- 1Chongqing Three Gorges Medical College, Chongqing, China
- 2Chongqing Medical University, Chongqing, China
Type 2 diabetes mellitus (T2DM) accelerates the progression of periodontitis through diverse pathways. Abnormal immune responses, excessive activation of inflammation, increased levels of advanced glycation end products, and oxidative stress have defined roles in the pathophysiological process of T2DM-associated periodontitis. Furthermore, in the periodontium of diabetic individuals, there are high levels of advanced glycation end-products and glucose. Meanwhile, progress in microbiomics has revealed that dysbacteriosis caused by T2DM also contributes to the progression of periodontitis. Lactobacillus, owing to its fine-tuning function in the local microbiota, has sparked tremendous interest in this field. Accumulating research on Lactobacillus has detailed its beneficial role in both diabetes and oral diseases. In this study, we summarize the newly discovered mechanisms underlying Lactobacillus-mediated improvement of T2DM-associated periodontitis and propose the application of Lactobacillus in the clinic.
1 Introduction
Periodontitis is a destructive chronic inflammatory disease characterized by lumen swelling, hemorrhage, auxiliary bone absorption, and loose teeth (1). Type 2 diabetes mellitus (T2DM), one of the most common chronic diseases, is often accompanied by systemic complications and is an independent risk factor for periodontitis. Meanwhile, numerous studies provide evidence of the reciprocal interactions between T2DM and periodontitis. Moderate to severe periodontitis has been reported to increase the risk of T2DM and lead to poor glycemic control in diabetic patients (2, 3). Moreover, the elevation in circulating IL-6, TNF-a, and CRP levels caused by periodontitis contributes to increased systemic inflammation, thus further aggravating insulin resistance in patients with T2DM (4). Correspondingly, diabetes increases the morbidity of periodontitis and accelerates the progression of periodontitis (5). The activation of inflammatory and oxidative stress signaling pathways, increased levels of advanced glycosylation end products (AGEs), and abnormal immune responses have been confirmed to play a role in diabetes-related periodontitis (6). In recent years, with the development of microbiomics, the role of dysbiosis caused by T2DM in periodontal disease has attracted attention. The increase in Porphyromonas gingivalis (P. gingivalis), Prevotella intermedia (P. intermedia), and other harmful bacteria and their metabolites associated with inflammation and insulin resistance in the gingiva causes periodontal tissue damage (7, 8). Therefore, interventions targeting the dysbiosis in the oral flora can improve the conditions of T2DM and periodontitis (9, 10).
Probiotics are “living microorganisms,” as defined by the World Health Organization and the Food and Agriculture Organization. Probiotics live in the human body and exert their beneficial effects (11) by suppressing the growth of pathogenic microorganisms, producing bioactive metabolites, and maintaining the balance of the local microenvironment (12). Among all defined probiotics, Lactobacillus is the most studied commercial probiotic. The first commercial Lactobacillus species was Limosilactobacillus reuteri (L. reuteri) (13). Since then, more strains have become commercially available, and they are heterofermentative Lactobacillus, such as Lactiplantibacillus plantarum (L. plantarum), Lacticaseibacillus rhamnosus (L. rhamnosus), Limosilactobacillus fermentum, Lactobacillus acidophilus (L. acidophilus), and Ligilactobacillus salivarius (L. salivarius) (14). These strains have been widely applied in acute diarrhea, cardiovascular disease (15), genitourinary tract infection (16), cancer (17), food allergy (18), Crohn’s disease (19), pouch inflammation (19), and colitis (20). More recently, Lactobacillus was found to be an immune regulator that activates lymphocytes in the gastrointestinal tract after colonizing the intestinal mucosa (21). Studies have also recognized the role of these strains in alleviating lactose intolerance and decreasing antibiotic-related side effects. Lactobacillus has also been extensively used in oral diseases such as ozostomia and caries due to its antibacterial effect (22). Regarding periodontitis, local application of Lactobacillus improved periodontitis directly by reducing the depth of periodontal pockets, alleviating gingival bleeding, and suppressing alveolar bone resorption (23).
The beneficial roles of Lactobacillus in T2DM have been widely defined. L. rhamnosus GG (LGG) has been shown to reduce the incidence of gestational diabetes (24). Another Lactobacillus species—L. acidophilus has been demonstrated to lower blood glucose in patients with T2DM (25). These results sparked tremendous interest and prompted us to explore the underlying mechanisms. The improvement of glucose metabolism, the removal of excess reactive oxygen species, relief of the inflammatory state, and regulation of the gut microbiota have all been shown to be potential mechanisms by which Lactobacillus improves T2DM, and these mechanisms are also involved in the treatment of periodontitis.
Recent intensive studies have revealed the complex regulatory network through which Lactobacillus impacts T2DM-associated periodontitis. Periodontitis is no longer attributed to an infection by a single or a few bacterial species. In essence, periodontitis is now classified as a dysbiotic disease, which arises as a result of the feed-forward loop involving polymicrobial communities and a dysregulated host inflammatory response. During this process, the beneficial role of Lactobacillus in regulating the immune system, oxidative stress and microbiota, antibacterial activity, and glucose metabolism helps delay the process of periodontitis. These mechanisms also contribute to improving blood glucose control in T2DM. In this article, we review the newly discovered pathogenic mechanisms and applications of Lactobacillus in patients with type 2 diabetic periodontitis; as such, we hope to provide a new and viable option for the clinical treatment of T2DM-associated periodontitis.
