- 1Faculty of Humanities and Arts, Macau University of Science and Technology, Taipa, Macau SAR, China
- 2State Key Laboratory of Quality Research in Chinese Medicines, Macau University of Science and Technology, Taipa, Macau SAR, China
- 3Faculty of Chinese Medicine, Macau University of Science and Technology, Taipa, Macau SAR, China
- 4Department of Comprehensive Surgery, Hengqin Hospital, The First Affiliated Hospital of Guangzhou Medical University, Guangdong-Macao in-Depth Cooperation Zone in Hengqin, Hengqin, China
- 5Department of Traditional Chinese Medicine, The Sixth Affiliated Hospital, Sun Yat-sen University, Guangzhou, China
- 6Centre for Epidemiology and Evidence-Based Practice, Department of Social and Preventive Medicine, Faculty of Medicine, University of Malaya, Kuala Lumpur, Malaysia
- 7Jiangsu Engineering Research Center of Cardiovascular Drugs Targeting Endothelial Cells, College of Health Sciences, School of Life Sciences, Jiangsu Normal University, Xuzhou, Jiangsu Province, China
- 8State Key Laboratory of Natural and Biomimetic Drugs, Peking University, Beijing, China
- 9Guangdong-Hong Kong-Macao Joint Laboratory for Contaminants Exposure and Health, Guangzhou, Guangdong Province, China
Lung cancer remains the leading cause of cancer-related mortality globally, with environmental pollutants identified as significant risk factors, especially for nonsmokers. The intersection of these pollutants with epigenetic mechanisms has emerged as a critical area of interest for understanding the etiology and progression of lung cancer. Epigenetic changes, including DNA methylation, histone modifications, and non-coding RNAs, can induce alterations in gene expression without affecting the DNA sequence and are influenced by environmental factors, contributing to the transformation of normal cells into malignant cells. This review assessed the literature on the influence of environmental pollutants on lung cancer epigenetics. A comprehensive search across databases such as PubMed, Web of Science, Cochrane Library, and Embase yielded 3,254 publications, with 22 high-quality papers included for in-depth analysis. These studies demonstrated the role of epigenetic markers, such as DNA methylation patterns of genes like F2RL3 and AHRR and alterations in the miRNA expression profiles, as potential biomarkers for lung cancer diagnosis and treatment. The review highlights the need to expand research beyond homogenous adult male groups typically found in high-risk occupational environments to broader population demographics. Such diversification can reduce biases and enhance the relevance of findings to various clinical contexts, fostering the development of personalized preventive and therapeutic measures. In conclusion, our findings underscore the potential of innovative epigenetic therapies, such as DNA demethylating drugs and histone modification agents, to counter environmental toxins’ carcinogenic effects. The growing interest in miRNA therapies and studies aiming to correct aberrant methylation patterns indicate significant strides toward better lung cancer management and a healthier future for global communities.
1 Introduction
Lung cancer is the most prevalent malignant tumor afflicting humanity, consistently occupying the highest rank in both global cancer incidence and mortality rates (1–3). According to GLOBOCAN estimates of incidence and mortality for 36 cancers in 185 countries worldwide, lung cancer is the most commonly diagnosed cancer in 2022, with nearly 2.5 million new cases and accounting for one in eight cancers worldwide (4). In our country, including the Macao Special Administrative Region, lung cancer ranks first in incidence and mortality rates among all malignant tumors (3, 5). Non-small cell lung cancer (NSCLC) comprises approximately 85% of all cases and includes adenocarcinoma, squamous cell carcinoma, and large cell carcinoma, among others (2, 6). Although smoking is a recognized primary risk factor for lung cancer, a significant number of lung cancer cases, particularly among Asian women who have never smoked, are associated with air pollution and environmental pollutants (7–9). These pollutants, including particulate matter, toxic metals, and nitrogen oxides, threaten everyone’s health (10–12). These pollutants penetrate the human respiratory system, potentially inducing epigenetic changes that lead to the transformation of normal cells into cancerous cells (13–16).
Due to the insidious onset of lung cancer, most patients are diagnosed at an advanced stage, missing the best opportunity for surgical treatment. Chemotherapy and targeted therapy are the main treatments for these patients. Chemotherapy often comes with many adverse reactions, and targeted therapy frequently leads to issues such as drug resistance, which results in many patients being unable to tolerate the drug treatment (10–12, 17). Over the past two decades, epigenetic research has advanced by leaps and bounds, offering a glimmer of hope for novel diagnostics and treatments of lung cancer. Epigenetics orchestrates the regulation of gene expression without altering the DNA sequence itself, thus revealing the intricate process of lung cancer formation from a genetic perspective. Alterations in epigenetics have been identified as crucial prognostic elements and potential therapeutic targets, with studies indicating that methylation patterns of specific genes, such as RASSF1A and RUNX3, are correlated with the prognosis and recurrence of lung cancer (16, 18). Epigenetic modifications, such as DNA methylation, histone modification, and non-coding RNAs, play a pivotal role in the onset and progression of lung cancer by regulating gene expression, impacting cell cycles, genomic imprinting, and X-chromosome inactivation (19–22). A deeper understanding of the underlying biological pathways elucidates how environmental pollutants induce such epigenetic changes. For instance, the p16INK4a pathway, often silenced by promoter hypermethylation, is crucial for cell cycle regulation and is frequently altered in lung carcinogenesis. Similarly, the PI3K/AKT signaling pathway can be activated by the demethylation of certain genes, contributing to tumorigenesis. Mediators such as reactive oxygen species (ROS) generated by pollutants can lead to oxidative stress, subsequently causing DNA damage and altered methylation patterns. Additionally, histone deacetylases (HDACs) and DNA methyltransferases (DNMTs) have been identified as critical enzymes that mediate these epigenetic changes, making them potential targets for therapeutic interventions. Hence, contemporary etiological studies of lung cancer are also focusing on the intersection of environmental pollutants and epigenetic mechanisms. An in-depth examination of these mechanisms provides novel strategies for treating lung cancer (23–25).
As industrialization accelerates, the incidence and mortality rates of lung cancer have surged dramatically, necessitating the implementation of urgent public health measures and innovative research to prevent and combat this disease (26–28). This article encapsulates the impact of environmental pollutants on the epigenetic alterations associated with lung cancer, and the underlying physiological mechanisms induced by these contaminants (Figure 1). Understanding the molecular mechanics of epigenetic changes and their correlation with environmental pollutants can pave the way for the development of novel therapeutics and preventive measures for lung cancer, ultimately enhancing patient survival quality and prognosis, and prolonging patient lifespan (29–32).
