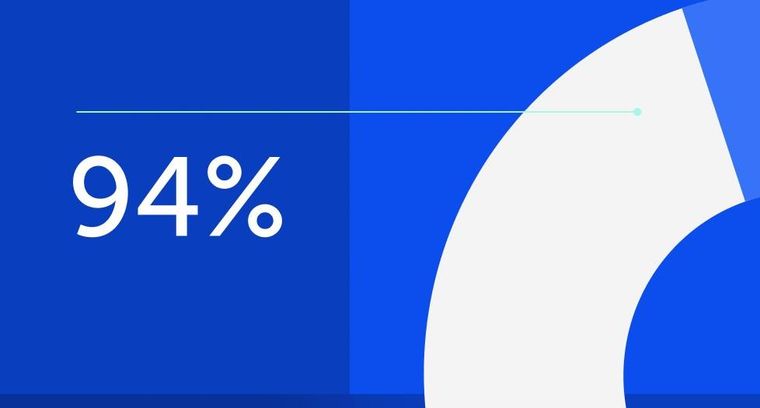
94% of researchers rate our articles as excellent or good
Learn more about the work of our research integrity team to safeguard the quality of each article we publish.
Find out more
ORIGINAL RESEARCH article
Front. Public Health, 30 November 2023
Sec. Infectious Diseases: Epidemiology and Prevention
Volume 11 - 2023 | https://doi.org/10.3389/fpubh.2023.1282413
This article is part of the Research TopicCurrent evidence on epidemiology and management of infections in critically ill patientsView all 5 articles
Background and aims: Carbapenem-resistant Acinetobacter baumannii (CRAB) has become a leading cause of nosocomial infections with an increasing impact on critically ill patients, yet there is limited data on contributing factors. This study was aim to evaluate the prevalence and risk factors, and clinical outcomes of CRAB infections among critically ill children in a tertiary university teaching hospital in China.
Methods: From January 2016 to December 2021, all children diagnosed with nosocomial Acinetobacter baumannii (A. baumannii) infections in the pediatric intensive care unit (PICU) were identified through the computerized microbiology laboratory databases. Among them, children suffering from CRAB infection were designated as a case group, while children with carbapenem susceptible A. baumannii (CSAB) infection were assigned to a control group. This retrospective case-control study was based on two groups of patients to determine potential clinical factors contributing to CRAB infection and death among critically ill children via univariate and multivariate analyses.
Results: During the 6-year study period, a total of 372 episodes of nosocomial A. baumannii infection in the PICU were eligible and included in the study. These isolates displayed moderate or high rates of resistance to all tested antimicrobials except colistin. The overall prevalence of CRAB and MDRAB (multidrug-resistant A. baumannii) was 78.0% and 80.9%, respectively. Several risk factors found to significantly increase CRAB infection included receiving invasive operation (OR = 9.412, p = 0.001), gastric intubation (OR = 2.478, p = 0.026), prior carbapenems exposure (OR = 2.543, p = 0.003), severe pneumonia (OR = 3.235, p = 0.001), and hemoglobin <110g/L (OR = 3.049, p = 0.005). Of 372 patients with CRAB infection, the mortality rate was 30.9% (115/372) and mortality did not differ between children with CRAB and CSAB infections. Septic shock (OR = 2.992, p = 0.001), AST > 46U/L (OR = 2.015, p = 0.005), bone marrow aspiration (OR = 2.704, p = 0.008), lymphocyte <20 % (OR = 1.992, p = 0.006) and age (OR = 1.094, p = 0.002) were independent risk factors for the death of A. baumanni infection.
Conclusions: This study highlights considerable incidence rate and remarkable mortality of children with A. baumanni (especially CRAB) infections, and identifies age-specific risk factors for CRAB infection and mortality in critically ill children. These risk factors should be taken into account in pediatric hospitals in order to establish early intervention and rational treatment to improve clinical outcomes.
Acinetobacter baumannii is one of the most important nosocomial pathogens, with the ability to cause infections such as ventilator-associated pneumonia, surgical site infections, urinary tract infections, bloodstream infections and meningitis (1, 2). These infections are more likely to occur in intensive care unit (ICU) patients, although transmission to patients in general wards and long-term care facilities is increasing (3). A study conducted at one hospital in India found that A. baumannii accounted for 9.4% of all Gram-negative bacteria in the entire hospital and 22.6% in the ICU (4). According to the Infectious Disease Surveillance of Pediatrics (ISPED) program in China, A. baumannii was among the 10 most frequent etiologic agents and was the fourth leading pathogen responsible for traumatic infections in children (5). The European Antimicrobial Resistance Surveillance Network (EARS-Net) reported that 29 countries isolated 7622 Acinetobacter spp, constituting 2.3% of all isolates in 2020 (6). In the United States, a national surveillance report claims that A. baumannii was responsible for 1.8% of all healthcare-associated infections, with an estimated 45,000 Acinetobacter infections per year and 1 million cases per year worldwide (7).
A. baumannii has become a successful nosocomial pathogen worldwide due to the combination of its environmental adaptation and rapid development of resistance to multiple antimicrobials (8). Given its innate or acquired capacity for antibiotic resistance, carbapenem-resistant or multidrug-resistant (MDR) A. baumannii has substantially increased. In China, an antimicrobial resistance surveillance network indicated that the prevalence of CRAB showed a significantly increasing trend from 39.0% in 2005 to 71.9% in 2022 with a peak of 79.0% in 2019 (9). In the USA, the proportion of CRAB in children was on the rise, which increased each year by 8% from 1999 to 2012 (10). Compared with 2016-2019, the percentage of carbapenems resistant to Acinetobacter spp. in the European Union/European Economic Area (EU/EEA) increased significantly in 2020, which was higher than that of carbapenems resistant to P. aeruginosa or K. pneumonia (6).
