- 1Shanghai Pudong New Area Center for Disease Control and Prevention, Shanghai, China
- 2Research Base of Key Laboratory of Surveillance and Early-warning on Infectious Disease in China CDC, Shanghai, China
Objective: This study aimed to evaluate the impact of nonpharmaceutical interventions (NPIs) taken to combat COVID-19 on the prevalence of respiratory viruses (RVs) of acute respiratory infections (ARIs) in Shanghai.
Methods: Samples from ARI patients were collected and screened for 17 respiratory viral pathogens using TagMan low density microfluidic chip technology in Shanghai from January 2019 to December 2020. Pathogen data were analyzed to assess changes in acute respiratory infections between 2019 and 2020.
Results: A total of 2,744 patients were enrolled, including 1,710 and 1,034 in 2019 and 2020, respectively. The total detection rate of RVs decreased by 149.74% in 2020. However, detection rates for human respiratory syncytial virus B (RSVB), human coronavirus 229E (HCoV229E), human coronavirus NL63 (HCoVNL63), and human parainfluenza virus 3 (HPIV3) increased by 91.89, 58.33, 44.68 and 24.29%, in 2020. The increased positive rates of RSVB, HPIV3, resulted in more outpatients in 2020 than in 2019. IFV detection rates declined dramatically across gender, age groups, and seasons in 2020.
Conclusion: NPIs taken to eliminate COVID-19 had an impact on the prevalence of respiratory viral pathogens, especially the IFVs in the early phases of the pandemic. Partial respiratory viruses resurged with the lifting of NPIs, leading to an increase in ARIs infection.
Introduction
Acute respiratory infections (ARIs) are common with significant morbidity and mortality worldwide, causing more than 2.5 million deaths in 2017 (1). Respiratory viruses (RVs), particularly influenza virus, coronavirus, parainfluenzavirus, adenovirus, respiratory syncytial virus, human metapneumovirus, bocavirus, rhinovirus, and enterovirus are known as common viral pathogens causing ARIs (2–10). In late 2019, a new respiratory virus, severe acute respiratory syndrome coronavirus 2 (SARS-CoV-2), emerged and caused more than 2.4 million deaths within a year (11).
Nonpharmaceutical interventions (NPIs) (12) were used to prevent the spread of SARS-CoV-2 nationwide at the beginning of COVID-19 in China, including travel restrictions, physical distancing measures and wearing masks. However, socialdistancing, masking, school closures, shelter-in-place, travel restrictions, etc. (13), also changed the prevalent characteristics of ARIs caused by RVs.
Since 2011, surveillance of RVs has begun with influenza-like illness (ILI) in outpatients (14) and ARI cases (15) in Shanghai. In China, the outbreak of COVID-19 has been quickly contained, but the impact of NPIs on ARIs is still unclear. The research was conducted to investigate and evaluate whether NPI could change the etiological characteristics of ARIs in patients before and during the COVID-19 epidemic in Shanghai between 2019 and 2020.
Materials and methods
Ethics statement
Data on participants in this study were collected from the respiratory surveillance system of Pudong New Area Center for Disease Control and Prevention. Participants gave their verbal informed consent before collecting their information and samples. Samples and information were not collected if refused. No written consent was obtained and no measures were taken to document the process as the data would be analyzed anonymously. All information was input in the respiratory surveillance system after collection. Pursuant to the Helsinki Declaration of 1975, the study protocol and consent procedure were approved by Pudong Centre for Disease Control and Prevention Ethics Review Committee.
Patients and specimen collection
Patients, judged by clinicians, had at least one of the following symptoms: cough, sore throat, runny nose or shortness of breath, with or without fever, were considered eligible for ARI (15). Respiratory samples (nasopharyngeal swab or sputum) and clinical data were collected and recorded by attending physicians of patients with ARI in 9 hospitals in Shanghai between January 2019 and December 2020 according to the ARI surveillance plan. The specimen was stored in 3.5-mL Viral Transport Media (VTM™, Yocon, Cat No: MT0301-1, Beijing, China) and transported to Shanghai Pudong New Area Center for Disease Control and Prevention for pathogen screening.
Screening for pathogens
Suspension of VTM was used directly for nucleic acid extraction. MagNA Pure 96 DNA and Viral NA Small Volume Kit (Roche Diagnostics, Cat. NO: 06543588001, Mannheim, Germany) were used to extract the nucleic acid on MagNA Pure 96 System (Roche Diagnostics, Switzerland) according to the manufacturer’s instructions. The input volume was 200 μL and the elution volume was set at 100 μL.
Pathogens, including Adenovirus (HAdV), Human Coronavirus 229E (HCoV229E), Human Coronavirus HKU1 (HCoVHKU1), Human Coronavirus NL63 (HCoVNL63), Human Coronavirus OC43 (HCoVOC43), Human Enterovirus (EV), Influenza A/H1-2009 (IFVA-H1), Influenza A/H3 (IFVA-H3), Influenza A (IFVA), Influenza B (IFVB), Human Bocavirus (HBoV), Human Metapneumovirus (HMPV), Human Parainfluenza virus 1 (HPIV1), Human Parainfluenza virus 2 (HPIV2), Human Parainfluenza virus 3 (HPIV3), Human Parainfluenza virus 4 (HPIV4), Human Respiratory Syncytial Virus A (RSVA), Human Respiratory Syncytial Virus B (RSVB), Human Rhinovirus (HRV) were screened by the TaqMan low density array (TLDA) method (16) with TagMan low-density microfluidic chip technology by TaqMan™ Fast Advanced Master Mix (Applied Biosystems, Cat. NO: 4398986, USA) according to the manufacturer’s instructions.
