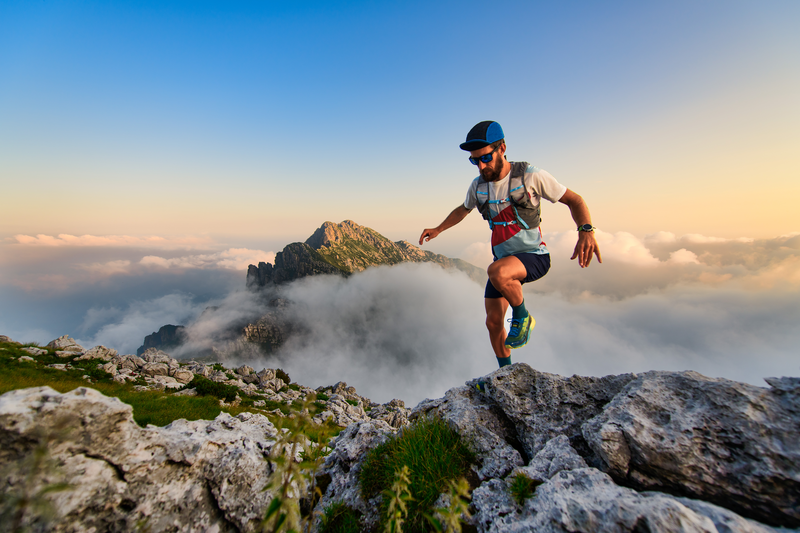
95% of researchers rate our articles as excellent or good
Learn more about the work of our research integrity team to safeguard the quality of each article we publish.
Find out more
ORIGINAL RESEARCH article
Front. Public Health , 13 December 2023
Sec. Infectious Diseases: Epidemiology and Prevention
Volume 11 - 2023 | https://doi.org/10.3389/fpubh.2023.1258981
Objectives: This study aimed to investigate the etiology, clinical features, and outcomes of community-acquired pneumonia (CAP) in adults. Understanding the causative pathogens is essential for effective treatment and prevention.
Design: Between 2016–2018, 518 hospitalized adults with CAP and 241 controls without symptoms were prospectively enrolled. Urine samples were collected for pneumococcal urinary antigen tests and nasopharyngeal swabs for viral and bacterial analysis, combined with routine diagnostic care.
Results: Among the included CAP patients, Streptococcus pneumoniae was the most common pathogen, detected in 28% of patients, followed by Haemophilus influenzae in 16%. Viruses were identified in 28%, and concurrent viruses and bacteria were detected in 15%. There was no difference in mortality, length of stay, or symptoms at hospitalization when comparing patients with bacterial, viral, or mixed etiologies. Among the control subjects without respiratory symptoms, S. pneumoniae, H. influenzae, or Moraxella catarrhalis were detected in 5–7%, and viruses in 7%.
Conclusion: Streptococcus pneumoniae emerged as the predominant cause of CAP, followed closely by viruses and H. influenzae. Intriguingly, symptoms and outcome were similar regardless of etiology. These findings highlight the complexity of this respiratory infection and emphasize the importance of comprehensive diagnostic and treatment strategies.
Clinical Trial Registration: ClinicalTrials.gov, identifier [NCT03606135].
Community-acquired pneumonia (CAP) remains a leading cause of infectious disease-related death (1), despite preventative measures aimed at combating CAP. These measures include pneumococcal vaccination of at-risk groups, yearly vaccinations for seasonal influenza viruses, and, since 2021, SARS-CoV-2 vaccination. In Sweden, the Haemophilus influenzae type b (Hib) vaccine has been included in the child immunization program since the early 1990s and conjugated pneumococcal vaccines (PCV) were introduced in 2009. Interestingly, PCV has led to a reduction of all-cause CAP in children, but a similar decrease has not been shown in older adults (2).
Streptococcus pneumoniae has been regarded as the primary etiology of CAP but other pathogens, such as H. influenzae and atypical bacteria, are also important. In addition, viruses have been recognized as the sole cause of CAP, and combined etiologies have been observed to a higher extent in patients with CAP treated in intensive care units (ICUs) (3, 4).
Understanding the etiology of CAP is crucial for accurate empirical antibiotic treatment, updating treatment guidelines, and future vaccine cost–benefit analyses. Currently, microbiological testing for CAP patients does not identify the etiology of most cases. However, incorporating molecular diagnostic methods can increase the detection rate of both viral and bacterial pathogens (5, 6). This is particularly important in an era of increasing antimicrobial resistance, as it offers the potential to de-escalate antibiotic treatment (6, 7). The aim of this study was to investigate the current etiology of CAP after the introduction of PCV in the child immunization program and to describe the clinical features based on detection of bacterial pathogens, viruses, or both.