The rapid increase and worldwide spread of CRAB infection have become a serious public health concern, as it is difficult to treat and associated with high mortality and morbidity. In 2019, the U.S. Centers for Disease Control (CDC) designated CRAB an urgent threat to public health, which caused approximately 8500 hospital-associated infections and 700 deaths in 2017 (7). It is important to note that CRAB infections can be disastrous for ICU patients. Among these patients, underlying diseases, immune deficiency and invasive operations all contributed to the higher risk of mortality, and carbapenem resistance exacerbates this process. In a retrospective study from Seoul National University Children's Hospital, carbapenem insensitivity was an important independent risk factor for death from A. baumannii bacteremia in PICU patients (11). Early recognition of CRAB infection and mortality determinants is necessary for effective intervention strategies and antibiotic treatment. Although numerous clinical studies regarding CRAB infections have been published, most of them have focused on patients with CRAB bacteremia (11, 12) or ventilator-associated pneumonia (13), and there is a scarcity of studies that investigate CRAB infections in critically ill children. Therefore, this study sought insights into CRAB infection in critically ill children. The aims of this study were (i) to determine the prevalence and potential risk factors for CRAB infection and (ii) to determine the outcomes of CRAB infection and its associated determinants among critically ill children.
This retrospective case-control study was performed at the PICU of the Shanghai Children's Medical Center (SCMC) in China and was approved by the Ethics Committee of Shanghai Children's Medical Center (SCMCIRB-K2023089-1). This hospital is one of the largest pediatric teaching hospitals affiliated with Shanghai Jiaotong University in China, and the PICU is a unit that treats critically ill children with life-threatening conditions such as respiratory failure, heart failure, respiratory cardiac arrest, shock, convulsions, and severe central nervous system depression. All episodes of A. baumannii infection that occurred in the PICU were identified through the Hospital Information System (HIS) between January 1st, 2016 and December 31st, 2021. Demographic, clinical, microbiological and outcome data for all episodes were collected. Only the first episode for each patient was included in our cohort, and cases with incomplete medical records were excluded. During the period of this study, patients who developed healthcare-associated infections with A. baumannii isolates showing resistance to carbapenems were assigned to a case group while those with isolates sensitive to carbapenems were assigned to a control group.
A. baumannii infection was defined as the isolation of A. baumannii in at least one culture specimen in patients with concomitant signs and symptoms of infection. All A. baumannii infections in this study followed the diagnostic criteria for nosocomial infections (14), combined with specific clinical manifestations and laboratory test results. The outcome was measured as the crude mortality after a positive culture. For statistical purposes, patients who were discharged as terminally ill and refused treatment were considered to have died at the time of hospital discharge.
The demographic and clinical data of enrolled patients were extracted from the hospital information system as follows: age, gender, the circumstances of birth (birth weight, prematurity, vaginal delivery, intrapartum asphyxia, firstborn, breastfeeding), clinical symptoms (fever, icterus, vomiting, diarrhea, thoracodynia, polypnea, cough), comorbidities/underlying disease, clinical outcome (in-hospital mortality, length of hospitalization), coinfection with other bacteria (S.aureus, E. coli, K. pneumoniae, P. aeruginosa, S.maltophila) or virus (cytomegalovirus, parainfluenza virus, respiratory syncytial virus, Epstein-Barr virus, influenza virus), medication and intervention therapy within 30 days (parenteral nutrition, central venous/urinary/drainage catheterization, tracheal/gastric intubation, bone marrow aspiration, lumbar puncture glucocorticoid therapy, blood transfusion and antibiotic exposure history), surgery experience within 3 months before infection.
In addition, laboratory tests for C-reactive protein (CRP), white blood cell count (WBC), platelet count (PLT), neutrophil/lymphocyte percentage (%), hemoglobin, albumin, serum urea, serum creatinine, alanine aminotransferase (ALT) and aspartate aminotransferase (AST), were also extracted from the computerized microbiology laboratory databases.
Bacterial species were firstly identified as A. baumannii complex by mass spectrometry (Vitek MS, Biomérieux, France), then were further confirmed by identifying the presence of the blaOXA-51-like carbapenemase gene that is specific to the A. baumannii species. Identification of the causative viral pathogens was by multiplex nucleic acid amplification or the immunological assays for rapid detection of antiviral antibodies or viral antigens.
Antimicrobial susceptibility testing of isolates was performed using a VITEK-2 compact system (bioMérieux, Marcy-l'Étoile, France) with broth microdilution and disk diffusion methods. Results were interpreted in accordance with the 2020 CLSI M100-S30 guidelines (15). The following drugs were tested: amikacin, gentamicin, tobramycin, meropenem, imipenem, ceftazidime, cefepime, cefoperazone/sulbactam, ciprofloxacin, levofloxacin, piperacillin, piperacillin/tazobactam and colistin. For the analysis, A. baumannii isolates that were resistant to one or more carbapenems (ertapenem, imipenem, or meropenem) were considered as carbapenem-resistant A. baumannii. In addition, A. baumannii isolates that were resistant to three or more types of antibacterial drugs simultaneously were identified as multidrug-resistant A. baumannii (MDR-AB), and which were resistant to all antibiotics except tigecycline and/or polymyxins were called extensively drug-resistant A. baumannii (XDR-AB) (16).