Epidemiological description and statistical analysis
On March 13, 2020, COVID-19 was declared a pandemic by WHO1 and on May 8, 2020, The Joint Prevention and Control Mechanism of the State Council issued a guideline on regular prevention and control of COVID-19.2 Accordingly, surveillance data were divided into three periods: Phase I (Jan. 1 to Mar. 1), Phase II (Mar. 1, to May 31), and Phase III (May 31 to Dec. 31). Positive rates and mean percent change for each RV were compared between phases with those of 2019.
The statistical analysis was performed using R 3.2.3 (R Core Team, R: A language and environment for statistical computing. R Foundation for Statistical Computing, Vienna, Austria). The Mantel–Haenszel Chi square test or Fisher’s exact two-tailed test was used to examine differences in discrete variable levels, with the Bonferroni corrected value of p of <(0.05/number of groups) indicating statistical significance.
Results
Characteristics of the study cases
During the two-year study period, a total of 2,744 patients were admitted the study, with 46.3% being female. The patients’ median age was 14.0 years (IQR: 4.00–43.0). The cases were divided into five groups, with 700 (25.50%), 674 (24.60%), 137 (4.99%), 768 (28.00%), and 465 (16.90%) cases, respectively. The most common symptoms of any virus positive patient observed were fever (12.21%), cough (10.39%), runny nose (6.89%), and sore throat (3.21%). Notably, the number of inpatient and outpatient visits in 2020 was significantly lower than the number in 2019. Additionally, there was a significant difference in underlying diseases observed at the time of hospitalization (Table 1).
Viral etiology detected in ARI cases
During the study period, eight different pathogens and their subtypes were detected, however, HBoV was not detected in 2020. The overall detection rate of viral infections was lower in 2020 (15.2%) compared to the same period in 2019 (34.5%), and this difference was found to be statistically significant (p < 0.05). Among the viruses detected, the positive rates of IFV-H1, IFV-H3, IFV-B, RSVA, HPIV1, HPIV2, and HCoVOC43 were decreased in 2020 compared to 2019. However, the detection rates of RSVB, HPIV3, HCoVNL63, and HCoV229E were significantly increased by 91.89, 24.29, 44.68 and 58.33% in 2020 (Table 2).
Changes of viral pathogens in hospital admission
