).
Overuse of these antibiotics and a lack of safe disposal may lead to resistance by promoting resistance development (48). For instance, cefuroxime is one of the major resistances against E.coli in this study, it is effective against Entrobacteriaceae bacteria, and it is a second-generation cephalosporin antibiotic (49). It is also used for the treatment of urinary tract infections (50). Therefore, the results of the resistance of E.coli in this study may be due to inappropriate treatment of human and animal wastes and their disposal in the environment. Hence, these untreated water sources contribute to AMR dissemination (28).
In this study, the pooled prevalence of MDR in E. coli was found to be 43.65%. This prevalence is lower than the 50.7% reported in a study conducted on water samples in Africa (45). The present finding is supported by earlier studies, which have highlighted the continued significance of E. coli resistance (42, 51). Additionally, in humans, animals and the environment, there have been reports for a high prevalence of AMR (47, 52, 53). Mostly, the present water treatment methods are unable to treat AMR adequately (48). Thus, improving basic hygiene and sanitation and advancing the treatment system will reduce the spread of resistant organisms (44).
LMICs are disproportionally affected by AMR due to the high infectious disease burden and poor antimicrobial use control and regulation (54). The sources of resistance can be environmental sources like sewerage systems, abattoirs, and waste from healthcare facilities that cannot be treated adequately and can contaminate drinking water sources (55). Although treatment systems for water and wastewater have been effective in reducing antimicrobial levels, findings revealed that antimicrobials are still present in drinking water (56, 57). This is a matter of concern because the existence of antibiotics in the environment can exert a strong selective pressure, promoting the acquisition and spread of resistance mechanisms among bacteria (58). Given these implications, it is highly recommended to advance and improve treatment systems to safeguard against the development and dissemination of AMR. By implementing more advanced treatment processes, it can minimize the existence of antimicrobials in sources of water.
4.1 Limitation of the study
This study was limited to publications in the English language and did not consider studies in other languages. In addition, this study focused exclusively on E.coli isolates and their resistance, neglecting the importance of multiple other enteric pathogens that have significant implications for public health. Moreover, the study did not identify the factors associated with E.coli resistance in drinking water.
5 Conclusion
AMR has emerged as a critical worldwide public health issue, particularly in LMICs. This study highlights E.coli isolates and their resistance to drinking water in LMICs were prevalent. The study revealed that E. coli resistance to various antimicrobial agents, with high resistance observed for contrimoxazole, amoxicillin, cefuroxime, and tetracycline. To tackle this concerning issue, it is essential to improve basic hygiene and sanitation practices. Additionally, advancing and upgrading water and wastewater treatment systems is essential to minimize the spread of resistant organisms. Taking a One-Health approach, it is recommended that concerned bodies and international organizations collaborate to mitigate health risks and minimize the environmental impact of AMR. For future researchers, it is advisable to conduct comprehensive investigations of other enteric pathogens in drinking water. Furthermore, identifying the drivers or factors contributing to AMR in drinking water would provide valuable insights for developing targeted interventions and strategies. By taking these actions, researchers and policymakers can work together to tackle the critical challenge of AMR in drinking water, particularly in LMICs where the burden is most severe.
Data availability statement
The raw data supporting the conclusions of this article will be made available by the authors, without undue reservation.
Author contributions
BD: Conceptualization, Investigation, Methodology, Software, Writing – original draft, Writing – review & editing. TW: Data curation, Investigation, Methodology, Supervision, Writing – review & editing. LA: Formal analysis, Methodology, Software, Supervision, Writing – review & editing. YT: Methodology, Supervision, Validation, Visualization, Writing – review & editing. DM: Data curation, Formal analysis, Methodology, Software, Writing – review & editing. AE: Methodology, Software, Supervision, Validation, Writing – review & editing. CD: Conceptualization, Investigation, Validation, Writing – original draft.
Funding
The author(s) declare that no financial support was received for the research, authorship, and/or publication of this article.