2 Related mechanisms
2.1 Reducing inflammation and regulating immunity
2.1.1 Regulating the immune response and cytokines
A possible underlying mechanism by which Lactobacillus improves T2DM-associated periodontitis is by promoting a beneficial host response and reducing adverse changes in periodontal tissue. Studies have shown that the probing pocket depth of patients with periodontal disease was significantly reduced following Lactobacillus treatment, which may be due to the immunomodulation by Lactobacillus that leads to anti-inflammatory effects (26). P. gingivalis induces the synthesis of proinflammatory mediators (such as TNF-α and IL-1β) by activating TLR-4. L. rhamnosus lr-32; L. acidophilus La-5 can adhere to human gingival epithelial cells (GECs) and downregulate the expression of TLR-4, therefore reducing the adhesion of pathogens and their invasion (27). In addition, P. gingivalis coordinates the host response by circumventing the defense mechanism triggered by gingival epithelial cells, thus expanding the wound area in periodontitis patients. The expression of CXCL8, a key factor that regulates the proliferation/migration of epithelial cells, can be upregulated by Lactobacillus (28). Lactobacillus can also accelerate re-epithelialization by upregulating the expression of members of the CXCL8-CXCR1/CXCR2 axis, thereby reducing or reversing the harmful effects of P. gingivalis infection and improving wound healing in periodontitis (29). In addition to CXCL8, Lactobacillus can also regulate re-epithelialization through indirect antagonism by inhibiting pathogen adhesion. Euterin and reutericyclin, derived from L. reuteri, are antibacterial substances that inhibit a wide range of pathogens by inducing oxidative stress in cells and preventing the blinding of peripheral pathogens to host tissue (30).
The balance between activated matrix metalloproteinases (MMPs) and their inhibitors (TIMPs) controls the degree of extracellular matrix (ECM) remodeling (31). During T2DM-associated periodontitis, the imbalance between activated MMP and TIMPs leads to the pathological destruction of the ECM (32, 33). Among MMPs, MMP-8 is the most abundant collagen-soluble MMP, which contributes to tissue destruction and remodeling in patients with periodontitis. Studies have shown that MMP-8 abundance is closely related to the severity of periodontal disease, and Lactobacillus reduces MMP-8 in gingival crevicular fluid (34). Additionally, MMP-9 is related to host defense mechanisms, and studies have shown that MMP-9 levels are increased and TIMP-1 levels are decreased in patients taking LGG.
Periodontitis is a common chronic inflammatory disease that is characterized by disordered glucose metabolism and the cytokines: interleukin-1 (IL-1), interleukin-6 (IL-6), tumor necrosis factor-α (TNF-α), and interleukin-17A (IL-17A) (35). Inflammatory byproducts and bacterial endotoxins and metabolites are the main causes of periodontal damage; of these, IL-6 is important for alveolar bone resorption. Accordingly, T2DM is recognized as a chronic, systemic, and low inflammation state. Experimental evidence from animals and humans shows that inflammation is critical to the induction of insulin resistance in obese individuals. Activated macrophages in adipose tissue are responsible for inflammation. Cytokines associated with blood sugar control include TNF-α, IL-6, and IL-10, which may have insulin receptor substrates (IRSs) that are converted to serine, which leads to insulin resistance. Therefore, the regulation of cytokines (reducing the production of anti-inflammatory cytokines and increasing the production of anti-inflammatory cytokines) is of crucial importance to improving T2DM-associated periodontitis. Many experiments have shown that cytokine levels can be altered by Lactobacillus.
Inflammation is mainly caused by bacterial components such as lipopolysaccharide (LPS), which is the primary component of the Gram-negative endotoxin extracellular membrane (26). Ketones produced by Lactobacillus through the polyunsaturated fatty acid (PUFA) pathway exert anti-inflammatory functions via mitogen-activated protein kinase (MAPK) and NF-κB signaling in LPS-induced macrophages; furthermore, the resulting ketophilic acid produced also suppresses the production of IL-6, IL-1β, and TNF-α (36). Various types of Lactobacillus reduced the severity of T2DM, thereby improving the condition of T2DM-associated periodontitis. Huang et al. confirmed that mixed therapy with L. plantarum K68 and FVF controlled the increase in IL-1β, IL-6, and TNF-α in insulin-resistant mice (37). Lacticaseibacillus casei (L. casei) inhibited macrophage production of TNF-α, reduced the level of TNF-α, IL-1β, and IL-6, and increased short-chain fatty acid (SCFA)-producing intestinal hyperlumaccharide-1 (GLP-1) levels (38). Additionally, further studies showed that other Lactobacillus strains also have similar effects. L. rhamnosus, L. acidophilus, L. brevis, and L. reuteri reduce the expression of TNF-α and increase the level of IL-10 (39). Increased IL-10 can downregulate the expression of proinflammatory cytokines such as IFN-γ and IL-2/IL-1β, thereby preventing T2DM-associated periodontitis. Lactobacillus also inhibits the progression of chronic periodontitis by inhibiting the secretory activity of Th17 lymphocytes, which are responsible for excessive cytokine responses in the pathogenesis of the disease and can lead to adverse changes in periodontal tissues (40). There are also reports that Lactobacillus can reduce the inflammatory state of other diseases.
2.1.2 Maintaining intestinal barrier function
Lactobacillus may also improve T2DM-associated periodontitis by helping to maintain intestinal barrier function. Based on the hypothesis of the leaky epithelium, intestinal microbiome dysregulation leads to increased intestinal permeability, which allows bacterial endotoxins to enter circulation, impair intestinal barrier function, and eventually cause an immune response that damages β cells and may lead to an increase in cytokine secretion, thus causing insulin resistance (41). Previous studies have shown that L. plantarum WCFS1 can induce the expression of genes related to the anti-inflammatory immune response, increase the immune response in the human intestinal tract (42), promote cell growth and proliferation, stimulate TLR2 as a regulator of epithelial integrity, and regulate the expression of epithelial tight junctions to help maintain the environmental balance of the intestine. Similarly, Zo-1 and occludin were repositioned near the tight junction following L. plantarum WCFS1 administration, which affects intestinal barrier function (43). L. rhamnosus GG also reduced intestinal permeability in mice fed a high-fructose diet, which stimulated goblet cell production of mucin and prevented LPS and other pathogens from crossing the intestinal barrier (44).
