- 1Chongqing Medical and Pharmaceutical College, Chongqing, China
- 2NHC Key Laboratory of Birth Defects and Reproductive Health, Chongqing Population and Family Planning Science and Technology Research Institute, Chongqing, China
In light of the undeniable and alarming fact that human fertility is declining, the harmful factors affecting reproductive health are garnering more and more attention. Iodoacetic acid (IAA), an emerging unregulated drinking water disinfection byproduct, derives from chlorine disinfection and is frequently detected in the environment and biological samples. Humans are ubiquitously exposed to IAA daily mainly through drinking water, consuming food and beverages made from disinfected water, contacting swimming pools and bath water, etc. Mounting evidence has indicated that IAA could act as a reproductive toxicant and bring about multifarious adverse reproductive damage. For instance, it can interfere with gonadal development, weaken ovarian function, impair sperm motility, trigger DNA damage to germ cells, perturb steroidogenesis, etc. The underlying mechanisms predominantly include cytotoxic and genotoxic effects on germ cells, disturbance of the hypothalamic–pituitary-gonadal axis, oxidative stress, inhibition of steroidogenic proteins or enzymes, and dysbiosis of gut microbiota. Nevertheless, there are still some knowledge gaps and limitations in studying the potential impact of IAA on reproduction, which urgently need to be addressed in the future. We suppose that necessary population epidemiological studies, more sensitive detection methods for internal exposure, and mechanism-based in-depth exploration will contribute to a more comprehensive understanding of characteristics and biological effects of IAA, thus providing an important scientific basis for revising sanitary standards for drinking water quality.
1 Introduction
Accumulating evidence has suggested that human fertility has gradually declined in recent decades, and it is estimated that approximately 186 million couples of reproductive age worldwide are influenced by infertility (1). Therefore, to identify the potential risk factors contributing to the decline in human fertility has become a significant public and clinical health concern. Apart from genetic factors, a growing body of population and experimental research has demonstrated that environmental factors, especially exposure to environmental pollutants, play a prominent role in unfavorable reproductive outcomes, such as infertility, abnormal pregnancy, miscarriage, preterm delivery, disorder of sex hormone levels, sexual dysfunction, etc. (2–6).
Drinking water disinfection is an effective means to eliminate waterborne diseases and is regarded as one of the most significant and practical public health achievements of the 20th century. In the disinfection process, however, disinfection byproducts (DBPs) are unintentionally produced from the reaction of highly reactive disinfectants with organic or inorganic components including bromide, chloride, and iodide in raw water (7). For instance, iodinated DBPs (I-DBPs) are formed during the disinfection of iodide-containing raw water. The unavoidable generation of DBPs in drinking water has aroused public concern for health. In particular, a large number of studies have linked DBPs exposure to various reproductive impairments and even infertility, such as steroidogenesis suppression, spermatogenetic dysfunction, diminished ovarian reserve (8–11). So far, over 700 DBPs have been discovered in drinking water, but only 11 of them are currently under supervision of regulatory agencies. Actually, an increasing body of literature has revealed that some hazardous health effects mediated by DBPs, including reproductive damage, are often attributed to unregulated DBPs, which in many cases exhibit greater toxicity or toxic potential than regulated DBPs (12, 13).
Iodoacetic acid (IAA) is an emerging unregulated iodinated DBP, which has attracted considerable attention in recent years due to its higher cytotoxicity and genotoxicity than other DBPs (14, 15). A large quantity of environmental monitoring data have shown that IAA is frequently detected in drinking water, and its concentration is up to 1.7 μg/L in the United States and Canada (16) and up to 2.18 μg/L in China (17). In coastal and inland regions with high iodine levels, the IAA concentration in drinking water may be higher with the increase in iodide contents in raw water. Humans are ubiquitously exposed to IAA on a daily basis, primarily through drinking water, consuming food and beverages made with disinfected water, and contact with swimming pools and bath water, among other sources (Figure 1). Therefore, given the high toxicity and persistent exposure to IAA, it is crucial to comprehensively evaluate its toxicity and detrimental health effects on organisms. In recent years, a series of adverse health effects of IAA have been gradually observed and reported, such as the carcinogenic effect (17), cytotoxic effect (18, 19), thyroid dysfunction (20, 21), gut microbiota disorder (22), developmental disturbance (23), retinal degeneration (24, 25), etc. Although direct evidence from population research is scarce, several in vivo and in vitro studies have proposed that IAA could act as a reproductive toxicant and potentially interfere with reproduction and fertility. For instance, our latest work uncovered that IAA possessed an anti-androgenic property and impeded testosterone biosynthesis in Leydig cells through the crosstalk between the GRP78/IRE1/XBP1 pathway and the cGAS/STING pathway (26). Hence, this paper reviewed the limited literature on IAA exposure in humans, animals, and cells to figure out the negative effect of IAA on reproduction, and further explored its underlying mechanisms of action. This review will facilitate a better understanding of deleterious effects of IAA on reproductive health and a more comprehensive assessment of toxic effects and biological features of IAA, thus providing an important scientific basis for revising sanitary standards for drinking water quality.