From September 2016 to September 2018, the prospective case–control study entitled “Etiology of community-acquired pneumonia in Sweden” (ECAPS) was conducted (ClinicalTrials.gov identifier: NCT03606135). The study was planned to cover two influenza seasons. This current study serves as a sub-analysis of the ECAPS cohort previously described by Hansen et al. (8). Patients aged 18 years or older presenting to the emergency department (ED) at Skåne University Hospital were screened for eligibility and included within 48 h of admission to the ED. The inclusion criteria consisted of having at least two out of ten predefined symptoms of respiratory tract infection, a radiological finding consistent with pneumonia according to the clinical radiologist on duty, and the ability to provide a urine sample. Exclusion criteria were hospitalization or pneumococcal vaccination within the last 30 days and previous enrolment in the study. A control group was continuously recruited throughout the study period at the Department of Orthopedics, within 48 h of admission. Exclusion criteria for the control group were symptoms of a respiratory tract infection within the past 14 days, or pneumococcal vaccination within 30 days prior to hospitalization.
Samples from study patients were collected on clinical indication in routine care and analyzed using standard methods at the local Clinical microbiology laboratory (Laboratory Medicine Skåne). Samples from blood, nasopharynx, pleural fluid, and the lower respiratory tract were included. Urinary samples were analyzed for Legionella pneumophila antigen. Standard-of-care methods are further described in the Supplementary material. As per protocol, a nasopharyngeal (NP) flocked swab and a urine sample were collected within 48 h of admission. Multiplex real-time PCR was conducted on the NP samples to detect 14 viral agents (influenza A H1N1, influenza A H3N2, influenza B, enterovirus, rhinovirus, parechovirus, adenovirus, human metapneumovirus [hMPV], and coronaviruses [OC43, NL63, 229E]), as well as parainfluenza virus 1 to 3 and respiratory syncytial virus (RSV) A/B. In addition, real-time PCR was used to identify 6 bacterial pathogens (S. pneumoniae, H. influenzae, Bordetella pertussis/parapertussis, Chlamydia pneumoniae, and Mycoplasma pneumoniae). The PCR protocols and references can be found in the Supplementary material.
Urine specimens were tested at Pfizer’s Vaccines Research and Development Laboratory (Pearl River, NY). The testing involved a pneumococcal urinary antigen, BinaxNOW S. pneumoniae® (Abbott Diagnostics, Scarborough, ME), as well as two serotype-specific urinary antigen tests, [urine antigen detection; UAD 1 and 2, covering a total of 24 pneumococcal serotypes as described by Hansen et al. (8) (Supplementary material)]. Complete sampling was defined as including a blood culture, nasopharyngeal culture, UAD, BinaxNOW S. pneumoniae® test, and viral/bacterial real-time PCR analysis of 20 pathogens, as per protocol.
Two NP swabs and a urine sample were also collected from the control patient group. One NP swab was cultured for bacteria and the other swab analyzed with real-time PCR for the same 22 respiratory pathogens as the study patients. For PCR analysis every other sample was included to account for seasonal variance (n = 241). The urine samples were analyzed with the same urinary antigens as the study patients, BinaxNOW S. pneumoniae® and UAD 1 and 2.
Symptoms and previous vaccinations were registered by the study nurse through patient interviews. Auscultatory findings and medical history were collected from the patient’s chart.
The pneumonia severity index (PSI) was calculated upon study inclusion and used as a marker of severity. This continuous score is divided into 5 sub-classes based on 20 predictors (9), with class IV-V indicating a moderate-to-high risk of mortality. Case fatality rates (CFR) at 30 or 90 days were used to measure mortality.
Statistical analysis was performed using RStudio 4.3.0 and SPSS version 29. For the comparison of proportions, we used either Pearson’s chi-squared test or Fisher’s exact test. Missing data were excluded from the analysis. In group comparisons of continuous parametric data, we employed ANOVA and t-tests, while the Kruskal-Wallis one way analysis of variance and Mann–Whitney U test were used for non-parametric data. A value of p below 0.05 was regarded as statistically significant, and we applied Bonferroni corrections where appropriate.