Characteristics of patients are presented with absolute frequencies and percentages for categorical variables, and with median and interquartile range (IQR) for continuous variables. To assess differences, the chi-square test or Fisher's exact test was used to compare the categorical variables; while the Student's t-test or Mann–Whitney rank sum test was used to analyze continuous variables. All tests were two-sided, and a p < 0.05 was considered as statistically significant. Univariate and multivariate analyses using logistic regression were performed to identify predictors of mortality and risk factors for CRAB infections. All significant variables in univariable comparisons were subsequently selected for inclusion in multivariate analysis. The Bonferroni correction was used to avoid alpha one error for multiple comparisons, and the statistical adjusted significance was accepted at the adjusted p < 0.0006. Results were presented as odds ratios (ORs), 95% confidence intervals (CIs), and p-values. All statistical analyses were performed with SPSS 26.0 software (IBM Corporation).
Through the hospital information system, a total of 372 children admitted to PICU in our hospital were diagnosed with A. baumannii infection between January 2016 and December 2021. The overall prevalence of A. baumannii infection in the PICU was 9.4% (372/3,974). Among them, the percentage of CRAB reached 78.0% (290/372), which was much higher than that in the whole hospital (54.3%, 660/1,215) over the 6 years. As shown in Figure 1, the proportions of CRAB in the PICU showed decreasing but fluctuating trends, from 89.7% in 2016 to 26.6% in 2021, which is consistent with the trend for CRAB in the hospital.
Figure 1. Comparison of trends in carbapenem-resistant A. baumannii in the PICU and whole hospital wards from 2016 to 2021.
The antimicrobial resistance profiles of the clinical isolates of A. baumannii are summarized in Table 1. Of the 372 isolated strains, 301 (80.9%) were MDR-AB and 26 (7.0%) were XDR-AB. Generally, all A. baumannii isolates showed moderate or high rates of resistance to all other antibiotics tested except colistin. Resistance to piperacillin, ceftazidime, ciprofloxacin and cefoperazone-sulbactam displayed decreasing trends from 2016 to 2021 (Figure 2). Specifically, piperacillin (85.5%) was found to be the most resistant antibiotic in this study, followed by cephalosporins (78.2%), carbapenems (78.0%), ciprofloxacin (76.9%), gentamicin (76.3%) and cefoperazone/sulbactam (76.1%). In contrast, amikacin exhibited the highest antimicrobial activity against these strains.
Figure 2. Resistance profile (%) of A. baumannii for six main antimicrobials in the PICU from 2016 to 2021.
More importantly, antibiotic resistance was even more severe among the CRAB isolates. Except for amikacin, more than 50% of CRAB strains were resistant to other antibiotics tested and a drug resistance rate of >90% was prevalent for piperacillin, ceftazidime, cefepime, ciprofloxacin, gentamicin, piperacillin/tazobactam and cefoperazone/sulbactam. Compared with CRAB, CSAB exhibited a significantly lower resistance rate to all antibiotics and the rate of resistance to piperacillin (34.1%) was the highest.
Briefly, the median age of these pediatric patients was 7.2 months, with a male to female ratio of 1.2. Among them, 203(54.6%) were firstborn and 190(51.1%) experienced breastfeeding. Moreover, 179 (48.1%) were born by vaginal delivery and birth weight > 2,500 grams was found in 290 (78.0%).
Polypnea (73.7%), fever (68.5%) and cough (66.4%) were the most common clinical symptoms and respiratory tract infection was the main form of infection. More than 80% of patients suffer from severe underlying diseases or complications, with severe pneumonia being the most common (81.5%). Hypoproteinemia and anemia occurred in 269 (72.3%) and 259 (69.6%) episodes in the progress of the disease, respectively. Additionally, it was found that co-infection of A. baumannii and other pathogens (bacteria or viruses) was highly prevalent, occurring in 78.2% of cases.
In the course of treatment, the most frequently used antibiotics were the third-generation cephalosporins (71.2%), followed by carbapenems (55.9%) and glycopeptides (33.5%). Furthermore, 237 (63.7%) cases received hormone therapy, while 299 (80.4%) cases underwent at least one blood transfusion within 30 days before infection. In addition, 338(90.9%) children were exposed to some sort of invasive procedures in a month prior to A. baumannii isolation, including 79.8% gastric intubation, 79.3% endotracheal intubation, 54.0% indwelling urinary catheters, 24.7% central venous catheters, and 9.7% drainage tubes. Bone marrow and lumbar punctures were performed in 10.5% and 16.1% of patients, respectively. Nearly half (44.4%) of the patients had undergone surgery within 3 months prior to infection.
In order to determine the risk factors for CRAB infection in critically ill children, a retrospective case-control analysis was conducted, including 290 children with CRAB infection and 82 children with CSAB infection.
The demographic and clinical characteristics of children with CRAB and CSAB infections are summarized in Table 2. On univariate analysis, children with CRAB infection tend to show more symptoms of fever (72.1 vs. 56.1%, p = 0.006) and polypnea (77.2 vs. 61.0%, p = 0.003), had a higher proportion of hemoglobin <110 g/L (91.7 vs. 70.7%, p < 0.0006), neutrophile>70% (37.6 vs. 22.0%, p = 0.008) and have lower proportion of vomiting (21.0 vs. 34.1, p = 0.014), neutrophile <50% (31.0 vs. 50.0%, p = 0.002), ALT <13 U/L(1.7 vs. 8.5%, p = 0.002) than those with CSAB infection. However, after the Bonferroni correction, only hemoglobin < 110 g/L remained significantly associated with CRAB infection (p < 0.0006).
Table 2. Baseline and clinical characteristics of 372 hospitalized children with A.baumannii infections.