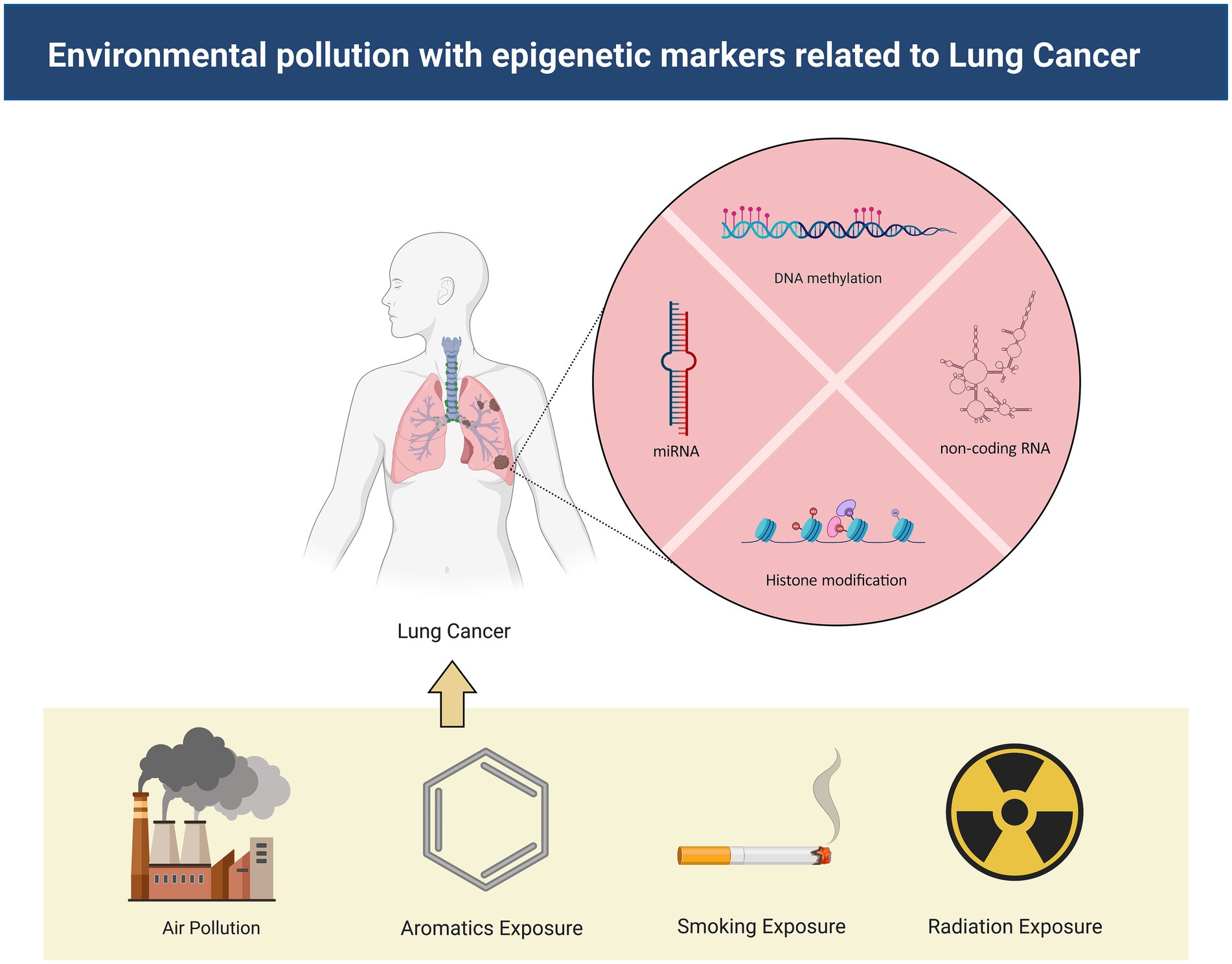
Figure 1. The mechanisms by which environmental pollutants lead to lung cancer. Environmental contaminants exert influence on non-coding RNA, DNA methylation, and histone modification, thereby instigating the onset of lung cancer.
2 Methods
2.1 Search strategy
Utilizing the electronic databases PubMed, Web of Science, Cochrane Library, and Embase, we carried out an exhaustive literature search for publications released prior to November 2023. The search was conducted using key terms that encompass the following: 1: environmental pollution (environmental biomarkers, air pollution, radiation, tobacco smoke pollution, aromatic hydrocarbons); 2: epigenetics (DNA methylation, histone, non-coding RNA); 3: lung cancer (primary bronchogenic carcinoma, non-small cell lung cancer, small cell lung cancer). The schema for the search is depicted in Table 1. Additionally, manual searches were also performed within the bibliographies of published articles and reviews. Adhering to the inclusion criteria, we discussed 22 high-quality papers from the initial pool of 3,254. The outcome of the search and the inclusion and exclusion process are shown in Figure 2.
2.2 Inclusion and exclusion criteria
The criteria for inclusion of literature were as follows: (1) Studies that exclusively investigate primary bronchogenic carcinoma; (2) Research defining air pollution factors according to the “Environmental Health” (33) criteria established by the World Health Organization, which includes environmental biomarkers, air pollution, radiation, tobacco smoke pollution, and aromatic hydrocarbons; (3) Studies involving any epigenetic mechanisms, inclusive of DNA methylation, histone modifications, and non-coding RNA; (4) Research conducted in human subjects; (5) The study designs included analyses of cohort, cross-sectional and longitudinal studies, as well as randomized, non-randomized and semi-randomized studies.
Exclusion criteria were: (1) Literature pertaining to metastatic lung cancer or studies not concerning lung cancer; (2) Studies without relevant environmental pollutant exposure; (3) Research lacking examination of epigenetic mechanisms; (4) Animal studies; (5) Academic theses, conference abstracts, books, reports, or non-empirical articles.
2.3 Data extraction
Two reviewers, A.J. Zhang and X.X. Luo, independently screened the titles, abstracts, and full texts of the retrieved articles, and sorted out the studies that met the inclusion criteria. Any disagreements between reviewers were resolved by discussion and reaching a consensus, with the contribution of a third independent reviewer, Y. Li, made the final decision when necessary.
Data were extracted from the included literature using a standardized data extraction form. The collected information included: (1) Basic details: author’s name, year of publication, region of publication, and number of cases included; (2) Type of study; (3) Clinical and pathological data of participants; (4) Experimental methods; (5) Outcome measures.
2.4 Assessment of evidence quality
As the included articles employed disparate methodological approaches, we employed a multi-method quality framework to assess the quality of the articles according to standardized criteria (34). The framework was categorized into four main quality categories: truth value, applicability, consistency and neutrality. In addition, we considered the context of the study, potential benefits and harms, and patient value systems when interpreting the results. A score was assigned to each category, with the average score across the four categories indicating that the overall quality of the article was rated as robust, high, moderate, low, or very low.
