- 1Centre for Environmental Sciences, Hasselt University, Diepenbeek, Belgium
- 2Department of Pharmaceutical and Pharmacological Sciences, Experimental Pharmacology, Vrije Universiteit Brussel, Jette, Belgium
- 3Toxicological Centre, University of Antwerp, Wilrijk, Belgium
- 4Biomedical Research Institute (BIOMED), Hasselt University, Diepenbeek, Belgium
- 5School of Public Health, Occupational and Environmental Medicine, Leuven University, Leuven, Belgium
Background/Aim: Human breast milk is the recommended source of nutrition for infants due to its complex composition and numerous benefits, including a decline in infection rates in childhood and a lower risk of obesity. Hence, it is crucial that environmental pollutants in human breast milk are minimized. Exposure to black carbon (BC) particles has adverse effects on health; therefore, this pilot study investigates the presence of these particles in human breast milk.
Methods: BC particles from ambient exposure were measured in eight human breast milk samples using a white light generation under femtosecond illumination. The carbonaceous nature of the particles was confirmed with BC fingerprinting. Ambient air pollution exposures (PM2.5, PM10, and NO2) were estimated using a spatial interpolation model based on the maternal residential address. Spearman rank correlation coefficients were obtained to assess the association between human breast milk’s BC load and ambient air pollution exposure.
Results: BC particles were found in all human breast milk samples. BC loads in human breast milk were strongly and positively correlated with recent (i.e., 1 week) maternal residential NO2 (r = 0.79; p = 0.02) exposure and medium-term (i.e., 1 month) PM2.5 (r = 0.83; p = 0.02) and PM10 (r = 0.93; p = 0.002) exposure.
Conclusion: For the first time, we showed the presence of BC particles in human breast milk and found a robust association with ambient air pollution concentrations. Our findings present a pioneering insight into a novel pathway through which combustion-derived air pollution particles can permeate the delicate system of infants.
Introduction
Human breast milk is a complex mixture of nutrients and bioactive compounds. It is the golden standard for infant feeding and nutrition and optimal for developing infants. The World Health Organization (WHO) and the United Nations Children’s Fund (UNICEF) recommend exclusive breastfeeding for the first 6 months of life for optimal growth, development, and health of the child (1, 2). Multiple studies reported various benefits of breastfeeding for children, including a decline in infection rates in childhood (3), lower risk of obesity (4), lower risk of type 2 diabetes mellitus (5), and lower risk of asthma (6). In addition, on a molecular level, being breastfed during infancy was associated with blood mtDNA content in adolescence. More precisely, the longer the adolescent was postnatally breastfed, the higher the blood mtDNA content was during adolescence, suggesting a duration-dependent effect of breastfeeding (7).
Unfortunately, concerns have emerged regarding the presence of environmental pollutants in human breast milk, and an increasing number of studies have reported their presence in human breast milk (8–12). A recent review by Martin-Carrasco et al. (13) described the presence of environmental chemicals, toxic metals, pesticides, mycotoxins, and per- and polyfluoroalkyl substances (PFAS) in both human breast milk and infant formula. Most of the published studies focused on the presence of environmental chemicals rather than particles, such as black carbon (BC). Environmental BC arises from the incomplete combustion of fossil fuels, wood, and biomass. BC is suspected to be one of the most harmful components responsible for the observed health effects from exposure to traffic-related air pollution (14). Not only were combustion-derived particles found in the frontal cortex of autopsy brain samples (15), kidney biopsies (16), and urine of healthy children (17), BC particles were also detected in placental tissue (18, 19), cord blood, and fetal tissues (i.e., liver, lung, and brain) (19). These findings show that BC has the potential to reach various organ systems. It is unknown whether infants are also exposed to BC via breastfeeding. Several rodent studies have already shown that other nanoparticles could be transferred to the offspring through breast milk (20–22). In fact, silver nanoparticles were distributed to breast milk and subsequently to the brains of breastfed pups (20). However, human studies investigating the presence of BC particles in human breast milk are lacking. Therefore, this study aims to explore the presence of these particles in human breast milk and assess preliminary associations with air pollutants. By shedding light on this issue, we can contribute to the ongoing efforts to mitigate exposure, promote a healthier environment, and ensure future generations’ optimal growth and development.
Materials and methods
Experimental protocol for BC detection in human breast milk
Eight breast milk samples expressed with a breast pump were obtained from non-smoking lactating mothers who voluntarily provided the samples in polyethylene breast milk bags. Samples were sealed and stored at −20°C until further analyses. Ethical approval (B371201216090) for this study was obtained from the Ethical Committees of Hasselt University (Diepenbeek, Belgium) and the East-Limburg Hospital (Genk, Belgium), with all participants providing informed consent. The study protocol was conducted in compliance with the Declaration of Helsinki.