As shown in Table 2, higher rates of severe pneumonia (87.9 vs. 58.5%, p < 0.0006), anemia (74.5 vs. 52.4%, p < 0.0006) and hypoproteinemia (77.6 vs. 53.7%, p < 0.0006) were observed in patients with CRAB infection. Furthermore, compared with CSAB-infected patients, more patients with CRAB infection experienced invasive procedures including tracheal intubation (87.6 vs. 50.0%, p < 0.0006), urinary catheterization (60.3 vs. 31.7%, p < 0.0006) and gastric intubation (87.6 vs. 50.0, p < 0.0006). In addition, more patients suffered from CRAB infection received a blood transfusion (87.2 vs. 56.1%, p < 0.0006) and specific antibiotic treatments before A. baumannii isolation, including carbapenems (63.8 vs. 28.0%, p < 0.0006), and glycopeptides (49.7 vs. 23.2%, p < 0.0006).
To further evaluate the independent predictors of CRAB acquisition in pediatric patients, a multivariate logistic regression analysis was performed (Table 3). Of note, receiving invasive operation (OR = 9.412, p = 0.001), was found to be the best independent risk factor for the development of CRAB infection. Hemoglobin < 110g/L (OR = 3.049, p = 0.005), severe pneumonia (OR = 3.235, p = 0.001), previous exposure to carbapenems (OR = 2.543, p = 0.003) and presence of gastric intubation within 1 month before infection (OR = 2.478, p = 0.026) were also significantly associated with CRAB acquisition.
Table 3. Risk factors for carbapenem-resistant A. baumannii infection with multivariate logistic regression analysis.
Of the 372 patients with A. baumannii infection, 115 patients (30.9%) died in the hospital. Univariate analyses of risk factors associated with mortality are shown in Table 4. Children who had a poor outcome were older in median age (p < 0.0006), had a higher proportion of icterus (p < 0.0006), CRP>8 mg/L (p < 0.0006), lymphocyte < 20% (p < 0.0006), PLT < 100 x 109/L (p < 0.0006) and AST>46U/L (p < 0.0006). Moreover, higher rates of septic shock (p < 0.0006) and bone marrow puncture (p < 0.0006) were more frequent in non-survivors. Besides, previous exposure to glycopeptides (p = 0.016), aminoglycosides (p = 0.019) and antifungal drugs (p = 0.001) in non-survivors was higher than those in survivors. However, there was no significant association between previous antibiotic exposure and clinical outcome after the Bonferroni correction.
Table 4. Univariate analysis for factors associated with mortality of children with A. baumannii infection.
In the final multivariable model, septic shock (OR = 2.992, p = 0.001), AST>46U/L (OR = 2.015, p = 0.005), bone marrow aspiration (OR = 2.704, p = 0.008), lymphocyte <20 % (OR = 1.992, p = 0.006) and age (OR = 1.094, p = 0.002) at infection onset were independent risk factors for mortality due to A. baumanni infection in the PICU (Table 5). However, mortality was not higher in patients with CRAB infection than in those with CSAB infection.
Table 5. Risk factors for mortality of Acinetobacter baumannii infection with multivariate logistic regression analysis.
CRAB infections are becoming one of the most cause of public healthcare threats worldwide (17) and carry an increased risk of infection-associated death for children. In our study, the rate of CRAB among A. baumannii infection in the PICU has reached as high as 78.0%, which is higher than in most countries in Europe (18, 19) and North America (20). A study of surveillance data from European Union countries found that the population-weighted mean proportion of carbapenem-non-susceptible Acinetobacter spp. isolates in ICUs reached 54.0% [95% CI 47.6–60.3%] (19). Data from 70 US medical centers in 2018–2020 showed the proportions of carbapenem-non-susceptible A. baumannii in ICUs and non-ICUs were 29.9% and 27.8%, respectively (20). Consequently, A. baumannii infection in the ICU should draw more attention in consideration of high carbapenem resistance rate. In addition to carbapenems resistance, A. baumannii showed high rates of resistance to cephalosporins, β-Lactam inhibitors, penicillins, quinolones, aminoglycosides and 81.0% exhibited multidrug resistance phenotypes. Moreover, CRAB showed significantly higher rates of resistance to all tested antimicrobials than CSAB, with the exception of colistin. High-resistance rates in CRAB will inevitably complicate the treatment of severe infections in critically ill patients and should accelerate efforts toward effective antimicrobial stewardship and infection control strategies.
We also observed a significant reduction in the proportion of CRAB from 2016 to 2021. The decrease in the prevalence of CRAB after 2018 may be attributable: (1) to strengthen environmental monitoring and disinfection of wards and offices, as well as staff disinfection during the COVID-19 pandemic; (2) Both medical staff and patients strictly complied with medical guidance and took good personal protection to reduce the risk of bacterial transmission during this time period; (3) Due to quarantine restrictions, fewer patients were admitted to the PICU and are less likely to develop nosocomial CRAB infections.
The present study identified several independent risk factors for developing CRAB infection in critically ill children, including invasive operation, gastric intubation, prior carbapenems exposure, severe pneumonia and low hemoglobin level. Increasing evidence indicates that prior invasive procedures, including the presence of mechanical ventilation, endotracheal intubation, central venous or umbilical artery catheterization, and abdominal drainage catheterization are associated with a higher risk of CRAB infections (13, 22–24). Invasive procedures can destroy intact skin, which may create opportunities for CRAB strains to colonize the skin or even enter internal tissues. Furthermore, CRAB strains can exhibit the natural ability to form biofilms on the catheter, causing infection by migrating into the epithelial cells of the internal tissue (21). Consistent with many other studies, we identified a series of invasive procedures, including tracheal intubation (p < 0.001), urinary catheterization (p < 0.001), gastric intubation (p < 0.001), drainage intubation (p = 0.037) and surgery (p = 0.018) were associated with CRAB infection in univariable analysis. However, only the use of gastric intubation remained as an independent risk factor. Clinically, most patients with gastric intubation have poor nutrition and gastrointestinal dysfunction, which increases the risk of intestinal bacterial translocation, including CRAB. Therefore, our results emphasize the importance of the strict application of catheterization and nursing in children.