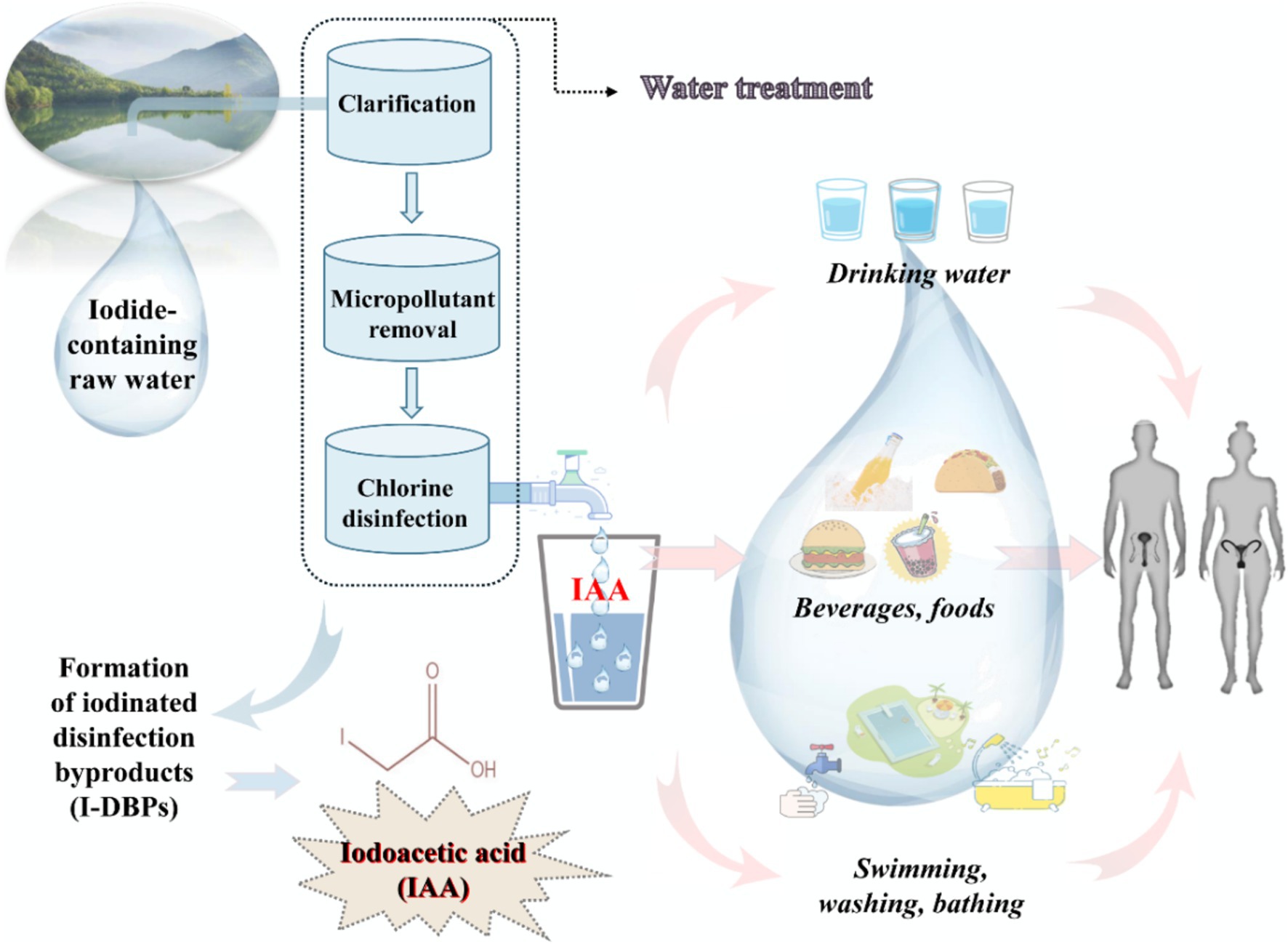
Figure 1. A schematic model demonstrated the underlying exposure routes of iodoacetic acid (IAA, an iodinated disinfection byproduct of drinking water).
2 Methodology
The search databases were Web of Science, PubMed, and Google Scholar. The retrieval time node ranged from Jan. 2000 to Jul. 2024. The retrieval strategy was optimized with the use of Boolean logical operators. The search terms included, but were not limited to, disinfection byproducts, DBPs, iodinated DBPs, I-DBPs, iodoacetic acid, IAA, reproduction, reproductive impairment, fertility, infertility, testis, prostate, epididymis, sperm, ovary, uterus, follicle, and steroid hormone. The inclusion criteria were as follows: (a) studies investigated the deleterious effects of IAA on reproduction; (b) studies were performed in humans, animals, or cells; (c) studies were available as full-text publications; and (d) studies were presented in English. Finally, a total of 98 studies were identified and 53 were included in this review.
3 IAA-induced harmful effects on reproductive health
After exposure to IAA, it could enter the body and accumulate in diverse tissues and organs, including reproductive organs. For instance, in a recent study on the internal exposure level of IAA, after oral exposure to 6 mg/kg of IAA, it was detected in rat testes and its concentration reached up to 1.12 ng/g (27). Therefore, long-term exposure to IAA, an emerging endocrine disruptor, is likely to bring a series of reproductive disorders to both humans and animals.
3.1 Hazardous effects of IAA on male reproductive health
The research by Ali et al. (28) was performed to evaluate the direct influence of IAA exposure on sperm using semen samples provided by healthy volunteers. After treatment with 25 μM of IAA for 1 h, apparent sperm DNA damage and genotoxic responses were observed using the comet assay. However, these harmful effects caused by IAA could be effectively antagonized and alleviated by the antioxidant butylated hydroxyanisole (BHA) or catalase. Therefore, it is supposed that the redox imbalance may be an important mode of action of IAA-triggered unfavorable effects on reproduction. Similar reproductive damage effects also appeared in an IAA-treated male mouse model. After exposure to 35 mg/kg of IAA for consecutive 28 days via oral gavage, IAA not only lowered the sperm motility by directly reducing the average path velocity (VAP) and straight line velocity (VSL) of sperm, but also brought about DNA double-strand breaks of spermatogenic cells, especially that of spermatocytes (9). Moreover, IAA also interfered with steroidogenesis, giving rise to an increase in serum LH levels and a borderline decrease in testosterone levels. SRB1 is responsible for the transport of high-density lipoprotein (HDL) cholesteryl esters into Leydig cells (29), and the inhibition of SRB1 protein expression could partially account for the declining trend of serum testosterone levels after IAA exposure (9). Similar results were also observed in our recent rat model. According to the previous investigation by Xia et al. (20), the acute oral LD50 value of IAA in male rats was 126 mg/kg. After exposure to 25 mg/kg (1/5 of LD50) of IAA for 31 days, the testicular histomorphology and ultrastructure of exposed rats were abnormally changed and testosterone biosynthesis was inhibited, followed by a decrease in the testosterone level in rat plasma (26). In reproductive/developmental toxicity screening tests, SD rats were treated with 22.5 mg/kg of IAA for consecutive 28 days and the sperm motility was declined in a dose-dependent manner. In addition, relative weights of testes and seminal vesicles plus coagulating glands (SVCG) in parental male rats were increased, while the anogenital distance (AGD) index of male pups was decreased after IAA exposure (23). These research findings are considered to be associated with the androgen disturbing characteristic of IAA. This perspective is supported by another study conducted in male rats. Sha et al. (22) also proposed that IAA could act as an androgen disruptor. After exposure to 16 mg/kg of IAA for consecutive 56 days, IAA perturbed the abundance of steroid hormone biosynthesis-related genes in the gut microbiota of male rats via the dysbiosis of intestinal flora and its metabolism, finally influencing androgen levels of male rats.