BC particles from ambient exposure present in breast milk were detected using a specific and sensitive detection technique based on the non-incandescence-related white light generation of the particles under femtosecond illumination, as previously described (23). To minimize contamination of the samples, pre-analytical quality control included washing steps of the imaging chambers and blank measurements of these chambers, resulting in no detectable BC particles. Every step was designed and monitored to preclude any possible contamination. We previously confirmed the carbonaceous nature and tissue embedment of the identified BC particles using rigorous validation experiments (18, 19) and performed BC fingerprinting in human breast milk using the reference particles (i.e., conductive carbon black (CCB); 2 μg/mL in Milli-Q; US Research Nanomaterials, Houston, TX, United States). Images of the human breast milk were collected at room temperature using an inverted Zeiss LSM880 confocal microscope (Carl Zeiss, Oberkochen, Germany) equipped with a femtosecond pulsed laser (810 nm, 120 fs, 80 MHz, MaiTai DeepSee, Spectra-Physics, Santa Clara, CA, United States) tuned to a central wavelength of 810 nm using a Plan-Apochromat 20x/0.8 (Carl Zeiss). Two photon-induced white light emission by carbonaceous particles was acquired in the non-descanned mode after spectral separation and emission filtering using 405/10 nm and 550/200 nm band-pass filters. Each breast milk sample was shaken for half an hour and aliquoted at 50 μL per imaging chamber, and five-by-five tile scans were collected 1 μm inwards from the bottom of the imaging chamber (i.e., 170 μm thick 24 × 24 mm coverslip). The resulting tile scans had a field of view of 2124.8 × 2124.8 μm2 containing 25 images with a 2,560 × 2,560 pixel resolution and were recorded with a 1.54 μs pixel dwell time at nine different locations in the imaging chamber. All images were acquired by ZEN Black 2.0 software (Carl Zeiss). An automated and customized MATLAB program (MATLAB 2010, Mathworks, Natick, MA, United States) was used to count the number of black carbon particles in the tile scans recorded for each breast milk-filled imaging chamber (18). First, a peak-finding algorithm detects connected pixels above a specific threshold value. Threshold values of 55 and 99.5% from the highest pixel intensity of the narrow 405/10 nm and broad 550/200 nm channels were used, respectively. These thresholds resulted in reproducible values, which were checked manually using Fiji (ImageJ v2·0, open-source software).1 Next, the detected pixels in both channels are compared, and only the matching ones are used to generate the output image and metrics. The average amount of detected BC particles in breast milk was normalized for the imaging volume using the focal volume estimated from the spatial resolution of the optical system (810 nm, identical settings, 20x/0.8): wx = wy = 0.48 μm and wz = 2.37 μm, defined as, respectively, the sizes of the point spread function in the XY-plane and along the optical axis (z-axis) (radius at the 1/e2 intensity level). Finally, the total relative number, i.e., the number of detected BC particles per mL breast milk, was defined.
Lipid measurements
About 2 mL of breast milk was weighed and liquid–liquid extracted with 5 mL (two times) of n-hexane:acetone (3:1, vol/vol). After extraction, 1 mL of extract was transferred to a small precleaned metal tray and dried at 110° C for 1 h. The weight difference of the tray was further used for lipid content calculation (in %).
Ambient exposure measurements
The ambient PM2.5, PM10, and NO2 concentrations (in μg/m3) were determined with a spatial interpolation model based on the maternal residential address. Full details on the model are described elsewhere (24). Briefly, land cover data from the CORINE land cover dataset were used to interpolate pollution data from the official fixed monitoring stations in Belgium, including 79, 80, and 93 stations for PM2.5, PM10, and NO2, respectively. This model provides interpolated air pollution values on a 4 × 4 km2 grid. We determined different exposure windows by computing the mean daily concentrations 1 week (i.e., recent exposure) and 1 month (i.e., medium-term exposure) before sampling. Residential distance (in meters) to a major road (i.e., a road with more than 10,000 motor vehicles per day) was calculated using geographic information system functions (ArcGIS 9.3; Esri Belux S.A., Wemmel, Belgium) based on the maternal residential address.
Statistical analysis
The data was analyzed and visualized using R (version 4.3.0), RStudio software (version 2023.03.0), and GraphPad Prism (version 9). All data are represented as means ± standard deviation (SD). To assess the relation between residential exposures or breast milk fat content and BC load in human breast milk, Spearman’s correlation coefficients were determined. The normal distribution was examined using the Shapiro–Wilk test.
Results
BC load in human breast milk
Human breast milk samples were obtained from mothers who were on average 32.1 ± 3.9 years old and most of them had a BMI between 18 and 25 kg/m2 (75.0%). To confirm the carbonaceous nature of the particles, BC fingerprinting was performed. The emission fingerprint of identified BC particles in human breast milk resembled the signal of the reference particles (i.e., CCB) (Figure 1A). BC particles were found in all studied samples (Figure 1B), with an average of 8.8 × 105 ± 4.3 × 105 particles per mL of human breast milk, ranging from 3.4 × 105 to 1.6 × 106. BC load and the fat content of the human breast milk samples were not significantly correlated (r = 0.38; p = 0.36).