Conflict of interest
The authors declare that the research was conducted in the absence of any commercial or financial relationships that could be construed as a potential conflict of interest.
Publisher’s note
All claims expressed in this article are solely those of the authors and do not necessarily represent those of their affiliated organizations, or those of the publisher, the editors and the reviewers. Any product that may be evaluated in this article, or claim that may be made by its manufacturer, is not guaranteed or endorsed by the publisher.
Supplementary material
The Supplementary material for this article can be found online at: https://www.frontiersin.org/articles/10.3389/fpubh.2024.1440908/full#supplementary-material
References
1. United Nations Environment (2023). Bracing for superbugs: strengthening environmental action in the one health response to antimicrobial resistance. UNEP - UN Environment Programme. (2023). Available at: http://www.unep.org/resources/superbugs/environmental-action (Accessed April 4, 2024).
2. Graham, DW, Bergeron, G, Bourassa, MW, Dickson, J, Gomes, F, Howe, A, et al. Complexities in understanding antimicrobial resistance across domesticated animal, human, and environmental systems. Ann N Y Acad Sci. (2019) 1441:17–30. doi: 10.1111/nyas.14036
3. Larsson, DGJ, and Flach, C-F. Antibiotic resistance in the environment. Nat Rev Microbiol. (2022) 20:257–69. doi: 10.1038/s41579-021-00649-x
4. Kalungia, AC, Mwambula, H, Munkombwe, D, Marshall, S, Schellack, N, May, C, et al. Antimicrobial stewardship knowledge and perception among physicians and pharmacists at leading tertiary teaching hospitals in Zambia: implications for future policy and practice. J Chemother. (2019) 31:378–87. doi: 10.1080/1120009X.2019.1622293
5. World Health Organization (2021). Antimicrobial resistance: key facts, 17 November. Available at: https://www.who.int/news-room/fact-sheets/detail/antimicrobial-resistance (Accessed April 4, 2024).
6. Murray, CJL, Ikuta, KS, Sharara, F, Swetschinski, L, Aguilar, GR, Gray, A, et al. Global burden of bacterial antimicrobial resistance in 2019: a systematic analysis. Lancet. (2022) 399:629–55.
7. WHO. The fight against antimicrobial resistance is closely linked to the sustainable development goals. Available at: https://www.who.int/europe/publications/i/item/WHO-EURO-2020-1634-41385-56394. (Accessed April 8, 2024).
8. Tang, KL, Caffrey, NP, Nóbrega, DB, Cork, SC, Ronksley, PE, Barkema, HW, et al. Restricting the use of antibiotics in food-producing animals and its associations with antibiotic resistance in food-producing animals and human beings: a systematic review and meta-analysis. Lancet Planet Health. (2017) 1:e316–27. doi: 10.1016/S2542-5196(17)30141-9
9. Panel (OHHLEP) OHH-LE Adisasmito, WB, Almuhairi, S, Behravesh, CB, Bilivogui, P, Bukachi, SA, et al. One health: a new definition for a sustainable and healthy future. PLoS Pathog. (2022) 18:e1010537. doi: 10.1371/journal.ppat.1010537
10. Brack, W, Barcelo Culleres, D, Boxall, ABA, Budzinski, H, Castiglioni, S, Covaci, A, et al. One planet: one health. A call to support the initiative on a global science–policy body on chemicals and waste. Environmental sciences. Europe. (2022) 34:21. doi: 10.1186/s12302-022-00602-6
11. Hernando-Amado, S, Coque, TM, Baquero, F, and Martínez, JL. Defining and combating antibiotic resistance from one health and Global Health perspectives. Nat Microbiol. (2019) 4:1432–42. doi: 10.1038/s41564-019-0503-9
12. O’Neill, J. Tackling drug-resistant infections globally: final report and recommendations Available at: https://apo.org.au/node/63983 (Accessed August 2, 2022).