2.1.3 Disrupting bacterial biofilm
The formation of bacterial biofilms in the oral cavity is considered the main cause of many pathological conditions in the oral cavity, and periodontitis is not an exception. Lactobacillus may inhibit oral biofilm formation and reduce harmful inflammatory immune responses (45). Lactobacillus may also contribute to the regulation of periodontal immune inflammation by altering the composition of bacterial biofilms. The oral colonization of Prevotella melaninogenica (P. melaninogenica) is associated with periodontitis. L. brevis CD2 and L. reuteri inhibit melanin-producing P. melaninogenica biofilms. The possible mechanism is that L. reuteri inhibits the production of bacterial ribonucleotide reductase and has antibacterial effects (46). A high concentration of L. acidophilus CFF significantly inhibited biofilm formation, removed biofilms, and stimulated monocytes/macrophages. A more than 90% reduction in biofilm formation was achieved with the highest concentrations of L. acidophilus WCS and CFF (47).
Whether by regulating cytokines, maintaining intestinal barrier function to reduce inflammation, or removing biofilms and reducing periodontal tissue damage through host responses, we speculate that Lactobacillus has the potential to reduce inflammation and regulate immunity, thus reducing the severity of T2DM-associated periodontitis.
2.2 Regulating oxidative stress
Reactive oxygen species (ROS) are key components of the neutral granulocyte antibacterial library. However, excessive ROS often leads to oxidative stress in periodontal tissue with increasing production of proinflammatory cytokines, such as IL-6 and TNF-α, in gingival epithelial cells that induces pathological changes and leads to the destruction of the structure supporting host teeth and the loss of teeth (48). ROS also increases insulin resistance and impairs the β cell membrane, thereby promoting the occurrence and development of diabetes (49). Therefore, regulating oxidative stress may be an effective way to treat T2DM-associated periodontitis.
Many studies have confirmed that Lactobacillus strains have high antioxidant capacity. The antioxidant mechanism of Lactobacillus is closely related to the removal of reactive oxygen species and the increase in antioxidants (50). The metabolites of L. plantarum, ketoacid, and hyaluronic acid, stimulate the expression of antioxidant-related genes in gingival epithelial cells, inhibit the oxidation process, and prevent inflammation (51). Accordingly, in vivo, L. plantarum increased glutathione peroxidase activity in diabetic rats and upregulated the expression of the peroxisome proliferator-activated receptors-α (PPAR-α) and γ (PPAR-γ) to prevent oxidative stress that damages insulin-secreting cells and protect pancreatic function. L. casei and Lacticaseibacillus paracasei (L. paracasei) also have the ability to scavenge free radicals and antioxidant activity; they can also restore the level of malondialdehyde (MDA), a marker of oxidative stress (52, 53). In addition to the above Lactobacillus strains that reduce oxidative stress, several other Lactobacillus strains also exert antioxidant effects in other ways.
Excessive NO leads to barrier dysfunction, and high levels of NO promote the infiltration of inflammatory cells into periodontal tissues by inducing pathological vascular permeability (54). NO plays a role in periodontal inflammation and bone loss, as has been confirmed in animal models. Relevant evidence shows that Lactobacillus reduces oxidative stress by inhibiting NO production. Lactobacillus reduces the expression of the nitric oxide synthase (iNOS) gene in fat, restores the overall energy balance in animal adipose tissue, and inhibits the inflammatory response (55). Studies also show that L. plantarum reverses intestinal imbalance caused by diabetes, increases intestinal ROS levels, and reduces intestinal iNOS expression induced by diabetes (56). In addition, arginine deaminase, an enzyme that metabolizes arginine into citrulline and ammonia, is present in L. brevis and helps L. brevis extract inhibit NO production by competing with NOS for the same substrate, arginine (57). Harisa et al. (58) demonstrated that in diabetic rats, the level of NO returned to normal after applying oral L. acidophilus, perhaps by reducing oxidative stress; thus, there was an antidiabetic effect.
2.3 Improving glucose metabolism
Another underlying mechanism by which Lactobacillus treats T2DM-associated periodontitis is the regulation of glucose metabolism. A variety of probiotics have been identified to directly control or reverse the elevation of glycosylated hemoglobin and fasting insulin levels. There is a meta-analysis of 12 randomized controlled trials on the role of probiotics in T2DM, 10 of which clearly indicate that Lactobacillus does significantly reduce HbA1c, fasting insulin levels, and HOMA-IR levels in T2DM (59).
2.3.1 Reducing glycogen synthesis and increasing glycogen consumption
Several investigations have shown that Lactobacillus may directly inhibit glucose synthesis and gluconeogenesis. For instance, L. plantarum CCFM0236, L. casei CCFM0412, and L. acidophilus SJLH001 increase α-glucosidase activity and reduce food intake, blood glucose level, and the glycosylated hemoglobin level; furthermore, L. acidophilus KLDS1.1003 and L. acidophilus KLDS1.0901 induced downregulation of the expression of glycogen synthase kinase in mice (60). There is more evidence showing that after the administration of L. rhamnosus, the mRNA expression of gluconeogenesis genes, especially glucose-6-phosphatase, in the gluconeogenesis pathway was inhibited (61). Probiotic-fermented milk prepared by L. rhamnosus MTCC: 5957, L. rhamnosus MTCC: 5897, and L. fermentans MTCC: 5898 significantly reduced the expression of key enzymes in the gluconeogenesis pathway (62). Dang F et al. applied L. paracasei TD062 to T2D mice and proved that it could downregulate the expression of gluconeogenic genes (52). In terms of glycogen consumption, probiotic-fermented milk containing L. acidophilus and L. casei has been shown to reduce FBG and HbA1c, increase muscle glucose uptake, and stimulate liver glucose absorption, which is consistent with the conclusions of another meta-analysis (59).
2.3.2 Decreasing insulin resistance
Studies have shown that the increase in blood glucose levels in rats is often accompanied by severe insulin resistance (63), and many Lactobacillus strains have decreased insulin resistance, thereby reducing the blood glucose level in diabetic rats. For example, L. casei CCFM419 reduce insulin resistance and hyperglycemia in T2D mice (38, 64). In diet-induced obesity model mice, Lactobacillus reduced insulin resistance and increased glucose tolerance, possibly by reducing endoplasmic reticulum stress in skeletal muscle, inhibiting macrophage activation, and promoting the transcription of glucose transporter 4 (65, 66).