3 Results
The search terms identified a total of 3,254 articles. A rigorous selection process led to the exclusion of some studies: 10 concerning metastatic lung cancer or not involving lung cancer, 7 with undefined types of environmental pollutants, 15 lacking examinations of epigenetic mechanisms, 14 based on non-human subjects, and 11 non-empirical articles. Ultimately, 22 published studies met the predetermined inclusion criteria (Table 2).
3.1 Characteristics of the included studies
Tables 2, 3 summarize the detailed characteristics of the included studies. According to quality assessment standards, six studies were of good quality, and 16 were of moderate quality. The identified research employed a variety of methods to detect alterations in lung cancer epigenetics: 16 studies conducted assays of specific candidate genes as shown in Table 4, which included F2RL3 and AHRR (35–38), CDKN2A, DLEC1, CDH1, DAPK, RUNX3, APC, WIF1, and MGMT (39), SATα, NBL2, and D4Z4 (40, 41), DNMT1, DNMT3a, DNMT3b, TET1, TET2, TET3 (42), L3MBTL1, NNAT, PEG10, GNAS Ex1A, MCTS2, SNURF/SNRPN, IGF2R, RB1, and CYP1B1 (43), CYP1A1 (44), miRNA (45–50). Additionally, some studies assessed the impact of environmental pollutants using different mediators, such as raised levels of DNA methyltransferase enzyme (DNA MTase) (51) and chemical modifications 5mC and 5hmC (52); 1 study conducted a whole-genome DNA methylation analysis using the Illumina Infinium HumanMethylation450 platform (36); 4 studies reviewed the effect of environmental pollutants on epigenetics (53–56).
3.2 Participant demographics
The inquiries predominantly explored the demographic of male adults, with a preponderance of professions including drivers and laborers. These investigations unveiled a considerable overlap in samples, as three studies (40, 41, 52) recruited truck drivers from China for analysis. The pathological phenotypes were most frequently assessed through DNA methylation patterns, some of which involved levels of gene expression. However, other epigenetic pathways, such as histone modifications or non-coding RNAs, have yet to be thoroughly examined.
3.3 Types of pertinent environmental pollutants
According to the research conducted by Xue et al. (57), environmental pollutants associated with lung cancer are categorized into two types: outdoor and indoor air pollutants (Table 5).
3.3.1 Outdoor air pollutants
Eight studies identified within the pertinent body of research analyzed the hazards posed by outdoor environmental pollutants, focusing primarily on particulate matter (PMs) and polycyclic aromatic hydrocarbons (PAHs) (35, 40, 41, 43, 45, 52, 53, 58). The composition of atmospheric particulates is complex, encompassing organic compounds (such as polycyclic aromatic hydrocarbons, dioxins, and benzene) and inorganic substances (like carbon, chlorides, nitrates, and sulfates) and metals. Due to their substantial surface area and robust adsorption capacity, PMs not only carry toxic metals and organic constituents but can also adsorb bacteria and virus (59). These pollutants enter the lungs via the respiratory system and could potentially elevate the risk of developing lung cancer.
3.3.2 Indoor air pollutants
An additional 10 studies discussed the possibility of indoor environmental pollutants—including tobacco smoke and coal for curing—inducing epigenetic modifications associated with lung cancer (36–39, 44, 46, 48–51). Indoor smoking and exposure to secondhand smoke are significant risk factors for lung cancer. Long-term exposure to environmental tobacco smoke, including secondhand aerosols from tobacco or electronic cigarettes, increases the risk of lung cancer (49, 51). Moreover, some reports suggest that the cumulative toxicity of co-existing air pollutants is an important consideration to take into account (57).
3.4 Alterations in the epigenetics of lung cancer
Epigenetic alterations—including changes in microRNAs (miRNAs), DNA methylation, and histone modifications—are major determinants in the development of disease phenotypes following exposure to air pollution (53, 55). The different types of lung cancer driven by epigenetic changes driven by environmental pollutants are shown in Figure 3.
3.4.1 The mechanisms of DNA methylation in driving lung cancer
DNA methylation represents a significant epigenetic change underlying the pathogenic mechanisms induced by air pollution (55). Changes in DNA methylation occurred after exposure to PMs (56). PM2.5 exposure suppresses p53 expression through promoter hypermethylation mediated by the ROS-protein kinase B (Akt)-DNMT3B pathway, suggesting that PM2.5 exposure could increase the risk of lung cancer (53). Furthermore, compounds produced by smoking (36–38) and perfluoroalkyl substances [PFAS, (42)] have been shown to affect gene expression in lung cancer cells by altering DNA methylation patterns. This alteration may lead to dysregulation of the cell cycle and apoptosis pathways, thereby promoting the onset and progression of lung cancer. The A549 lung cancer cell line serves as a research model, providing crucial experimental evidence for understanding how these environmental factors impact lung cancer (42, 44).
3.4.2 The mechanisms of histone modifications in driving lung cancer
Some studies also show that differential histone modifications involve PM-induced inflammatory responses and oxidative stress, particularly leading to pulmonary diseases (53). Long-term exposure to PM2.5 downregulates the expression of histone demethylase Kdm6a in lung macrophages, which may result in increased methylation of H3K4 and H3K9 in the promoter regions of IL-6 and IFN-β. Exposure to cigarette smoke reduces the activity of histone deacetylases (HDACs) and decreases the expression of HDAC1, HDAC2, and HDAC3 in macrophages, resulting in an inflammatory response. Exposure to particulates leads to an imbalance in the expression of histone acetyltransferases (HATs) and HDACs in human bronchial epithelial cells, as well as an increase in the acetylation of certain histones, such as H4, which in turn triggers inflammation (55).
3.4.3 The mechanisms of miRNAs in driving lung cancer
Sima et al. (47) analyzed the expression of miRNAs associated with exposure to air pollutants and lung cancer. Twenty-five miRNAs were correlated with exposure to air pollution and lung cancer, with miR-222, miR-21, miR-126-3p, miR-155, and miR-425 being the most significant. They play pivotal roles in promoting or inhibiting angiogenesis, inflammation, and the progression of lung cancer. Additionally, a specific set of upregulated or downregulated miRNAs was observed in the progression of bronchogenic carcinoma in smokers, ranging from normal lung to hyperplasia, metaplasia, carcinoma in situ, and finally, to lung squamous cell carcinoma (LUSC) (46). Exposure to cigarette smoke leads to an early, pronounced reduction in the expression of the tumor-suppressor miR-487b through promoter methylation, thereby facilitating lung oncogenesis through Wnt signaling (50). Similarly, tumor suppressor miR-196b is silenced early through promoter methylation in the same experimental model, giving a selective growth advantage to precancerous cells. A case–control study showed a strong correlation between methylation of miR-196b in sputum and the occurrence of lung cancer (48).
