- 1Clinic of Children’s Diseases, Faculty of Medicine, Institute of Clinical Medicine, Vilnius University, Vilnius, Lithuania
- 2Department of Pathology and Forensic Medicine, Faculty of Medicine, Institute of Biomedical Sciences, Vilnius University, Vilnius, Lithuania
- 3Human Ecology Multidisciplinary Research Group, Department of Public Health, Faculty of Medicine, Institute of Health Sciences, Vilnius University, Vilnius, Lithuania
- 4Clinic of Children’s Diseases, Medical Academy, Lithuanian University of Health Sciences, Kaunas, Lithuania
- 5Faculty of Medicine, Vilnius University, Vilnius, Lithuania
- 6Kantonsspital Münsterlingen, Münsterlingen, Switzerland
- 7Institute of Biosciences, Life Sciences Center, Vilnius University, Vilnius, Lithuania
- 8Laboratory of Ecotoxicology, Nature Research Centre, Vilnius, Lithuania
- 9Department of Rehabilitation, Physical and Sports Medicine, Institute of Health Sciences, Faculty of Medicine, Vilnius University, Vilnius, Lithuania
- 10Department of Mathematical Statistics, Vilnius Gediminas Technical University, Vilnius, Lithuania
- 11Clinic of Asthma, Allergy and Chronic Respiratory Diseases, Vilnius, Lithuania
Background: It has been reported that the disease-initiated and disease-mediated effects of aerosol pollutants can be related to concentration, site of deposition, duration of exposure, as well as the specific chemical composition of pollutants.
Objectives: To investigate the microelemental composition of dust aggregates in primary schools of Vilnius and determine trace elements related to acute upper respiratory infections among 6-to 11-year-old children.
Methods: Microelemental analysis of aerosol pollution was performed using dust samples collected in the classrooms of 11 primary schools in Vilnius from 2016 to 2020. Sites included areas of its natural accumulation behind the radiator heaters and from the surface of high cupboards. The concentrations of heavy metals (Pb, W, Sb, Sn, Zr, Zn, Cu, Ni, Mn, Cr, V, and As) in dust samples were analyzed using a SPECTRO XEPOS spectrometer. The annual incidence rates of respiratory diseases in children of each school were calculated based on data from medical records.
Results: The mean annual incidence of physician-diagnosed acute upper respiratory infections (J00-J06 according to ICD-10A) among younger school-age children was between 25.1 and 71.3% per school. A significant correlation was found between vanadium concentration and the number of episodes of acute upper respiratory infections during each study year from 2016 to 2020. The lowest was r = 0.67 (p = 0.024), and the highest was r = 0.82 (p = 0.002). The concentration of vanadium in the samples of dust aggregates varied from 12.7 to 52.1 parts per million (ppm). No significant correlations between the other trace elements and the incidence of upper respiratory infections were found, which could be caused by a small number of study schools and relatively low concentrations of other heavy metals found in the samples of indoor dust aggregates.
Conclusion: A significant and replicable correlation was found between the concentration of vanadium in the samples of natural dust aggregates collected in primary schools and the incidence of acute upper respiratory infections in children. Monitoring the concentration of heavy metals in the indoor environment can be an important instrument for the prevention and control of respiratory morbidity in children.
1 Introduction
Children are facing an increased number of health issues due to the deterioration of air quality (1, 2). They are particularly vulnerable to air pollution, especially during periods of faster growth and development (3–5). Greater permeability of respiratory epithelium and immature defense mechanisms can also play an important role. The primary school is a relatively new environment for children in terms of environmental pollution (6). Children spend more time at school (up to 4–8 h usually in the same classroom) than anywhere else except their own home. School is a new environment for these children in terms of environmental pollution. Prior research has focused mainly on the mass concentration of particulate matter PM2.5 (particles equal to or less than 2.5 μm) and PM10 (particles equal to or less than 10 μm) (7–12). However, we recently found an important role of the accumulation mode (0.3–1.0 μm) of aerosol particles in asthma morbidity among younger (6–11 years) school-age children (13). It was earlier reported that the effects of inhaled aerosols depend on the size of the particles, concentration, duration of exposure, place of precipitation in the respiratory tract as well as their specific chemical composition (14). Heavy metals can impair important biochemical processes posing a threat to human health, plant growth, and animal life (15, 16). They can also cause toxicity in certain organs of the human body, such as nephrotoxicity, neurotoxicity, hepatotoxicity, skin toxicity and cardiovascular toxicity. This can have adverse effects of the physical growth of children and a deleterious impact on bronchial epithelial cells, among other harmful results (17). Aerosol particles can coagulate and deposit in the form of dust; thus, it is most convenient to study the microelemental composition of the aerosols by collecting dust samples where they naturally accumulate. Such places in the classrooms not usually subjected to wet cleaning are the surfaces of high cupboards and places behind the radiator heaters where aerosol particles are deposited due to thermophoretic forces (18).
The aims of our study were to analyze the microelemental composition of dust and their concentration in the samples of natural dust aggregates taken from Vilnius primary schools and to determine which trace elements can be related to the incidence of acute upper respiratory infections in younger school-age children.
2 Materials and methods
2.1 Description of studied schools
This study was carried out in Vilnius, Lithuania (54o41′17′′N, 25o15′8′′E). Primary school children (children in the age range of 6–11 years, grades 1–4) were enrolled. Invitations were sent to 107 Vilnius schools to participate in the study; 25 responded and agreed to participate. Every other school was randomly selected for inclusion in the study. One school did not have primary classes and was rejected. Finally, 11 schools were selected to participate in the study (Figure 1). A unique number was assigned for each school to protect the privacy of study participants. Schools numbered 1, 5, 7, and 10 were located in the downtown area, schools numbered 2, 3, 4, 6, and 8 were located in the peripheral part of the city, and those numbered 9 and 11 were located in the suburbs.