Antibiotic exposure remains one of the most important risk factors for the acquisition of CRAB. A study from Thailand found that prior use of cephalosporins increased the risk of CRAB ventilator-associated pneumonia in neonates (13). Previous exposure to aminoglycosides or carbapenems has long been considered as a high-risk factor contributing to CRAB infection in hospitalized patients (25–27). As identified in previous studies, prior carbapenems exposure is a critical risk factor for developing CRAB infections in this study. In fact, the increased number of infections due to multidrug-resistant Acinetobacter in the PICU requires empirical broad-spectrum antimicrobials, especially carbapenems. Long-term and high frequency use of such antibiotics makes A. baumannii undergo gene mutation or acquire new resistance genes to survive under the pressure of antibiotics, resulting in changes in the resistance characteristics of bacteria (28). This phenomenon was mainly observed when the treatment had occurred between 6 months (ceftazidime) and 9 months (imipenem and levofloxacin) prior (29). Considering these factors, hospitals should strengthen antibiotic management and pay more attention to patients with CSAB infection who have prior exposure to carbapenems.
Risk factors for nosocomial acquisition of antibiotic-resistant microorganisms include not only prior exposure to antibiotics, but also variables related to host defense and infection control measures. Previous reports have shown the disease severity [Acute Physiology and Chronic Health Evaluation (APACHE) II score] was a significant risk factor for the acquisition of CRAB (30, 31). Compatible with these reports, our study revealed that patients who acquired CRAB infection often had severe pneumonia and low hemoglobin level. They had a weaker immune system and were more vulnerable to invasive maneuvers and multi-drug resistant bacteria. The ICU environment with high CRAB colonization and a crowd of ventilated patients may serve as a reservoir for cross-transmission and provoke exogenous acquisition of CRAB.
To explore the possible influence of CRAB infection on the outcome of critically ill children, their demographic and clinical characteristics were systematically evaluated. In our study, the crude in-hospital mortality of the 372 patients with A. baumannii infection was 30.9% (115/372), which was significantly higher than the 30-day all-cause mortality rate (22.4%) of patients with A. baumannii complex bacteraemia reported in the Chinese Antimicrobial Resistance Surveillance of Nosocomial Infections (CARES) Network (22). This is partly because the patient has serious underlying diseases or complications and/or inappropriate treatment.
Predictors of mortality have been extensively studied in patients with A. baumannii bacteremia. A multicenter retrospective study in Taiwan has revealed that carbapenem resistance conferred enhanced risks for mortality in patients with A. baumannii complex bacteremia (32). According to another national prospective study from CARES, carbapenem resistance was also reported as an independent variable for 30-day all-cause mortality in patients with A. baumannii bacteremia (23). Interestingly, a retrospective study in the United States found the mortality was significantly higher in patients with non-CSAB bacteremia than in those with CSAB bacteremia, but they no longer found a significant association between CRAB and increased risk of death after adjusting for potential confounders (33). In our study, we did not observe that CRAB was associated with an apparent unfavorable impact on morbidity and mortality in A. baumannii infection. Currently, there is ongoing controversy as to whether carbapenem resistance results in an increased risk of mortality in patients infected with A. baumannii (34). These discrepancies may be attributed in part to differences in the study population or the sample size, or an overall lack of statistical power.
In addition to carbapenem resistance, disease severity (such as high APACHE II scores and shock state) has also been reported in many studies to be independently associated with poor outcomes in patients with A. baumannii bacteremia (35, 36). Consistent with these studies, we found septic shock, AST > 46 U/L, lymphocyte < 20 %, and age were independently associated with poor prognosis in A. baumannii infection. Septic shock is a life-threatening condition that results from the combined effects of bacteria and the host's chemical mediators of inflammation (37). It is well known that mortality increases with each hour that appropriate antimicrobial therapy is delayed in patients with septic shock (38). Serum levels of aspartate aminotransferases (AST) has been regarded as an important marker of liver injury. Its serum concentrations are highly variable and are affected by a myriad of factors, including liver function, dietary protein intake, metabolic rate, medication status, and hydration status (39), etc. These factors often occur in ICU patients, leading to their immune deficiency, which supports that the patient's physical condition is closely related to their survival. Lymphocytes are the primary cells of the immune system including T cells, B cells and natural killer cells. These cells are responsible for producing cytotoxic T cells or antibodies that directly eliminating invading pathogens (viruses, bacteria), and regulate the immune response (40). Low concentrations of lymphocytes suppressed natural and adaptive immunity, further played an adverse role in the disease progression. An International Study from China and Germany indicated lymphocytes (%) was one of five clinical predictors of the mortality in patients with COVID-19 infection (41). Previous studies have reported age (>65 years) as an independent risk factor for A. baumannii infection-related deaths in hospitalized patients and deaths increased by a factor of 1.04 with every additional year of age (42). Similar to this result, our founding suggests that age contributing to A. baumannii infection-related deaths in critically ill children. This may be due to their low immunity and long-term underlying diseases without effective treatment and control.
Bone marrow aspiration is a valuable procedure commonly utilized for evaluation of hematologic abnormalities and suspected infection in patients with fever of unknown origin (43). This invasive procedure also destroy intact skin and probably create conditions for A. baumannii to colonize the skin and even enter the bone marrow, leading to severe bone marrow infections that threaten the patient's life. A 51-year-old man with chronic lymphocytic leukemia developed acute osteomyelitis and bloodstream infection caused by Staphylococcus aureus after bone marrow aspiration and renal aspiration biopsy (44). Therefore, the strict application and care of bone marrow aspiration in children are closely related to the prognosis of patients.