4 Discussion
Epigenetic characteristics mirror the shifts in cellular environments and are also discernible within the human circulatory system across a spectrum of diseases (60, 61). Exploration of these epigenetic attributes may lead to the identification of sensitive biomarkers, which hold promise for the early screening of lung cancer as well as the monitoring of the clinical treatment outcomes. This study is dedicated to revisiting the extensive interplay between environmental pollutants and lung cancer, with a comprehensive analysis of current research highlighting the crucial role that epigenetic modifications play in the etiology of lung cancer. Specifically, DNA methylation of genes such as F2RL3 and AHRR is accentuated (62–65), aberrant miRNA expression patterns stand out as additional key epigenetic markers (49, 66–68). In the future, these could serve as potential targets for diagnosing and treating lung cancer. However, such studies are predominantly confined to specific demographics, primarily consisting of adult males exposed to highly polluted environments, which may introduce biases and affect the objectivity of the data.
Corresponding research indicates that the impact of environmental pollutants on diverse populations is multifaceted, with contributing factors encompassing genetic characteristics, occupation, lifestyle choices, and socioeconomic status (69). In particular, alterations in DNA methylation at specific genomic loci constitute a fundamental aspect of the initiation and progression of lung cancer (70–73). Epigenetic variations observed within the CDKN2A gene, engendered by exogenous environmental elements, exemplify the paradigmatic mechanisms of tumor formation instigated by external environmental factors through the genesis of heterotypic cells (74).
The horizon of avant-garde therapeutic approaches brims with potential. Although minimization of exposure remains an unwavering pillar, the advent of molecular treatment regimens, ingeniously devised to rectify epigenetic aberrations, heralds a significant leap forward in therapeutic innovation. Pertaining to DNA demethylation (75–77), histone modification pharmacologic (75, 78, 79) and miRNA therapeutic interventions (54, 80, 81). Research into histone-modifying drugs and miRNA therapies may revolutionize the treatment approaches for individuals exposed to environmental toxins, heralding a paradigm shift in managing pollution-related lung cancer. The development of these treatments necessitates rigorous investigations to ascertain their safety and efficacy. Clinical trials examining the effectiveness of agents like azacitidine in correcting methylation patterns associated with pollution-induced lung malignancies are imperative (82, 83), as well as clinical trials evaluating the effectiveness of drugs like azacitidine in correcting methylation patterns in pollution-related lung cancers. Further assessment of the anti-inflammatory properties of HDAC inhibitors is also imperative (84, 85). Such endeavors in therapeutic experimentation bear the potential to catalyze transformative changes in care for individuals plagued by environmental toxins. Consequently, the research must be conducted meticulously to ensure beneficial outcomes.
4.1 Limitations
The limitations of our review merit recognition and warrant attention. Initially, the caliber of evidence extracted from the 18 documents included was heterogeneous, with some studies potentially needing more rigorous methodological design, comprehensive data collection, or extensive peer-review processes. Such imperfections in quality may impinge upon the reliability and universality of the research findings, as lower quality investigations could introduce biases or overlook critical variables, our literature search was confined solely to published articles in English, introducing a language bias that may have excluded pertinent studies published in other tongues, which could provide insights into the epigenetic impacts of environmental pollutants on lung cancer. Consequently, our findings do not encompass the complete scope of global research and may lead to an incomplete understanding of the subject matter.
The robustness of the discussions presented might also be questioned, as they may not have considered all alternative explanations, counterarguments, or the full breadth of complex interactions between environmental pollutants and genetic susceptibility across different populations. The discussions may also need more comprehensiveness in resolving the heterogeneity of the study populations and methodologies, potentially limiting the strength of the conclusions drawn.
These limitations underscore the necessity for a cautious interpretation of the review outcomes. Future research should strive to include a broader scope of studies, encompassing multiple languages and more diverse populations, to offer a more comprehensive understanding of the effects of environmental pollutants on lung cancer through epigenetic alterations. Furthermore, ensuring that discussions in future reviews are grounded in extensive consideration of all pertinent factors and opposing viewpoints will enhance the research findings’ validity and practical applicability.
5 Conclusion
The current review delves into an increasing body of evidence that underscores how environmental pollutants act as catalysts for carcinogenesis within pulmonary tissues, focusing on epigenetic mechanisms. Studies on epigenetic markers—particularly DNA methylation of pivotal genes such as F2RL3 and AHRR, as well as alterations in miRNA profiles affecting gene expression—have emerged as significant indicators for diagnosing and treating lung cancer. However, focusing solely on homogenous male adult populations within specific high-risk occupational environments may fall short of a comprehensive picture, as it fails to encapsulate the demographic and occupational diversity prevalent in a broader population base. Additionally, as suggested by prior comprehensive reviews, these epigenetic characteristics may extend beyond the biomarkers for lung cancer, representing the organism’s response to environmental stressors.
In light of these findings, it is imperative to expand the research scope to include more diverse population groups, thereby mitigating the risk of biased data that may not represent the entirety of vulnerable cohorts. Widening the demographic reach of these studies can greatly enhance the validity of research outcomes and facilitate their application across varied clinical settings. Moreover, it allows for formulating of personalized preventive measures and interventions, considering the intricate interplay products between unique epigenomic landscapes, environmental exposures, lifestyles, and genetic susceptibilities.
Looking ahead, the pursuit of innovative treatments such as drugs targeting DNA demethylation and histone modification offers new avenues for combatting pollution-induced malignancies. Rigorous scrutiny and clinical trials of these emerging therapeutic modalities, coupled with the burgeoning interest in miRNA therapies, highlight their potential to significantly impact on individuals affected by the deleterious effects of environmental toxins. Research aimed at correcting aberrant methylation patterns with drugs like azacitidine, as well as exploring the anti-inflammatory properties of HDAC inhibitors represent scientific endeavors and steps toward a healthier future for the global community.
Author contributions
AZ: Conceptualization, Data curation, Methodology, Writing – original draft. XL: Conceptualization, Investigation, Writing – original draft. YL: Conceptualization, Methodology, Writing – review & editing. LY: Conceptualization, Data curation, Writing – original draft. XiL: Data curation, Writing – review & editing. QY: Investigation, Writing – review & editing. ZZ: Data curation, Writing – review & editing. GH: Resources, Supervision, Validation, Writing – review & editing. ZL: Project administration, Supervision, Validation, Writing – review & editing. QW: Funding acquisition, Project administration, Supervision, Validation, Writing – review & editing. JW: Project administration, Supervision, Validation, Writing – review & editing.