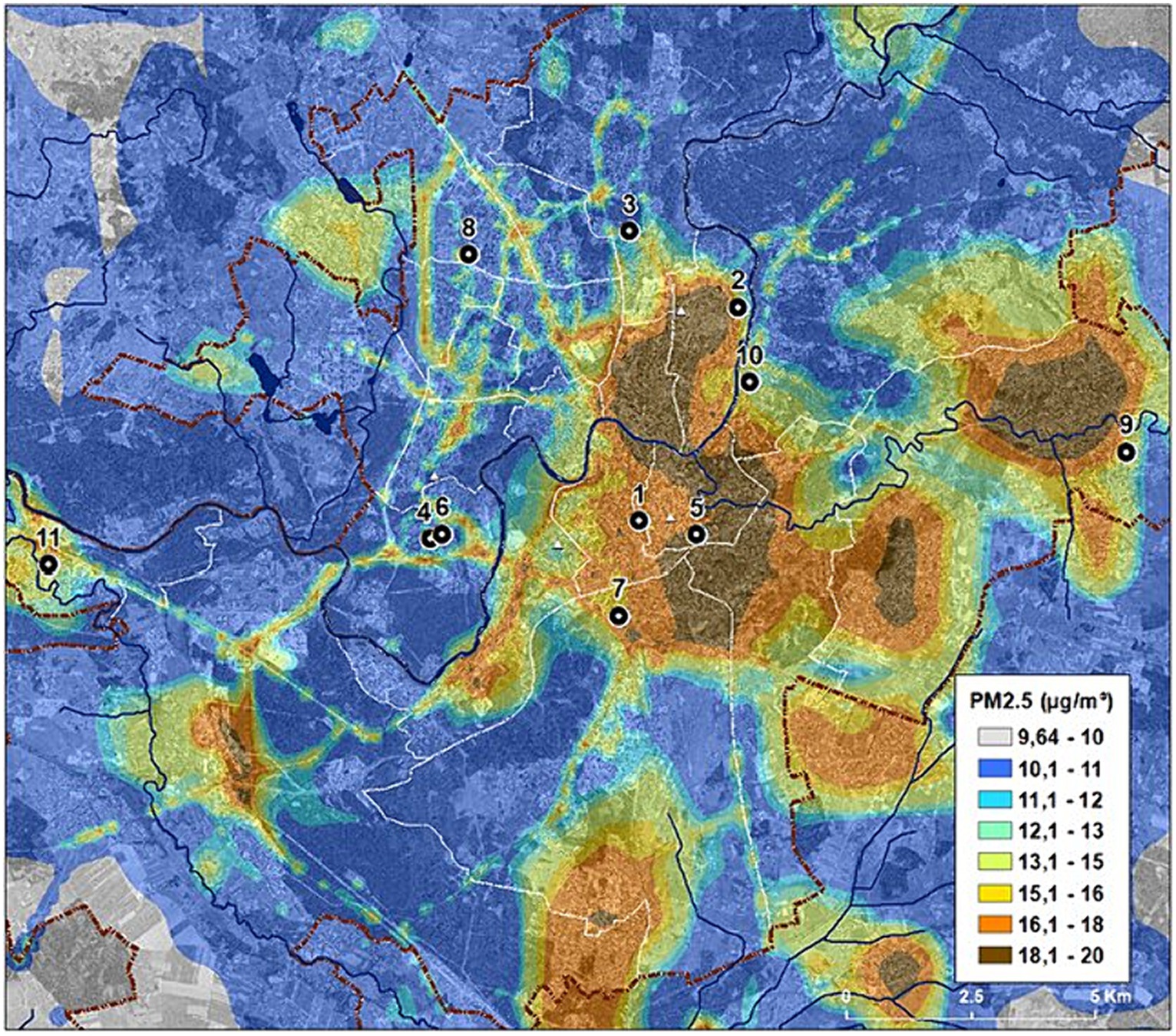
Figure 1. Location of the schools engaged in the research. The scheme of average annual ambient PM2.5 concentrations in Vilnius on 2018. Adapted from the public domain “Air Pollution Dispersion Maps” of the Environmental Protection Agency of Lithuania (19), published with permission, available at https://aaa.lrv.lt/lt/veiklos-sritys/oras/oro-uzterstumo-sklaidos-zemelapiai-duomenys-fonines-koncentracijos-paov-skaiciavimams/.
2.2 Collecting dust samples in schools
Filtering aerosol samples for trace element analysis in classrooms is known to be difficult, because collecting a significant sample mass can be a lengthy process. Therefore, we decided to replace the aerosol samples with dust samples, which usually accumulated in places inaccessible during cleaning in the classrooms. The adequacy of these samples is fairly obvious and saves considerable time. In this case, we obtained concentrations of microelements averaged over time via dust accumulation. Dust sampling in classrooms was taken from sites where dust accumulated: the surfaces of high cupboards (where aerosol particles are deposited due to coagulation) and behind central radiator heaters where aerosol particles are deposited due to thermophoretic forces. These natural dust aggregates have a purely aerosol origin and were collected mainly against the corners of the rear walls of radiator heaters. Other sources of dust such as particles brought into the classroom from the street with shoes were not included. The dust was collected using a vacuum cleaner on an analytical filter FPP type (Petryanov’s filters) in plastic boxes with a volume of 60 mL while tightly filled with dust.
2.3 Measurements of heavy metal concentrations in samples
Dust samples were crushed in a porcelain mortar and dried at 40°C until there was a steady mass in the drying chamber. Approximately 3 grams (g) of the dried sample was mixed and homogenized with 1 g of wax. The samples were then pressed into steel rings with a pressure of 15 kilonewtons (kN) to produce the final pellets. This preparation concentrated sample components and introduced some degree of homogeneity for improved analytical accuracy and precision. Samples were analyzed using a SPECTRO XEPOS (XEPOS HE) simultaneous ED-XRF spectrometer from SPECTRO Analytical Instruments. This model was equipped with an air-cooled, 50-W end-window X-ray tube—a bright laboratory-quality source optimized for maximum energy generation. The measurement time of one sample was 600 s, and the accuracy of elemental composition was less than 10%. The limit of detection (LOD) of trace elemental composition of samples is presented in Table 1. To further heighten stability, the excitation chamber, including the X-ray tube, optics, and detector, was maintained under constant vacuum conditions even in periods between measurements. The concentrations of Pb, W, Sb, Sn, Zr, Rb, Cu, Ni, Mn, Cr, V, As, Ba, Br, and Zn were thus measured in dust samples.
2.4 Data on morbidity in schools under study
The annual incidence of doctor-diagnosed acute infections of the upper respiratory tract (J00–J06) among 6- to 11-year-old pupils in each school was calculated based on clinical records of health care providers collected by the National Institute of Hygiene: acute nasopharyngitis (common cold) (J00), acute sinusitis (J01), acute pharyngitis (J02), acute tonsilitis (J03), acute laryngitis and tracheitis (J04), acute obstructive laryngitis and epiglottitis (J05), and acute upper respiratory infections of multiple and unspecified sites (J06). According to national legislation, personal codes of children and codes of diagnoses based on the Australian Modification of the International Statistical Classification of Diseases and Related Health Problems (ICD-10-AM) were received by the National Institute of Hygiene. The incidence of acute upper respiratory infections (J00–J06) per school represents an annual number of doctor-diagnosed cases among school children of 6–11 years of age divided by the total number of children of this age group in the school and multiplied by 100.