Adiponectin (APN) is known as a fat factor that prevents the development of insulin resistance and T2DM. Increased serum APN is related to an increase in insulin sensitivity (67). Fibroblast growth factor 21 (FGF21) can promote the expression of adiponectin, and LGGs may alleviate PPAR-α through butyric acid activation, thereby effectively increasing the expression of FGF21 and the insulin sensitivity of mice (68). β cells also play an important role in blood glucose control; they can activate PI3K and Akt and regulate glycogen synthase (69) to control blood glucose. Matsuzaki et al. reported that Lactobacillus increased insulin binding in a T2DM mouse model and prevented cell destruction (70).
2.3.3 Increasing insulin levels in the body
There is no doubt that insulin plays a crucial role in T2DM-associated periodontitis, and blood glucose instability is often accompanied by insufficient amounts of insulin. Evaluation of insulin status in rats revealed that Lactobacillus may increase insulin levels by increasing insulin synthesis and inhibiting insulin decomposition and consumption. Glucagon-like peptide 1 (GLP-1) is a key incretin-stimulating hormone that plays a major role in insulin secretion (71). Application of a specific Lactobacillus strain (L. reuteri SD5865) increased insulin secretion by increasing GLP-1 release of incretin (72). Lactobacillus can also stimulate insulin secretion by regulating autonomic neurotransmitters, inhibiting the expression and activity of insulin-degrading enzyme (IDE), and reducing insulin consumption to slow the decline in insulin and the rise of blood glucose levels (73).
As a special substance, SCFAs, which are organic fatty acids produced by bacterial fermentation in the distal intestine, can be quickly transferred to the blood system and regulate glucose metabolism by a variety of mechanisms (74). The mechanisms by which SCFAs regulate glucose homeostasis may include (1) promoting the proliferation of intestinal epithelial cells and helping to maintain the integrity of the intestinal barrier; (2) reducing gluconeogenesis and inhibiting glycogen decomposition; and (3) increasing GLP-1 secretion, β cell quality, and function; stimulating intestinal endocrine cells to secrete peptide YY (PYY) and glucagon-like peptide 1 (GLP-1); and accelerating glucose-mediated insulin secretion. L. paracasei HII01 and L. casei CCFM419 may improve hyperglycemia by inhibiting the SCFA pathway (63), which further shows that Lactobacillus can alter glucose metabolism in several ways.
In summary, T2DM-associated periodontitis is characterized by a glucose standard, so it is feasible for Lactobacillus to improve glucose metabolism in different ways, including lowering blood sugar levels directly (reducing glycogen synthesis and increasing glycogen consumption) or indirectly (decreasing insulin resistance and increasing insulin levels in the body).
2.4 Direct resistance to pathogens
Compared with non-diabetic subjects, diabetic subjects had higher levels of Streptococcus sanguinis, Prevotella nigrescens, Treponema denticola, Streptococcus intermedius, and Streptococcus oralis in their dental plaque (75). Studies have clearly shown that most Lactobacillus strains are capable of inhibiting Actinomycetes, P. gingivalis, P. intermedia, and Streptococcus mutans growth, with the strongest antimicrobial activity being seen with parthenogenic heterogeneous fermenting Lactobacillus (L. plantarum, L. paracasei, and L. rhamnosus) and L. salivarius (76). The possible underlying mechanisms by which Lactobacillus directly inhibits the growth of other bacteria are the secretion of antibacterial proteins called bacteriocins and the production of toxic metabolites such as hydrogen peroxide, which may be produced by enzymes such as pyruvate oxidase, lactate oxidase, NADH oxidase, and NADH-independent reductase in Lactobacillus. Bacteriocins produced by Lactobacillus include the salivaricin produced by L. salivarius, the reuterin produced by L. reuteri, and the phytotoxin produced by L. plantarum (23). Bacteriocin forms short-lived pores on biofilms by interacting with different compounds, such as nisin, lipid II, and phosphorus, to eliminate pathogens. Lactobacillus produces lactic acid, an effective microbicide to prevent the colonization of bacterial and viral pathogens, and hydrogen peroxide and bacteriocin, which play an antibacterial role against oral pathogens. For example, the specific strain L. delbrueckii can inhibit P. gingivalis growth in vitro through the autolytic release of protein, which produces hydrogen peroxide and reacts with the Fenton of iron in the cell to form reactive oxygen species (77), thereby damaging DNA and inhibiting cell activity. Accordingly, another study found that in microaerobic environments in vivo, such as the interface between gingival crevices and teeth, L. delbrueckii also produces hydrogen peroxide, and hydrogen peroxide can diffuse freely in close enough proximity to P. gingivalis to impact the growth of P. gingivalis (78).
In addition to directly reducing pathogens, downregulating virulence gene expression to treat T2DM-associated periodontitis is also a viable strategy. Pathogenic bacteria in dental plaque biofilms invade periodontal tissues and secrete toxins such as lipopolysaccharide to destroy the supporting structure of teeth, thus negatively impacting periodontal health. Heat-inactivated L. acidophilus has been proven to bind to HOK cells from the oral cavity, which is the first step in treating T2DM-associated periodontitis. Following coaggregation with heat-inactivated Lactobacillus, the expression of the virulence gene fap2 of Fusarium nucleatum was significantly reduced, and its self-aggregation, adhesion, and invasion were largely restricted; consequently, the expression of proinflammatory genes in oral epithelial cells triggered by Fusarium nucleatum was prevented (79).
Overall, in terms of the direct removal of pathogenic bacteria, high concentrations of Lactobacillus CFF can achieve more than 99.99% bacterial clearance. In terms of attenuating the expression of virulence genes, Lactobacillus strongly attenuates the transcription of P. gingivalis virulence genes, thus reducing their virulence (47). Moreover, the metabolite ketone of Lactobacillus significantly inhibited P. gingivalis both in vivo and in vitro by reducing alveolar bone damage and suppressing P. gingivalis activity, proliferation rate, and CT values (36).