The detection rates of IFVA-H1 (5.1 to 0.45%, p < 0.05), HAdV (8.4 to 2.7%, p < 0.05), RSVA (3.45% to.1454%, p < 0.05) and HMPV (3.15 to 0.45%, p < 0.05) in inpatients and IFVA-H1 (9.49 to 0.49%, p < 0.05), IFVA-H3 (8.34 to 1.6%, p < 0.05), IFVB (6.14 to 2.22%, p < 0.05) and HMPV (1.92 to 0.49%, p < 0.05) in outpatients in 2019 were significantly higher than those in 2020. However, the detection rates of HPIV3 (0.96 to 2.22%, p < 0.05) and RSVB (0.77 to 1.85%) in outpatients were increased significantly in 2020 (Table 2).
Change of viral pathogens in gender
During the study period, significant differences were observed in the detection rate of IFVA-H1, IFVA-H3, IFVB, and HMPV for female ARI patients (8.22 to 0.44%, p < 0.05; 5.38 to 1.75%, p < 0.05; 5.15 to 2.63%, p < 0.05; and 2.21 to 0.66%, p < 0.05, respectively) and male patients (7.37 to 0.63%, p < 0.05; 5.92 to 1.46%, p < 0.05; and 2.57 to 0.42%, p < 0.05, respectively; Table 3).
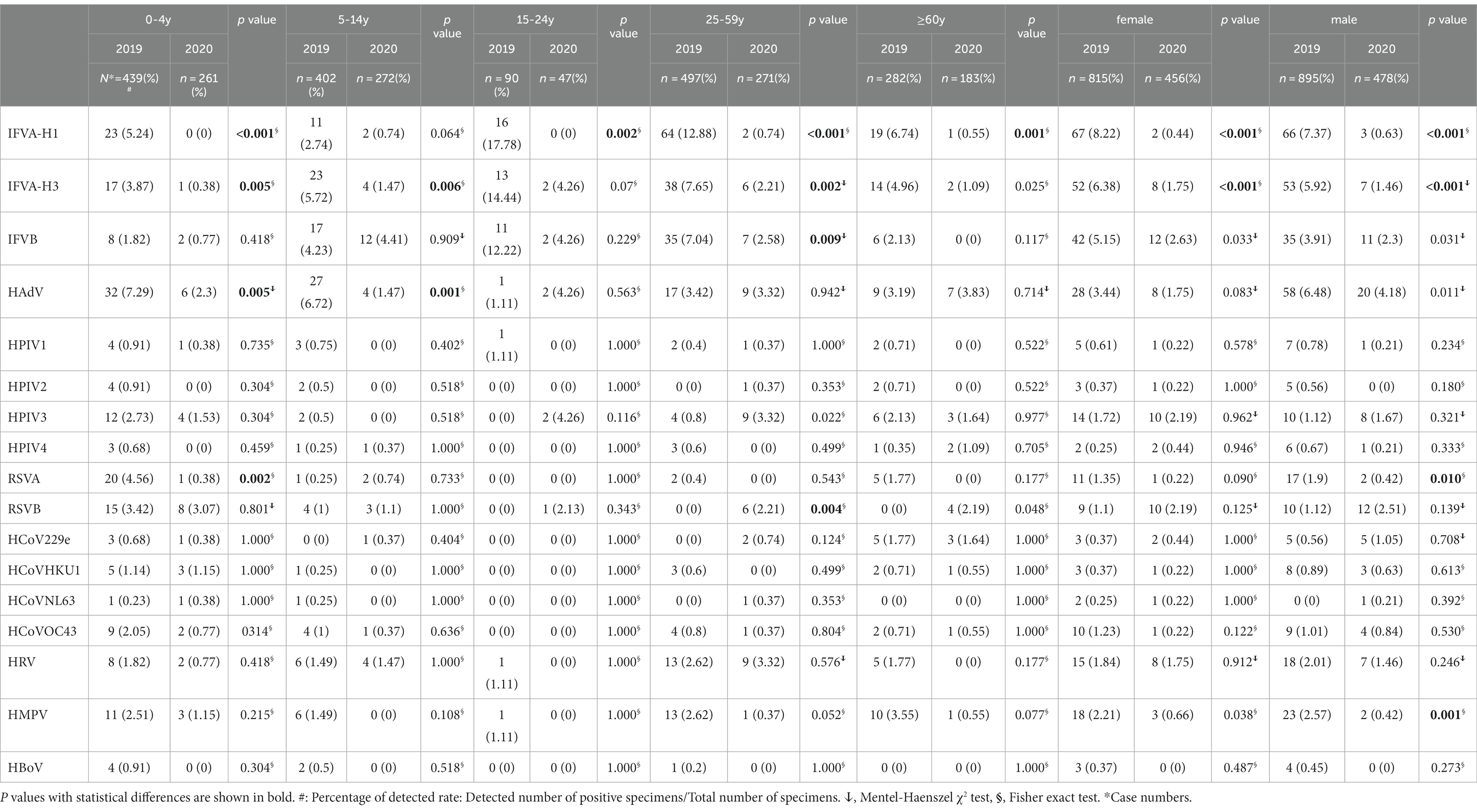
Table 3. Distribution of viral etiology of the ARI-patients by age and gender, Shanghai, China, 2019–2020.
Changes of viral pathogens in age groups
During the study period, the prevalence of IFVA-H1, IFVA-H3, HAdV, and RSVA was higher in the 0–4 year age group in 2019 compared to 2020 by 100, 90.19, 68.45% and 91.67, respectively. Additionally, the 5–14 year age group had a higher prevalence of IFVA-H3, HAdV, and HMPV in 2019 than in 2020 by 74.3, 78.13 and 100%, respectively. For the 15–24 year age group, IFVA-H1was more prevalent in 2019 compared to 2020 while HPIV3 was exactly the opposite, the decrease or increase rate was 100%. Similarly, IFVA-H1, IFVA-H3, IFV-B, and HMPV were significantly more prevalent in the 25–59 year age group in 2019 by 94.25, 71.11, 63.35, and 85.88%, respectively. The older 60 years age group also saw higher prevalence of IFVA-H1, IFVA-H3, in 2019 by 91.84 and 78.02%, respectively. The differences between years were statistically significant (p < 0.05; Table 3).
Changes of viral pathogens in different seasons
In October of 2019, only 5.77% (6/104) of respiratory viral pathogens were screened, compared to 70.14% (155/221) at the beginning of the year. It is evident that during the winter months of 2019 and 2020, there was a significant increase in respiratory virus prevalence. From July 2020, the detection rate gradually rose until reaching its peak in December of that year. Notably, there were no respiratory viruses detected in March, April, May, or July of 2020 (see Figure 1).
Changes of viral pathogens in different career
All 8 surveillance respiratory pathogens were detected in nursery children and students in 2019 and no RVs were detected in teachers in 2020. ARI-patients of medical personnel and farmers were infected with RSV and HCoV in 2020, respectively (Figure 2).