2.5 Statistical analysis
This study employed the linear regression model to determine the dependence of respiratory diseases on air pollution. The dependence of the annual incidence of respiratory infections on the microelemental concentration of dust samples can be expressed via a linear function where the dependent value is incidence (%), and the independent value is microelemental concentration (ppm).
We used Pearson’s correlation to evaluate the correlation between the elemental composition of dust samples and the incidence of acute upper respiratory infections in children. A p-value of < 0.05 was considered significant. IBM SPSS Statistics 23 was used for statistical analysis.
3 Results
3.1 The incidence of acute respiratory infections in children and elemental composition of dust samples
The incidence of upper respiratory infections per school ranged from 17.0% to 75.7%. The highest incidence was observed in Schools 9, 11, 6, and 10: 75.1%, 74.2%, 57.4%, and 52.2%, respectively. If we consider the incidence for the entire study period of 2016–2020, then the highest incidence rate was observed in 2019. This may be due to cross-diagnosis between influenza and upper respiratory tract infections (influenza cases were the highest in 2019) (20). Data on the elemental composition (Pb, W, Sb, Sn, Zr, Rb, Cu, Ni, Mn, Cr, V, As, Ba, Br, Zn) of dust samples in 11 schools in Vilnius are presented in Table 2.
Regression analysis was performed for all elements in Table 2. It turned out that a significant correlation was found only between vanadium concentrations and acute upper respiratory infections (J00–J06) in 2016, 2017, 2018, and 2020 years: r = 0.67, p = 0.024; r = 0.75, and p = 0.008; r = 0.82. p = 0.002; r = 0.73, and p = 0.01, respectively. In 2019 year, the correlation was not significant: r = 0.57, p = 0.067. The correlation between the concentration of vanadium and the average value of acute upper respiratory infections for the 2016–2020 years was also evaluated (r = 0.73, p = 0.01) because dust accumulation is an integral value for a longer time. The results are summarized in Figure 2. Linear regression equations were obtained and indicated reliable results. The linear regression data for the concentrations of vanadium (ppm) in dust samples and the incidence of acute upper respiratory tract infections in 11 schools (annual data) are presented in Table 3.
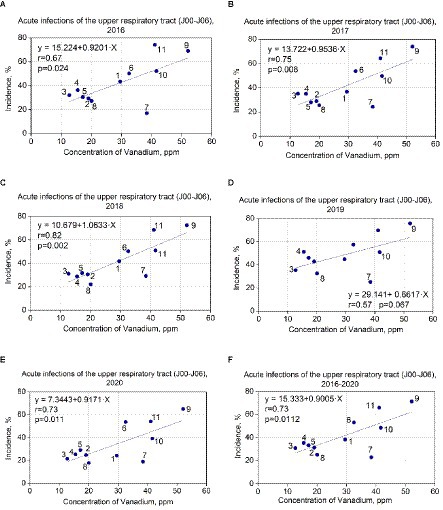
Figure 2. (A–F) Correlation between vanadium concentrations in dust samples and incidence of acute upper respiratory infections among pupils in studied schools.
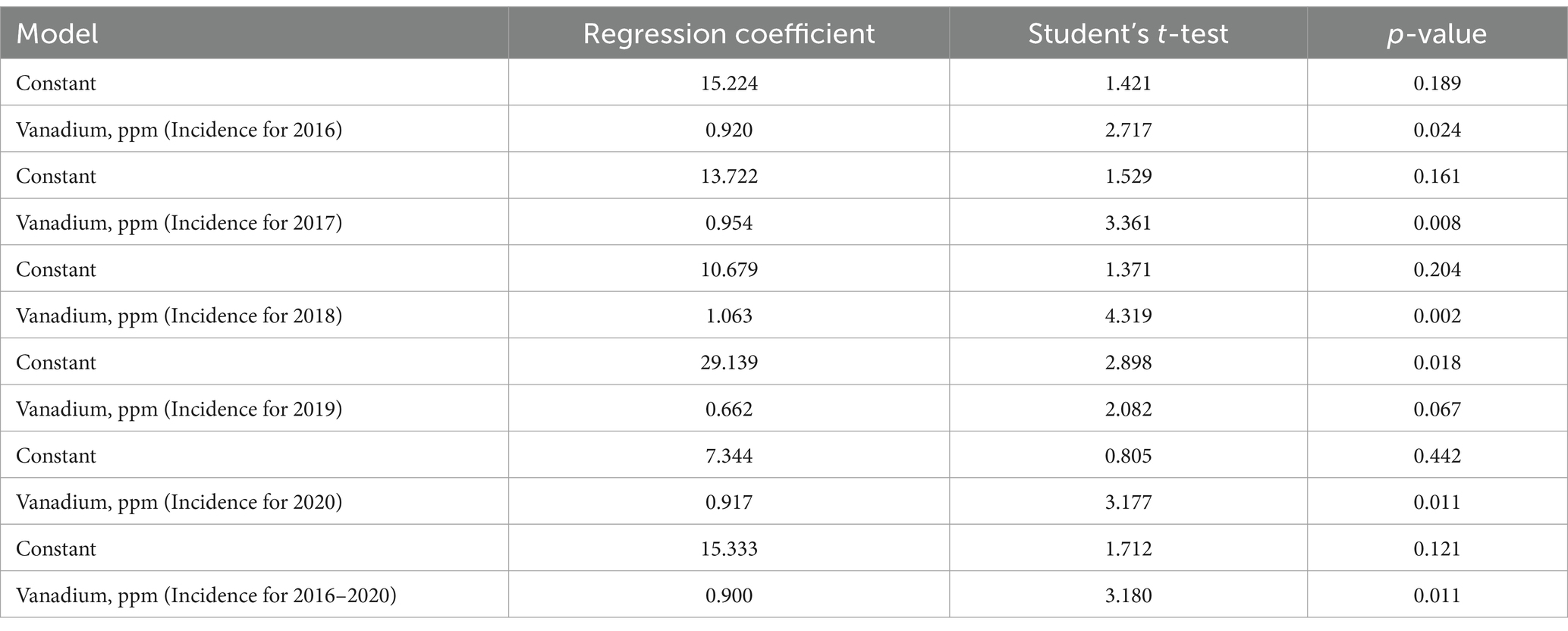
Table 3. Results of linear regression data for vanadium concentrations in dust samples and incidence of acute upper respiratory infections.