Funding
The author(s) declare that financial support was received for the research, authorship, and/or publication of this article. This work was supported by grants from the Science and Technology Development Fund, Macau SAR (File No. 0098/2021/A2 and 0048/2023/AFJ), Macau University of Science and Technology’s Faculty Research Grant (No. FRG-23-003-FC, FRG-24-049-FA) and Open funding of Guangdong-Hong Kong-Macao Joint Laboratory for Contaminants Exposure and Health (No. GHMJLCEH-09).
Conflict of interest
The authors declare that the research was conducted in the absence of any commercial or financial relationships that could be construed as a potential conflict of interest.
Publisher’s note
All claims expressed in this article are solely those of the authors and do not necessarily represent those of their affiliated organizations, or those of the publisher, the editors and the reviewers. Any product that may be evaluated in this article, or claim that may be made by its manufacturer, is not guaranteed or endorsed by the publisher.
References
1. Bray, F, Ferlay, J, Soerjomataram, I, Siegel, RL, Torre, LA, and Jemal, A. Global cancer statistics 2018: GLOBOCAN estimates of incidence and mortality worldwide for 36 cancers in 185 countries. CA Cancer J Clin. (2018) 68:394–424. doi: 10.3322/caac.21492
2. Jonna, S, and Subramaniam, DS. Molecular diagnostics and targeted therapies in non-small cell lung cancer (NSCLC): an update. Discov Med. (2019) 27:167–70.
3. Xia, C, Dong, X, Li, H, Cao, M, Sun, D, He, S, et al. Cancer statistics in China and United States, 2022: profiles, trends, and determinants. Chin Med J. (2022) 135:584–90. doi: 10.1097/CM9.0000000000002108
4. Bray, F, Laversanne, M, Sung, H, Ferlay, J, Siegel, RL, Soerjomataram, I, et al. Global cancer statistics 2022: GLOBOCAN estimates of incidence and mortality worldwide for 36 cancers in 185 countries. CA Cancer J Clin. (2024) 74:229–63. doi: 10.3322/caac.21834
5. Taskforce for Annual Report of Macao Cancer Registry. (2020). 2020 Annual Report of Macao Cancer Registry. Available at: https://ssm.gov.mo/docs/25291/25291_83af9211caf1406abead778c4f9b9574_000.pdf (Accessed August 1, 2022).
6. Ettinger, DS, Wood, DE, Akerley, W, Bazhenova, LA, Borghaei, H, Camidge, DR, et al. Non-small cell lung Cancer, version 6.2015. J National Comprehensive Cancer Network: JNCCN. (2015) 13:515–24. doi: 10.6004/jnccn.2015.0071
7. Tseng, C-H, Tsuang, B-J, Chiang, C-J, Ku, K-C, Tseng, J-S, Yang, T-Y, et al. The relationship between air pollution and lung Cancer in nonsmokers in Taiwan. J Thoracic Oncol: Official Pub Int Association For the Study of Lung Cancer. (2019) 14:784–92. doi: 10.1016/j.jtho.2018.12.033
8. Wang, N, Mengersen, K, Tong, S, Kimlin, M, Zhou, M, Wang, L, et al. Short-term association between ambient air pollution and lung cancer mortality. Environ Res. (2019) 179:108748. doi: 10.1016/j.envres.2019.108748
9. Xing, DF, Xu, CD, Liao, XY, Xing, TY, Cheng, SP, Hu, MG, et al. Spatial association between outdoor air pollution and lung cancer incidence in China. BMC Public Health. (2019) 19:1377. doi: 10.1186/s12889-019-7740-y
10. Aokage, K, Yoshida, J, Hishida, T, Tsuboi, M, Saji, H, Okada, M, et al. Limited resection for early-stage non-small cell lung cancer as function-preserving radical surgery: a review. Jpn J Clin Oncol. (2017) 47:7–11. doi: 10.1093/jjco/hyw148
11. Donington, JS, Koo, CW, and Ballas, MS. Novel therapies for non-small cell lung cancer. J Thorac Imaging. (2011) 26:175–85. doi: 10.1097/RTI.0b013e3182161709
12. Hirsch, FR, Scagliotti, GV, Mulshine, JL, Kwon, R, Curran, WJ, Wu, Y-L, et al. Lung cancer: current therapies and new targeted treatments. Lancet (London, England). (2017) 389:299–311. doi: 10.1016/S0140-6736(16)30958-8
13. Kato, A, Shimizu, K, Shimoichi, Y, Fujii, H, Honoki, K, and Tsujiuchi, T. Aberrant DNA methylation of E-cadherin and p16 genes in rat lung adenocarcinomas induced by N-nitrosobis(2-hydroxypropyl)amine. Mol Carcinog. (2006) 45:106–11. doi: 10.1002/mc.20162
14. Shimizu, K, Kato, A, Shigemura, M, Fujii, H, Honoki, K, and Tsujiuchi, T. Aberrant methylation patterns of the Rassf1a gene in rat lung adenocarcinomas induced by N-nitrosobis(2-hydroxypropyl)amine. Mol Carcinog. (2006) 45:112–7. doi: 10.1002/mc.20173
15. Shimizu, K, Shigemura, M, Fujii, H, Honoki, K, and Tsujiuchi, T. Reduced expression of the Connexin26 gene and its aberrant DNA methylation in rat lung adenocarcinomas induced by N-nitrosobis(2-hydroxypropyl)amine. Mol Carcinog. (2006) 45:710–4. doi: 10.1002/mc.20207
16. Yanagawa, N, Tamura, G, Oizumi, H, Kanauchi, N, Endoh, M, Sadahiro, M, et al. Promoter hypermethylation of RASSF1A and RUNX3 genes as an independent prognostic prediction marker in surgically resected non-small cell lung cancers. Lung Cancer (Amsterdam, Netherlands). (2007) 58:131–8. doi: 10.1016/j.lungcan.2007.05.011
17. Duma, N, Santana-Davila, R, and Molina, JR. Non-small cell lung Cancer: epidemiology, screening, diagnosis, and treatment. Mayo Clin Proc. (2019) 94:1623–40. doi: 10.1016/j.mayocp.2019.01.013
18. Brock, MV, Hooker, CM, Ota-Machida, E, Han, Y, Guo, M, Ames, S, et al. DNA methylation markers and early recurrence in stage I lung cancer. N Engl J Med. (2008) 358:1118–28. doi: 10.1056/NEJMoa0706550