We included 518 patients in the final analysis (Supplementary Figure S1), with a median age of 73 years (Table 1). Among them, 236 (46%) were females, and a minority of 13 (3%) resided in a nursing home. Fourteen patients (3%) were admitted to the ICU, and the case fatality rates (CFR) at 30 and 90 days were 4% (n = 21) and 8% (n = 43), respectively. Our control group (n = 241), matched for season, had a median age of 64 years, with 124 (52%) being females. Table 1 provides a detailed description of the CAP patient cohort and controls is described by Hansen et al. (8).
One or more potential pathogens were detected by any method in 351 patients (68%). Among these, bacteria were found in 286 (55%), viruses in 144 (28%), and both bacteria and viruses in 79 (15%) patients. The proportions of microbiological findings are illustrated in Figure 1. The most common pathogens were S. pneumoniae (n = 147, 28%) followed by H. influenzae (n = 84, 16%), rhinovirus (9%), and influenza A/B (8%) (Supplementary Table S1). We detected multiple viruses in only two patients, but 79 (55%) of all viral cases also had a bacterial finding, with S. pneumoniae and rhinovirus being the most frequent combination. Thirty-eight patients (7%) had two or more identified bacteria, most commonly S. pneumoniae and H. influenzae. Moraxella catarrhalis was detected in 39 (8%) of CAP patients, of which 11 (28%) were in combination with another bacterium.
Figure 1. Etiology of CAP within the cohort. In (A) the pie chart shows the proportions of microbiological findings in the whole cohort. A minor part (23 individuals only) was not tested for viral pathogens; 2.1% in the group with bacteria only, and 2.3% in the group “no finding”. In (B) the bars in the figure represent bacteria or viruses indicated on the x-axis. Some patients had multiple pathogens detected. The group “Other bacteria” (n = 5) includes Neisseria meningtidis, Fusobacterium nucleatum and Enterococcus faecalis, whereas group labeled as “Other viruses” (n = 18) consists of parainfluenza virus 1 to 3, adenovirus and coronavirus (OC43, NL63, 229E). The group “Enterobacterales / P. aeruginosa” (n = 10) includes Escherichia coli, Proteus Mirabilis, Enterobacter cloacae, Klebsiella pneumoniae and Pseudomonas aeruginosa. The group “Other streptococci” (n = 11) includes β-hemolytic streptococci and Streptococcus anginosus.
If we only consider cases where complete sampling was performed (n = 370, 71%) S. pneumoniae was detected in 30% (n = 113), H. influenzae in 17% (n = 63), M. catarrhalis in 9% (n = 35), and M. pneumoniae in 6% (n = 21). Co-detections are listed in Supplementary Table S2, and the detection in relation to the diagnostic method is shown in Supplementary Tables S3, S4.
The incidence of S. pneumoniae peaked during the winter seasons, as did M. pneumoniae. However, H. influenzae was not detected with the same seasonal variance. Influenza A dominated the winter season 2016–2017, and influenza B was dominant in 2017–2018. Rhino/enteroviruses were the only viruses detected during the summer periods (Supplementary Figure S2).
In contrast to patients with CAP, a bacterium was detected in 20% (47/241) of our controls with an equal distribution of S. pneumoniae, H. influenzae and M. catarrhalis. The carriage rate of viruses was only 7% (17/241), and rhinovirus was the most common species (Table 2).
Viruses were a significantly more common finding in male patients, with viruses detected in 35% (97/277) either as a single finding or in combination with bacteria, compared to 22% (47/218) in female patients (p = 0.002). In cases where no viruses were detected, males and females were equally represented. Viral detection increased with age, while single bacterial findings were more common in younger patients. Among CAP-patients under 50 years of age, M. pneumoniae was the most frequently detected bacterial species (27/59, 46%), while other bacterial species were more evenly distributed in older age groups (Table 3). Patients with viruses were more likely to have coronary heart disease and a higher BMI (Table 4).
The case fatality rate (CFR) at 30 (p = 0.8) and 90 (p = 0.075) days as well as the mean length of stay (p = 0.3) did not differ significantly depending on the cause of infection (Table 4). However, men had a significantly higher CFR than women at 30 days (6.4% vs. 1.3%; p = 0.003) but not at 90 days (10.6% vs. 5.5%; p = 0.051). No significant difference was found in the pneumonia severity index (PSI)-score (p > 0.05 in all comparisons) among infections caused by viruses, bacteria, or both (Table 4). CRP levels (mg/L) were significantly higher in patients where bacteria were detected, both with and without concurrent viral detection, compared to the groups with no findings or single viral detection (Supplementary Figure S3).