In conclusion, though employing different exposure models, routes, dosages, and periods, above evidence from populations and animals has clearly indicated that IAA has the interference effect on male reproductive health. IAA could influence gonadal development, facilitate sperm DNA damage, impair testicular histomorphology and ultrastructure, decline sperm motility, and perturb the biosynthesis of male steroid hormones (Table 1). Nevertheless, it should be noted that though some progress has been made, most of the evidence comes from the experimental models, and the evidence from population studies is relatively insufficient. Moreover, there are still knowledge gaps that merit further research. For instance, could IAA interfere with the microenvironment of sperm production by affecting the blood-testis barrier? Could IAA disturb feedback regulation of the hypothalamic–pituitary-testis (HPT) axis by regulating hormone receptor binding? Without doubt, more population epidemiological research and further mechanism-based studies are needed in the future to address these pending concerns.
3.2 Hazardous effects of IAA on female reproductive health
Compared with studies on male reproduction, research on the detrimental impact of IAA on female reproductive health is more abundant. The study by Xia et al. (20) revealed that after exposure to 24 mg/kg of IAA for consecutive 28 days through oral gavage, the ovarian weight of female SD rats was significantly downregulated, while the hypothalamic weight was upregulated. Intriguingly, IAA had different effects on the gonadal development of female offspring. During prenatal, gestational, and lactational exposure to 500 mg/L of IAA, it reduced the vaginal opening rate and the percentage of atretic follicles of female pups, but elevated the absolute weight of ovaries and the anogenital index. Moreover, IAA also gave rise to a borderline decline in progesterone and FSH levels and an increase in testosterone levels in female pups (30). In addition, there is evidence that the estradiol level is also a potential disturbing target for IAA. After exposure to IAA for consecutive 35 days, IAA significantly decreased estradiol levels in female CD-1 mice. Besides, IAA not only shortened the estrous cyclicity, but also disturbed the mRNA level of ovarian genes (31, 32). Gonzalez et al. (33) proposed that IAA-mediated female reproductive impairment was closely associated with the disturbance of the hypothalamic–pituitary-ovary (HPO) axis, as evidenced by an increase in kisspeptin mRNA levels in the hypothalamic arcuate nucleus and a decrease in FSHβ-positive cell numbers and FSHβ mRNA levels in the pituitary in female CD-1 mice. What’s more, IAA led to DNA damage and induced P21/Cdknɑ mRNA levels in the pituitary in female mice. Likewise, gut microbiota dysbiosis also played an important role in IAA-aroused abnormality of hormone levels in females. It was reported that IAA exposure for 8 weeks led to an upregulation of androstanediol levels in female SD rats by disturbing gut microbiota (22). Furthermore, growing in vitro studies indicate that IAA has potent cytotoxic and genotoxic effects on ovarian cells, thereby interfering with the growth, maturation, and function of ovaries. This is evidenced by a series of studies carried out in Chinese hamster ovary (CHO) cells (34–36). IAA treatment not only increased the mutant frequency (MF) in ovarian cells, but also promoted the damage to genomic DNA and lowered the rate of DNA repair of ovarian cells (37, 38), which were partially attributed to oxidative stress. Because the antioxidant BHA or catalase could substantially reverse IAA-mediated genomic DNA damage of CHO cells (37). These findings are in agreement with that of a subsequent investigation. IAA treatment promoted reactive oxygen species (ROS) production and mitochondrial stress, and then contributed to genomic DNA damage as well as the suppression of cellular GAPDH, ATP, and pyruvate levels in ovarian cells (39, 40). Apart from DNA damage, Jiao et al. (41) uncovered IAA could disrupt mouse oocyte maturation by triggering abnormal spindle assembly and chromosome misalignment and causing the metaphase I arrest. In addition, several studies discovered that IAA not only restrained antral follicle growth and proliferation, estrogen receptor α (ERα) levels, and steroidogenesis, but also declined estradiol levels in mouse ovarian follicles (15, 42). In terms of underlying effects of IAA on estradiol and ERα levels, however, there are inconsistent findings. In human choriocarcinoma placental (JEG-3) cells, IAA exerted an estrogenic effect and then promoted estradiol levels in JEG-3 cells (43). Another study uncovered that IAA regulated ERα expressions in a species-specific manner. Specifically speaking, IAA showed the robust estrogenic activity on human ERα (hERα), whereas strongly refrained the estrogenic activity on zebrafish ERα (zERα) (44). We speculate that the specificity of species and cells may be the dominant reason for this disparity. Additionally, the difference in IAA concentrations used in these studies would also account for the inconsistence in part. For instance, the IAA concentration used in the study by Mestres et al. (43) was 0.01 to 0.5 μM, while that in the research by Jeong et al. (15) was 2 to 15 μM. Maybe, there exists a low-dose stimulant effect, which deserves further study.