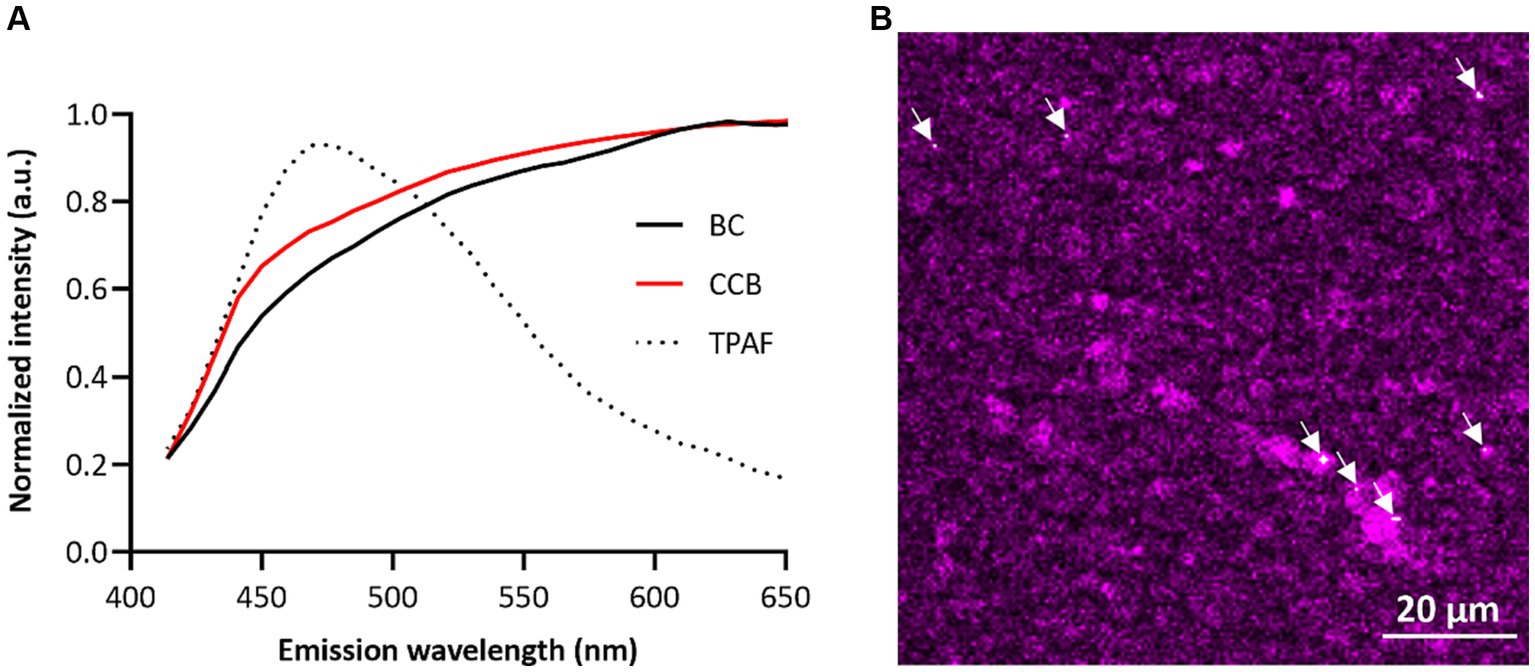
Figure 1. Evidence of BC particles in human breast milk. (A) Emission fingerprinting in human breast milk of BC particles, reference particles (CCB), and two-photon autofluorescence (TRAF) under femtosecond-pulsed illumination. (B) Localization of BC particles in human breast milk. Autofluorescence of human breast milk is given in magenta, and BC particles are shown in white (indicated by the white arrowhead). Scale bar: 20 μm.
BC load in human breast milk and residential exposures
The average maternal residential recent and medium-term air pollution exposures are provided in Table 1, while sample-specific information on residential exposures is listed in Supplementary Table S1. BC loads in human breast milk were strongly and positively correlated with recent maternal residential NO2 exposure (r = 0.79; p = 0.02). Furthermore, medium-term maternal residential PM2.5 (r = 0.83; p = 0.02) and PM10 (r = 0.93; p = 0.0002) exposure were very strongly correlated with BC load in human breast milk (Figure 2). Residential proximity to a major road, ranging from 55.3 to 1775.9 meters (Supplementary Table S1), was not significantly associated with BC load in human breast milk (r = −0.62; p = 0.11; Supplementary Figure S1).