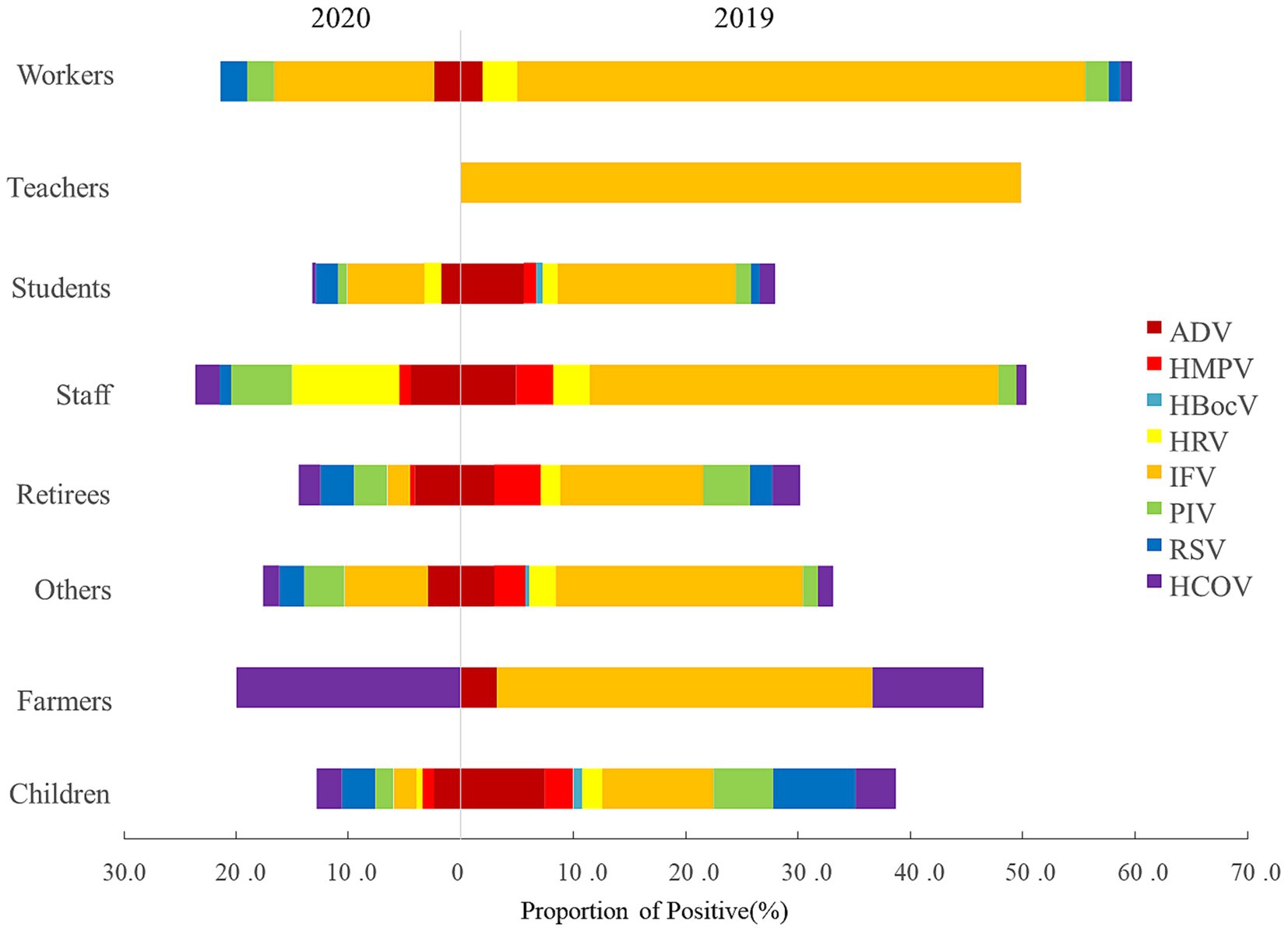
Figure 2. Career distribution of viral etiology in ARI-patients from 2019 to 2020, in Shanghai, China.
Change pattern of positive rate
The detection rates of the RVs decreased from 2019 to 2020 by 22.53% (from 63.05% to 40.52), 37.89% (from 37.89 to 0%), and 9.94% (from 22.71 to 12.77%) in three phases, respectively (Table 4).
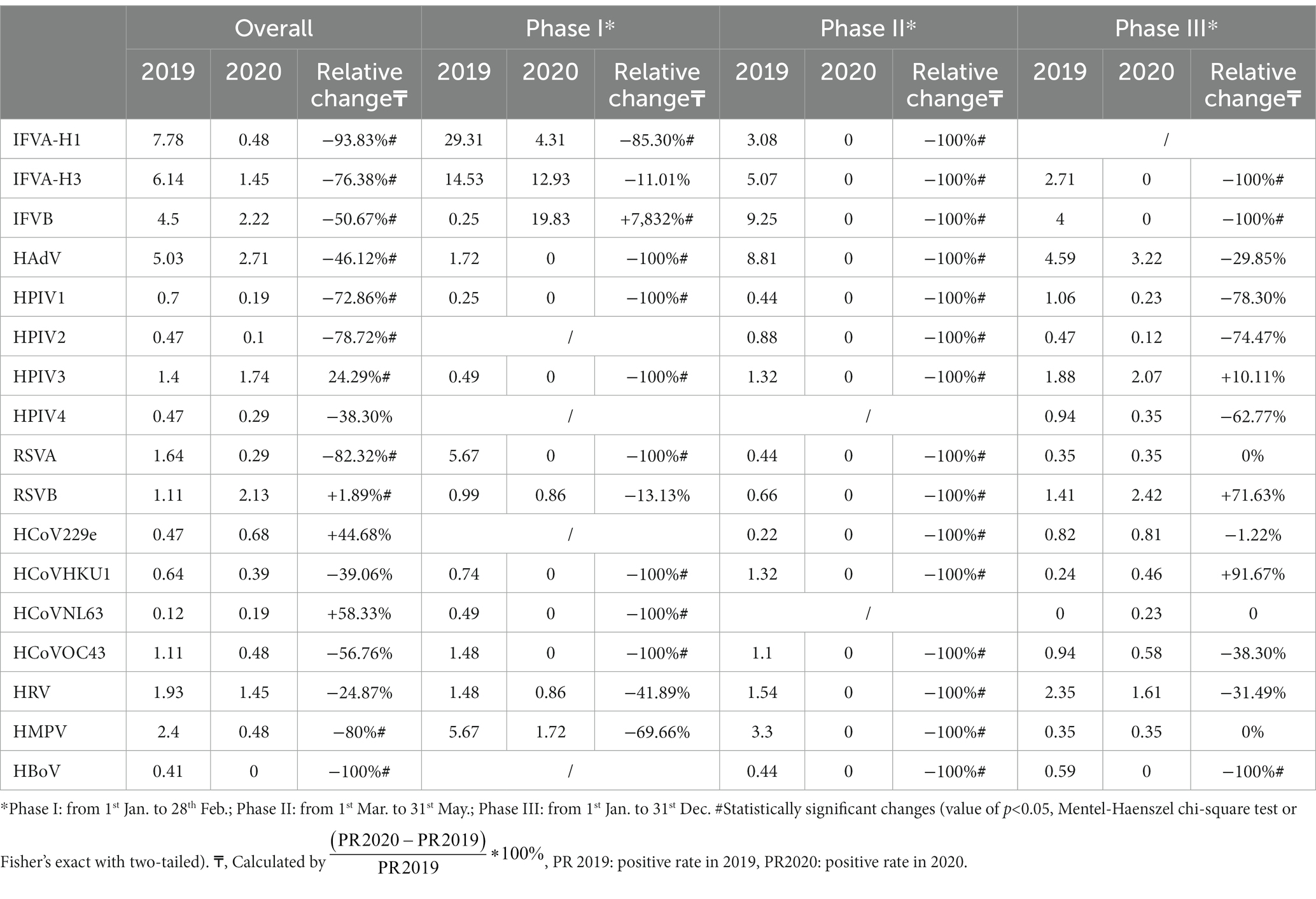
Table 4. Comparison of test positive rate (%) of respiratory viruses between 2019 and 2020 in Shanghai.