13. Nadimpalli, ML, Marks, SJ, Montealegre, MC, Gilman, RH, Pajuelo, MJ, Saito, M, et al. Urban informal settlements as hotspots of antimicrobial resistance and the need to curb environmental transmission. Nat Microbiol. (2020) 5:787–795. doi: 10.1038/s41564-020-0722-0
14. United Nation. Human rights to water and sanitation. UN-Water. Available at: https://www.unwater.org/water-facts/human-rights-water-and-sanitation (Accessed April 9, 2024).
15. Walker, GT, Quan, J, Higgins, SG, Toraskar, N, Chang, W, Saeed, A, et al. Predicting antibiotic resistance in gram-negative Bacilli from resistance genes. Antimicrob Agents Chemother. (2019) 63:e02462–18. doi: 10.1128/AAC.02462-18
16. Bürgmann, H, Frigon, D, H Gaze, W, M Manaia, C, Pruden, A, Singer, AC, et al. Water and sanitation: an essential battlefront in the war on antimicrobial resistance. FEMS Microbiol Ecol. (2018) 94:94. doi: 10.1093/femsec/fiy101
17. Khan, S. Antimicrobial resistance in environment and antimicrobial stewardship IntechOpen (2023). doi: 10.5772/intechopen.113224
18. Tan, Q, Li, W, Zhang, J, Zhou, W, Chen, J, Li, Y, et al. Presence, dissemination and removal of antibiotic resistant bacteria and antibiotic resistance genes in urban drinking water system: a review. Front Environ Sci Eng. (2019) 13:36. doi: 10.1007/s11783-019-1120-9
19. Zheng, J, Chen, T, and Chen, H. Antibiotic resistome promotion in drinking water during biological activated carbon treatment: is it influenced by quorum sensing? Sci Total Environ. (2018) 612:1–8. doi: 10.1016/j.scitotenv.2017.08.072
20. Xu, L, Ouyang, W, Qian, Y, Su, C, Su, J, and Chen, H. High-throughput profiling of antibiotic resistance genes in drinking water treatment plants and distribution systems. Environ Pollut. (2016) 213:119–26. doi: 10.1016/j.envpol.2016.02.013
21. Abo-State, M, Mahdy, H, Ezzat, S, Shakour, E, and Elbahnasawy, M. Antimicrobial resistance profiles of Enterobacteriaceae isolated from Rosetta branch of River Nile. Egypt World Appl Sci J. (2012) 19:1234–43. doi: 10.5829/idosi.wasj.2012.19.09.2785
22. Leslie, E, Hinds, J, and Hai, FI. Causes, factors, and control measures of opportunistic premise plumbing pathogens—a critical review. Appl Sci. (2021) 11:4474. doi: 10.3390/app11104474
23. WHO publishes list of bacteria for which new antibiotics are urgently needed. Available at: https://www.who.int/news/item/27-02-2017-who-publishes-list-of-bacteria-for-which-new-antibiotics-are-urgently-needed (Accessed April 22, 2024).