2.5 Regulating the microbiota
2.5.1 Regulating the oral microbiota
Periodontitis is a mixed anaerobic infection disease that may be in great part related to periodontal pathogens, including P. gingivalis, P. intermedia, Treponema denticola, and Clostridium nucleatum (80). Lactobacillus might modulate subgingival microflora to treat T2DM-associated periodontitis, which is mainly related to reducing the number of target pathogens through competitive colonization. Lactobacillus has good colonization potential (23) and is able to compete with P. gingivalis in terms of adhering to epithelial cells (27). A previous study showed that the level of pathogenic bacteria (especially P. gingivalis) in the periodontal pocket decreased after the application of Lactobacillus, such as L. salivarius WB21 (81). Lactobacillus may play a beneficial role in the oral cavity through direct and indirect interactions with microorganisms in the dental plaque. Lactic acid produced by Lactobacillus creates an acidic environment, which inhibits the growth of Gingival actinomycetes, Intermediate actinomycetes, and Streptococcus mutans. This effect may be the reason why Lactobacillus inhibits the growth of these microorganisms.
In addition to the above bacteria, the symbiosis of Candida albicans and Actinomycetes increases significantly in the periodontal environment; therefore, Candida albicans is also closely related to periodontitis (82). Thus, the inhibition of Candida albicans by Lactobacillus likely contributes to the balance of the oral microbiota. Animal studies have shown that Lactobacillus can reduce Candida infection, inhibit the growth of Candida by producing antibacterial compounds, and prevent its adhesion to epithelial cells through competitive colonization. In addition, in an in vitro model simulating gastrointestinal conditions, Lactobacillus also inhibited the growth of Candida (83), possibly by competing for the same receptor site.
2.5.2 Regulating the gut microbiota
The role of periodontal-related bacteria in the development of T2DM-associated periodontitis is clearly documented by studies showing that periodontal pathogens lead to intestinal flora disorder by moving to the intestine in rat and mouse models (84). Accordingly, the intestinal microbiota of T2DM patients is disordered, with a decrease in butyric acid-producing microorganisms and an increase in various opportunistic pathogenic bacteria. The proportions of Bacteroides and Firmicutes in T2DM patients were positively correlated with blood glucose concentration (85). Many Lactobacillus strains play a therapeutic role in T2DM-associated periodontitis by increasing beneficial intestinal flora and reducing harmful intestinal flora. We have previously mentioned the important role of SCFAs in blood glucose regulation, and beneficial bacteria such as Firmicutes, Actinobacteria, Anaerobes, Enterococcus faecalis, Lactobacillus, and Bifidobacteria increase the yield of SCFAs (86). The abundance of mucus spores and SCFA-producing bacteria (Streptococcus and Enterococcus faecalis) was significantly increased following Lactobacillus treatment. Overall, the role of Lactobacillus in maintaining oral and intestinal homeostasis should not be underestimated.
3 Application of Lactobacillus on type 2 diabetic periodontitis
Despite the mechanisms described above, there are not many studies on the direct effect of Lactobacillus on type 2 diabetic periodontitis patients, but indirectly through the therapeutic effect of Lactobacillus on type 2 diabetes or periodontitis. In this study, we present the available evidence on the direct use of Lactobacillus in diabetic periodontitis in Table 1.
4 Conclusion
In this article, we summarized the underlying mechanism by which Lactobacillus is therapeutic in T2DM-associated periodontitis. Lactobacillus plays a direct role in treating periodontitis and indirectly treats diabetes to alleviate the symptoms of T2DM-associated periodontitis. Figure 1 lists a large number of Lactobacillus strains that are therapeutic for periodontitis or T2DM, while Table 2 provides more detail on the actual effectiveness of each strain.
Lactobacillus improves the activity of periodontitis associated with T2DM. As shown in Figure 2, this may be related to intricate and complex mechanisms, including but not limited to reducing inflammation, modulating oxidative stress, improving glucose metabolism, direct antagonism of pathogenic bacteria, and modulating the microbiota. Lactobacillus may reduce inflammation by downregulating virulence gene expression, modulating cytokine levels, inhibiting NO production, reducing intestinal permeability, and enhancing intestinal barrier function. In terms of improving glucose metabolism, Lactobacillus can directly inhibit glucose synthesis, reduce glucose production, decrease insulin resistance, and protect blood glucose beta cells. It has also been suggested that lactobacilli can exert glucose-lowering effects by reducing bacterial translocation and modulating the intestinal ACSL3 pathway (91), but the relevant evidence is not sufficient. Most importantly, Lactobacillus intervention significantly altered the composition of the microbial community by reducing the number of pathogenic bacteria associated with T2DM-associated periodontitis in the oral microbiota and gut microbiota and increasing the number of beneficial bacteria, thereby showing therapeutic activity against T2DM-associated periodontitis. In summary, Lactobacillus are a promising option in the treatment of T2DM-associated periodontitis because they play a key role in host metabolism, regulate intestinal microecology, and reduce inflammation. Clinicians may consider including Lactobacillus in their treatment plans when treating T2DM-associated periodontitis.