For vanadium data in 2016 (in case of incidence of acute upper respiratory infections), the F-statistic is 7.381, the p-value is 0.024, and the coefficient of determination (R2) is 0.45 (for the 2016 year). The F-statistic is 11.293, the p-value is 0.008, and R2 is 0.56 for 2017. The F-statistic is 18.661, the p-value is 0.002, and R2 is 0.68 for 2018. The F statistic is 4.332, the p-value is 0.067, and R2 is 0.32 for 2019. The F-statistic is 10.096, the p-value is 0.011, and R2 is 0.53 for 2020. The F-statistic is 10.114, the p-value is 0.011, and R2 is 0.52 for the average of 2016–2020.
Thus, p-values are less than 0.05, which indicates coefficient reliability. The coefficients of determination (R2 = 0.45, R2 = 0.56, R2 = 0.68, R2 = 0.53, R2 = 0.52) demonstrate that the vanadium data (in the case of incidence of acute upper respiratory infections) correspond to the linear regression. We checked that our linear regression assumptions concerning the residual are satisfied. The residuals are normal (Kolmogorov–Smirnov test), and their mean does not differ from 0. The summary of the results is presented in Figures 2A–F.
According to the linear regression equation, an increase in the concentration of vanadium by 1 ppm leads to an increase in the incidence of acute upper respiratory infections by 0.92–1.06% in 2016, 2017, 2018, and 2020 (Figures 2A–C,E). The exception is 2019 (0.66%; Figure 2D) possibly due to the highest incidence of influenza in that year. It can be caused by cross-diagnosis between influenza and upper respiratory tract infections—especially in cases where a viral search was not conducted.
4 Discussion
This is the first study devoted to the relationship between the chemical composition of natural dust aggregates and respiratory morbidity in children. Trace element analysis can indicate the time-dependent pollution history of public buildings or residential areas. It allows us to evaluate the concentration of heavy metals in pre-aggregate aerosol forms of pollutants. A significant correlation was found between the concentration of vanadium in dust samples and physician-diagnosed upper respiratory infections in younger school-age children. The concentrations of vanadium in Vilnius schools were 12.69–52.09 ppm.
Vanadium is important for normal cell function and development (21, 22). It exists in all tissues involved in glucose homeostasis, lipid metabolism, and antioxidant functions. For the general population, food is the major source of vanadium exposure (23–25). Despite the positive effect of vanadium on various processes in humans, a safe dose is still unclear. High concentrations can lead to various pathological alterations including modified intracellular enzyme systems, which have an impact on digestion, respiration, etc. (26–29).
Two-thirds of vanadium enters in aerosol form due to anthropogenic sources, such as the burning of fossil fuel, vehicle emissions and leaks when used it in industrial production (30). Therefore, it is not surprising that the maximum concentrations of vanadium in dust samples are seen in Schools No. 9, 10, and 11. School No. 9 is located in a suburb of Vilnius where there are 20 industrial enterprises and many houses use stove heating. School No. 10 is located next to a very busy transport artery. School No. 11 is located near a big paper factory. The children in School No. 6 are in the zone of influence of the thermal power plant when the wind is from the South.
Vanadium is especially dangerous in the form of aerosols. Only <1–2% of vanadium is absorbed by the body during oral ingestion (23), but up to 90% of vanadium is absorbed when inhaled (31, 32). At the same time, the greatest harm comes from aerosols carrying vanadium with sizes below 2.5 microns, because these particles can reach the alveolar sacs. Prolonged inhalation of vanadium aerosols can cause shortness of breath, wheezing, cough, epistaxis, headache, dizziness, fatigue, as well as cancer (29, 33). One hour per day exposure of rabbits to vanadium of 20–40 mg/m3 during several months caused chronic rhinitis, tracheitis, emphysema, atelectasis, and bronchopneumonia (34). Continuous exposure to a concentration of 10–30 mg/m3 was distinctly toxic to rabbits, causing bronchitis and pneumonia, loss of weight, and bloody diarrhea. With rats, 10 mg/m3 was toxic, and a small exposure of 3–5 mg/m3 caused the same symptoms with 2 months delay. A lethal exposure was considered to be 70 mg/m3 if prolonged for more than 20 h. In another study, groups of 50 male and 50 female F344 rats were exposed to 0, 0.5, 1, or 2 mg vanadium pentoxide/m3 (0, 0.28, 0.56, and 1.1 mg vanadium/m3) 6 h/day, 5 days/week for 104 weeks. Alveolar histiocytic infiltrates were observed in males and females rats exposed to ≥0.28 mg vanadium/m3 (35). An inhalation model demonstrated that vanadium generates histological and physiological changes in different cells and organs including the lung, lymphoid organs, and the immune system. Oxidative and nitrosative stress play a relevant role in the vanadium toxic mechanisms (30, 36).
The U.S. Environmental Protection Agency (EPA) published a review (37) that summarized 67 human studies devoted to the health effects of vanadium on infants, children, pregnant women, the general population (adults) and occupational workers. The predominant health outcomes investigated included respiratory, cardiovascular, nervous system and immune system effects. Twenty studies examined the effects of vanadium on the respiratory system; however, only two of these (38, 39) were devoted to children’s respiratory health. Patel et al. reported that increases in ambient vanadium concentrations (0.0033 mg/m3) were associated significantly (p = 0.0003) with an increased probability (31%) of wheezing in children through age two (38). Gehring et al. did not find an association between vanadium in PM2.5 or PM10 and children’s respiratory health (39). However, PM constituents, in particular iron, copper and zinc, may increase the risk of asthma and allergies in schoolchildren. Five studies (40–44) were devoted to general population-adult respiratory health and 13 studies were on occupational exposure and respiratory health. An association has been reported between hospitalization for respiratory illness and observed vanadium concentrations in PM2.5 outdoors (41). Urinary vanadium, nickel, and antimony have been reported to be associated with increased exhaled nitric oxide (42). Zenz and Berg studied responses in nine human volunteers exposed to vanadium pentoxide from 1 to 0.2 mg/m3 (particle size, 98% < 5 μm) for 8 h in a controlled environmental chamber (40). Coughing began and then remained in all subjects for 7 to 10 days. However, Lagorio et al. (43) and Wu et al. (44) found no adverse effects of vanadium on the adult respiratory system. There is even more limited and controversial data on the disease initiation and/or modification effects of vanadium in children (37, 45).
In our study, a significant and replicable correlation was found between the concentration of vanadium in the samples of natural dust aggregates and the incidence of doctor-diagnosed acute upper respiratory infections in younger school-age children in the years 2016–2020. The exception was 2019, when a moderate, but non-significant correlation was found. It can be caused by a very high incidence of influenza cases and misdiagnosis of other than influenza respiratory infections especially when the diagnosis was done without virus verification. The highest incidence of influenza among children in the period of 2016–2020 was observed in 2019.