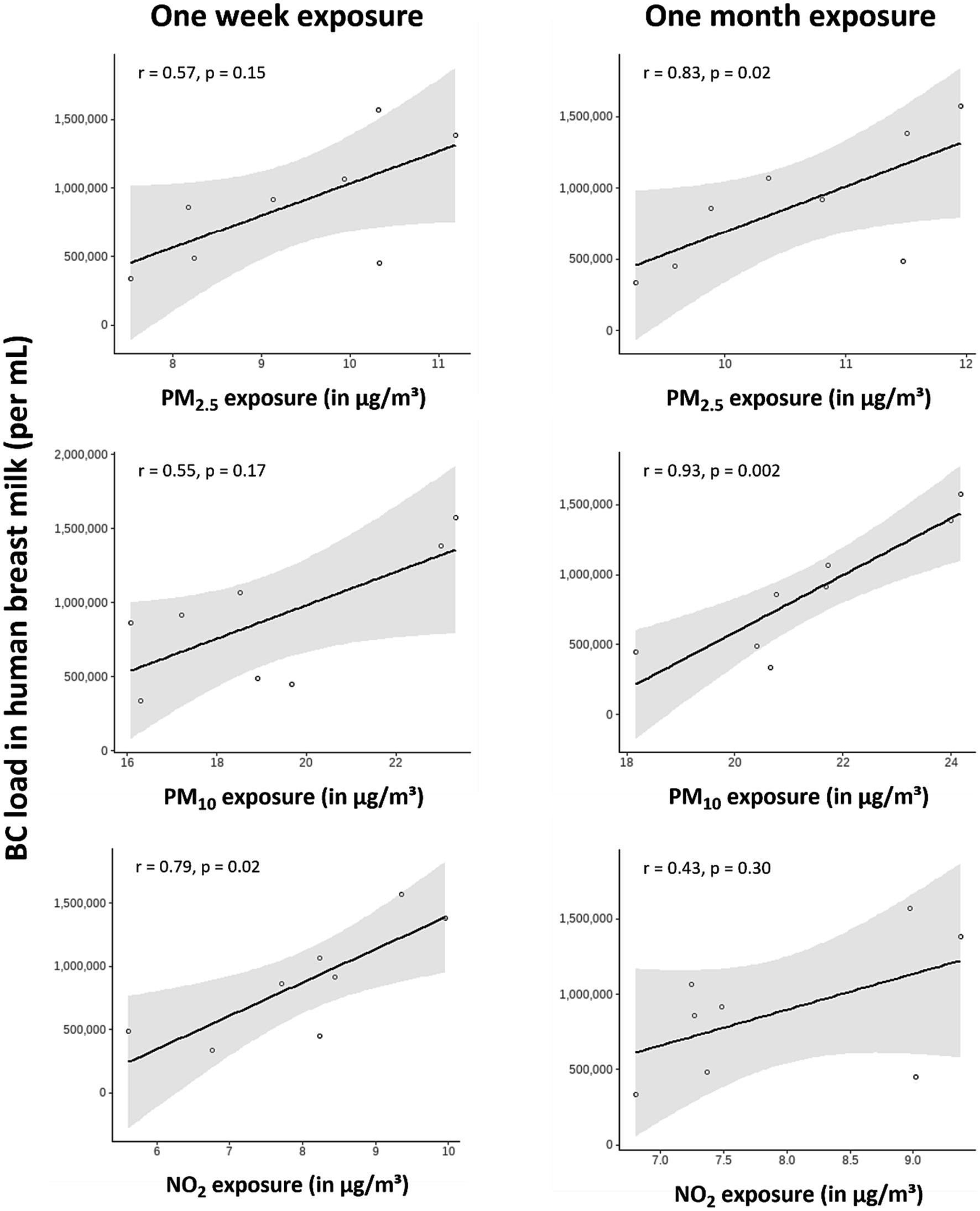
Figure 2. Correlation between BC load in human breast milk and maternal residential air pollution exposures 1 week before sampling (i.e., recent) or 1 month before sampling (i.e., medium-term). Spearman’s correlation coefficients were obtained for all exposures. The solid lines indicate the regression lines with the 95% CIs (grey areas).
Discussion
Our pilot study showed, for the first time, that BC is present in human breast milk and found a strong association with ambient air pollution levels, suggesting that BC particles may be transferred to breast milk after inhalation through the mother. Here, we propose a novel pathway through which BC particles can directly enter the infant’s system, in addition to being exposed via inhalation.
The Developmental Origins of Health and Disease (DOHaD) hypothesis states that environmental exposures during crucial stages of development and growth may have significant consequences on an individual’s short- and long-term health (25). Therefore, it is important to understand the optimal environmental factors, such as diet, during infancy. As optimized nutrition in the first 1,000 days of life (i.e., from conception through the second birthday) is critical for the healthy development of the newborn (26), understanding to which extent environmental pollutants reach the infant through breastfeeding is critical for identifying strategies to minimize exposure and protect mothers’ and infants’ health and well-being. Mothers are exposed to various environmental pollutants on a daily basis, potentially contaminating human breast milk and affecting children’s early development (27). Most of the published studies focused on the presence of environmental chemicals, such as polychlorinated biphenyls, persistent organic pollutants, PFAS, organochlorine pesticides, polybrominated diphenyl esters, and phthalates (8, 13). However, human studies investigating the presence of environmental particles are scarce. Ragusa and colleagues detected microplastics for the first time in human breast milk, with a size ranging from 3 to 12 μm (28). Furthermore, nanoplastics had a high binding affinity to secretory immunoglobulin A, an antibody in human breast milk that plays a crucial role in disease protection, potentially interfering with the development of the infant’s immune system (29).