In summary, a mass of toxicological evidence has convincingly demonstrated that IAA has the adverse effect on female reproductive health. IAA could postpone gonadal development, exert cytotoxic and genotoxic effects on ovarian cells, disrupt oocyte maturation, bring about genomic DNA damage, impede DNA repair, affect steroid hormone biosynthesis, etc. (Table 2). Similarly, it should be noted that there is a lack of population epidemiological research in the current evidence. Therefore, it is quite necessary to carry out some cohort studies in the future to search for direct evidence, which could reveal the reliable association between IAA exposure and female reproductive outcomes.
4 Potential mechanisms of IAA-mediated reproductive damage
At present, the modes of action by which IAA triggers harmful effects on reproductive health have not been clearly characterized. After reviewing the current toxicological evidence from in vivo and in vitro studies, we summarized that the potential mechanisms of IAA-mediated reproductive impairment predominantly consisted of cytotoxic and genotoxic effects on germ cells, disturbance of the hypothalamic–pituitary-gonadal (HPG) axis, oxidative stress, inhibition of steroidogenic proteins or enzymes, and dysbiosis of gut microbiota (Figure 2).
4.1 Cytotoxic and genotoxic effects
In recent years, IAA has been paid increasing attention because of its potent cytotoxicity and genotoxicity. Previous research has shown that IAA is more cytotoxic and genotoxic than the chlorinated and brominated analogues, such as chloroacetic acid and bromoacetic acid in mammalian cell assays (34). The study on human semen samples revealed that IAA exposure aroused apparently genotoxic and cytotoxic responses and then directly damaged sperm DNA, ultimately impairing semen quality (28). The similar finding was observed in IAA-exposed mice. After four-week exposure, IAA directly brought about DNA damage of spermatogenic cells and then decreased the average path velocity (VAP) and straight line velocity (VSL) of sperms (9). Our recent work has also indicated that the Leydig cell is one of the potential targets of IAA. Following IAA exposure, it not only reduced the amount of Leydig cells in rat testes, but also restrained the cell viability and growth (26). A large number of studies have suggested that IAA could damage female reproductive health by producing strong cytotoxicity and genotoxicity to germ cells. The study by Komaki et al. (38) uncovered that IAA led to the genomic DNA damage and lowered the rate of DNA repair of ovarian cells, thereby weakening ovarian functions. IAA treatment also directly injured oocytes of female mice by its cytotoxic and genotoxic effects, as evidenced by the abnormal spindle assembly and chromosome misalignment, metaphase I arrest, DNA damage, oocyte maturation disorder, etc. (41). Moreover, the antral follicle is also suggested to be the cytotoxic and genotoxic target of IAA, as IAA could restrain antral follicle growth and proliferation (15, 42). Therefore, it is not difficult to see that IAA could give rise to reproductive impairment via the directly cytotoxic and genotoxic effects on germ cells.
4.2 Disturbance of the hypothalamic–pituitary-gonadal axis
The hypothalamic–pituitary-gonadal (HPG) axis has pleotropic biological functions and its homeostasis plays an essential role in reproduction and fertility. Literature has indicated that the HPG axis may be one of potential targets of IAA. Xia et al. (20) discovered that IAA could perturb the development of the HPG axis-associated organs and then interfere with its functions. After 4 weeks of exposure to IAA, IAA directly increased the hypothalamic weight while decreased the ovarian weight in female mice. The findings are in agreement with a later study by Gonzalez et al. (33), who also put forward that IAA-caused female reproductive injury was partially attributed to the abnormality of the hypothalamic–pituitary-ovary (HPO) axis. After IAA treatment, the kisspeptin mRNA level in hypothalamic arcuate nuclei was induced, but the FSHβ-positive cell number and FSHβ mRNA level in pituitaries were both declined in female mice, thereby disordering the feedback regulation of the HPO axis. Likewise, in IAA-exposed male mice, the normal feedback regulation of the hypothalamic–pituitary-testis (HPT) axis was also broken down (9). The increase in LH levels secreted by the pituitary failed to stimulate the testis to biosynthesize more testosterone as expected, but instead led to a marginal downregulation. Similarly, the disturbance of the HPT axis was also observed in IAA-treated rats. Mou et al. (26) reported that after IAA exposure, plasma levels of GnRH produced by the hypothalamus and LH produced by the pituitary were not upregulated as expected to respond to the decline in testosterone by IAA. Thus, we suppose that IAA could impair reproductive health by interfering with the development and feedback regulation of the HPG axis.
4.3 Oxidative stress
Oxidative stress will happen when the generation of reactive oxygen species (ROS) prevails over the antioxidant capacity of cells. Oxidative stress functions significantly in a series of harmful reproductive outcomes, such as the inhibition of steroid hormone production, decline in semen quality, dysfunction in ovarian function, infertility, etc. (29, 45). Mounting literature from toxicological studies has indicated that oxidative stress contributes to the reproductive impairment mediated by IAA. Due to the insufficient antioxidant capacity and lack of DNA repair mechanisms, sperm is sensitive to and vulnerable to redox imbalance (46). Semen samples from healthy volunteers were treated with 25 μM of IAA for 1 h and the redox homeostasis of spermatids was destroyed, followed by the sperm DNA damage. However, antioxidants including BHA and catalase could effectively antagonize and alleviate oxidative stress-triggered injury effects on sperm (28). Apart from sperms, germ cells are also sensitive to oxidative stress. Following IAA exposure, IAA triggered DNA double-strand breaks of spermatogonia and Leydig cells in rat testes through oxidative stress, as evidenced by an increase in levels of 8-OHdG and γ-H2A.X (26). Several in vitro investigations also revealed that IAA treatment significantly stimulated ROS production and oxidative stress, subsequently resulting in genomic DNA damage of Chinese hamster ovary (CHO) cells and cell dysfunction (37, 39). Above evidence demonstrates that oxidative stress is one of common and important modes of action by which IAA leads to reproductive damage.