24. Mahmud, ZH, Kabir, MH, Ali, S, Moniruzzaman, M, Imran, KM, Nafiz, TN, et al. Extended-Spectrum Beta-lactamase-producing Escherichia coli in drinking water samples from a forcibly displaced, densely populated community setting in Bangladesh. Front Public Health. (2020) 8:228. doi: 10.3389/fpubh.2020.00228
25. Fakhr, AE, Gohar, MK, and Atta, AH. Impact of some ecological factors on fecal contamination of drinking water by Diarrheagenic antibiotic-resistant Escherichia coli in Zagazig City. Egypt Int J Microbiol. (2016) 2016:6240703. doi: 10.1155/2016/6240703
26. Kichana, E, Opare-Boafoa, MS, and Bekoe, EMO. Prevalence of multidrug-resistant Escherichia coli in household drinking water in rural Ghana. J Water Sanit Hygiene Dev. (2022) 12:862–8. doi: 10.2166/washdev.2022.082
27. Larson, A, Hartinger, SM, Riveros, M, Salmon-Mulanovich, G, Hattendorf, J, Verastegui, H, et al. Antibiotic-resistant Escherichia coli in drinking water samples from rural Andean households in Cajamarca. Peru Am J Trop Med Hyg. (2019) 100:1363–8. doi: 10.4269/ajtmh.18-0776
28. Ahmed, H, Zolfo, M, Williams, A, Ashubwe-Jalemba, J, Tweya, H, Adeapena, W, et al. Antibiotic-resistant Bacteria in drinking water from the Greater Accra region, Ghana: a cross-sectional study, December 2021–march 2022. Int J Environ Res Public Health. (2022) 19:12300. doi: 10.3390/ijerph191912300
29. Dhengesu, D, Lemma, H, Asefa, L, and Tilahun, D. Antimicrobial resistance profile of Enterobacteriaceae and drinking water quality among households in Bule Hora town. South Ethiopia Risk Manag Healthc Policy. (2022) 15:1569–80. doi: 10.2147/RMHP.S370149
30. Odonkor, ST, Simpson, SV, Morales Medina, WR, and Fahrenfeld, NL. Antibiotic-resistant Bacteria and resistance genes in isolates from Ghanaian drinking water sources. J Environ Public Health. (2022) 2022:2850165. doi: 10.1155/2022/2850165
31. Yenew, C, Kebede, M, and Mulat, M. Drinking water antimicrobial resistance enteric bacterial load and public health risk in northwest, Ethiopia |. Ethiop Med J. (2022)
32. Augustin, J-C, and Carlier, V. Lessons from the organization of a proficiency testing program in food microbiology by interlaboratory comparison: analytical methods in use, impact of methods on bacterial counts and measurement uncertainty of bacterial counts. Food Microbiol. (2006) 3:1–38. doi: 10.1016/j.fm.2005.01.010
33. Founou, RC, Founou, LL, and Essack, SY. Clinical and economic impact of antibiotic resistance in developing countries: a systematic review and meta-analysis. PLoS One. (2017) 12:e0189621. doi: 10.1371/journal.pone.0189621
34. WHo. Global action plan on antimicrobial resistance. 2016. Available at: https://www.who.int/publications-detail-redirect/9789241509763. Accessed April 22, 2024.
35. Sanganyado, E, and Gwenzi, W. Antibiotic resistance in drinking water systems: occurrence, removal, and human health risks. Sci Total Environ. (2019) 669:785–797. doi: 10.1016/j.scitotenv.2019.03.162
36. Page, MJ, McKenzie, JE, Bossuyt, PM, Boutron, I, Hoffmann, TC, Mulrow, CD, et al. The PRISMA 2020 statement: an updated guideline for reporting systematic reviews. Syst Rev. (2021) 10:89. doi: 10.1186/s13643-021-01626-4
37. World Bank Open Data. Available at: https://data.worldbank.org (Accessed April 16, 2024).
38. Moola, S, Munn, Z, Tufanaru, C, Aromataris, E, Sears, K, Sfetcu, R, et al. (2017). Chapter 7: Systematic reviews of etiology and risk. In: eds. E. Aromataris and Z. Munn Joanna Briggs Institute Reviewer’s Manual. The Joanna Briggs Institute, Available at: https://reviewersmanual.joannabriggs.org/
39. Aromataris, E, Lockwood, C, Porritt, K, Pilla, B, and Jordan, Z. JBI Manual for Evidence Synthesis. JBI. (2024). Available at: https://synthesismanual.jbi.global.