There are a limited number of studies on the microelemental composition of aerosol particles in residential premises (46–49) and schools (50–52). In these cases (46, 48, 49), indoor dust does not characterize the composition of the aerosol particles because the dust can be of various origins. As a rule, the dust was collected by a vacuum cleaner from the floor surfaces. One study was conducted in kindergartens (53), but it did not target the composition of the aerosols, and dust was collected from floors and window sills.
Studies of the microelemental composition of aerosol particles is a very laborious process. This also applies to the time of sampling when using special filters, i.e., from 8 h to several days or even months. To date, this method has been used to determine the composition of aerosols (47, 50–52). This challenging analysis likely explains the paucity of data on the elemental composition of aerosol pollution. Indeed, data about the microelemental composition of aerosol particles and their relation to diseases in children are rare and insufficient (54–57).
High PM2.5 values in residential areas have been associated with lower lung function among urban children. An association was found between short-term vanadium exposure and DNA methylation in the asthma gene loci (58). A study of children (10–12 years of age) living in the vicinity of a facility involved in hydrometallurgical processing of vanadium-rich slag found significant decreases in lymphocyte stimulation with phytohemagglutinin and an increase in the incidence of viral and bacterial respiratory infections (59).
Data from occupational studies suggest that the lowest-observed-adverse-effect level of vanadium is assumed to be 20 μg/m3, based on chronic upper respiratory tract symptoms (60). Particularly high PMC values (up to 230 μg/m3) were earlier reported in the air of classrooms School No. 9 (61), while vanadium concentration in dust samples from the same school was as high as 52 ppm. This allows us to calculate the hypothetic amount of inhaled vanadium before it turns to dust aggregates. Thus, in the case of inhalation of 1 m3 of air, 230 μg of particulate matter including 0.012 μg of vanadium can enter the respiratory tract of a child. Taking into account one school year (39 weeks, 5 days per week, and 6 h per day) and an inhalation rate of 0.9 m3/h (62), that is 1,053 m3/year, and we can estimate that the annual inhaled amount of vanadium can be 12.64 μg. However, we do not know the proportion of inhaled vanadium that has settled in the lungs.
Studies of the effects of heavy metals on the development of respiratory morbidity in children (with different levels of microelemental concentrations in polluted air) are rarer and more controversial (37, 45, 59). The EPA emphasizes the need for more in-depth research on vanadium to identify the mechanism of this microelement’s effects on human health and the possibility of determining safe concentrations. It seeks to analyze the impact of short-term and long-term cumulative exposures (37).
Nevertheless, we hypothesize that vanadium-induced damage to the respiratory system may be complex. These may include the destruction of the barrier function of the bronchial wall due to chronic inflammation, reduced clearance of bacteria in the lungs, and reduced capacity of alveolar macrophages. It has been earlier reported, that exposure of human fibroblasts to vanadate effectively causes DNA strand breaks, and co-exposure of cells to other genotoxic agents may result in persistent DNA damage (24). We can also extrapolate our suggestions from brake abrasion dust (BAD) research which shows the dose-dependent relationship between BAD and the impaired ability of immune cells to ingest respiratory pathogens and enhanced inflammatory signaling in a transient but metal-dependent manner (63). Responses to particles were characterized by decreased mitochondrial depolarization, increased secretion of IL-8, IL-10 and TNF-α as well as decreased phagocytosis of S. aureus. Mitochondrial dysfunction leading to early airway remodeling was recently identified in preschool children with infection-induced recurrent wheezing syndrome (64).
The World Health Organization (WHO) has no data on the annual exposure level of children and adults to vanadium (60). However, WHO considered annual average values for inhalation of vanadium for urban areas to be in the range of 0.05–0.18 μg/m3. In the most densely populated areas, maximum vanadium concentrations reach up to 2 μg/m3. In Lithuania, the maximum permissible indoor concentration of vanadium for both adults and children is 1 μg/m3 (27). In the present study, we found a linear relationship between respiratory morbidity in children and the concentration of vanadium in dust aggregates. This suggests that any concentration of vanadium inhaled by children can increase respiratory morbidity caused by viruses and bacteria. It supposes the necessity to re-evaluate the current understanding of the safe limits of inhaled vanadium in children.
There are some limitations of our study. Eleven schools participating in our survey might be insufficient to analyze all health-damage effects of pollutants. In contrast to vanadium, the concentrations of other heavy metals in dust samples did not reach the threshold values, and a correlation with the incidence of upper respiratory infections was not found. Thus, the disease initiation and/or modification effects of these heavy metals need a larger research sample. Another limitation is related to an unknown period of dust accumulation in one school or another. The incidence of respiratory infections was calculated based on medical records only and some home-cared mild respiratory infections can be missed or improperly recorded.
Our research is the first attempt to evaluate the impact of the chemical composition of indoor air pollutants on the respiratory morbidity of younger school-age children using samples of natural dust aggregates taken from primary schools. It opens the possibility for further prospective studies with the evaluation of known periods of dust accumulation and dose–response effects of different pollutants.
5 Conclusion
The incidence of acute upper respiratory infections among 6- to 11-year-old children is related to the concentration of vanadium in the natural dust aggregates collected from their primary schools. Unlike the difficulties of aerosol sampling using filters, samples of natural dust aggregates collected in classrooms are very convenient and reliable materials for studying the time-dependent trace element composition of indoor pollutants. Regular assessment of indoor air quality including the post-aerosol dust aggregates can be an important tool for the prevention and control of respiratory morbidity in children.
Data availability statement
The original contributions presented in the study are included in the article/supplementary material, further inquiries can be directed to the corresponding author.
Author contributions
NP: Writing – review & editing, Writing – original draft, Visualization, Methodology, Formal analysis, Conceptualization. VT: Software, Writing – review & editing. LaV: Formal analysis, Writing – review & editing. IJ: Investigation, Writing – review & editing. VS: Investigation, Writing – review & editing. IV: Formal analysis, Writing – review & editing. VV: Validation, Writing – review & editing. RV: Investigation, Writing – review & editing. AlV: Investigation, Writing – review & editing. TA: Formal analysis, Writing – review & editing. LuV: Writing – review & editing. MB: Formal analysis, Writing – review & editing. JN: Software, Writing – review & editing. NT: Writing – review & editing, Writing – original draft, Methodology, Conceptualization. ArV: Supervision, Writing – review & editing, Writing – original draft, Data curation, Conceptualization.
Funding
The author(s) declare that financial support was received for the research, authorship, and/or publication of this article. The study was funded by project no. S-MIP-23-128 of the Lithuanian State Research Council.
Acknowledgments
The authors are very thankful to the staff and children of the studied schools for their participation in our survey as well as the State Institute of Hygiene for the data on morbidity collection and Lithuanian State Geological Service under the Lithuanian Ministry of Environment for the analysis of the microelement content of the dust samples.