While studies investigating environmental particles in human breast milk are limited, several rodent studies have shown that nanoparticles could be transferred to the offspring through breast milk (20–22, 30, 31). In rats, silver nanoparticles were distributed to breast milk (20, 31) and subsequently to the brains of breastfed pups (20). In addition, zinc oxide nanoparticles were distributed to the intestine and liver of rat pups via breast milk (22). Furthermore, quantum dots (i.e., semiconductor nanocrystals) were detected in the breast milk of rats and the stomach and intestine of their offspring, inducing growth restriction (30). In mice, metal oxide nanoparticles were found in breast milk and induced growth retardation in their offspring (21). To date, no research has focused on the presence of traffic-related particulate matter, such as BC, in human breast milk, making our study the first to provide evidence that BC can be detected in this human biological matrix.
A study assessing the deposition and retention of ultrafine carbon particles in the respiratory tract found that 75% of inhaled carbon particles in healthy nonsmokers were persistent in the airways 1 day after exposure. However, only about 3% of the carbon particles in the lung periphery were cleared within 24 h (32). Due to the stability of the carbon particles, long-term retention in the maternal lung periphery is expected. Although this might increase the inter-individual variability of BC load, we found a strong association between ambient air pollution exposures and BC load in human breast milk. We observed a stronger correlation between BC load in human breast milk and ambient PM10 levels than between BC load in human breast milk and ambient PM2.5 concentrations. Although typically more than 90% of BC resided in the PM2.5 size fraction, a one-year measurement period of BC, PM2.5, and PM10 in Finland showed that on an annual level, BC accounted for 14 ± 8% and 7 ± 4% of the total PM2.5 and PM10, respectively (33). Furthermore, the study by Gong W and colleagues (34) reported a similarly high correlation between BC and PM2.5 and PM10. We observed two strong correlations, yet given the restricted sample size, we are careful not to overinterpret this difference. In contrast with our study, living close to a major road was associated with higher urinary BC load (17). We found a similar trend in our results, but did not reach the significance level, possibly due to the limited sample size. Bongaerts et al. (19) reported the BC load in maternal blood to range between ~3.0 × 104 and 1.6 × 106 particles per mL blood, which is slightly lower compared with the BC load in human milk in our study, although our limited sample size should be considered in this comparison.
Notably, BC particles might have an affinity for attaching to the fat globules within human breast milk. Given that the fat content in human breast milk varies within feeds (i.e., foremilk and hindmilk), during lactation, and depends on the individual maternal characteristics (35, 36), this could lead to a higher variability in BC load in human breast milk samples. However, we did not find a significant correlation between the fat content and BC load in human breast milk.
While it may be concerning to find environmental pollutants in human breast milk, it is crucial to emphasize that this should not undermine breastfeeding. Numerous studies and reviews have consistently stated that the risks of not breastfeeding far outweigh the potential risks associated with the exposure to pollutants (37, 38) and also the data of the current study do not argue against breastfeeding. Nonetheless, some studies have hinted at the possibility that pollutants in human breast milk could somewhat diminish the developmental advantages of breastfeeding (9). However, it’s worth noting that this attenuation is not statistically significant when considering other influential factors in child development, such as parental influence and home environment, after appropriate control measures are applied (9). Thus, instead of discouraging breastfeeding, these findings should serve as a clarion call for governments and policymakers to take decisive action on environmental health. By addressing and mitigating the sources of pollution in our environment, we can create a safer and healthier world for children to grow up in. This endeavor aligns seamlessly with the United Nations’ Sustainable Development Goals, which aim to ensure that future generations have the best chance at healthy development within a sustainable and clean environment (39).
Strengths and limitations
Our study has several strengths. We detected for the first time BC particles in human breast milk samples. Our biocompatible and label-free detection method enables the precise and highly sensitive detection of ambient BC particles in their biological context (23). We confirmed the carbonaceous nature of the BC particles in human breast milk through emission fingerprinting. In addition to PM2.5 and PM10 levels, we included ambient NO2 concentrations, as its concentration is above the average of the European concentration and is generally higher in the Flemish Region of Belgium, where road traffic is the leading source of NO2 emission, compared to the Walloon Region (40). Some limitations should also be acknowledged. In this pilot study, we used a limited sample size (n = 8) to assess the presence of ambient BC particles in human breast milk. A larger sample size is required to provide a better estimation of the variability of BC load in human milk. Nonetheless, our study showed that BC particles can reach the nursing infant through breastfeeding, as the particles were detected in all studied samples and were strongly correlated with modelled air pollution exposures. The study design does not allow to draw conclusions about the translocation of BC from the intestine to the blood. The potentially harmful effects of BC exposure can be caused by transfer to the circulation but can also be due to its ability to disrupt the milk or gut microbiome of the child. Although other sources of exposure (e.g., while commuting or at work) can influence an individual’s exposure to air pollutants, mother’s residential exposures were strongly and positively linked with particles present in maternal and cord blood (19), as well as in placental tissue (17–19), suggesting a suitable estimation of individual exposures.