Streptococcus pneumoniae was identified as the leading cause of CAP, followed by H. influenzae. Rhinovirus was the most prevalent virus and was frequently found in conjunction with bacteria. Establishing an etiology in CAP-patients is challenging. We included samples from routine care as well as per-protocol testing, using a comprehensive range of diagnostic assays including culture, real-time PCR, and urinary antigens. The overall diagnostic yield, encompassing both viral and bacterial pathogens, was 68%, which falls within the range reported in previous studies (38–87%) (6, 10–14).
Regarding S. pneumoniae our results align with other European studies, where 29–42% of hospitalized CAP patients were found to be infected with pneumococci (3, 6, 12–15). These numbers, however, are higher than the detection rates of 8–12% reported in recent American studies (10, 16). It has been suggested that widespread pneumococcal vaccination in adults, along with the decline in cigarette smoking in the US, could be contributing factors to the lower detection rates observed (4). Furthermore, it is worth noting that none of the American studies included upper respiratory samples. Although smoking prevalence is lower in Sweden compared to the US (17, 18), S. pneumoniae was still detected in 127 (25% of our cohort), even after excluding positive NP-samples.
A previous study by Johansson et al. (13) reported a higher prevalence of S. pneumoniae (38%) in adult CAP patients before the introduction of PCV in the Swedish child immunization program, compared to 28% in our study. However, their diagnostic methods involved a higher usage of sputum samples, and they did not have access to UAD, which makes direct comparison challenging.
Haemophilus influenzae was identified in 84 cases (16%) and emerged as the second most detected pathogen. Results from other studies show considerable variation, ranging from almost absent with detection rates ≤5% (11, 12) to recent studies where H. influenzae was identified as the predominant bacterial etiology with detection rates of 16–40% (5, 6). However, it is important to note that earlier studies did not include DNA detection. Both German and Danish studies have observed increased rates of H. influenzae in CAP over the past decade (19, 20). Additionally, an increased carriage rate of non-typeable H. influenzae has been noticed in children following the introduction of PCV13 in the child immunization program (21), and certain strains have exhibited increased invasive potential (22), which may explain this phenomenon. These findings highlight the need for further surveillance as an increased incidence of H. influenzae in CAP may necessitate changes in treatment guidelines (23). The prevalence of M. catarrhalis was similar in both controls and CAP-patients, with rates of 5 and 9%, respectively, as reported by Lieberman et al. (24). These findings suggest that the presence of M. catarrhalis in NP samples should be interpreted with caution.
In the tested patients, viruses were found in 29% of cases. Other studies have reported viral detection rates ranging from 15 to 57%, with rhinovirus or influenza virus being the most frequently identified (6, 10, 11, 14). In our study, rhinovirus and hMPV were detected in 10% (n = 50) and 5% (n = 26) of patients, respectively. It is important to note that all but two of these cases were missed in routine diagnostic testing highlighting the underestimation of viruses in CAP in routine care. Testing for influenza virus and initiating antiviral therapy has been shown to reduce antibiotic usage and improve patient outcomes (25). In the control group, a virus was detected in 7% of cases, with rhinovirus being the most common. This finding could be attributed to asymptomatic carriage or persistence of viral RNA from a previous infection. Previous studies have found rhinovirus in asymptomatic controls, albeit at lower rates (11).
In the present study, 55% of all viral detections were found in combination with bacteria. When comparing single pathogen findings to viral-bacterial co-detection, we found no significant difference in mortality rates. Furthermore, there were no significant differences observed in the length of hospital stay or PSI-score. Previous studies have reported higher proportions of viral-bacterial etiologies in ICU patients (3, 26), but no significant differences in mortality rates (6, 27), and varying results in PSI-score and length of stay (10, 27, 28).
It is known that male sex is a risk factor for CAP (29). However, interestingly, in our investigation males were only predominant in the groups with viral pathogen detection. When only bacteria were identified, both sexes were equally represented. As observed previously (26), single bacterial findings were more common in younger patients, whereas viruses or the absence of any detected pathogen, was more common among older patients. This finding may be explained by a lower threshold for hospitalization of older patients. In our study, symptoms were not a reliable predictor in distinguishing between etiology. All symptoms, except malaise, decreased with age, highlighting the need for increased awareness of CAP in older adults and the possibility of reducing antibiotic treatment with increased sampling in this group. CRP levels were generally high in hospitalized patients with radiographically confirmed CAP, with a median level of 132 mg/L. However, significantly higher levels were observed in patients with bacterial detection compared to those with no finding or single viral detection. Additionally, CRP levels were significantly higher in patients with co-detection of bacteria and viruses compared to those with single viral findings. This indicates that CRP levels below 200 mg/L do not discriminate bacterial pneumonia or superinfection from sole viral infection, and further studies on reliable predictors to support decisions to refrain from antibiotics are needed. Furthermore, we only registered the first recorded CRP value, which means that we do not know whether patients had a higher value later during their hospital stay. However, this addresses the challenge of deciding on empirical treatment in the emergency room.