Significant changes were identified in test-positive rates for IFV-H1, IFV-H3, IFVB, HAdV, HPIV1, HPIV2, HPIV3, RSVA, RSVB, HBoV and HMPV from 2019 to 2020. The largest decline in annual cumulative positive rates was observed by HBoV (100, 0.41 to 0%), followed by IFVA-H1 (93.83, 7.78 to 0.48%), RSVB (82.32, 1.64 to 0.29%) and HMPV (80, 2.4 to 0.48%). However, the annual cumulative positive rates for RSVB, HCoVNL63, and HCoV229e increased by 91.89% (1.11 to 2.13%), 58.33% (0.12 to 0.19%), and 44.68% (0.47 to 0.68%), respectively (Table 4).
Change pattern in RV detection rates varied significantly in three phases. In Phase I, the results showed that positive rates of HAdV (100%), HPIV1 (100%), HPIV2 (100%), HPIV3 (100%), RSVA (100%), HCoVHKU1 (100%), HCoVNL63 (100%), HCoVOC43 (100%), and IFVA-H1 (85.30%) decreased by more than 85%. The relative change in IFVB’s positive rate was the only increase in this phase and the change ratio was 7,832%. In Phase II, dramatic reductions in RV detection rates were observed and no RV detected in this phase in 2020. In Phase III, the positive rates for IFVA-H3 (100%), IFVB (100%), HBoV (100%) still decreased dramatically. However, positive rates for HCoVHKU1, RSVB and HPIV3 continued to increase, with percentage changes of 91.67, 71.63 and 10.11%, respectively (Table 4).
The detection rates of respiratory viruses decreased across three phases, representing a 22.53, 37.89, and 9.94% reduction. Notably, the annual cumulative positive rates of HBoV, IFVA-H1, RSVB, and HMPV all underwent substantial changes. Some viruses demonstrated an increase in the detection-positive rate, including RSVB, HCoVNL63, and HCoV229e.
Discussion
To combat the COVID-19 pandemic, the Wuhan government implemented measures such as closing entertainment venues, suspending indoor public transport, and banning public gatherings (17). Subsequently, non-pharmaceutical interventions (NPIs) such as limiting social gatherings, wearing masks, practicing hand hygiene, and postponing the spring 2020 semester in primary and secondary schools were put in place to prevent the spread of SARS-CoV-2. There were no effective vaccines available globally at the time, so these NPIs were implemented to mitigate the spread of the virus (13). These interventions also had an impact on other respiratory viruses, including influenza, which was the most significant viral pathogen in ARI cases in Shanghai (14, 15).
This study analyzed the prevalence of respiratory viruses causing acute respiratory infections (ARIs) in Shanghai from 2019 to 2020. Results showed no significant differences in demographic characteristics among ARI patients in 2019 and 2020 (Table 1). Samples of ARI patients were detected by the TaqMan Low Density Array (TLDA) method from January 2019 to December 2020, despite the fact that ARI surveillance in Shanghai began in 2012 (15). This reflects the real impact of non-pharmacological interventions (NPIs) on the prevalence of respiratory viral pathogens in ARI patients.
In light of the directive to remain confined from March 1st to May 30th, 2020, the quantity of specimen collection for ARI cases decreased, which caused the decrease of the respiratory viral pathogens. Although it remains possible that ARIs were present in Shanghai during this period, this measure successfully suppressed the occurrence of respiratory viral pathogens. Correspondingly, a reduction in the number of ARI cases recorded through the surveillance system occurred, decreasing from 1,710 to 1,034, alongside a decrease in the percentage of detection of all viral pathogens, from 34.5 to 15.2%. Notably, the prevalence of ARI cases recorded was comparable to that of 2011–2015 in Shanghai (14, 15), 2016–2019 in Rome (17), and 2019–2020 in Canada (18).
Our study indicates that there are no significant differences in the demographics of ARI patients monitored over 2 years, as shown in Table 1. However, the implementation of NPIs has led to a decline in both outpatient and inpatient visits due to ARI, with the modes of transmission, including droplets, aerosols, and physical contact, being the same as those observed in COVID-19 cases, which were first detected in Shanghai after March 1, 2020. The above pattern has also been observed in respiratory tract infections in other countries, including Japan (19), Germany (20), and the United States (21).