Conflict of interest
The authors declare that the research was conducted in the absence of any commercial or financial relationships that could be construed as a potential conflict of interest.
Publisher’s note
All claims expressed in this article are solely those of the authors and do not necessarily represent those of their affiliated organizations, or those of the publisher, the editors and the reviewers. Any product that may be evaluated in this article, or claim that may be made by its manufacturer, is not guaranteed or endorsed by the publisher.
References
1. Valiulis, A, Bousquet, J, Veryga, A, Suprun, U, Sergeenko, D, Cebotari, S, et al. Vilnius declaration on chronic respiratory diseases: multisectoral care pathways embedding guided self-management, mHealth and air pollution in chronic respiratory diseases. Clin Transl Allergy. (2019) 9:7. doi: 10.1186/s13601-019-0242-2
2. Bousquet, J, Schunemann, HJ, Togias, A, Erhola, M, Hellings, PW, Zuberbier, T, et al. Next-generation ARIA care pathways for rhinitis and asthma: a model for multimorbid chronic diseases. Clin Transl Allergy. (2019) 9:44. doi: 10.1186/s13601-019-0279-2
3. Manisalidis, I, Stavropoulou, E, Stavropoulos, A, and Bezirtzoglou, E. Environmental and health impacts of air pollution: a review. Front Public Health. (2020) 8:8–14. doi: 10.3389/fpubh.2020.00014
4. Stein, MM, Hrusch, CL, Gozdz, J, Igartua, C, Pivniouk, V, Murray, SE, et al. Innate immunity and asthma risk in Amish and Hutterite farm children. N Engl J Med. (2016) 375:411–21. doi: 10.1056/NEJMoa1508749
5. Liu, W, Huang, C, Cai, J, Fu, Q, Zou, Z, Sun, C, et al. Prenatal and postnatal exposures to ambient air pollutants associated with allergies and airway diseases in childhood: a retrospective observational study. Environ Int. (2020) 142:105853. doi: 10.1016/j.envint.2020.105853
6. Dai, X, Shyamali, DSC, and Lodge, CJ. The relationship of early-life household air pollution with childhood asthma and lung function. Eur Respir Rev. (2022) 31:220020. doi: 10.1183/16000617.0020-2022
7. Liu, MM, Wang, D, Zhao, Y, Liu, YQ, Huang, MM, Liu, Y, et al. Effects of outdoor and indoor air pollution on respiratory health of Chinese children from 50 kindergartens. J Epidemiol. (2013) 23:280–7. doi: 10.2188/jea.JE20120175
8. Krugly, E, Martuzevicius, D, Sidaraviciute, R, Ciuzas, D, Prasauskas, T, Kauneliene, V, et al. Characterization of particulate and vapor phase polycyclic aromatic hydrocarbons in indoor and outdoor air of primary schools. Atmos Environ. (2014) 82:298–306. doi: 10.1016/j.atmosenv.2013.10.042
9. Deng, Q, Lu, C, Yu, Y, Li, Y, Sundell, J, and Norbäck, D. Early life exposure to traffic-related air pollution and allergic rhinitis in preschool children. Respir Med. (2016) 121:67–73. doi: 10.1016/j.rmed.2016.10.016
10. Gayer, A, Mucha, D, Adamkiewicz, L, and Badyta, A. Children exposure to PM2.5 in kindergarten classrooms equipped with air purifiers – a pilot study. Fire Env Safety Eng MATEC Web Conf. (2018) 247:00016. doi: 10.1051/matecconf/201824700016
11. Moslem, A, Rad, A, de Prado, BP, Alahabadi, A, Ebrahimi Aval, H, Miri, M, et al. Association of exposure to air pollution and telomere length in preschool children. Sci Total Environ. (2020) 722:137933. doi: 10.1016/j.scitotenv.2020.137933
12. Zakaria, IB, and Mahyuddin, N. An overview of indoor air pollution in the Malaysian kindergarten environment. IOP Conf Ser Earth Environ Sci. (2022) 1013:12005. doi: 10.1088/1755-1315/1013/1/012005
13. Juskiene, I, Prokopciuk, N, Franck, U, Valiulis, A, Valskys, V, Mesceriakova, V, et al. Indoor air pollution effects on pediatric asthma are submicron aerosol particle–dependent. Eur J Pediatr. (2022) 181:2469–80. doi: 10.1007/s00431-022-04443-6
14. Salma, I, Balásházy, W-HR, Hofmann, W, and Záray, G. Effect of particle mass size distribution on the deposition of aerosols in the human respiratory system. J Aerosol Sci. (2002) 33:119–32. doi: 10.1016/S0021-8502(01)00154-9
15. Ward, IN, Brooks, RR, Roberts, E, and Boswell, C. Heavy metal pollution from automotive emission and its effect on roadside soils and pastures in New Zealand. Environ Sci Technol. (1977) 11:917–20. doi: 10.1021/es60132a007
16. Michalke, B. Element speciation definitions, analytical methodology, and some examples. fEcotoxicol Environ Saf. (2003) 56:122–39. doi: 10.1016/s0147-6513(03)00056-3
17. Mitra, S, Chakraborty, AJ, Tareq, AM, Emran, TB, Nainu, F, Khusro, A, et al. Impact of heavy metals on the environment and human health: novel therapeutic insights to counter the toxicity. J King Saud Univ-Sci. (2022) 34:101865. doi: 10.1016/j.jksus.2022.101865
18. Yu, S, Wang, J, Xia, GD, and Zon, LX. Thermophoretic force on nonspherical particles in the free-molecule regime. Phys Rev E. (2018) 97:053106. doi: 10.1103/PhysRevE.97.053106
19. Environmental Protection Agency of Lithuania. Air Pollution Dispersion Maps, Data. Available at: https://aaa.lrv.lt/lt/veiklos-sritys/oras/oro-uzterstumo-sklaidos-zemelapiai-duomenys-fonines-koncentracijos-paov-skaiciavimams/ (2018). (Accessed 4 September 2023).
20. National Institute of Hygiene. Health Statistics. Available at: https://www.hi.lt/sveikatos-statistika.html (2023). (Accessed 4 September 2021).