This study focused on the clinical characteristics of A. baumannii infection in critically ill children in China. The main limitations were its single-center retrospective nature and the relatively small sample size, which may affect the power of the analysis to identify significant factors. Furthermore, we could not perform the matching process, which would be useful in limiting selection bias. This was mainly due to the relatively small number of patients in the control group and the fact that both groups of patients had similar underlying diseases. Finally, there is no data exploring the genotype and/or resistance mechanism of CRAB isolates, which would shed new light on the spread of CRAB.
In conclusion, this study highlights high rates of CRAB and MDRAB in critically ill children with A. baumannii infection. Children with invasive operation, gastric intubation, prior carbapenems exposure, severe pneumonia and low hemoglobin level were more likely to develop CRAB infections. Further, the severity of illness and bone marrow aspiration were independently associated with the mortality due to A. baumanni infection among critically ill children. Based on these above risk factors, the combined application of targeted interventions and rational antibiotic therapy could effectively control the emergence and spread of CRAB in the ICU. Of course, these control measures are crucial and must be extended to other healthcare settings for the eradication of CRAB, which will ultimately improve the outcomes for A. baumannii infection in the world.
The original contributions presented in the study are included in the article/Supplementary material, further inquiries can be directed to the corresponding authors.
The studies involving humans were approved by the Ethics Committee of Shanghai Children's Medical Center. The studies were conducted in accordance with the local legislation and institutional requirements. The Ethics Committee/Institutional Review Board waived the requirement of written informed consent for participation from the participants or the participants' legal guardians/next of kin because this was a retrospective observational study, and it did not cause any disruption to patients.
YZ: Data curation, Formal analysis, Investigation, Software, Writing—review & editing. GX: Conceptualization, Formal analysis, Investigation, Methodology, Writing—review & editing. FM: Conceptualization, Formal analysis, Investigation, Methodology, Writing—review & editing. WH: Data curation, Investigation, Writing—review & editing. HW: Methodology, Project administration, Supervision, Writing—review & editing. XW: Project administration, Supervision, Writing—original draft, Writing—review & editing, Methodology, Funding acquisition.
The author(s) declare financial support was received for the research, authorship, and/or publication of this article. This study was supported by the National Natural Science Foundation of China (grant 81301392) and the Training Program for Clinical Medical Young Talents in Shanghai (HYWJ201605).
The authors declare that the research was conducted in the absence of any commercial or financial relationships that could be construed as a potential conflict of interest.
All claims expressed in this article are solely those of the authors and do not necessarily represent those of their affiliated organizations, or those of the publisher, the editors and the reviewers. Any product that may be evaluated in this article, or claim that may be made by its manufacturer, is not guaranteed or endorsed by the publisher.
The Supplementary Material for this article can be found online at: https://www.frontiersin.org/articles/10.3389/fpubh.2023.1282413/full#supplementary-material
1. Joly-Guillou ML. Clinical impact and pathogenicity of Acinetobacter. Clin Microbiol Infect. (2005) 11:868–73. doi: 10.1111/j.1469-0691.2005.01227.x
2. Munoz-Price LS, Weinstein RA. Acinetobacter infection. N Engl J Med. (2008) 358:1271–81. doi: 10.1056/NEJMra070741
3. Falagas ME, Karveli EA, Siempos II, Vardakas KZ. Acinetobacter infections: a growing threat for critically ill patients. Epidemiol Infect. (2008) 136:1009–19. doi: 10.1017/S0950268807009478
4. Uwingabiye J, Frikh M, Lemnouer A, Bssaibis F, Belefquih B, Maleb A, et al. Acinetobacter infections prevalence and frequency of the antibiotics resistance: comparative study of intensive care units versus other hospital units. Pan Afr Med J. (2016) 23:191. doi: 10.11604/pamj.2016.23.191.7915
5. Fu P, Xu H, Jing C, Deng J, Wang H, Hua C, et al. Bacterial epidemiology and antimicrobial resistance profiles in children reported by the ISPED program in China, 2016 to 2020. Microbiol Spectr. (2021) 9:e0028321. doi: 10.1128/Spectrum.00283-21
6. European Antimicrobial Resistance Surveillance Network. Surveillance of Antimicrobial Resistance in Europe. Annual Report of the European Antimicrobial Resistance Surveillance Network (EARS-Net). (2020). Available online at: https://www.ecdc.europa.eu/sites/default/files/documents/AER-EARS-Net-2020.pdf (accessed January 15, 2021).
7. Centers for Disease Control and Prevention. Antibiotic Resistant Threats in the United States 2019. (2019). Available online at: https://www.cdc.gov/drugresistance/pdf/threats-report/2019-ar-threats-report-508.pdf (accessed January 15, 2020).
8. Peleg AY, Seifert H, Paterson DL. Acinetobacter baumannii: emergence of a successful pathogen. Clin Microbiol Rev. (2008) 21:538–82. doi: 10.1128/CMR.00058-07
9. China Antimicrobial Surveillance Network. (2023). Available online at: http://www.chinets.com/Data/AntibioticDrugFast (accessed January 15, 2023).