Patients infected with IFVA had clinical symptoms such as fever and chills (22). Generally, the clinical symptoms of RVs’ infection with ARI are mild and should always be ignored. It was surprising to find that the incidence of most clinical features of ARI decreased significantly in our study. Future studies should investigate whether NPIs could relieve infection symptoms. Numerous previous studies (23–27) have shown a decrease in seasonal influenza cases in Europe, Canada, New Zealand, Japan, and the United States in late 2020. Our study indicates that IFVA incidence declined significantly during phase I and phase II, indicating that the prevalence of IFVA in early 2020 may be ending. The relative surge in IFVB cases was noteworthy, with a 7,832% increase observed in phase I, suggesting that IFVB prevalence in Shanghai began in late 2020. However, with the implementation of NPIs, the incidence and positive rates of IFVB became zero in phase II and phase III, effectively preventing the spread of the virus.
Studies in children about ADV infection showed that ADV positive rates in 2020 were significantly lower than the same period in 2019 in Hangzhou (28), Shenzhen (29), China. Our results also showed that ADV was the main RV that infected children under 14 years of age other than IFV, and ADV detection rates were much lower than in 2020.
At the beginning of 2020, a significant decline in the detection rate of RSVA was observed among children below the age of four, which is consistent with findings reported by Australian researchers (30). Furthermore, there was no notable increase in the winter season of 2020, as RSVA has consistently been the leading cause of viral pneumonia in children (23). We found that the increased positive rate of RSVB in the medical personal group in the 25-59y age group, which was also a viral pathogen in our study, increased the detection rate in this age group in Phase III in 2020.
All pathogens were affected by NPI because the test positive rates for Phase II in 2020 were zero. IFVA-H1, HAdV, HPIV1, HPIV3, RSVA, HCoVHKU1, HCoVNL63, and HCoVOC43 decreased significantly and IFVB increased dramatically in phase I. IFVA-H1 and IFVB decreased significantly in phase III. However, the detection rates of IPIV3, RSVB, and HCOVHKU1 increased (Table 4).
The biological characteristics of HCoV are similar to those of SARS-CoV-2. Interestingly, the existing four HCoVs have not changed significantly, which means that the NPIs implemented to prevent the spread of SARS-CoV-2 did not prevent existing human CoVs, especially for HCoVHKU1.
Our study used the same detection methods and patient distribution did not change significantly. There are still a number of limitations and shortcomings. Firstly, the viral activity did not know as the method was based on TLDA, one kind of polymerase chain reaction (PCR), which could not distinguish whether the virus was alive or not, although Ct values can provide information on contagiousness and NPI measures could not be inferred with the detection results. Second, the COVID-19 pandemic could change the behavior of patients with respiratory infections by viral pathogens and their health-seeking behavior could change in the future. Third, the study period is only 2 years, and the small number of Phase II and Phase III samples may lead to some errors and lack of analysis. Fourth, NPIs lead to a harsh environment for respiratory viruses, which may increase the rate of viral mutation in the near future.
Understanding the circulation pattern and prevalence of respiratory viruses is crucial for designing strategies to combat infections and outbreaks. The utilization of NPIs to combat COVID-19 has resulted in behavioral and habitual changes among individuals, consequently impacting the transmission of other respiratory viruses.
Data availability statement
The original contributions presented in the study are included in the article/supplementary material, further inquiries can be directed to the corresponding authors.
Ethics statement
The studies involving humans were approved by Pudong Centre for Disease Control and Prevention Ethics Review Committee. The studies were conducted in accordance with the local legislation and institutional requirements. The human samples used in this study were acquired from The acute respiratory surveillance in Pudong New Area, Shanghai, which began in 2010. Written informed consent for participation was not required from the participants or the participants’ legal guardians/next of kin in accordance with the national legislation and institutional requirements.
Author contributions
LP: Writing – original draft, Conceptualization, Funding acquisition, Data curation, Software. QC: Writing – original draft, Data curation and Software. LH: Conceptualization and Funding acquisition. YY: Investigation. YH: Investigation. QL: Investigation. WZ: Investigation. BZ: Investigation. XZ: Data curation and Software. All authors contributed to the article and approved the submitted version.
Funding
This study was funded by Three-Year Initiative Plan for Strengthening Public Health System Construction in Shanghai (Grant no. GWVI-3), Research Grant for Health Science and Technology of Pudong Health Bureau of Shanghai (Grant no. PW2020A-75), Shanghai Municipal Health Commission’s Clinical Research Project (Grant no. 202240094), Public Health Highland Subject of Pudong Health Commission of Shanghai (Grant no. PWYggy2021-01), Shanghai Municipal Health Commission Key Disciplines (GWVI-11.1-02 Infectious Diseases), Key Discipline Program of Pudong New Area Health System (No. PWZxk2022-25), The Leader of Reserve Subjects of Shanghai Pudong New District Center for Disease Control and Prevention (Grant no. PDCDC-HBXD2020-04).
Acknowledgments
The authors appreciated the staff of the nine sentinel hospitals in Pudong New Area for their assistance with samples and data collection.
Conflict of interest
The authors declare that the research was conducted in the absence of any commercial or financial relationships that could be construed as a potential conflict of interest.
Publisher’s note
All claims expressed in this article are solely those of the authors and do not necessarily represent those of their affiliated organizations, or those of the publisher, the editors and the reviewers. Any product that may be evaluated in this article, or claim that may be made by its manufacturer, is not guaranteed or endorsed by the publisher.