Conclusion
We found evidence of BC particles in human breast milk, suggesting a novel pathway through which BC particles can directly enter the infant’s system. While the presence of environmental BC particles in human breast milk is a cause for concern, it also serves as a call to action. The findings of our study could have important implications for public health policies aimed at promoting breastfeeding and protecting infants from the potential harms of environmental pollutants.
Data availability statement
The data used in this study are not publicly available because they contain information that could compromise research participant privacy but are available within General Data Protection Regulation restrictions from the corresponding author upon reasonable request.
Ethics statement
The studies involving humans were approved by the Ethical Committees of Hasselt University (Diepenbeek, Belgium) and the East-Limburg Hospital (Genk, Belgium). The studies were conducted in accordance with the local legislation and institutional requirements. The participants provided their written informed consent to participate in this study.
Author contributions
CC: Conceptualization, Formal analysis, Investigation, Visualization, Writing – original draft, Writing – review & editing. EB: Investigation, Validation, Writing – review & editing. KV: Validation, Visualization, Writing – review & editing. BR: Data curation, Writing – review & editing. AS: Formal analysis, Writing – review & editing. ET: Writing – review & editing. GP: Investigation, Writing – review & editing. MA: Methodology, Writing – review & editing. TN: Conceptualization, Methodology, Writing – review & editing. MP: Conceptualization, Supervision, Writing – review & editing.
Funding
The author(s) declare financial support was received for the research, authorship, and/or publication of this article. CC is financially supported by the Special Research Fund (BOF) from Hasselt University (BOF22PD04) and Fund Orcadia (2022-E2210890-228297), managed by the King Baudouin Foundation. The Research Foundation Flanders (FWO) financially supported EB (G08231N) and KV (G059219). AS is financially supported by the SURREAL project, which has received funding from the European Union’s Horizon 2020 Research and Innovation Programme under grant agreement no 956780. TN is financially supported by the Methusalem Fund.
Conflict of interest
MA and TN declare that aspects of the work are subject of a patent application (method for detecting or quantifying carbon black and/or black carbon particles, US20190025215A1) filed by Hasselt University (Hasselt, Belgium) and KU Leuven (Leuven, Belgium).
The remaining authors declare that the research was conducted in the absence of any commercial or financial relationships that could be construed as a potential conflict of interest.
The author(s) declared that they were an editorial board member of Frontiers, at the time of submission. This had no impact on the peer review process and the final decision.
Publisher’s note
All claims expressed in this article are solely those of the authors and do not necessarily represent those of their affiliated organizations, or those of the publisher, the editors and the reviewers. Any product that may be evaluated in this article, or claim that may be made by its manufacturer, is not guaranteed or endorsed by the publisher.
Supplementary material
The Supplementary material for this article can be found online at: https://www.frontiersin.org/articles/10.3389/fpubh.2023.1333969/full#supplementary-material
Footnotes
References
1. Assembly WH. Global Strategy for Infant and Young Child Feeding: The Optimal duration of Exclusive Breastfeeding. Geneva, Switzerland: World Health Organization (2001).
3. Kramer, MS, and Kakuma, R. Optimal duration of exclusive breastfeeding. Cochrane Database Syst Rev. (2012) 2012:CD003517. doi: 10.1002/14651858.CD003517.pub2
4. Horta, BL, and Victora, CG. Long-Term Effects of Breastfeeding: A Systematic Review. World Health Organization (2013). Available at: https://www.who.int/publications/i/item/9789241505307
5. Horta, BL, and de Lima, NP. Breastfeeding and type 2 diabetes: systematic review and Meta-analysis. Curr Diab Rep. (2019) 19:1. doi: 10.1007/s11892-019-1121-x
6. Oddy, WH, Holt, PG, Sly, PD, Read, AW, Landau, LI, Stanley, FJ, et al. Association between breast feeding and asthma in 6 year old children: findings of a prospective birth cohort study. BMJ. (1999) 319:815–9. doi: 10.1136/bmj.319.7213.815