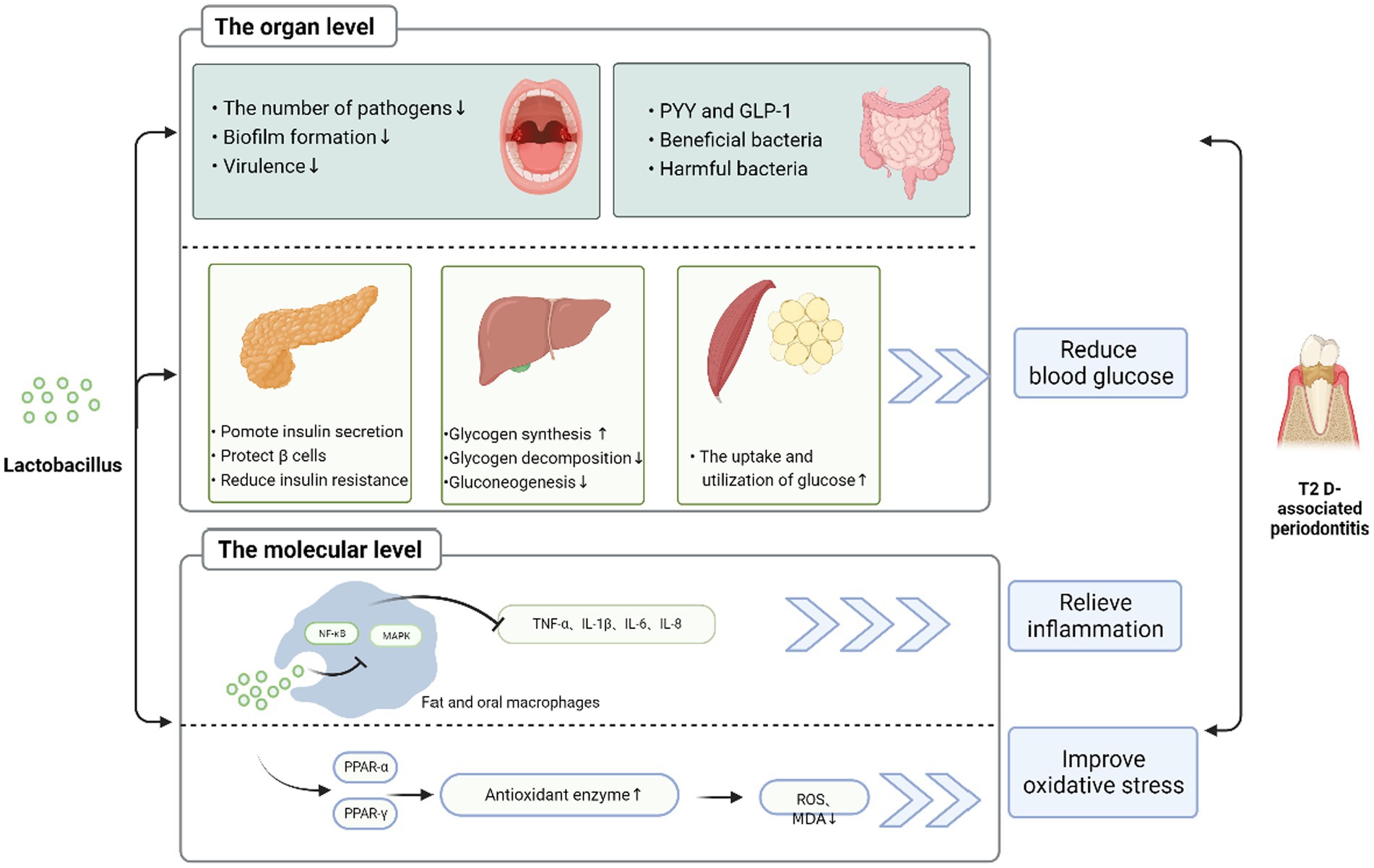
Figure 2. Possible mechanism of Lactobacillus improving diabetes mellitus with T2DM-associated periodontitis Lactobacillus can improve T2DM-associated periodontitis in various ways. (1) At the tissue level, Lactobacillus can promote insulin secretion and protect the pancreas β cells, reduce insulin resistance in the pancreas; increase glycogen synthesis; reduce glycogen decomposition and gluconeogenesis in the liver; increase PYY and GLP-1; increase beneficial bacteria and reduce harmful bacteria in the gut; increase glucose utilization in fat and muscle groups. Through these ways, it can reduce blood sugar, thereby reducing inflammation. In addition, Lactobacillus reduces the number of pathogenic bacteria, biofilm formation, and virulence in oral cavity and improves T2DM-associated periodontitis. (2) At the molecular level, Lactobacillus through MAPK and NF-κB signaling pathway, reduces the level of inflammatory factors. It is also possible to adjust PPAR-α and PPAR-γ to upregulate the level of antioxidant enzymes, thereby reducing ROS and MDA, improving oxidative stress, and ameliorating periodontitis in type II diabetes.
Nevertheless, some studies contradict our conclusions. A previous study found that eating soybean milk containing L. plantarum had no effect on serum Apn or inflammation (92). Similar results were reported by Hatakka et al. (93) in their study, probiotic supplementation did not result in significant changes in cytokines (such as TNF-a) within or between groups. This may be due to the beneficial effects of Lactobacillus supplementation, which may be related to the following factors: the specific Lactobacillus strains, the formulation of Lactobacillus probiotics (single or multiple strains, or with no prebiotics), the intervention time, dosage, and other factors. The anti-inflammatory properties of Lactobacillus are strain- and time-specific. Many studies have recommended regular consumption of preparations containing Lactobacillus to maintain health benefits. However, there are many strains of Lactobacillus, and the optimal dosage and frequency of each strain have not been explored, which may be the reason for the limited use of Lactobacillus in the clinic. Hence, further studies are needed to evaluate the best combination and application of Lactobacillus strains or synthetic probiotics containing Lactobacillus to prolong the effect of Lactobacillus on T2DM-associated periodontitis individuals and to explore the deeper mechanisms of T2DM-associated periodontitis treatment. In addition, there is not much evidence on the direct treatment of T2DM-associated periodontitis by Lactobacillus, but more studies on the significant effects on T2DM and the reduction in the effect of AGEs in T2DM-associated periodontitis. In addition, most of the current studies are based on animals, and there is a lack of research on patients with T2DM-associated periodontitis, so more research on this aspect is needed in future.
Author contributions
SC was responsible for literature review, data sorting, and draft writing. YZ was responsible for determining the title, revising, and approving the manuscript. All authors contributed to the article and approved the submitted version.