Footnotes
1. ^https://www.who.int/director-general/speeches/detail/who-director-general-s-opening-remarks-at-the-mission-briefing-on-covid-19---13-march-2020
2. ^https://english.www.gov.cn/policies/latestreleases/202005/08/content_WS5eb54d41c6d0b3f0e9497377.html
References
1. Collaborators GCoD. Global, regional, and national age-sex-specific mortality for 282 causes of death in 195 countries and territories, 1980–2017: a systematic analysis for the global burden of disease study 2017. Lancet. (2018) 392:1736–88. doi: 10.1016/S0140-6736(18)32203-7
2. Kenmoe, S, Sadeuh-Mba, SA, Vernet, MA, Penlap Beng, V, Vabret, A, and Njouom, R. Molecular epidemiology of enteroviruses and rhinoviruses in patients with acute respiratory infections in Yaounde, Cameroon. Influenza Other Respir Viruses. (2021) 15:641–50. doi: 10.1111/irv.12851
3. Zhao, Y, Shen, J, Wu, B, Liu, G, Lu, R, and Tan, W. Genotypic diversity and epidemiology of human rhinovirus among children with severe acute respiratory tract infection in Shanghai, 2013–2015. Front Microbiol. (2018) 9:641. doi: 10.3389/fmicb.2018.01836
4. Malta, FC, Varella, RB, Guimarães, MAAM, Miagostovich, MP, and Fumian, TM. Human bocavirus in Brazil: molecular epidemiology, viral load and co-infections. Pathogens. (2020) 9:645. doi: 10.3390/pathogens9080645
5. Barrera, B, Olivares, F, Ruiz, L, Fierro, V, Gutiérrez, V, and López, M. Human metapneumovirus: etiological agent of severe acute respiratory infections in hospitalized and deceased patients with a negative diagnosis of influenza. Pathogens. (2020) 9:85. doi: 10.3390/pathogens9020085
6. Sandesh Kini, BSK, Chandy, S, Shamsundar, R, and Shet, A. Prevalence of respiratory syncytial virus infection among children hospitalized with acute lower respiratory tract infections in southern India. World J Clin Pediatr. (2019) 8:33–42. doi: 10.5409/wjcp.v8.i2.33
7. Yao, L-H, Wang, C, Wei, T-L, Wang, H, Ma, F-L, and Zheng, L-S. Human adenovirus among hospitalized children with respiratory tract infections in Beijing, China, 2017–2018. Virol J. (2019) 16:78. doi: 10.1186/s12985-019-1185-x
8. Álvarez-Argüelles, ME, Rojo-Alba, S, Pérez Martínez, Z, Leal Negredo, Á, Boga Riveiro, JA, Alonso Álvarez, MA, et al. New clinical and seasonal evidence of infections by human Parainfluenzavirus. Eur J Clin Microbiol Infect Dis. (2018) 37:2211–7. doi: 10.1007/s10096-018-3363-y
9. Veiga, ABG, Martins, LG, Riediger, I, Mazetto, A, Debur, MC, and Gregianini, TS. More than just a common cold: endemic coronaviruses OC43, HKU1, NL63, and 229E associated with severe acute respiratory infection and fatality cases among healthy adults. J Med Virol. (2020). 93:1002–1007. doi: 10.2139/ssrn.3590488
10. Ye, C, Zhu, W, Yu, J, Li, Z, Zhang, Y, Wang, Y, et al. Understanding the complex seasonality of seasonal influenza a and B virus transmission: evidence from six years of surveillance data in Shanghai, China. Int J Infect Dis. (2019) 81:57–65. doi: 10.1016/j.ijid.2019.01.027
11. Dong, E, Du, H, and Gardner, L. An interactive web-based dashboard to track COVID-19 in real time. Lancet Infect Dis. (2020) 20:533–4. doi: 10.1016/S1473-3099(20)30120-1
12. Li, Z, Chen, Q, Feng, L, Rodewald, L, Xia, Y, Yu, H, et al. Active case finding with case management: the key to tackling the COVID-19 pandemic. Lancet. (2020) 396:63–70. doi: 10.1016/S0140-6736(20)31278-2
13. Li, ZJ, Yu, LJ, Zhang, HY, Shan, CX, Lu, QB, Zhang, XA, et al. Broad impacts of COVID-19 pandemic on acute respiratory infections in China: an observational study. Clin Infect Dis. (2021) 75:e1054–e1062. doi: 10.1093/cid/ciab942
14. Fu, Y, Pan, L, Sun, Q, Zhu, W, Zhu, L, Ye, C, et al. The clinical and etiological characteristics of influenza-like illness (ILI) in outpatients in Shanghai, China, 2011 to 2013. PLoS One. (2015) 10:e0119513. doi: 10.1371/journal.pone.0119513
15. Ye, C, Zhu, W, Yu, J, Li, Z, Fu, Y, Lan, Y, et al. Viral pathogens among elderly people with acute respiratory infections in Shanghai, China: preliminary results from a laboratory-based surveillance, 2012-2015. J Med Virol. (2017) 89:1700–6. doi: 10.1002/jmv.24751
16. Kodani, M, Yang, G, Conklin, LM, Travis, TC, Whitney, CG, Anderson, LJ, et al. Application of Taq man low-density arrays for simultaneous detection of multiple respiratory pathogens. J Clin Microbiol. (2011) 49:2175–82. doi: 10.1128/JCM.02270-10
17. Ciotti, M, Maurici, M, Santoro, V, Coppola, L, Sarmati, L, De Carolis, G, et al. Viruses of respiratory tract: an observational retrospective study on hospitalized patients in Rome, Italy. Microorganisms. (2020) 8:501. doi: 10.3390/microorganisms8040501