4.4 Inhibition of steroidogenic proteins or enzymes
It is known that steroidogenic proteins or enzymes play an essential role in steroid hormone biosynthesis. For instance, testosterone biosynthesis in Leydig cells is exquisitely catalyzed and modulated by a series of steroidogenic proteins or enzymes, such as SRB1, StAR, P450scc, P450c17, etc. Growing evidence has suggested that the steroidogenic proteins or enzymes are the susceptible target of environmental contaminants. The study by Duan et al. (47) disclosed that triclosan exposure inhibited the JAK1/STAT1 pathway via miR-142-5p to limit the transcription and translation of steroidogenic P450c17, thereby restraining testosterone biosynthesis. Another research uncovered that after beta-cypermethrin exposure, overexpressed intronic miR-140-5p negatively regulated steroidogenic StAR, P450scc, and 3β-HSD by targeting SF-1, thereby lowering testosterone levels in rat plasma (48). Likewise, some previous work exhibited that IAA exposure could inhibit steroidogenic protein expressions or enzyme activities. After 28 days of exposure to IAA, IAA depressed SRB1 protein expressions and then reduced the transport of lipid droplet into Leydig cells, leading to a decrease in raw materials for testosterone biosynthesis and a causal decline in testosterone levels in mouse serum (9). This finding is consistent with that of other investigations (22, 26, 42). Hence, these results manifest that IAA could disturb steroid hormone biosynthesis by affecting steroidogenic proteins or enzymes, thereby bringing about detrimental effects on reproductive health.
4.5 Dysbiosis of gut microbiota
With a deeper understanding of the gut microbiota, accumulating evidence has shown that the gut microbiota is closely involved in reproductive health and functions significantly through various means, such as gut metabolism, nutrition intake, ROS generation, inflammation (49). The study by Colldén et al. (50) uncovered that the gut microbiota took part in the synthesis and metabolism of androgen and acted as a dominant regulator of androgen metabolism in intestinal contents. Zhang et al. (51) disclosed that the gut microbiota could cross the blood-testis barrier to modulate spermatogenesis, putting forward that the gut microbiota could be employed to treat male infertility by improving the semen quality and spermatogenesis. Toxicological research suggests that exposure to certain DBPs could give rise to dysbiosis of gut microbiota in organisms. The study by Xue et al. (53) discovered that after exposure to dichloroacetamide (DCAcAm), an emerging disinfection byproduct, the gut microbiota composition was disordered in adult zebrafish. Similarly, IAA exposure facilitated gut microbiota dysbiosis, changed the steroid hormone biosynthesis-related gene abundance in the gut microbiota, and perturbed gut microbiota metabolism of male and female rats, ultimately functioning as an androgen disruptor (22). Together, IAA could adversely affect reproductive health through dysbiosis of gut microbiota, which might be considered as a novel mode of action.
5 Conclusion and perspectives
Reproductive health is closely related to the sustainable development of mankind and thus, the declining trend in human reproductive health has been an increasing concern all over the world. IAA, as an emerging unregulated iodinated disinfection byproduct, is frequently examined in drinking water and the detected concentration is up to 2.18 μg/L. In recent years, IAA has been considered as an androgen disruptor and may exert an estrogenic effect. Growing in vivo and in vitro toxicological studies have suggested a possible association between IAA exposure and multiple hazardous effects on reproductive health, such as impairing the sperm motility, disturbing steroidogenesis, triggering DNA damage to germ cells, interfering with oocyte maturation and estrous cyclicity, affecting gonadal development, etc. Although some progress has been made in exploring the impact of IAA on reproductive health, there are still some limitations that need to be further addressed. Firstly, sufficient population epidemiological data are absent from the available evidence. Therefore, it is quite meaningful to carry out necessary population cohort studies to reveal the correlation between IAA internal exposure levels and reproductive outcomes. Secondly, the sensitivity and reliability of methods for detecting the internal exposure level of IAA in organisms should be further improved and optimized. It was not until May 2024 that a latest work reported that the modified QuEChERS sample preparation combined with gas chromatography-tandem triple quadrupole mass spectrometry (GC–MS/MS) could successfully detect the internal exposure levels of IAA in various biological samples (plasma, urine, feces, liver, kidney, and other tissues) (27). With the further verification and application of the improved method, it is expected that more population studies on the internal exposure level of IAA will appear soon. Thirdly, most current toxicological studies are descriptive and cross-sectional, lacking in-depth mechanism dissection, which increases the difficulty of targeted intervention and prevention. Hence, mechanism-based exploration should be further advanced and deepened by adopting new techniques and methods like multi-omics, which is employed in reproductive research to better understand the cellular-level molecular mechanism related to gametes and the role of reproduction-related proteins (52). Taken together, this work preliminarily sheds light on the harmful effects of IAA on reproductive health, but we still have more work to do in the face of this emerging disinfection byproduct, IAA.
Author contributions
MH: Data curation, Formal analysis, Investigation, Methodology, Writing – original draft. LM: Data curation, Formal analysis, Investigation, Writing – original draft. JQ: Formal analysis, Investigation, Writing – original draft. CL: Conceptualization, Funding acquisition, Supervision, Validation, Writing – review & editing.
Funding
The author(s) declare that financial support was received for the research, authorship, and/or publication of this article. This study was funded by the Natural Science Foundation of Chongqing, China (cstc2022jxjl130001).
Conflict of interest
The authors declare that the research was conducted in the absence of any commercial or financial relationships that could be construed as a potential conflict of interest.