Conflict of interest
The authors declare that the research was conducted in the absence of any commercial or financial relationships that could be construed as a potential conflict of interest.
Publisher’s note
All claims expressed in this article are solely those of the authors and do not necessarily represent those of their affiliated organizations, or those of the publisher, the editors and the reviewers. Any product that may be evaluated in this article, or claim that may be made by its manufacturer, is not guaranteed or endorsed by the publisher.
References
1. Zhou, X, Zhang, W, Liu, X, Zhang, W, and Li, Y. Interrelationship between diabetes and periodontitis: role of hyperlipidemia. Arch Oral Biol. (2015) 60:667–74. doi: 10.1016/j.archoralbio.2014.11.008
2. Papapanou, PN, Sanz, M, Buduneli, N, Dietrich, T, Feres, M, Fine, DH, et al. Periodontitis: consensus report of workgroup 2 of the 2017 world workshop on the classification of periodontal and Peri-implant diseases and conditions. J Periodontol. (2018) 89 Suppl 1:S173–82. doi: 10.1002/JPER.17-0721
3. Ide, R, Hoshuyama, T, Wilson, D, Takahashi, K, and Higashi, T. Periodontal disease and incident diabetes: a seven-year study. J Dent Res. (2011) 90:41–6. doi: 10.1177/0022034510381902
4. Graziani, F, Gennai, S, Solini, A, and Petrini, M. A systematic review and meta-analysis of epidemiologic observational evidence on the effect of periodontitis on diabetes an update of the EFP-AAP review. J Clin Periodontol. (2018) 45:167–87. doi: 10.1111/jcpe.12837
5. Polepalle, T, Moogala, S, Boggarapu, S, Pesala, DS, and Palagi, FB. Acute phase proteins and their role in periodontitis: a review. J Clin Diagn Res. (2015) 9:ZE01–5. doi: 10.7860/JCDR/2015/15692.6728
6. Steward, FC, and Mott, RL. Cells, solutes, and growth: salt accumulation in plants reexamined. Int Rev Cytol. (1970) 28:275–370. doi: 10.1016/s0074-7696(08)62546-2
7. Lamont, RJ, Koo, H, and Hajishengallis, G. The oral microbiota: dynamic communities and host interactions. Nat Rev Microbiol. (2018) 16:745–59. doi: 10.1038/s41579-018-0089-x
8. Iatcu, CO, Steen, A, and Covasa, M. Gut microbiota and complications of Type-2 diabetes. Nutrients. (2021) 14:166. doi: 10.3390/nu14010166
9. Sookkhee, S, Chulasiri, M, and Prachyabrued, W. Lactic acid bacteria from healthy oral cavity of Thai volunteers: inhibition of oral pathogens. J Appl Microbiol. (2001) 90:172–9. doi: 10.1046/j.1365-2672.2001.01229.x
10. Qin, J, Li, Y, Cai, Z, Li, S, Zhu, J, Zhang, F, et al. A metagenome-wide association study of gut microbiota in type 2 diabetes. Nature. (2012) 490:55–60. doi: 10.1038/nature11450
11. Hill, C, Guarner, F, Reid, G, Gibson, GR, Merenstein, DJ, Pot, B, et al. Expert consensus document. The international scientific Association for Probiotics and Prebiotics consensus statement on the scope and appropriate use of the term probiotic. Nat Rev Gastroenterol Hepatol. (2014) 11:506–14. doi: 10.1038/nrgastro.2014.66
12. Kim, S, and Woo, GJ. Prevalence and characterization of antimicrobial-resistant Escherichia coli isolated from conventional and organic vegetables. Foodborne Pathog Dis. (2014) 11:815–21. doi: 10.1089/fpd.2014.1771
13. Kandler, O. Archaebacteria and phylogeny of organisms. Naturwissenschaften. (1981) 68:183–92. doi: 10.1007/BF01047198
14. Colloca, ME, Ahumada, MC, Lopez, ME, and Nader-Macias, ME. Surface properties of lactobacilli isolated from healthy subjects. Oral Dis. (2000) 6:227–33. doi: 10.1111/j.1601-0825.2000.tb00118.x
15. Zhao, X, Zhong, X, Liu, X, Wang, X, and Gao, X. Therapeutic and improving function of lactobacilli in the prevention and treatment of cardiovascular-related diseases: a novel perspective from gut microbiota. Front Nutr. (2021) 8:693412. doi: 10.3389/fnut.2021.693412
16. Marcotte, H, Larsson, PG, Andersen, KK, Zuo, F, Mikkelsen, LS, Brandsborg, E, et al. An exploratory pilot study evaluating the supplementation of standard antibiotic therapy with probiotic lactobacilli in south African women with bacterial vaginosis. BMC Infect Dis. (2019) 19:824. doi: 10.1186/s12879-019-4425-1
17. Nami, Y, Haghshenas, B, Haghshenas, M, Abdullah, N, and Yari, KA. The prophylactic effect of probiotic Enterococcus lactis IW5 against different human Cancer cells. Front Microbiol. (2015) 6:1317. doi: 10.3389/fmicb.2015.01317
18. Ho, HE, and Bunyavanich, S. Microbial adjuncts for food allergen immunotherapy. Curr Allergy Asthma Rep. (2019) 19:25. doi: 10.1007/s11882-019-0859-1
19. Gionchetti, P, Rizzello, F, Venturi, A, Brigidi, P, Matteuzzi, D, Bazzocchi, G, et al. Oral bacteriotherapy as maintenance treatment in patients with chronic pouchitis: a double-blind, placebo-controlled trial. Gastroenterology. (2000) 119:305–9. doi: 10.1053/gast.2000.9370
20. Madsen, K, Cornish, A, Soper, P, McKaigney, C, Jijon, H, Yachimec, C, et al. Probiotic bacteria enhance murine and human intestinal epithelial barrier function. Gastroenterology. (2001) 121:580–91. doi: 10.1053/gast.2001.27224
21. Loskutova, IE. Effectiveness of using Maliutka and Malysh adapted propionic-acidophilus mixtures in the combined treatment of congenital hypotrophy. Vopr Pitan. (1985) 3:17–20.