The absence of a gold standard poses a difficulty in determining the accuracy of diagnostic tests in CAP. However, sampling from the lower respiratory tract has been preferred. In our study, only 12% of the patients had lower respiratory tract samples taken, reflecting the challenge of obtaining high-quality sputum samples prior to antibiotic administration, as reported in other studies (10, 11). We included nasopharyngeal culture, as recommended in Swedish guidelines (23), when sputum was not obtainable. Additionally, PCRs for S. pneumoniae and H. influenzae were included. Several recent studies have shown the diagnostic value of using PCR for diagnosing CAP using NP swabs (5, 30).
To address concerns regarding colonization of the upper respiratory tract, we included a control group. Streptococcus pneumoniae was detected by nasopharyngeal culture or PCR in 4% of the controls, compared to 17% of the study patients. Haemophilus influenzae was relatively more common in the controls, with a prevalence of 6% compared to 16% in patients with CAP. Since PCR was not quantitative, it was challenging to establish a cut-off for excluding individuals with only carriage. However, PCR still detected most cases.
Our study has several strengths, including a well-defined cohort, a high frequency of per-protocol samples, and a continuously enrolled control group covering the entire study period. However, the study also has limitations. Obtaining consent from severely ill patients was more difficult, which may have resulted in a somewhat healthier study population compared to the overall cohort of hospitalized CAP patients. The nasopharyngeal samples for PCR were collected after antibiotic administration, but since PCR can detect dead bacteria, it should not be affected by antibiotic use. Since molecular testing, except for atypical bacteria, only included S. pneumoniae and H. influenzae, these bacteria were more likely to be detected. Additionally, most positive cases for S. pneumoniae were identified through urinary antigens, a method only available for pneumococci and L. pneumophila. For the latter, only 36% were tested, potentially resulting in missed cases. Despite our efforts, we were unable to identify a pathogen in 33% of cases, which could lead to an underestimation of either viral or bacterial agents.
In conclusion, S. pneumoniae was the most common finding, followed by H. influenzae and viruses, symptoms and outcomes were similar regardless of etiology. Routine diagnostics underestimated all findings especially viruses which were particularly common in men. Further research is needed to understand the importance of viruses in CAP. Development and implementation of rapid diagnostic tests for both bacteria and viruses could help in distinguishing between viral and bacterial CAP to avoid over-use of antibiotics. The high numbers of H. influenzae indicates possible shifts in etiology which should be monitored. Finally, our results suggest that improving the coverage of pneumococcal and influenza vaccines for targeted risk groups, as well as the development of new antiviral treatments for respiratory viruses, could potentially decrease the morbidity of CAP.
The original contributions presented in the study are included in the article/Supplementary material, further inquiries can be directed to the corresponding author.
The studies involving humans were approved by Ethical approval was granted by the Lund University regional ethics committee (approval nos: 2016/220 and 2016/340), and written informed consent was obtained from all patients. The studies were conducted in accordance with the local legislation and institutional requirements. Written informed consent for participation was not required from the participants or the participants' legal guardians/next of kin in accordance with the national legislation and institutional requirements.
KH: Conceptualization, Data curation, Formal Analysis, Investigation, Methodology, Project administration, Writing – original draft, Writing – review & editing. LYY: Conceptualization, Data curation, Formal Analysis, Investigation, Methodology, Project administration, Visualization, Writing – original draft, Writing – review & editing. LW: Methodology, Project administration, Resources, Supervision, Writing – review & editing. ER: Data curation, Methodology, Project administration, Writing – review & editing. TG: Data curation, Methodology, Writing – review & editing. AN: Methodology, Supervision, Writing – review & editing. JA: Conceptualization, Funding acquisition, Supervision, Writing – review & editing. KR: Conceptualization, Funding acquisition, Methodology, Resources, Supervision, Writing – review & editing.