Publisher’s note
All claims expressed in this article are solely those of the authors and do not necessarily represent those of their affiliated organizations, or those of the publisher, the editors and the reviewers. Any product that may be evaluated in this article, or claim that may be made by its manufacturer, is not guaranteed or endorsed by the publisher.
References
1. Inhorn, MC, and Patrizio, P. Infertility around the globe: new thinking on gender, reproductive technologies and global movements in the 21st century. Hum Reprod Update. (2015) 21:411–26. doi: 10.1093/humupd/dmv016
2. Guo, Y, Chen, Q, Zhan, Y, Zhou, W, Zhang, H, Zhou, N, et al. Semen damage contributed over 50% to air-pollutant-induced infertility: a prospective cohort study of 3940 men in China. Sci Total Environ. (2023) 885:163532. doi: 10.1016/j.scitotenv.2023.163532
3. Laine, JE, Bailey, KA, Rubio-Andrade, M, Olshan, AF, Smeester, L, Drobná, Z, et al. Maternal arsenic exposure, arsenic methylation efficiency, and birth outcomes in the biomarkers of exposure to arsenic (BEAR) pregnancy cohort in Mexico. Environ Health Perspect. (2015) 123:186–92. doi: 10.1289/ehp.1307476
4. Lefebvre, T, Fréour, T, Duval, G, Ploteau, S, Marchand, P, le Bizec, B, et al. Associations between internal concentrations of fluorinated and organochlorinated chemicals in women and in vitro fertilization outcomes: a multi-pollutant study. Environ Pollut. (2022) 313:120087. doi: 10.1016/j.envpol.2022.120087
5. Qu, J, Wu, L, Mou, L, and Liu, C. Polystyrene microplastics trigger testosterone decline via GPX1. Sci Total Environ. (2024) 947:174536. doi: 10.1016/j.scitotenv.2024.174536
6. Rivera-Nunez, Z, Wright, JM, and Meyer, A. Exposure to disinfectant byproducts and the risk of stillbirth in Massachusetts. Occup Environ Med. (2018) 75:742–51. doi: 10.1136/oemed-2017-104861
7. Farré, MJ, Day, S, Neale, PA, Stalter, D, Tang, JYM, and Escher, BI. Bioanalytical and chemical assessment of the disinfection byproduct formation potential: role of organic matter. Water Res. (2013) 47:5409–21. doi: 10.1016/j.watres.2013.06.017
8. Deng, YL, Luo, Q, Liu, C, Zeng, JY, Lu, TT, Shi, T, et al. Urinary biomarkers of exposure to drinking water disinfection byproducts and ovarian reserve: a cross-sectional study in China. J Hazard Mater. (2022) 421:126683. doi: 10.1016/j.jhazmat.2021.126683
9. Liang, Y, Huang, X, Fang, L, Wang, M, Yu, C, and Guan, Q. Effect of iodoacetic acid on the reproductive system of male mice. Front Pharmacol. (2022) 13:958204. doi: 10.3389/fphar.2022.958204
10. Liu, XY, Zhang, M, Gu, XL, Deng, YL, Liu, C, Miao, Y, et al. Urinary biomarkers of drinking-water disinfection byproducts in relation to diminished ovarian reserve risk: a case-control study from the TREE cohort. Sci Total Environ. (2024) 912:168729. doi: 10.1016/j.scitotenv.2023.168729
11. Zhang, M, Liu, XY, Deng, YL, Liu, C, Zeng, JY, Miao, Y, et al. Associations between urinary biomarkers of exposure to disinfection byproducts and semen parameters: a repeated measures analysis. J Hazard Mater. (2023) 461:132638. doi: 10.1016/j.jhazmat.2023.132638
12. Allen, JM, Plewa, MJ, Wagner, ED, Wei, X, Bokenkamp, K, Hur, K, et al. Drivers of disinfection byproduct cytotoxicity in U.S. drinking water: should other DBPs be considered for regulation? Environ Sci Technol. (2022) 56:392–402. doi: 10.1021/acs.est.1c07998
13. Richardson, SD, and Plewa, MJ. To regulate or not to regulate? What to do with more toxic disinfection by-products? J Environ Chem Eng. (2020) 8:103939. doi: 10.1016/j.jece.2020.103939
14. Dong, H, Qiang, Z, and Richardson, SD. Formation of iodinated disinfection byproducts (I-DBPs) in drinking water: emerging concerns and current issues. Acc Chem Res. (2019) 52:896–905. doi: 10.1021/acs.accounts.8b00641
15. Jeong, CH, Gao, L, Dettro, T, Wagner, ED, Ricke, WA, Plewa, MJ, et al. Monohaloacetic acid drinking water disinfection by-products inhibit follicle growth and steroidogenesis in mouse ovarian antral follicles in vitro. Reprod Toxicol. (2016) 62:71–6. doi: 10.1016/j.reprotox.2016.04.028
16. Richardson, SD, Fasano, F, Ellington, JJ, Crumley, FG, Buettner, KM, Evans, JJ, et al. Occurrence and mammalian cell toxicity of iodinated disinfection byproducts in drinking water. Environ Sci Technol. (2008) 42:8330–8. doi: 10.1021/es801169k
17. Wei, X, Wang, S, Zheng, W, Wang, X, Liu, X, Jiang, S, et al. Drinking water disinfection byproduct iodoacetic acid induces tumorigenic transformation of NIH3T3 cells. Environ Sci Technol. (2013) 47:5913–20. doi: 10.1021/es304786b