19. Christiani, DC. Ambient air pollution and lung Cancer: nature and nurture. Am J Respir Crit Care Med. (2021) 204:752–3. doi: 10.1164/rccm.202107-1576ED
20. Orru, H, Ebi, KL, and Forsberg, B. The interplay of climate change and air pollution on health. Current Environ Health Reports. (2017) 4:504–13. doi: 10.1007/s40572-017-0168-6
21. Tang, W, Pei, Y, Zheng, H, Zhao, Y, Shu, L, and Zhang, H. Twenty years of China's water pollution control: experiences and challenges. Chemosphere. (2022) 295:133875. doi: 10.1016/j.chemosphere.2022.133875
22. The, L. UK air pollution and public health. Lancet (London, England). (2017) 389:1860. doi: 10.1016/S0140-6736(17)31271-0
23. Hascher, A, Haase, A-K, Hebestreit, K, Rohde, C, Klein, H-U, Rius, M, et al. DNA methyltransferase inhibition reverses epigenetically embedded phenotypes in lung cancer preferentially affecting polycomb target genes. Clin Cancer Res: Official J American Association For Cancer Res. (2014) 20:814–26. doi: 10.1158/1078-0432.CCR-13-1483
24. Lantuéjoul, S, Salameire, D, Salon, C, and Brambilla, E. Pulmonary preneoplasia--sequential molecular carcinogenetic events. Histopathology. (2009) 54:43–54. doi: 10.1111/j.1365-2559.2008.03182.x
25. Wistuba, II, and Gazdar, AF. Lung cancer preneoplasia. Annu Rev Pathol. (2006) 1:331–48. doi: 10.1146/annurev.pathol.1.110304.100103
26. Chen, W, Zheng, R, Baade, PD, Zhang, S, Zeng, H, Bray, F, et al. Cancer statistics in China, 2015. CA Cancer J Clin. (2016) 66:115–32. doi: 10.3322/caac.21338
27. Jemal, A, Bray, F, Center, MM, Ferlay, J, Ward, E, and Forman, D. Global cancer statistics. CA Cancer J Clin. (2011) 61:69–90. doi: 10.3322/caac.20107
28. Siegel, RL, Miller, KD, and Jemal, A. Cancer statistics, 2016. CA Cancer J Clin. (2016) 66:7–30. doi: 10.3322/caac.21332
29. Duan, J, Zhong, B, Fan, Z, Zhang, H, Xu, M, Zhang, X, et al. DNA methylation in pulmonary fibrosis and lung cancer. Expert Rev Respir Med. (2022) 16:519–28. doi: 10.1080/17476348.2022.2085091
30. Liang, W, Zhao, Y, Huang, W, Gao, Y, Xu, W, Tao, J, et al. Non-invasive diagnosis of early-stage lung cancer using high-throughput targeted DNA methylation sequencing of circulating tumor DNA (ctDNA). Theranostics. (2019) 9:2056–70. doi: 10.7150/thno.28119
31. Tsujiuchi, T, Masaoka, T, Sugata, E, Onishi, M, Fujii, H, Shimizu, K, et al. Hypermethylation of the dal-1 gene in lung adenocarcinomas induced by N-nitrosobis(2-hydroxypropyl)amine in rats. Mol Carcinog. (2007) 46:819–23. doi: 10.1002/mc.20316
32. Yang, Z, Qi, W, Sun, L, Zhou, H, Zhou, B, and Hu, Y. DNA methylation analysis of selected genes for the detection of early-stage lung cancer using circulating cell-free DNA. Advan Clin Experimental Med: Official Organ Wroclaw Medical University. (2019) 28:355–60. doi: 10.17219/acem/84935
33. The World Health Organization (WHO). (2023). Ambient air pollution data [database]. Home/data/GHO/themes/air pollution data portal/ambient air pollution data. Retrieved from https://www.who.int/data/gho/data/themes/air-pollution/ambient-air-pollution (Accessed on December 1, 2023)
34. Attard, A, and Larkin, M. Art therapy for people with psychosis: a narrative review of the literature. Lancet Psychiatry. (2016) 3:1067–78. doi: 10.1016/S2215-0366(16)30146-8
35. Alhamdow, A, Essig, YJ, Krais, AM, Gustavsson, P, Tinnerberg, H, Lindh, CH, et al. Fluorene exposure among PAH-exposed workers is associated with epigenetic markers related to lung cancer. Occup Environ Med. (2020) 77:488–95. doi: 10.1136/oemed-2020-106413
36. Baglietto, L, Ponzi, E, Haycock, P, Hodge, A, Bianca Assumma, M, Jung, CH, et al. DNA methylation changes measured in pre-diagnostic peripheral blood samples are associated with smoking and lung cancer risk. Int J Cancer. (2017) 140:50–61. doi: 10.1002/ijc.30431
37. Fasanelli, F, Baglietto, L, Ponzi, E, Guida, F, Campanella, G, Johansson, M, et al. Hypomethylation of smoking-related genes is associated with future lung cancer in four prospective cohorts. Nat Commun. (2015) 6:10192. doi: 10.1038/ncomms10192
38. Lee, DH, Hwang, SH, Lim, MK, Oh, JK, Song, DY, Yun, EH, et al. Performance of urine cotinine and hypomethylation of AHRR and F2RL3 as biomarkers for smoking exposure in a population-based cohort. PLoS One. (2017) 12:e0176783. doi: 10.1371/journal.pone.0176783
39. Huang, X, Wu, C, Fu, Y, Guo, L, Kong, X, and Cai, H. Methylation analysis for multiple gene promoters in non-small cell lung cancers in high indoor air pollution region in China. Bull Cancer. (2018) 105:746–54. doi: 10.1016/j.bulcan.2018.05.004
40. Guo, L, Byun, HM, Zhong, J, Motta, V, Barupal, J, Zheng, Y, et al. Effects of short-term exposure to inhalable particulate matter on DNA methylation of tandem repeats. Environ Mol Mutagen. (2014) 55:322–35. doi: 10.1002/em.21838
41. Hou, L, Zhang, X, Zheng, Y, Wang, S, Dou, C, Guo, L, et al. Altered methylation in tandem repeat element and elemental component levels in inhalable air particles. Environ Mol Mutagen. (2014) 55:256–65. doi: 10.1002/em.21829
42. Jabeen, M, Fayyaz, M, and Irudayaraj, J. Epigenetic modifications, and alterations in cell cycle and apoptosis pathway in A549 lung carcinoma cell line upon exposure to perfluoroalkyl substances. Toxics. (2020) 8:112. doi: 10.3390/toxics8040112
43. Liang, Y, Hu, L, Li, J, Liu, F, Jones, KC, Li, D, et al. Short-term personal PM2. 5 exposure and change in DNA methylation of imprinted genes: panel study of healthy young adults in Guangzhou city, China. Environ Pollut. (2021) 275:116601. doi: 10.1016/j.envpol.2021.116601