7. Cosemans, C, Nawrot, TS, Janssen, BG, Vriens, A, Smeets, K, Baeyens, W, et al. Breastfeeding predicts blood mitochondrial DNA content in adolescents. Sci Rep. (2020) 10:387. doi: 10.1038/s41598-019-57276-z
8. Lehmann, GM, LaKind, JS, Davis, MH, Hines, EP, Marchitti, SA, Alcala, C, et al. Environmental Chemicals in Breast Milk and Formula: exposure and risk assessment implications. Environ Health Perspect. (2018) 126:96001. doi: 10.1289/EHP1953
9. LaKind, JS, Lehmann, GM, Davis, MH, Hines, EP, Marchitti, SA, Alcala, C, et al. Infant dietary exposures to environmental chemicals and infant/child health: a critical assessment of the literature. Environ Health Perspect. (2018) 126:96002. doi: 10.1289/EHP1954
10. Zheng, G, Schreder, E, Dempsey, JC, Uding, N, Chu, V, Andres, G, et al. Per- and polyfluoroalkyl substances (PFAS) in breast Milk: concerning trends for current-use PFAS. Environ Sci Technol. (2021) 55:7510–20. doi: 10.1021/acs.est.0c06978
11. Landrigan, PJ, Sonawane, B, Mattison, D, McCally, M, and Garg, A. Chemical contaminants in breast milk and their impacts on children's health: an overview. Environ Health Perspect. (2002) 110:A313–5. doi: 10.1289/ehp.021100313
12. Szukalska, M, Merritt, TA, Lorenc, W, Sroczyńska, K, Miechowicz, I, Komorowicz, I, et al. Toxic metals in human milk in relation to tobacco smoke exposure. Environ Res. (2021) 197:111090. doi: 10.1016/j.envres.2021.111090
13. Martín-Carrasco, I, Carbonero-Aguilar, P, Dahiri, B, Moreno, IM, and Hinojosa, M. Comparison between pollutants found in breast milk and infant formula in the last decade: a review. Sci Total Environ. (2023) 875:162461. doi: 10.1016/j.scitotenv.2023.162461
14. Janssen, NA, Hoek, G, Simic-Lawson, M, Fischer, P, van Bree, L, ten Brink, H, et al. Black carbon as an additional indicator of the adverse health effects of airborne particles compared with PM10 and PM2.5. Environ Health Perspect. (2011) 119:1691–9. doi: 10.1289/ehp.1003369
15. Maher, BA, Ahmed, IA, Karloukovski, V, MacLaren, DA, Foulds, PG, Allsop, D, et al. Magnetite pollution nanoparticles in the human brain. Proc Natl Acad Sci U S A. (2016) 113:10797–801. doi: 10.1073/pnas.1605941113
16. Rasking, L, Koshy, P, Bongaerts, E, Bové, H, Ameloot, M, Plusquin, M, et al. Ambient black carbon reaches the kidneys. Environ Int. (2023) 177:107997. doi: 10.1016/j.envint.2023.107997
17. Saenen, ND, Bové, H, Steuwe, C, Roeffaers, MBJ, Provost, EB, Lefebvre, W, et al. Children's urinary environmental carbon load. A novel marker reflecting residential ambient air pollution exposure? Am J Respir Crit Care Med. (2017) 196:873–81. doi: 10.1164/rccm.201704-0797OC
18. Bové, H, Bongaerts, E, Slenders, E, Bijnens, EM, Saenen, ND, Gyselaers, W, et al. Ambient black carbon particles reach the fetal side of human placenta. Nat Commun. (2019) 10:3866. doi: 10.1038/s41467-019-11654-3
19. Bongaerts, E, Lecante, LL, Bové, H, Roeffaers, MBJ, Ameloot, M, Fowler, PA, et al. Maternal exposure to ambient black carbon particles and their presence in maternal and fetal circulation and organs: an analysis of two independent population-based observational studies. Lancet Planet Health. (2022) 6:e804–11. doi: 10.1016/S2542-5196(22)00200-5
20. Morishita, Y, Yoshioka, Y, Takimura, Y, Shimizu, Y, Namba, Y, Nojiri, N, et al. Distribution of silver nanoparticles to breast milk and their biological effects on breast-fed offspring mice. ACS Nano. (2016) 10:8180–91. doi: 10.1021/acsnano.6b01782
21. Cai, J, Zang, X, Wu, Z, Liu, J, and Wang, D. Translocation of transition metal oxide nanoparticles to breast milk and offspring: the necessity of bridging mother-offspring-integration toxicological assessments. Environ Int. (2019) 133:105153. doi: 10.1016/j.envint.2019.105153
22. Hussain, A, Kumar, S, and Kaul, G. Postnatal distribution of ZnO nanoparticles to the breast milk through oral route and their risk assessment for breastfed rat offsprings. Hum Exp Toxicol. (2020) 39:1318–32. doi: 10.1177/0960327120921441
23. Bové, H, Steuwe, C, Fron, E, Slenders, E, D'Haen, J, Fujita, Y, et al. Biocompatible label-free detection of carbon black particles by femtosecond pulsed laser microscopy. Nano Lett. (2016) 16:3173–8. doi: 10.1021/acs.nanolett.6b00502
24. Janssen, S, Dumont, G, Fierens, F, and Mensink, C. Spatial interpolation of air pollution measurements using CORINE land cover data. Atmos Environ. (2008) 42:4884–903. doi: 10.1016/j.atmosenv.2008.02.043
25. Barker, DJ . Fetal origins of coronary heart disease. BMJ. (1995) 311:171–4. doi: 10.1136/bmj.311.6998.171