40. Munn, Z, Moola, S, Lisy, K, Riitano, D, and Tufanaru, C. Methodological guidance for systematic reviews of observational epidemiological studies reporting prevalence and cumulative incidence data. Int J Evid Based Healthc. (2015) 13:147–53. doi: 10.1097/XEB.0000000000000054
41. Higgins, JPT, and Thompson, SG. Quantifying heterogeneity in a meta-analysis. Stat Med. (2002) 21:1539–58. doi: 10.1002/sim.1186
42. WHO. Antimicrobial resistance in the WHO African region: a systematic literature review. WHO | regional Office for Africa. (2024). Available at: https://www.afro.who.int/publications/antimicrobial-resistance-who-african-region-systematic-literature-review. Accessed April 21, 2024.
43. Irek, EO, Amupitan, AA, and Obadare TO Aboderin, AO. A systematic review of healthcare-associated infections in Africa: an antimicrobial resistance perspective. Afr J Lab Med. (2018) 7:796. doi: 10.4102/ajlm.v7i2.796
44. Ayukekbong, JA, Ntemgwa, M, and Atabe, AN. The threat of antimicrobial resistance in developing countries: causes and control strategies. Antimicrob Resist Infect Control. (2017) 6:47. doi: 10.1186/s13756-017-0208-x
45. Ramatla, T, Ramaili, T, Lekota, KE, Ndou, R, Mphuti, N, Bezuidenhout, C, et al. A systematic review and meta-analysis on prevalence and antimicrobial resistance profile of Escherichia coli isolated from water in africa (2000–2021). Heliyon. (2023) 9:e16123. doi: 10.1016/j.heliyon.2023.e16123
46. WHO. Drinking-water. WHO fact sheet on water: key facts, access to water, water and health. 2023. Available at: https://www.who.int/news-room/fact-sheets/detail/drinking-water (Accessed April 24, 2024).
47. Gemeda, BA, Assefa, A, Jaleta, MB, Amenu, K, and Wieland, B. Antimicrobial resistance in Ethiopia: a systematic review and meta-analysis of prevalence in foods, food handlers, animals, and the environment. One Health. (2021) 13:100286. doi: 10.1016/j.onehlt.2021.100286
48. Duarte, AC, Rodrigues, S, Afonso, A, Nogueira, A, and Coutinho, P. Antibiotic resistance in the drinking water: old and new strategies to remove antibiotics, resistant Bacteria, and resistance genes. Pharmaceuticals. (2022) 15:393. doi: 10.3390/ph15040393
49. Chang, U-I, Kim, HW, and Wie, S-H. Use of cefuroxime for women with community-onset acute pyelonephritis caused by cefuroxime-susceptible or -resistant Escherichia coli. Korean J Intern Med. (2016) 31:145–55. doi: 10.3904/kjim.2016.31.1.145
50. Venkatesh, S, Chauhan, L, Gadpayle, A, Jain, T, Wattal, C, Aneja, S, et al. National Treatment Guidelines for antimicrobial use in infectious diseases. (2016).
51. GBD. Antimicrobial resistance collaborators. Global mortality associated with 33 bacterial pathogens in 2019: a systematic analysis for the global burden of disease study 2019. Lancet. (2019) 2022:2221–48. doi: 10.1016/S0140-6736(21)02724-0
52. Fujita, AW, Werner, K, Jacob, JT, Tschopp, R, Mamo, G, Mihret, A, et al. Antimicrobial resistance through the Lens of one health in Ethiopia: a review of the literature among humans, animals, and the environment. Int J Infect Dis. (2022) 119:120–9. doi: 10.1016/j.ijid.2022.03.041
53. Sonola, VS, Katakweba, AS, Misinzo, G, and Matee, MIN. Occurrence of multi-drug-resistant Escherichia coli in chickens, humans, rodents and household soil in Karatu, northern Tanzania. Antibiotics. (2021) 10:1137. doi: 10.3390/antibiotics10091137
54. Kariuki, S, Kering, K, Wairimu, C, Onsare, R, and Mbae, C. Antimicrobial resistance rates and surveillance in sub-Saharan Africa: where are we now? Infect Drug Resist. (2022) 15:3589–609. doi: 10.2147/IDR.S342753