44. Sato, A, and Ishigami, A. Effects of heated tobacco product aerosol extracts on DNA methylation and gene transcription in lung epithelial cells. Toxicol Appl Pharmacol. (2023) 475:116637. doi: 10.1016/j.taap.2023.116637
45. Pan, HL, Wen, ZS, Huang, YC, Cheng, X, Wang, GZ, Zhou, YC, et al. Down-regulation of microRNA-144 in air pollution-related lung cancer. Sci Rep. (2015) 5:14331. doi: 10.1038/srep14331
46. Schembri, F, Sridhar, S, Perdomo, C, Gustafson, AM, Zhang, X, Ergun, A, et al. MicroRNAs as modulators of smoking-induced gene expression changes in human airway epithelium. Proc Natl Acad Sci. (2009) 106:2319–24. doi: 10.1073/pnas.0806383106
47. Sima, M, Rossnerova, A, Simova, Z, and Rossner, P Jr. The impact of air pollution exposure on the MicroRNA machinery and lung cancer development. J Personalized Med. (2021) 11:60. doi: 10.3390/jpm11010060
48. Tellez, CS, Juri, DE, Do, K, Bernauer, AM, Thomas, CL, Damiani, LA, et al. EMT and stem cell–like properties associated with miR-205 and miR-200 epigenetic silencing are early manifestations during carcinogen-induced transformation of human lung epithelial cells. Cancer Res. (2011) 71:3087–97. doi: 10.1158/0008-5472.CAN-10-3035
49. Wang, G, Wang, R, Strulovici-Barel, Y, Salit, J, Staudt, MR, Ahmed, J, et al. Persistence of smoking-induced dysregulation of miRNA expression in the small airway epithelium despite smoking cessation. PLoS One. (2015) 10:e0120824. doi: 10.1371/journal.pone.0120824
50. Xi, S, Xu, H, Shan, J, Tao, Y, Hong, JA, Inchauste, S, et al. Cigarette smoke mediates epigenetic repression of miR-487b during pulmonary carcinogenesis. J Clin Invest. (2013) 123:1241–61. doi: 10.1172/JCI61271
51. Hammons, GJ, Yan, Y, Lopatina, NG, Jin, B, Wise, C, Blann, EB, et al. Increased expression of hepatic DNA methyltransferase in smokers. Cell Biol Toxicol. (1999) 15:389–94. doi: 10.1023/A:1007658000971
52. Sanchez-Guerra, M, Zheng, Y, Osorio-Yanez, C, Zhong, J, Chervona, Y, Wang, S, et al. Effects of particulate matter exposure on blood 5-hydroxymethylation: results from the Beijing truck driver air pollution study. Epigenetics. (2015) 10:633–42. doi: 10.1080/15592294.2015.1050174
53. Guo, C, Lv, S, Liu, Y, and Li, Y. Biomarkers for the adverse effects on respiratory system health associated with atmospheric particulate matter exposure. J Hazard Mater. (2022) 421:126760. doi: 10.1016/j.jhazmat.2021.126760
54. Li, G, Fang, J, Wang, Y, Wang, H, and Sun, CC. MiRNA-based therapeutic strategy in lung cancer. Curr Pharm Des. (2017) 23:6011–8. doi: 10.2174/1381612823666170725141954
55. Mukherjee, S, Dasgupta, S, Mishra, PK, and Chaudhury, K. Air pollution-induced epigenetic changes: disease development and a possible link with hypersensitivity pneumonitis. Environ Sci Pollut Res. (2021) 28:55981–6002. doi: 10.1007/s11356-021-16056-x
56. Wu, Y, Qie, R, Cheng, M, Zeng, Y, Huang, S, Guo, C, et al. Air pollution and DNA methylation in adults: a systematic review and meta-analysis of observational studies. Environ Pollut. (2021) 284:117152. doi: 10.1016/j.envpol.2021.117152
57. Xue, Y, Wang, L, Zhang, Y, Zhao, Y, and Liu, Y. Air pollution: a culprit of lung cancer. J Hazard Mater. (2022) 434:128937. doi: 10.1016/j.jhazmat.2022.128937
58. Li, J, Li, WX, Bai, C, and Song, Y. Particulate matter-induced epigenetic changes and lung cancer. Clin Respir J. (2017) 11:539–46. doi: 10.1111/crj.12389
59. Liu, J, Chen, Y, Cao, H, and Zhang, A. Burden of typical diseases attributed to the sources of PM2. 5-bound toxic metals in Beijing: an integrated approach to source apportionment and QALYs. Environ Int. (2019) 131:105041. doi: 10.1016/j.envint.2019.105041
60. Bhargava, A, Bunkar, N, Aglawe, A, Pandey, KC, Tiwari, R, Chaudhury, K, et al. Epigenetic biomarkers for risk assessment of particulate matter associated lung cancer. Curr Drug Targets. (2018) 19:1127–47. doi: 10.2174/1389450118666170911114342
61. Ladd-Acosta, C. Epigenetic signatures as biomarkers of exposure. Current environmental health reports. (2015) 2:117–25. doi: 10.1007/s40572-015-0051-2
62. Hong, Y, and Kim, WJ. DNA methylation markers in lung cancer. Curr Genomics. (2021) 22:79–87. doi: 10.2174/1389202921999201013164110
63. Nikolaidis, G, Raji, OY, Markopoulou, S, Gosney, JR, Bryan, J, Warburton, C, et al. DNA methylation biomarkers offer improved diagnostic efficiency in lung cancer. Cancer Res. (2012) 72:5692–701. doi: 10.1158/0008-5472.CAN-12-2309
64. Tsou, JA, Hagen, JA, Carpenter, CL, and Laird-Offringa, IA. DNA methylation analysis: a powerful new tool for lung cancer diagnosis. Oncogene. (2002) 21:5450–61. doi: 10.1038/sj.onc.1205605
65. Wei, B, Wu, F, Xing, W, Sun, H, Yan, C, Zhao, C, et al. A panel of DNA methylation biomarkers for detection and improving diagnostic efficiency of lung cancer. Sci Rep. (2021) 11:16782. doi: 10.1038/S41598-021-96242-6
66. Del Vescovo, V, Grasso, M, Barbareschi, M, and Denti, MA. MicroRNAs as lung cancer biomarkers. World J Clinical Oncol. (2014) 5:604–20. doi: 10.5306/wjco.v5.i4.604
67. Inamura, K, and Ishikawa, Y. MicroRNA in lung cancer: novel biomarkers and potential tools for treatment. J Clin Med. (2016) 5:36. doi: 10.3390/jcm5030036