21. Verma, S, Cam, MC, and McNeil, JH. Nutritional factors that can favorably influence the glucose/insulin system: vanadium. J Am Coll Nutr. (1998) 17:11–8. doi: 10.1080/07315724.1998
22. Sabbioni, E, and Marafante, E. Metabolic patterns of vanadium in the rat. Bioinorg Chem. (1978) 9:389–407. doi: 10.1016/S0006-3061(00)80148-9
24. Ivancsits, S, Pilger, A, Diem, E, Schaffer, A, and Rüdiger, HW. Vanadate induces DNA strand breaks in cultured human fibroblasts at doses relevant to occupational exposure. Mutat Res. (2002) 26:519. doi: 10.1016/s1383-5718(02)00138-9
25. Mukherjee, B, Patra, B, Mahapatra, S, Banerjee, P, Tiwari, A, and Chatterjee, M. Vanadium-an element of atypical biological significance. Toxicol Lett. (2004) 150:135–43. doi: 10.1016/j.toxlet.2004.01.009
26. Tripathi, D, Mani, V, and Pal, RP. Vanadium in the biosphere and its role in biological processes. Biol Trace Elem Res. (2018) 186:52–67. doi: 10.1007/s12011-018-1289-y
27. Lithuanian Hygiene Standards. The Maximum Permissible Concentration of Chemical Substances (Pollutants) in the Air of the Living Environment. Available at: https://www.esavadai.lt/dokumentai/4769-hn-352007-didziausia-leidziama-cheminiu-medziagu-tersalu-koncentracija-gyvenamosios-aplinkos-ore-pakeitimai-nuo-2016-05-01/ (2016). (Accessed 3 November 2022).
28. The National Institute for Occupational Safety and Health (NIOSH) Centers of Disease Control and Prevention. Pocket Guide to Chemical Hazards. Vanadium Dust. Available at: www.cdc.gov/niosh/npg/npgd0653.html (2019). (Accessed 15 May 2022).
29. Occupational Safety and Health Administration (OSHA). Chemical Sampling Information Vanadium Fume (as V2O5). (2011). Available at: https://www.osha.gov/chemicaldata/chemResult.html?RecNo=217 (Accessed 30 May 2022).
30. Rojas-Lemus, M, López-Valdez, N, Bizarro-Nevares, P, González-Villalva, A, Ustarroz-Cano, M, Zepeda-Rodríguez, A, et al. Toxic effects of inhaled vanadium attached to particulate matter: a literature review. Int J Environ Res Public Health. (2021) 18:8457. doi: 10.3390/ijerph18168457
31. Léonard, A, and Gerber, GB. Mutagenicity, carcinogenicity, and teratogenicity of thallium compounds. Mutat Res. (1997) 387:47–53. doi: 10.1016/s1383-5742(97)00022-7
32. World Health Organization (WHO). International program on chemical safety. Concise international chemical assessment document. Vanadium pentoxide and other inorganic vanadium compounds (2001). Available at: https://apps.who.int/iris/handle/10665/42365 (Accessed 15 April 2021).
33. National Research Council, Committee on Biologic Effects of Atmospheric Pollutants. Vanadium. Washington, DC: The National Academies Press (1974).
34. Sjoberg, SG. Vanadium pentoxide dust. A clinical and experimental investigation on its effect after inhalation. Acta Med Scand Suppl. (1950) 238:1–188.
35. National Toxicology Program. NTP toxicology and carcinogensis studies of vanadium pentoxide (CAS no. 1314-62-1) in F344/N rats and B6C3F1 mice (inhalation). Natl Toxicol Program Tech Rep Ser. (2002) 507:1–343.
36. Zhao, CN, Xu, Z, Wu, GC, Mao, YM, Liu, LN, Wu, Q, et al. The emerging role of air pollution in autoimmune diseases. Autoimmun Rev. (2019) 18:607–14. doi: 10.1016/j.autrev.2018.12.010
37. U.S. Environmental Protection Agency (EPA). IRIS Assessment Plan for Inhalation Exposure to Vanadium and Compounds (Scoping and Problem Formulation Materials). (2021). Washington, DC, EPA/635/R-21/077. Available at: https://cfpub.epa.gov/ncea/iris_drafts/recordisplay.cfm?deid=351650 (Accessed 15 September 2023).
38. Patel, MM, Hoepner, L, Garfinkel, R, Chillrud, S, Reyes, A, Quinn, JW, et al. Ambient metals, elemental carbon, and wheeze and cough in new York City children through 24 months of age. Am J Respir Crit Care Med. (2009) 1:1107–13. doi: 10.1164/rccm.200901-0122OC
39. Gehring, U, Beelen, R, Eeftens, M, Hoek, G, de Hoogh, K, de Jongste, JC, et al. Particulate matter composition and respiratory health: the PIAMA birth cohort study. Epidemiology. (2015) 26:300–9. doi: 10.1097/EDE.0000000000000264
40. Zenz, C, and Berg, BA. Human responses to controlled vanadium pentoxide exposure. Arch Environ Health. (1967) 14:709–12. doi: 10.1080/00039896.1967.10664824
41. Bell, ML, Ebisu, K, Leaderer, BP, Gent, JF, Lee, HJ, Koutrakis, P, et al. Associations of PM2.5 constituents and sources with hospital admissions: analysis of four counties in Connecticut and Massachusetts (USA) for persons ≥ 65 years of age. Environ Health Perspect. (2014) 122:138–44. doi: 10.1289/ehp.1306656
42. Li, W, Xiao, L, Zhou, Y, Wang, D, Ma, J, Xie, L, et al. Plasma CC16 mediates the associations between urinary metals and fractional exhaled nitric oxide: a cross-sectional study. Environ Pollut. (2020) 258:113713. doi: 10.1016/j.envpol.2019.113713
43. Lagorio, S, Forastiere, F, Pistelli, R, Iavarone, I, Michelozzi, P, Fano, V, et al. Air pollution and lung function among susceptible adult subjects: a panel study. Environ Health. (2006) 5:11. doi: 10.1186/1476-069X-5-11
44. Wu, S, Deng, F, Hao, Y, Shima, M, Wang, X, Zheng, C, et al. Chemical constituents of fine particulate air pollution and pulmonary function in healthy adults: the healthy volunteer natural relocation study. J Hazard Mater. (2013) 260:183–91. doi: 10.1016/j.jhazmat.2013.05.018
45. Agency for Toxic Substances and Disease Registry. Toxicological Profile for Vanadium. Atlanta, GA: ATSDR. Department of Health and Human Services. (2012). Available at: https://www.ncbi.nlm.nih.gov/books/NBK592343/ (Accessed 3 October 2023).