26. Beluska-Turkan, K, Korczak, R, Hartell, B, Moskal, K, Maukonen, J, Alexander, DE, et al. Nutritional gaps and supplementation in the first 1000 days. Nutrients. (2019) 11:2891. doi: 10.3390/nu11122891
27. Leibson, T, Lala, P, and Ito, S. Chapter 24 - drug and chemical contaminants in breast milk: effects on neurodevelopment of the nursing infant In: W Slikker, MG Paule, and C Wang, editors. Handbook of Developmental Neurotoxicology. 2nd ed: Academic Press (2018). 275–84. Available at: https://www.sciencedirect.com/book/9780128094051/handbook-of-developmental-neurotoxicology#book-info
28. Ragusa, A, Notarstefano, V, Svelato, A, Belloni, A, Gioacchini, G, Blondeel, C, et al. Raman microspectroscopy detection and characterisation of microplastics in human breastmilk. Polymers. (2022) 14:2700. doi: 10.3390/polym14132700
29. Enyoh, CE, Ovuoraye, PE, Qingyue, W, and Wang, W. Examining the impact of nanoplastics and PFAS exposure on immune functions through inhibition of secretory immunoglobin a in human breast milk. J Hazard Mater. (2023) 459:132103. doi: 10.1016/j.jhazmat.2023.132103
30. Yang, L, Kuang, H, Zhang, W, Wei, H, and Xu, H. Quantum dots cause acute systemic toxicity in lactating rats and growth restriction of offspring. Nanoscale. (2018) 10:11564–77. doi: 10.1039/C8NR01248B
31. Melnik, EA, Buzulukov, YP, Demin, VF, Demin, VA, Gmoshinski, IV, Tyshko, NV, et al. Transfer of silver nanoparticles through the placenta and breast Milk during in vivo experiments on rats. Acta Nat. (2013) 5:107–15. doi: 10.32607/20758251-2013-5-3-107-115
32. Möller, W, Felten, K, Sommerer, K, Scheuch, G, Meyer, G, Meyer, P, et al. Deposition, retention, and translocation of ultrafine particles from the central airways and lung periphery. Am J Respir Crit Care Med. (2008) 177:426–32. doi: 10.1164/rccm.200602-301OC
33. Viidanoja, J, Sillanpää, M, Laakia, J, Kerminen, V-M, Hillamo, R, Aarnio, P, et al. Organic and black carbon in PM2.5 and PM10: 1 year of data from an urban site in Helsinki, Finland. Atmos Environ. (2002) 36:3183–93. doi: 10.1016/S1352-2310(02)00205-4
34. Gong, W, Zhang, T, Zhu, Z, Ma, Y, Ma, X, and Wang, W. Characteristics of PM1.0, PM2.5, and PM10, and their relation to black carbon in Wuhan, Central China. Atmos. (2015) 6:1377–87. doi: 10.3390/atmos6091377
35. Pietrzak-Fiećko, R, and Kamelska-Sadowska, AM. The comparison of nutritional value of human Milk with other Mammals' Milk. Nutrients. (2020) 12:1404. doi: 10.3390/nu12051404
36. Neville, MC, Keller, RP, Seacat, J, Casey, CE, Allen, JC, and Archer, P. Studies on human lactation. I. Within-feed and between-breast variation in selected components of human milk. Am J Clin Nutr. (1984) 40:635–46. doi: 10.1093/ajcn/40.3.635
37. Mead, MN . Contaminants in human milk: weighing the risks against the benefits of breastfeeding. Environ Health Perspect. (2008) 116:A427–34. doi: 10.1289/ehp.116-a426
38. Lapillonne, A, Bocquet, A, Briend, A, Chouraqui, JP, Darmaun, D, Feillet, F, et al. Pollutants in breast milk: a public health perspective – a commentary of the nutrition Committee of the French Society of pediatrics. J Pediatr Gastroenterol Nutr. (2021) 72:343–6. doi: 10.1097/MPG.0000000000002928
39. United Nations. (2023). Sustainable Development Goals. Available at: https://sdgs.un.org/goals (Accessed October 4, 2023).
Keywords: black carbon, human breast milk, public health, infants, air pollution
Citation: Cosemans C, Bongaerts E, Vanbrabant K, Reimann B, Silva AI, Tommelein E, Poma G, Ameloot M, Nawrot TS and Plusquin M (2024) Black carbon particles in human breast milk: assessing infant’s exposure. Front. Public Health. 11:1333969. doi: 10.3389/fpubh.2023.1333969
Edited by:
Xianliang Wang, National Institute of Environmental Health, ChinaReviewed by:
Hakan Tinnerberg, University of Gothenburg, SwedenJingyuan Xiong, Sichuan University, China
Copyright © 2024 Cosemans, Bongaerts, Vanbrabant, Reimann, Silva, Tommelein, Poma, Ameloot, Nawrot and Plusquin. This is an open-access article distributed under the terms of the Creative Commons Attribution License (CC BY). The use, distribution or reproduction in other forums is permitted, provided the original author(s) and the copyright owner(s) are credited and that the original publication in this journal is cited, in accordance with accepted academic practice. No use, distribution or reproduction is permitted which does not comply with these terms.
*Correspondence: Michelle Plusquin, michelle.plusquin@uhasselt.be