18. Procházka, E, Escher, BI, Plewa, MJ, and Leusch, FDL. In vitro cytotoxicity and adaptive stress responses to selected haloacetic acid and halobenzoquinone water disinfection byproducts. Chem Res Toxicol. (2015) 28:2059–68. doi: 10.1021/acs.chemrestox.5b00283
19. Wang, J, Jia, R, Zheng, X, Sun, Z, Liu, R, and Zong, W. Drinking water disinfection byproduct iodoacetic acid interacts with catalase and induces cytotoxicity in mouse primary hepatocytes. Chemosphere. (2018) 210:824–30. doi: 10.1016/j.chemosphere.2018.07.061
20. Xia, Y, Mo, Y, Yang, Q, Yu, Y, Jiang, M, Wei, S, et al. Iodoacetic acid disrupting the thyroid endocrine system in vitro and in vivo. Environ Sci Technol. (2018) 52:7545–52. doi: 10.1021/acs.est.8b01802
21. Xiao, J, Sha, Y, Huang, Y, Long, K, Wu, H, Mo, Y, et al. Drinking water disinfection byproduct iodoacetic acid affects thyroid hormone synthesis in Nthy-ori 3-1 cells. Ecotoxicol Environ Saf. (2023) 257:114926. doi: 10.1016/j.ecoenv.2023.114926
22. Sha, Y, Wu, H, Guo, Y, Liu, X, Mo, Y, Yang, Q, et al. Effects of iodoacetic acid drinking water disinfection byproduct on the gut microbiota and its metabolism in rats. J Environ Sci. (2022) 117:91–104. doi: 10.1016/j.jes.2022.02.048
23. Long, K, Sha, Y, Mo, Y, Wei, S, Wu, H, Lu, D, et al. Androgenic and teratogenic effects of iodoacetic acid drinking water disinfection byproduct in vitro and in vivo. Environ Sci Technol. (2021) 55:3827–35. doi: 10.1021/acs.est.0c06620
24. Nan, Y, Zhang, Q, Ren, C, Huang, X, Gao, J, Li, XX, et al. Functional evaluation of iodoacetic acid induced photoreceptor degeneration in the cat. Sci China Life Sci. (2013) 56:524–30. doi: 10.1007/s11427-013-4483-3
25. Rösch, S, Johnen, S, Mazinani, B, Müller, F, Pfarrer, C, and Walter, P. The effects of iodoacetic acid on the mouse retina. Graefes Arch Clin Exp Ophthalmol. (2015) 253:25–35. doi: 10.1007/s00417-014-2652-0
26. Mou, L, Sun, D, Qu, J, Tan, X, Wang, S, Zeng, Q, et al. GRP78/IRE1 and cGAS/STING pathway crosstalk through CHOP facilitates iodoacetic acid-mediated testosterone decline. J Hazard Mater. (2024) 476:135101. doi: 10.1016/j.jhazmat.2024.135101
27. Yu, H, Wu, L, Xuan, D, Peng, Q, Qu, W, and Zhou, Y. Development and validation of a GC-MS/MS method for the determination of iodoacetic acid in biological samples. Anal Bioanal Chem. (2024) 416:3185–94. doi: 10.1007/s00216-024-05266-0
28. Ali, A, Kurzawa-Zegota, M, Najafzadeh, M, Gopalan, RC, Plewa, MJ, and Anderson, D. Effect of drinking water disinfection by-products in human peripheral blood lymphocytes and sperm. Mutat Res. (2014) 770:136–43. doi: 10.1016/j.mrfmmm.2014.08.003
29. Ha, M, Guan, X, Wei, L, Li, P, Yang, M, and Liu, C. Di-(2-ethylhexyl) phthalate inhibits testosterone level through disturbed hypothalamic-pituitary-testis axis and ERK-mediated 5α-reductase 2. Sci Total Environ. (2016) 563-564:566–75. doi: 10.1016/j.scitotenv.2016.04.145
30. Gonsioroski, A, Plewa, MJ, and Flaws, JA. Effects of prenatal and lactational exposure to iodoacetic acid on the F1 generation of mice. Biol Reprod. (2022) 107:650–63. doi: 10.1093/biolre/ioac079
31. Gonsioroski, A, Laws, M, Mourikes, VE, Neff, A, Drnevich, J, Plewa, MJ, et al. Iodoacetic acid exposure alters the transcriptome in mouse ovarian antral follicles. J Environ Sci. (2022) 117:46–57. doi: 10.1016/j.jes.2022.01.018
32. Gonsioroski, A, Meling, DD, Gao, L, Plewa, MJ, and Flaws, JA. Iodoacetic acid affects estrous cyclicity, ovarian gene expression, and hormone levels in mice. Biol Reprod. (2021) 105:1030–42. doi: 10.1093/biolre/ioab108
33. Gonzalez, RVL, Weis, KE, Gonsioroski, AV, Flaws, JA, and Raetzman, LT. Iodoacetic acid, a water disinfection byproduct, disrupts hypothalamic, and pituitary reproductive regulatory factors and induces toxicity in the female pituitary. Toxicol Sci. (2021) 184:46–56. doi: 10.1093/toxsci/kfab106
34. Plewa, MJ, Simmons, JE, Richardson, SD, and Wagner, ED. Mammalian cell cytotoxicity and genotoxicity of the haloacetic acids, a major class of drinking water disinfection by-products. Environ Mol Mutagen. (2010) 51:871–8. doi: 10.1002/em.20585
35. Plewa, MJ, Wagner, ED, Richardson, SD, Thruston, AD, Woo, YT, and McKague, AB. Chemical and biological characterization of newly discovered iodoacid drinking water disinfection byproducts. Environ Sci Technol. (2004) 38:4713–22. doi: 10.1021/es049971v
36. Zhang, SH, Miao, DY, Liu, AL, Zhang, L, Wei, W, Xie, H, et al. Assessment of the cytotoxicity and genotoxicity of haloacetic acids using microplate-based cytotoxicity test and CHO/HGPRT gene mutation assay. Mutat Res. (2010) 703:174–9. doi: 10.1016/j.mrgentox.2010.08.014
37. Cemeli, E, Wagner, ED, Anderson, D, Richardson, SD, and Plewa, MJ. Modulation of the cytotoxicity and genotoxicity of the drinking water disinfection byproduct iodoacetic acid by suppressors of oxidative stress. Environ Sci Technol. (2006) 40:1878–83. doi: 10.1021/es051602r