46. Yoshinaga, J, Yamasaki, K, Yonemura, A, Ishibashi, Y, Kaido, T, Mizuno, K, et al. Lead and other elements in house dust of Japanese residences-source of lead and health risks due to metal exposure. Environ Pollut. (2014) 189:223–8. doi: 10.1016/j.envpol.2014.03.003
47. Srithawirat, T, Latif, MT, and Sulaiman, FR. Indoor PM10 and its heavy metal composition at a roadside residential environment, Phitsanulok, Thailand. Atmosfera. (2016) 29:311–22. doi: 10.20937/ATM.2016.29.04.03
48. Naimabadi A Gholami ARamezani, AM. Determination of heavy metals and health risk assessment in indoor dust from different functional areas in Neyshabur, Iran. Indoor Built Env. (2020) 30:1781–95. doi: 10.1177/1420326X20963378
49. Dingle, JH, Kohl, L, Khan, N, Meng, M, Shi, YA, Pedroza-Brambila, M, et al. Sources and composition of metals in indoor house dust in a mid-size Canadian city. Environ Pollut. (2021) 289:117867. doi: 10.1016/j.envpol.2021.117867
50. Oeder, S, Dietrich, S, Weichenmeier, I, Schober, W, Pusch, G, Jörres, RA, et al. Toxicity and elemental composition of particulate matter from outdoor and indoor air of elementary schools in Munich, Germany. Indoor Air. (2012) 22:148–58. doi: 10.1111/j.1600-0668.2011.00743.x
51. Rivas, I, Viana, M, Moreno, T, Pandolfi, M, Amato, F, Reche, C, et al. Child exposure to indoor and outdoor air pollutants in schools in Barcelona, Spain. Environ Int. (2014) 69:200–12. doi: 10.1016/j.envint.2014.04.009
52. Tran, TD, Nguyen, PM, Nghiem, DT, Le, TH, Tu, MB, Alleman, LY, et al. Assessment of air quality in school environments in Hanoi, Vietnam: a focus on mass-size distribution and elemental composition of indoor-outdoor ultrafine/fine/coarse particles. Atmos. (2020) 11:519. doi: 10.3390/atmos11050519
53. Maghakyan, N, Sahakyan, L, Belyaeva, O, Kafyan, M, and Tepanosyan, G. Assessment of heavy metal pollution level and children’s health risk in a kindergarten area (Yerevan). National Academy of Sciences of RA. Elec J Nat Sci. (2017) 1:10–5.
54. Eeftens, M, Hoek, G, Gruzieva, O, Mölter, A, Agius, R, Beelen, R, et al. Elemental composition of particulate matter and the association with lung function. Epidemiology. (2014) 25:648–57. doi: 10.1097/EDE.0000000000000136
55. Choi, HS, Suh, MJ, Hong, SC, and Kang, JW. The association between the concentration of heavy metals in the indoor atmosphere and atopic dermatitis symptoms in children aged between 4 and 13 years: a pilot study. Children. (2021) 8:1004. doi: 10.3390/children8111004
56. Canepari, S, Astolfi, ML, Drago, G, Ruggieri, S, Tavormina, EE, Cibella, F, et al. PM2.5 elemental composition in indoor residential environments and co-exposure effects on respiratory health in an industrial area. Environ Res. (2023) 216:114630. doi: 10.1016/j.envres.2022.114630
57. Carrion-Matta, A, Kang, CM, Gaffin, JM, Hauptman, M, Phipatanakul, W, Koutrakis, P, et al. Classroom indoor PM2.5 sources and exposures in inner-city schools. Environment. (2019) 131:104–968. doi: 10.1016/j.envint
58. Jung, KH, Torrone, D, Lovinsky-Desir, S, Perzanowski, M, Bautista, J, Jezioro, JR, et al. Short-term exposure to PM2.5 and vanadium and changes in asthma gene DNA methylation and lung function decrements among urban children. Respir Res. (2017) 18:63. doi: 10.1186/s12931-017-0550-9
59. Lener, J, Kucera, J, Kodl, M, and Skokanová, V. Health effects of environmental exposure to vanadium In: J Nriagu, editor. Vanadium in the environment. Part 2: Health effects. New York: John Wiley & Sons, Inc. (1998). 1–19.
60. World Health Organization (WHO). Vanadium. WHO regional Office for Europe: Copenhagen, Denmark, chapter 6.12; (2000). Available at: https://www.euro.who.int/en/search?q=vanadium (Accessed 6 July 2023).
61. Prokopciuk, N, Franck, U, Dudoitis, V, Tarasiuk, N, Juskiene, I, and Valiulis, A. On the seasonal aerosol pollution levels and its sources in some primary schools in Vilnius, Lithuania. Environ Sci Pollut Res. (2020) 27:15592–606. doi: 10.1007/s11356-020-08093-9
62. Linn, WS, Shamoo, DA, and Hackney, JD. Documentation of activity patterns in 'highrisk' groups exposed to ozone in the Los Angeles area In: RL Berglund, editor. Tropospheric ozone and the environment II: Effects, modeling and control. Pittsburgh, PA: Air and Waste Management Association (1992). 701–12.
63. Selley, L, Schuster, L, Marbach, H, Forsthuber, T, Forbes, B, Gant, TW, et al. Brake dust exposure exacerbates inflammation and transiently compromises phagocytosis in macrophages. Metallomics. (2020) 12:371–86. doi: 10.1039/c9mt00253g
Keywords: dust aggregates, microelemental composition, vanadium, respiratory infections, primary school, children
Citation: Prokopciuk N, Taminskiene V, Vaideliene L, Juskiene I, Svist V, Valiulyte I, Valskys V, Valskiene R, Valiulis A, Aukstikalnis T, Vaidelys L, Butikis M, Norkuniene J, Tarasiuk N and Valiulis A (2024) The incidence of upper respiratory infections in children is related to the concentration of vanadium in indoor dust aggregates. Front. Public Health. 12:1339755. doi: 10.3389/fpubh.2024.1339755
Edited by:
Maria Salome Giao, Dyson, United KingdomReviewed by:
Rajendra Prasad Parajuli, Tribhuvan University, NepalEmma Marie Stapleton, The University of Iowa, United States
Julia Fussell, Imperial College London, United Kingdom
Copyright © 2024 Prokopciuk, Taminskiene, Vaideliene, Juskiene, Svist, Valiulyte, Valskys, Valskiene, Valiulis, Aukstikalnis, Vaidelys, Butikis, Norkuniene, Tarasiuk and Valiulis. This is an open-access article distributed under the terms of the Creative Commons Attribution License (CC BY). The use, distribution or reproduction in other forums is permitted, provided the original author(s) and the copyright owner(s) are credited and that the original publication in this journal is cited, in accordance with accepted academic practice. No use, distribution or reproduction is permitted which does not comply with these terms.
*Correspondence: Nina Prokopciuk, bmluYS5wcm9rb3BjaXVrQG1mLnZ1Lmx0
†ORCID: Nina Prokopciuk https://orcid.org/0000-0002-7387-9074
Arunas Valiulis https://orcid.org/0000-0002-0287-9915