The author(s) declare financial support was received for the research, authorship, and/or publication of this article. This work was supported by the Knut and Alice Wallenberg Foundation (KR; 2018.0318), Anna and Edwin Berger Foundation (KR), an unrestricted grant from Pfizer (KR and JA), Swedish Heart Lung Foundation (KR; #20180401, www.hjart-lungfonden.se), the Royal Physiographical Society in Lund (Forssman's Foundation), the Skåne County Council's research and development foundation (KR), and the Swedish Research Council (KR; #2019-01053, www.vr.se). One of the funding sources, Pfizer, was involved in laboratory analysis of UAD and Binax testing on urine samples. None of the funders had any role in study design, data interpretation, or writing of the report. KR reports an unrestricted grant from Pfizer during the conduct of the study outside the submitted work. The remaining authors have nothing to disclose.
We thank Clinical microbiology, Laboratory Medicine Skåne (Lund, Sweden) and particularly Izabella Wettermyr for sample analysis, Anna Söderlund Strand for implementing the viral PCR methods and Ann Cathrine Petersson for implementing bacterial PCR methods. We are also grateful to Birgitta Andersson for technical help and Louise Qvist for data collection, as well as to all patients who participated in the study. The results have been presented as a poster at ECCMID 2023 in Copenhagen, Denmark.
KR reports an unrestricted grant from Pfizer during the conduct of the study outside the submitted work.
The remaining authors declare that the research was conducted in the absence of any commercial or financial relationships that could be construed as a potential conflict of interest.
All claims expressed in this article are solely those of the authors and do not necessarily represent those of their affiliated organizations, or those of the publisher, the editors and the reviewers. Any product that may be evaluated in this article, or claim that may be made by its manufacturer, is not guaranteed or endorsed by the publisher.
The Supplementary material for this article can be found online at: https://www.frontiersin.org/articles/10.3389/fpubh.2023.1258981/full#supplementary-material
1. Troeger, C, Blacker, B, Khalil, IA, Rao, PC, Cao, J, Zimsen, SRM, et al. Estimates of the global, regional, and national morbidity, mortality, and aetiologies of lower respiratory infections in 195 countries, 1990–2016: a systematic analysis for the global burden of disease study 2016. Lancet Infect Dis. (2018) 18:1191–210. doi: 10.1016/S1473-3099(18)30310-4
2. Naucler, P, Henriques-Normark, B, Hedlund, J, Galanis, I, Granath, F, and Örtqvist, Å. The changing epidemiology of community-acquired pneumonia: nationwide register-based study in Sweden. J Intern Med. (2019) 286:689–701. doi: 10.1111/joim.12956
3. Cilloniz, C, Ewig, S, Polverino, E, Marcos, MA, Esquinas, C, Gabarrus, A, et al. Microbial aetiology of community-acquired pneumonia and its relation to severity. Thorax. (2011) 66:340–6. doi: 10.1136/thx.2010.143982
4. Gadsby, NJ, and Musher, DM. The microbial etiology of community-acquired pneumonia in adults: from classical bacteriology to host transcriptional signatures. Clin Microbiol Rev. (2022) 35:e0001522–2. doi: 10.1128/cmr.00015-22
5. Demars, Y, Brahier, T, Rotzinger, DC, Brouillet, R, Jaton, K, Opota, O, et al. Utility of polymerase chain reaction in nasopharyngeal swabs for identifying respiratory Bacteria causing community-acquired pneumonia. Microbiol Spectr. (2022) 10:e0037922. doi: 10.1128/spectrum.00379-22
6. Gadsby, NJ, Russell, CD, McHugh, MP, et al. Comprehensive molecular testing for respiratory pathogens in community-acquired pneumonia. Clin Infect Dis. (2016) 62:817–23. doi: 10.1093/cid/civ1214
7. Murray, CJL, Ikuta, KS, Sharara, F, Swetschinski, L, Robles Aguilar, G, Gray, A, et al. Global burden of bacterial antimicrobial resistance in 2019: a systematic analysis. Lancet. (2022) 399:629–55. doi: 10.1016/S0140-6736(22)02185-7