10. Logan LK, Gandra S, Trett A, Weinstein RA, Laxminarayan R. Acinetobacter baumannii resistance trends in children in the United States, 1999-2012. J Pediatric Infect Dis Soc. (2019) 8:136–42. doi: 10.1093/jpids/piy018
11. Choe YJ, Lee HJ, Choi EH. Risk factors for mortality in children with acinetobacter baumannii bacteremia in South Korea: the role of carbapenem resistance. Microb Drug Resist. (2019) 25:1210–8. doi: 10.1089/mdr.2018.0465
12. Lee HY, Hsu SY, Hsu JF, Chen CL, Wang YH, Chiu CH. Risk factors and molecular epidemiology of Acinetobacter baumannii bacteremia in neonates. J Microbiol Immunol Infect. (2018) 51:367–76. doi: 10.1016/j.jmii.2017.07.013
13. Thatrimontrichai A, Techato C, Dissaneevate S, Janjindamai W, Maneenil G, Kritsaneepaiboon S, et al. Risk factors and outcomes of carbapenem-resistant Acinetobacter baumannii ventilator-associated pneumonia in the neonate: A case-case-control study. J Infect Chemother. (2016) 22:444–9. doi: 10.1016/j.jiac.2016.03.013
14. Ministry of Health of the People's Republic of China. Diagnostic criteria for nosocomial infection (for trial implementation). Natl Med J China. (2001) 5:61–7.
15. Clinical and Laboratory Standards Institute (CLSI). Performance Standards for Antimicrobial Susceptibility Testing. Wayne, PA: CLSI Document. (2021).
16. Magiorakos AP, Srinivasan A, Carey RB, Carmeli Y, Falagas ME, Giske CG, et al. Multidrug-resistant, extensively drug-resistant and pandrug-resistant bacteria: an international expert proposal for interim standard definitions for acquired resistance. Clin Microbiol Infect. (2012) 18:268–81. doi: 10.1111/j.1469-0691.2011.03570.x
17. Giamarellou H, Antoniadou A, Kanellakopoulou K. Acinetobacter baumannii: a universal threat to public health? Int J Antimicrob Agents. (2008) 32:106–19. doi: 10.1016/j.ijantimicag.2008.02.013
18. Said D, Willrich N, Ayobami O, Noll I, Eckmanns T, Markwart R. The epidemiology of carbapenem resistance in Acinetobacter baumannii complex in Germany (2014-2018): an analysis of data from the national Antimicrobial Resistance Surveillance system. Antimicrob Resist Infect Control. (2021) 10:45. doi: 10.1186/s13756-021-00909-8
19. Ayobami O, Willrich N, Suwono B, Eckmanns T, Markwart R. The epidemiology of carbapenem-non-susceptible Acinetobacter species in Europe: analysis of EARS-Net data from 2013 to 2017. Antimicrob Resist Infect Control. (2020) 9:89. doi: 10.1186/s13756-020-00750-5
20. Sader HS, Mendes RE, Streit JM, Carvalhaes CG, Castanheira M. Antimicrobial susceptibility of Gram-negative bacteria from intensive care unit and non-intensive care unit patients from United States hospitals (2018-2020). Diagn Microbiol Infect Dis. (2022) 102:115557. doi: 10.1016/j.diagmicrobio.2021.115557
21. Yang CH, Su PW, Moi SH, Chuang LY. Biofilm formation in Acinetobacter Baumannii: genotype-phenotype correlation. Molecules. (2019) 24:1849. doi: 10.3390/molecules24101849
22. Zhou HY, Yuan Z, Du YP. Prior use of four invasive procedures increases the risk of Acinetobacter baumannii nosocomial bacteremia among patients in intensive care units: a systematic review and meta-analysis. Int J Infect Dis. (2014) 22:25–30. doi: 10.1016/j.ijid.2014.01.018
23. Liu Y, Wang Q, Zhao C, Chen H, Li H, Wang H, et al. Prospective multi-center evaluation on risk factors, clinical characteristics and outcomes due to carbapenem resistance in Acinetobacter baumannii complex bacteraemia: experience from the Chinese Antimicrobial Resistance Surveillance of Nosocomial Infections (CARES) Network. J Med Microbiol. (2020) 69:949–59. doi: 10.1099/jmm.0.001222
24. Kim SY, Jung JY, Kang YA, Lim JE, Kim EY, Lee SK, et al. Risk factors for occurrence and 30-day mortality for carbapenem-resistant Acinetobacter baumannii bacteremia in an intensive care unit. J Korean Med Sci. (2012) 27:939–47. doi: 10.3346/jkms.2012.27.8.939
25. Chen Y-H, Chiueh CC, Lee Y-J. Risk factors of carbapenem-resistant Acinetobacter baumannii Infection among Hospitalized Patients. J Exp Clin Med. (2014) 6:143–6. doi: 10.1016/j.jecm.2014.06.003
26. Sultan AM, Seliem WA. Identifying risk factors for healthcare-associated infections caused by carbapenem-resistant Acinetobacter baumannii in a neonatal intensive care unit. Sultan Qaboos Univ Med J. (2018) 18:e75–80. doi: 10.18295/squmj.2018.18.01.012
27. Lee SO, Kim NJ, Choi SH, Hyong Kim T, Chung JW, Woo JH Ryu J, et al. Risk factors for acquisition of imipenem-resistant Acinetobacter baumannii: a case-control study. Antimicrob Agents Chemother. (2004) 48:224–8. doi: 10.1128/AAC.48.3.1070.2004
28. Kyriakidis I, Vasileiou E, Pana ZD, Tragiannidis A. Acinetobacter baumannii antibiotic resistance mechanisms. Pathogens. (2021) 10:373. doi: 10.3390/pathogens10030373
29. Huertas Vaquero M, Asencio Egea MA, Carranza González R, Padilla Serrano A, Conde García MC, Tenias Burillo JM, et al. Association between antibiotic pressure and the risk of colonization/infection by multidrug-resistant Acinetobacter baumannii complex: a time series analysis. Rev Esp Quimioter. (2021) 34:623–30. doi: 10.37201/req/061.2021