38. Komaki, Y, Pals, J, Wagner, ED, Mariñas, BJ, and Plewa, MJ. Mammalian cell DNA damage and repair kinetics of monohaloacetic acid drinking water disinfection by-products. Environ Sci Technol. (2009) 43:8437–42. doi: 10.1021/es901852z
39. Dad, A, Jeong, CH, Pals, JA, Wagner, ED, and Plewa, MJ. Pyruvate remediation of cell stress and genotoxicity induced by haloacetic acid drinking water disinfection by-products. Environ Mol Mutagen. (2013) 54:629–37. doi: 10.1002/em.21795
40. Dad, A, Jeong, CH, Wagner, ED, and Plewa, MJ. Haloacetic acid water disinfection byproducts affect pyruvate dehydrogenase activity and disrupt cellular metabolism. Environ Sci Technol. (2018) 52:1525–32. doi: 10.1021/acs.est.7b04290
41. Jiao, X, Gonsioroski, A, Flaws, JA, and Qiao, H. Iodoacetic acid disrupts mouse oocyte maturation by inducing oxidative stress and spindle abnormalities. Environ Pollut. (2021) 268:115601. doi: 10.1016/j.envpol.2020.115601
42. Gonsioroski, A, Meling, DD, Gao, L, Plewa, MJ, and Flaws, JA. Iodoacetic acid inhibits follicle growth and alters expression of genes that regulate apoptosis, the cell cycle, estrogen receptors, and ovarian steroidogenesis in mouse ovarian follicles. Reprod Toxicol. (2020) 91:101–8. doi: 10.1016/j.reprotox.2019.10.005
43. Mestres, J, Pérez-Albaladejo, E, Porte, C, and Postigo, C. High-throughput analysis of the steroid profile in placental cell cultures to evaluate endocrine disrupting effects of contaminant exposure. J Chromatogr A. (2022) 1667:462886. doi: 10.1016/j.chroma.2022.462886
44. Lee, SA, Park, CG, Esterhuizen, M, Choi, I, Ryu, CS, Yang, JH, et al. Investigating endocrine disrupting impacts of nine disinfection byproducts on human and zebrafish estrogen receptor alpha. Front Biosci. (2023) 28:48. doi: 10.31083/j.fbl2803048
45. Evans, EPP, Scholten, JTM, Mzyk, A, Reyes-San-Martin, C, Llumbet, AE, Hamoh, T, et al. Male subfertility and oxidative stress. Redox Biol. (2021) 46:102071. doi: 10.1016/j.redox.2021.102071
46. Tremellen, K . Oxidative stress and male infertility—a clinical perspective. Hum Reprod Update. (2008) 14:243–58. doi: 10.1093/humupd/dmn004
47. Duan, P, Huang, X, Ha, M, Li, L, and Liu, C. miR-142-5p/DAX1-dependent regulation of P450c17 contributes to triclosan-mediated testosterone suppression. Sci Total Environ. (2020) 717:137280. doi: 10.1016/j.scitotenv.2020.137280
48. Duan, P, Ha, M, Huang, X, Zhang, P, and Liu, C. Intronic miR-140-5p contributes to beta-cypermethrin-mediated testosterone decline. Sci Total Environ. (2022) 806:150517. doi: 10.1016/j.scitotenv.2021.150517
49. Li, X, Cheng, W, Shang, H, Wei, H, and Deng, C. The interplay between androgen and gut microbiota: is there a microbiota-gut-testis axis. Reprod Sci. (2022) 29:1674–84. doi: 10.1007/s43032-021-00624-0
50. Colldén, H, Landin, A, Wallenius, V, Elebring, E, Fändriks, L, Nilsson, ME, et al. The gut microbiota is a major regulator of androgen metabolism in intestinal contents. Am J Physiol Endocrinol Metab. (2019) 317:E1182–92. doi: 10.1152/ajpendo.00338.2019
51. Zhang, P, Feng, Y, Li, L, Ge, W, Yu, S, Hao, Y, et al. Improvement in sperm quality and spermatogenesis following faecal microbiota transplantation from alginate oligosaccharide dosed mice. Gut. (2021) 70:222–5. doi: 10.1136/gutjnl-2020-320992
52. Panner Selvam, MK, Baskaran, S, and Agarwal, A. Proteomics of reproduction: prospects and perspectives. Adv Clin Chem. (2019) 92:217–43. doi: 10.1016/bs.acc.2019.04.005
Keywords: drinking water disinfection byproduct, fertility, iodoacetic acid, reproductive damage, reproductive toxicant
Citation: Ha M, Mou L, Qu J and Liu C (2024) Impacts of iodoacetic acid on reproduction: current evidence, underlying mechanisms, and future research directions. Front. Public Health. 12:1434054. doi: 10.3389/fpubh.2024.1434054
Edited by:
Renata Sisto, National Institute for Insurance against Accidents at Work (INAIL), ItalyReviewed by:
Luisa Campagnolo, University of Rome Tor Vergata, ItalyLang Tran, Institute of Occupational Medicine, United Kingdom
Copyright © 2024 Ha, Mou, Qu and Liu. This is an open-access article distributed under the terms of the Creative Commons Attribution License (CC BY). The use, distribution or reproduction in other forums is permitted, provided the original author(s) and the copyright owner(s) are credited and that the original publication in this journal is cited, in accordance with accepted academic practice. No use, distribution or reproduction is permitted which does not comply with these terms.
*Correspondence: Changjiang Liu, Y2pfNTE0QDE2My5jb20=