8. Hansen, K, Rünow, E, Torisson, G, Theilacker, C, Palmborg, A, Pan, K, et al. Radiographically confirmed community-acquired pneumonia in hospitalized adults due to pneumococcal vaccine serotypes in Sweden, 2016–2018—the ECAPS study. Front Public Health. (2023) 11:1086648. doi: 10.3389/fpubh.2023.1086648
9. Fine, MJ, Auble, TE, Yealy, DM, Hanusa, BH, Weissfeld, LA, Singer, DE, et al. A prediction rule to identify low-risk patients with community-acquired pneumonia. N Engl J Med. (1997) 336:243–50. doi: 10.1056/NEJM199701233360402
10. Musher, DM, Roig, IL, Cazares, G, Stager, CE, Logan, N, and Safar, H. Can an etiologic agent be identified in adults who are hospitalized for community-acquired pneumonia: results of a one year study. J Infect. (2013) 67:11–8. doi: 10.1016/j.jinf.2013.03.003
11. Jain, S, Self, WH, Wunderink, RG, Fakhran, S, Balk, R, Bramley, AM, et al. Community-acquired pneumonia requiring hospitalization among U.S. Adults. N Engl J Med. (2015) 373:415–27. doi: 10.1056/NEJMoa1500245
12. Holter, JC, Müller, F, Bjørang, O, Samdal, HH, Marthinsen, JB, Jenum, PA, et al. Etiology of community-acquired pneumonia and diagnostic yields of microbiological methods: a 3-year prospective study in Norway. BMC Infect Dis. (2015) 15:64. doi: 10.1186/s12879-015-0803-5
13. Johansson, N, Kalin, M, Tiveljung-Lindell, A, Giske, CG, and Hedlund, J. Etiology of community-acquired pneumonia: increased microbiological yield with new diagnostic methods. Clin Infect Dis. (2010) 50:202–9. doi: 10.1086/648678
14. Bjarnason, A, Westin, J, Lindh, M, Andersson, LM, Kristinsson, KG, Löve, A, et al. Incidence, etiology, and outcomes of community- acquired pneumonia: a population-based study. Open Forum Infect Dis. (2018) 5:ofy010. doi: 10.1093/ofid/ofy010
15. Torres, A, Menéndez, R, España, PP, Fernández-Villar, JA, Marimón, JM, Cilloniz, C, et al. The evolution and distribution of pneumococcal serotypes in adults hospitalized with community-acquired pneumonia in Spain using a serotype-specific urinary antigen detection test: the CAPA study, 2011–2018. Clin Infect Dis. (2021) 73:1075–85. doi: 10.1093/cid/ciab307
16. Isturiz, R, Grant, L, Gray, S, Alexander-Parrish, R, Jiang, Q, Jodar, L, et al. Expanded analysis of 20 pneumococcal serotypes associated with radiographically confirmed community-acquired pneumonia in hospitalized US adults. Clin Infect Dis. (2021) 73:1216–22. doi: 10.1093/cid/ciab375
17. Public Health Agency of Sweden (Folkhälsomyndigheten). Smoking (2022). Available at https://www.folkhalsomyndigheten.se/livsvillkor-levnadsvanor/andts/utvecklinginom-andts-anvandning-och-ohalsa/anvandning/anvandning-av-tobaks-och-nikotinprodukter/vuxnas-bruk-av-tobaks--och-nikotinprodukter/. Accessed July 7th, 2023
18. Cornelius, ME, Wang, TW, Jamal, A, Loretan, CG, and Neff, LJ. Tobacco product use among adults - United States, 2019. MMWR Morb Mortal Wkly Rep. (2020) 69:1736–42. doi: 10.15585/mmwr.mm6946a4
19. Braeken, DCW, Essig, A, Panning, M, et al. Shift in bacterial etiology from the CAPNETZ cohort in patients with community-acquired pneumonia: data over more than a decade. Infection. (2021) 49:533–7. doi: 10.1007/s15010-021-01605-w
20. Fally, M, Israelsen, S, Anhøj, J, Benfield, T, Tarp, B, Kolte, L, et al. The increasing importance of HaemophilusInfluenzae in community-acquired pneumonia: results from a Danish cohort study. Infect Dis (Lond). (2021) 53:122–30. doi: 10.1080/23744235.2020.1846776
21. Cleary, DW, Devine, VT, Morris, DE, Osman, K, Gladstone, R, Bentley, S, et al. Pneumococcal vaccine impacts on the population genomics of non-typeable Haemophilus influenzae. Microb Genom. (2018) 4:209. doi: 10.1099/mgen.0.000209
22. Månsson, V, Skaare, D, Riesbeck, K, and Resman, F. The spread and clinical impact of ST14CC- PBP3 type IIb/a, a clonal group of non-typeable Haemophilus influenzae with chromosomally mediated β-lactam resistance-a prospective observational study. Clin Microbiol Infect. (2017) 23:209.e1-.e7. doi: 10.1016/j.cmi.2016.11.006