30. Wong TH, Tan BH, Ling ML, Song C. Multi-resistant Acinetobacter baumannii on a burns unit–clinical risk factors and prognosis. Burns. (2002) 28:349–57. doi: 10.1016/S0305-4179(02)00012-8
31. Smolyakov R, Borer A, Riesenberg K, Schlaeffer F, Alkan M, Porath A, et al. Nosocomial multi-drug resistant Acinetobacter baumannii bloodstream infection: risk factors and outcome with ampicillin-sulbactam treatment. J Hosp Infect. (2003) 54:32–8. doi: 10.1016/S0195-6701(03)00046-X
32. Wei Z, Zhou S, Zhang Y, Zheng L, Zhao L, Cui Y, et al. Microbiological characteristics and risk factors on prognosis associated with Acinetobacter baumannii bacteremia in general hospital: a single-center retrospective study. Front Microbiol. (2022) 13:1051364. doi: 10.3389/fmicb.2022.1051364
33. Sheng WH, Liao CH, Lauderdale TL, Ko WC, Chen YS, Liu JW, et al. A multicenter study of risk factors and outcome of hospitalized patients with infections due to carbapenem-resistant Acinetobacter baumannii. Int J Infect Dis. (2010) 14:e764–9. doi: 10.1016/j.ijid.2010.02.2254
34. Chopra T, Marchaim D, Awali RA, Krishna A, Johnson P, Tansek R, et al. Epidemiology of bloodstream infections caused by Acinetobacter baumannii and impact of drug resistance to both carbapenems and ampicillin-sulbactam on clinical outcomes. Antimicrob Agents Chemother. (2013) 57:6270–5. doi: 10.1128/AAC.01520-13
35. Esterly JS, Griffith M, Qi C, Malczynski M, Postelnick MJ, Scheetz MH. Impact of carbapenem resistance and receipt of active antimicrobial therapy on clinical outcomes of Acinetobacter baumannii bloodstream infections. Antimicrob Agents Chemother. (2011) 55:4844–9. doi: 10.1128/AAC.01728-10
36. Russo A, Bassetti M, Ceccarelli G, Carannante N, Losito AR, Bartoletti M, et al. Bloodstream infections caused by carbapenem-resistant Acinetobacter baumannii: clinical features, therapy and outcome from a multicenter study. J Infect. (2019) 79:130–8. doi: 10.1016/j.jinf.2019.05.017
37. Goodwin JK, Schaer M. Septic shock. Vet Clin North Am Small Anim Pract. (1989) 19:1239–58. doi: 10.1016/S0195-5616(89)50137-2
38. Dellinger RP, Levy MM, Carlet JM, Bion J, Parker MM, Jaeschke R, et al. Surviving Sepsis Campaign: international guidelines for management of severe sepsis and septic shock: 2008. Intensive Care Med. (2008) 34:17–60. doi: 10.1007/s00134-007-0934-2
39. Sookoian S, Pirola CJ. Liver enzymes, metabolomics and genome-wide association studies: from systems biology to the personalized medicine. World J Gastroenterol. (2015) 21:711–25. doi: 10.3748/wjg.v21.i3.711
40. Larosa DF, Orange JS. 1. Lymphocytes. J Allergy Clin Immunol. (2008) 121:S364–9. doi: 10.1016/j.jaci.2007.06.016
41. Bai T, Zhu X, Zhou X, Grathwohl D, Yang P, Zha Y, et al. Reliable and interpretable mortality prediction with strong foresight in COVID-19 patients: an international study from China and Germany. Front Artif Intell. (2021) 4:672050. doi: 10.3389/frai.2021.672050
42. Alrahmany D, Omar AF, Alreesi A, Harb G, Ghazi IM. Acinetobacter baumannii infection-related mortality in hospitalized patients: risk factors and potential targets for clinical and antimicrobial stewardship interventions. Antibiotics (Basel). (2022) 11:1086. doi: 10.3390/antibiotics11081086
43. Tomasian A, Jennings JW. Bone marrow aspiration and biopsy: techniques and practice implications. Skeletal Radiol. (2022) 51:81–8. doi: 10.1007/s00256-021-03882-w
Keywords: carbapenem-resistant Acinetobacter baumannii, epidemiology, pediatric, risk factor, mortality
Citation: Zhang Y, Xu G, Miao F, Huang W, Wang H and Wang X (2023) Insights into the epidemiology, risk factors, and clinical outcomes of carbapenem-resistant Acinetobacter baumannii infections in critically ill children. Front. Public Health 11:1282413. doi: 10.3389/fpubh.2023.1282413
Received: 24 August 2023; Accepted: 13 November 2023;
Published: 30 November 2023.
Edited by:
Erika Paola Plata Menchaca, View Hospital, QatarReviewed by:
Reza Valadan, Mazandaran University of Medical Sciences, IranCopyright © 2023 Zhang, Xu, Miao, Huang, Wang and Wang. This is an open-access article distributed under the terms of the Creative Commons Attribution License (CC BY). The use, distribution or reproduction in other forums is permitted, provided the original author(s) and the copyright owner(s) are credited and that the original publication in this journal is cited, in accordance with accepted academic practice. No use, distribution or reproduction is permitted which does not comply with these terms.
*Correspondence: Xing Wang, d3hfNTE2NkAxNjMuY29t; Haiying Wang, bGlhbmcxOTk2MTExMUBzaHV0Y20uZWR1LmNu
†These authors have contributed equally to this work
Disclaimer: All claims expressed in this article are solely those of the authors and do not necessarily represent those of their affiliated organizations, or those of the publisher, the editors and the reviewers. Any product that may be evaluated in this article or claim that may be made by its manufacturer is not guaranteed or endorsed by the publisher.
Research integrity at Frontiers
Learn more about the work of our research integrity team to safeguard the quality of each article we publish.