68. Shen, J, Todd, NW, Zhang, H, Yu, L, Lingxiao, X, Mei, Y, et al. Plasma microRNAs as potential biomarkers for non-small-cell lung cancer. Lab Investig. (2011) 91:579–87. doi: 10.1038/labinvest.2010.194
69. Carrier, M, Apparicio, P, Séguin, AM, and Crouse, D. The application of three methods to measure the statistical association between different social groups and the concentration of air pollutants in Montreal: a case of environmental equity. Transp Res Part D: Transp Environ. (2014) 30:38–52. doi: 10.1016/j.trd.2014.05.001
70. Dai, Z, Lakshmanan, RR, Zhu, WG, Smiraglia, DJ, Rush, LJ, Frühwald, MC, et al. Global methylation profiling of lung cancer identifies novel methylated genes. Neoplasia. (2001) 3:314–23. doi: 10.1038/sj.neo.7900162
71. Rauch, TA, Wang, Z, Wu, X, Kernstine, KH, Riggs, AD, and Pfeifer, GP. DNA methylation biomarkers for lung cancer. Tumour Biol. (2012) 33:287–96. doi: 10.1007/s13277-011-0282-2
72. Tsou, JA, Galler, JS, Siegmund, KD, Laird, PW, Turla, S, Cozen, W, et al. Identification of a panel of sensitive and specific DNA methylation markers for lung adenocarcinoma. Mol Cancer. (2007) 6:1–13. doi: 10.1186/1476-4598-6-70
73. Vaissière, T, Hung, RJ, Zaridze, D, Moukeria, A, Cuenin, C, Fasolo, V, et al. Quantitative analysis of DNA methylation profiles in lung cancer identifies aberrant DNA methylation of specific genes and its association with gender and cancer risk factors. Cancer Res. (2009) 69:243–52. doi: 10.1158/0008-5472.CAN-08-2489
74. Tam, KW, Zhang, W, Soh, J, Stastny, V, Chen, M, Sun, H, et al. CDKN2A/p16 inactivation mechanisms and their relationship to smoke exposure and molecular features in non–small-cell lung cancer. J Thorac Oncol. (2013) 8:1378–88. doi: 10.1097/JTO.0b013e3182a46c0c
75. Belinsky, SA, Klinge, DM, Stidley, CA, Issa, JP, Herman, JG, March, TH, et al. Inhibition of DNA methylation and histone deacetylation prevents murine lung cancer. Cancer Res. (2003) 63:7089–93.
76. Vendetti, FP, and Rudin, CM. Epigenetic therapy in non-small-cell lung cancer: targeting DNA methyltransferases and histone deacetylases. Expert Opin Biol Ther. (2013) 13:1273–85. doi: 10.1517/14712598.2013.819337
77. Zöchbauer-Müller, S, Minna, JD, and Gazdar, AF. Aberrant DNA methylation in lung cancer: biological and clinical implications. Oncologist. (2002) 7:451–7. doi: 10.1634/theoncologist.7-5-451
78. Huffman, K, and Martinez, ED. Pre-clinical studies of epigenetic therapies targeting histone modifiers in lung cancer. Front Oncol. (2013) 3:235. doi: 10.3389/fonc.2013.00235
79. Schiffmann, I, Greve, G, Jung, M, and Lübbert, M. Epigenetic therapy approaches in non-small cell lung cancer: update and perspectives. Epigenetics. (2016) 11:858–70. doi: 10.1080/15592294.2016.1237345
80. Barger, JF, and Nana-Sinkam, SP. MicroRNA as tools and therapeutics in lung cancer. Respir Med. (2015) 109:803–12. doi: 10.1016/j.rmed.2015.02.006
81. Wu, M, Wang, G, Tian, W, Deng, Y, and Xu, Y. MiRNA-based therapeutics for lung cancer. Curr Pharm Des. (2017) 23:5989–96. doi: 10.2174/1381612823666170714151715
82. Cheng, H, Zou, Y, Shah, CD, Fan, N, Bhagat, TD, Gucalp, R, et al. First-in-human study of inhaled Azacitidine in patients with advanced non-small cell lung cancer. Lung Cancer. (2021) 154:99–104. doi: 10.1016/j.lungcan.2021.02.015
83. Vendetti, FP, Topper, M, Huang, P, Dobromilskaya, I, Easwaran, H, Wrangle, J, et al. Evaluation of azacitidine and entinostat as sensitization agents to cytotoxic chemotherapy in preclinical models of non-small cell lung cancer. Oncotarget. (2015) 6:56–70. doi: 10.18632/oncotarget.2695
84. Komatsu, N, Kawamata, N, Takeuchi, S, Yin, D, Chien, W, Miller, CW, et al. SAHA, a HDAC inhibitor, has profound anti-growth activity against non-small cell lung cancer cells. Oncol Rep. (2006) 15:187–91. doi: 10.3892/or.15.1.187
85. Platta, CS, Greenblatt, DY, Kunnimalaiyaan, M, and Chen, H. The HDAC inhibitor trichostatin a inhibits growth of small cell lung cancer cells. J Surg Res. (2007) 142:219–26. doi: 10.1016/j.jss.2006.12.555
Keywords: epigenetics, lung cancer, DNA methylation, environment pollution, miRNA
Citation: Zhang A, Luo X, Li Y, Yan L, Lai X, Yang Q, Zhao Z, Huang G, Li Z, Wu Q and Wang J (2024) Epigenetic changes driven by environmental pollutants in lung carcinogenesis: a comprehensive review. Front. Public Health. 12:1420933. doi: 10.3389/fpubh.2024.1420933
Edited by:
Arpit Bhargava, Ram Krishna Dharmarth Foundation University, IndiaReviewed by:
Peifen Zhang, Sun Yat-sen University Cancer Center (SYSUCC), ChinaSina Azadnajafabad, Washington University in St. Louis, United States
Copyright © 2024 Zhang, Luo, Li, Yan, Lai, Yang, Zhao, Huang, Li, Wu and Wang. This is an open-access article distributed under the terms of the Creative Commons Attribution License (CC BY). The use, distribution or reproduction in other forums is permitted, provided the original author(s) and the copyright owner(s) are credited and that the original publication in this journal is cited, in accordance with accepted academic practice. No use, distribution or reproduction is permitted which does not comply with these terms.
*Correspondence: Guanghui Huang, Z2hodWFuZzFAbXVzdC5lZHUubW8=; Zheng Li, bGl6aGVuZ2NwdUAxNjMuY29t; Qibiao Wu, cWJ3dUBtdXN0LmVkdS5tbw==, Jue Wang, d2FuZ2p1ZUBtdXN0LmVkdS5tbw==
†These authors have contributed equally to this work