23. Athlin, S, Lidman, C, Lundqvist, A, Naucler, P, Nilsson, AC, Spindler, C, et al. Management of community-acquired pneumonia in immunocompetent adults: updated Swedish guidelines 2017. Infect Dis (Lond). (2018) 50:247–72. doi: 10.1080/23744235.2017.1399316
24. Lieberman, D, Shleyfer, E, Castel, H, Terry, A, Harman-Boehm, I, Delgado, J, et al. Nasopharyngeal versus oropharyngeal sampling for isolation of potential respiratory pathogens in adults. J Clin Microbiol. (2006) 44:525–8. doi: 10.1128/JCM.44.2.525-528.2006
25. Deshpande, A, Klompas, M, Yu, PC, Imrey, PB, Pallotta, AM, Higgins, T, et al. Influenza testing and treatment among patients hospitalized with community-acquired pneumonia. Chest. (2022) 162:543–55. doi: 10.1016/j.chest.2022.01.053
26. Karhu, J, Ala-Kokko, TI, Vuorinen, T, Ohtonen, P, and Syrjälä, H. Lower respiratory tract virus findings in mechanically ventilated patients with severe community-acquired pneumonia. Clin Infect Dis. (2014) 59:62–70. doi: 10.1093/cid/ciu237
27. Huijskens, EGW, Koopmans, M, Palmen, FMH, van Erkel, AJM, Mulder, PGH, and Rossen, JWA. The value of signs and symptoms in differentiating between bacterial, viral and mixed a etiology in patients with community-acquired pneumonia. J Med Microbiol. (2014) 63:441–52. doi: 10.1099/jmm.0.067108-0
28. Johansson, N, Kalin, M, and Hedlund, J. Clinical impact of combined viral and bacterial infection in patients with community-acquired pneumonia. Scand J Infect Dis. (2011) 43:609–15. doi: 10.3109/00365548.2011.570785
29. Corica, B, Tartaglia, F, D’Amico, T, Romiti, GF, and Cangemi, R. Sex and gender differences in community-acquired pneumonia. Intern Emerg Med. (2022) 17:1575–88. doi: 10.1007/s11739-022-02999-7
30. Boix-Palop, L, Obradors, M, Xercavins, M, Picó-Plana, E, Canales, L, Dietl, B, et al. Improvement of pneumococcal pneumonia diagnosis using quantitative real-time PCR targeting lytA in adult patients: a prospective cohort study. Clin Microbiol Infect. (2022) 28:138.e1-.e7. doi: 10.1016/j.cmi.2021.05.049
Keywords: CAP, community acquired pneumonia, ECAPS, Haemophilus influenzae, influenza virus, lower respiratory tract infection, Mycoplasma pneumoniae, Moraxella catarrhalis
Citation: Hansen K, Yamba Yamba L, Wasserstrom L, Rünow E, Göransson T, Nilsson A, Ahl J and Riesbeck K (2023) Exploring the microbial landscape: uncovering the pathogens associated with community-acquired pneumonia in hospitalized patients. Front. Public Health. 11:1258981. doi: 10.3389/fpubh.2023.1258981
Received: 14 July 2023; Accepted: 10 November 2023;
Published: 13 December 2023.
Edited by:
Alessandro Muzzi, GlaxoSmithKline, ItalyReviewed by:
Feroze A. Ganaie, University of Alabama at Birmingham, United StatesCopyright © 2023 Hansen, Yamba Yamba, Wasserstrom, Rünow, Göransson, Nilsson, Ahl and Riesbeck. This is an open-access article distributed under the terms of the Creative Commons Attribution License (CC BY). The use, distribution or reproduction in other forums is permitted, provided the original author(s) and the copyright owner(s) are credited and that the original publication in this journal is cited, in accordance with accepted academic practice. No use, distribution or reproduction is permitted which does not comply with these terms.
*Correspondence: Kristian Riesbeck, a3Jpc3RpYW4ucmllc2JlY2tAbWVkLmx1LnNl
†These authors share first authorship
Disclaimer: All claims expressed in this article are solely those of the authors and do not necessarily represent those of their affiliated organizations, or those of the publisher, the editors and the reviewers. Any product that may be evaluated in this article or claim that may be made by its manufacturer is not guaranteed or endorsed by the publisher.
Research integrity at Frontiers
Learn more about the work of our research integrity team to safeguard the quality of each article we publish.