18. Park, KY, Seo, S, Han, J, and Park, JY. Respiratory virus surveillance in Canada during the COVID-19 pandemic: An epidemiological analysis of the effectiveness of pandemic-related public health measures in reducing seasonal respiratory viruses test positivity. PLoS One. (2021) 16:e0253451. doi: 10.1371/journal.pone.0253451
19. Fukuda, Y, Tsugawa, T, Nagaoka, Y, Ishii, A, Nawa, T, Togashi, A, et al. Surveillance in hospitalized children with infectious diseases in Japan: pre- and post-coronavirus disease 2019. J Infect Chemother. (2021) 27:1639–47. doi: 10.1016/j.jiac.2021.07.024
20. Barschkett, M, Koletzko, B, and Spiess, CK. COVID-19 associated contact restrictions in Germany: marked decline in Children’s outpatient visits for infectious diseases without increasing visits for mental health disorders. Children (Basel). (2021) 8:278. doi: 10.3390/children8090728
21. Kaur, R, Schulz, S, Fuji, N, and Pichichero, M. COVID-19 pandemic impact on respiratory infectious diseases in primary care practice in children. Front Pediatr. (2021) 9:722483. doi: 10.3389/fped.2021.722483
22. Zhao, X, Meng, Y, Li, D, Feng, Z, Huang, W, Li, X, et al. Retrospective study of clinical characteristics and viral etiologies of patients with viral pneumonia in Beijing. Pulmonary Circulation. (2021) 11:1–10. doi: 10.1177/20458940211011027
23. Sitammagari, K, Murphy, S, Kowalkowski, M, Chou, SH, Sullivan, M, Taylor, S, et al. Insights from rapid deployment of a “virtual hospital” as standard care during the COVID-19 pandemic. Ann Intern Med. (2021) 174:192–9. doi: 10.7326/M20-4076
24. Huang, QS, Wood, T, Jelley, L, Jennings, T, Jefferies, S, Daniells, K, et al. Impact of the COVID-19 nonpharmaceutical interventions on influenza and other respiratory viral infections in New Zealand. Nat Commun. (2021) 12:1001. doi: 10.1038/s41467-021-21157-9
25. Xu, M, Liu, P, Su, L, Cao, L, Zhong, H, Lu, L, et al. Comparison of respiratory pathogens in children with lower respiratory tract infections before and during the COVID-19 pandemic in Shanghai, China. Front Pediatr. (2022) 10:10. doi: 10.3389/fped.2022.881224
26. Haruka Sakamoto, MI. Peter Ueda seasonal influenza activity during the SARS-CoV-2 outbreak in Japan. JAMA. (2020) 323:1969–71. doi: 10.1001/jama.2020.6173
27. Loren Rodgers, MS, Smith, A, Dietz, S, Jayanthi, P, Yuan, Y, Bull, L, et al. Changes in seasonal respiratory illnesses in the United States during the coronavirus disease (COVID-19) pandemic. Clin Infect Dis 2021. (2019) 73:S110–7. doi: 10.1093/cid/ciab311
28. Li, W, Zhu, Y, Lou, J, Chen, J, Xie, X, and Mao, J. Rotavirus and adenovirus infections in children during COVID-19 outbreak in Hangzhou, China. Translational Pediatrics. (2021) 10:2281–6. doi: 10.21037/tp-21-150
29. Li, L, Wang, H, Liu, A, Wang, R, Zhi, T, Zheng, Y, et al. Comparison of 11 respiratory pathogens among hospitalized children before and during the COVID-19 epidemic in Shenzhen, China. Virol J. (2021) 18:202. doi: 10.1186/s12985-021-01669-y
30. Smith-Vaughan, HC, Binks, MJ, Beissbarth, J, Chang, AB, McCallum, GB, Mackay, IM, et al. Bacteria and viruses in the nasopharynx immediately prior to onset of acute lower respiratory infections in indigenous Australian children. Eur J Clin Microbiol Infect Dis. (2018) 37:1785–94. doi: 10.1007/s10096-018-3314-7
Keywords: COVID-19, acute respiratory infection, nonpharmaceutical interventions, viral pathogen, Shanghai
Citation: Pan L, Yuan Y, Cui Q, Zhang X, Huo Y, Liu Q, Zou W, Zhao B and Hao L (2024) Impact of the COVID-19 pandemic on the prevalence of respiratory viral pathogens in patients with acute respiratory infection in Shanghai, China. Front. Public Health. 12:1230139. doi: 10.3389/fpubh.2024.1230139
Edited by:
Philippe Gautret, IHU Mediterranee Infection, FranceReviewed by:
Mark Aaron Poritz, Co-Diagnostics, Inc., United StatesPhilippe Colson, IHU Mediterranee Infection, France
Copyright © 2024 Pan, Yuan, Cui, Zhang, Huo, Liu, Zou, Zhao and Hao. This is an open-access article distributed under the terms of the Creative Commons Attribution License (CC BY). The use, distribution or reproduction in other forums is permitted, provided the original author(s) and the copyright owner(s) are credited and that the original publication in this journal is cited, in accordance with accepted academic practice. No use, distribution or reproduction is permitted which does not comply with these terms.
*Correspondence: Lifeng Pan, YWxleHBhbjA4MDRAMTYzLmNvbQ==; Lipeng Hao, aGxwbWFpbEAxMjYuY29t
†These authors have contributed equally to this work