55. Hu, Y, Gao, GF, and Zhu, B. The antibiotic resistome: gene flow in environments, animals and human beings. Front Med. (2017) 11:161–8. doi: 10.1007/s11684-017-0531-x
56. Xu, L, Zhang, H, Xiong, P, Zhu, Q, Liao, C, and Jiang, G. Occurrence, fate, and risk assessment of typical tetracycline antibiotics in the aquatic environment: a review. Sci Total Environ. (2021) 753:141975. doi: 10.1016/j.scitotenv.2020.141975
57. Ben, Y, Hu, M, Zhang, X, Wu, S, Wong, MH, Wang, M, et al. Efficient detection and assessment of human exposure to trace antibiotic residues in drinking water. Water Res. (2020) 175:115699. doi: 10.1016/j.watres.2020.115699
58. Karkman, A, Do, TT, Walsh, F, and Virta, MPJ. Antibiotic-resistance genes in waste water. Trends Microbiol. (2018) 26:220–8. doi: 10.1016/j.tim.2017.09.005
59. Abera, B, Kibret, M, Goshu, G, and Melaku, M. Bacterial quality of drinking water sources and antimicrobial resistance profile of Enterobacteriaceae in Bahir Dar city, Ethiopia. J Water Sanitation Hygiene Dev. (2014) 4:384–90. doi: 10.2166/washdev.2014.105
60. Bonso, M, Bedada, D, and Dires, S. Bacterial contamination and antimicrobial resistance in drinking water from food and drinking establishments in Shashemane town. Ethiopia Environ Health Insights. (2023) 17:11786302231216864. doi: 10.1177/11786302231216864
61. Chen, Z, Yu, D, He, S, Ye, H, Zhang, L, Wen, Y, et al. Prevalence of antibiotic-resistant Escherichia coli in drinking water sources in Hangzhou City. Front Microbiol. (2017) 8:8. doi: 10.3389/fmicb.2017.01133
62. Hartinger, SM, Medina-Pizzali, ML, Salmon-Mulanovich, G, Larson, AJ, Pinedo-Bardales, M, Verastegui, H, et al. Antimicrobial resistance in humans, animals, water and household environs in rural Andean Peru: exploring dissemination pathways through the one health Lens. Int J Environ Res Public Health. (2021) 18:4604. doi: 10.3390/ijerph18094604
63. Sahoo, KC, Tamhankar, AJ, Sahoo, S, Sahu, PS, Klintz, SR, and Lundborg, CS. Geographical variation in antibiotic-resistant Escherichia coli isolates from stool, cow-dung and drinking water. Int J Environ Res Public Health. (2012) 9:746–59. doi: 10.3390/ijerph9030746
Keywords: antimicrobial resistance, Escherichia coli, drinking water, one health, low and middle income countries
Citation: Desye B, Woldetsadik Mawugatie T, Asmare L, Tsega Y, Melak D, Endawkie A and Daba C (2024) Antimicrobial resistance profile of Escherichia coli in drinking water from one health perspective in low and middle income countries. Front. Public Health. 12:1440908. doi: 10.3389/fpubh.2024.1440908
Edited by:
Visva Bharati Barua, University of North Carolina at Charlotte, United StatesReviewed by:
Piyush Baindara, University of Missouri, United StatesYabing Li, Michigan State University, United States
Tista Prasai Joshi, Nepal Academy of Science and Technology (NAST), Nepal
Copyright © 2024 Desye, Woldetsadik Mawugatie, Asmare, Tsega, Melak, Endawkie and Daba. This is an open-access article distributed under the terms of the Creative Commons Attribution License (CC BY). The use, distribution or reproduction in other forums is permitted, provided the original author(s) and the copyright owner(s) are credited and that the original publication in this journal is cited, in accordance with accepted academic practice. No use, distribution or reproduction is permitted which does not comply with these terms.
*Correspondence: Belay Desye, YmVsYXlkZXN5ZS4yMDAxQGdtYWlsLmNvbQ==