22. Gao, X, Jiang, S, Koh, D, and Hsu, CY. Salivary biomarkers for dental caries. Periodontol. (2000) 70:128–41. doi: 10.1111/prd.12100
23. Nguyen, T, Brody, H, Radaic, A, and Kapila, Y. Probiotics for periodontal health-current molecular findings. Periodontol. (2000) 2021:254–67. doi: 10.1111/prd.12382
24. Luoto, R, Laitinen, K, Nermes, M, and Isolauri, E. Impact of maternal probiotic-supplemented dietary counselling on pregnancy outcome and prenatal and postnatal growth: a double-blind, placebo-controlled study. Br J Nutr. (2010) 103:1792–9. doi: 10.1017/S0007114509993898
25. Moroti, C, Souza Magri, LF, de Rezende, CM, Cavallini, DC, and Sivieri, K. Effect of the consumption of a new symbiotic shake on glycemia and cholesterol levels in elderly people with type 2 diabetes mellitus. Lipids Health Dis. (2012) 11:29. doi: 10.1186/1476-511X-11-29
26. Jebin, AA, Nisha, KJ, and Padmanabhan, S. Oral microbial shift following 1-month supplementation of probiotic chewable tablets containing Lactobacillus reuteri UBLRu-87 as an adjunct to phase 1 periodontal therapy in chronic periodontitis patients: a randomized controlled clinical trial. Contemp Clin Dent. (2021) 12:121–7. doi: 10.4103/ccd.ccd_135_20
27. Albuquerque-Souza, E, Balzarini, D, Ando-Suguimoto, ES, Ishikawa, KH, Simionato, MRL, Holzhausen, M, et al. Probiotics alter the immune response of gingival epithelial cells challenged by Porphyromonas gingivalis. J Periodontal Res. (2019) 54:115–27. doi: 10.1111/jre.12608
28. Sturm, A, Baumgart, DC, d'Heureuse, JH, Hotz, A, Wiedenmann, B, and Dignass, AU. CXCL8 modulates human intestinal epithelial cells through a CXCR1 dependent pathway. Cytokine. (2005) 29:42–8. doi: 10.1016/j.cyto.2004.09.007
29. Albuquerque-Souza, E, Ishikawa, KH, Amado, PP, Nicoli, JR, Holzhausen, M, and Mayer, MPA. Probiotics improve re-epithelialization of scratches infected by Porphyromonas gingivalis through up-regulating CXCL8-CXCR1/CXCR2 axis. Anaerobe. (2021) 72:102458. doi: 10.1016/j.anaerobe.2021.102458
30. Stamatova, I, and Meurman, JH. Probiotics and periodontal disease. Periodontology. (2000) 51:141–51. doi: 10.1111/j.1600-0757.2009.00305.x
31. Saglam, M, Kantarci, A, Dundar, N, and Hakki, SS. Clinical and biochemical effects of diode laser as an adjunct to nonsurgical treatment of chronic periodontitis: a randomized, controlled clinical trial. Lasers Med Sci. (2014) 29:37–46. doi: 10.1007/s10103-012-1230-0
32. Reynolds, JJ. Collagenases and tissue inhibitors of metalloproteinases: a functional balance in tissue degradation. Oral Dis. (1996) 2:70–6. doi: 10.1111/j.1601-0825.1996.tb00206.x
33. Tüter, G, Serdar, M, Kurtiş, B, Walker, SG, Atak, A, Toyman, U, et al. Effects of scaling and root planing and subantimicrobial dose doxycycline on gingival crevicular fluid levels of matrix metalloproteinase-8, −13 and serum levels of HsCRP in patients with chronic periodontitis. J Periodontol. (2010) 81:1132–9. doi: 10.1902/jop.2010.090694
34. Ince, G, Gursoy, H, Ipci, SD, Cakar, G, Emekli-Alturfan, E, and Yilmaz, S. Clinical and biochemical evaluation of lozenges containing Lactobacillus reuteri as an adjunct to non-surgical periodontal therapy in chronic periodontitis. J Periodontol. (2015) 86:746–54. doi: 10.1902/jop.2015.140612
35. Cloitre, A, Halgand, B, Sourice, S, Caillon, J, Huck, O, Bugueno, IM, et al. IL-36gamma is a pivotal inflammatory player in periodontitis-associated bone loss. Sci Rep. (2019) 9:19257. doi: 10.1038/s41598-019-55595-9
36. Sulijaya, B, Takahashi, N, and Yamazaki, K. Lactobacillus-derived bioactive metabolites for the regulation of periodontal health: evidences to clinical setting. Molecules. (2020) 25:2088. doi: 10.3390/molecules25092088
37. Huang, HY, Korivi, M, Tsai, CH, Yang, JH, and Tsai, YC. Supplementation of Lactobacillus plantarum K68 and fruit-vegetable ferment along with high fat-fructose diet attenuates metabolic syndrome in rats with insulin resistance. Evid Based Complement Alternat Med. (2013) 2013:943020. doi: 10.1155/2013/943020
38. Wang, G, Li, X, Zhao, J, Zhang, H, and Chen, W. Lactobacillus casei CCFM419 attenuates type 2 diabetes via a gut microbiota dependent mechanism. Food Funct. (2017) 8:3155–64. doi: 10.1039/c7fo00593h
39. Pena, JA, and Versalovic, J. Lactobacillus rhamnosus GG decreases TNF-alpha production in lipopolysaccharide-activated murine macrophages by a contact-independent mechanism. Cell Microbiol. (2003) 5:277–85. doi: 10.1046/j.1462-5822.2003.t01-1-00275.x
40. Kazmierczyk-Winciorek, M, Nedzi-Gora, M, and Slotwinska, SM. The immunomodulating role of probiotics in the prevention and treatment of oral diseases. Cent Eur J Immunol. (2021) 46:99–104. doi: 10.5114/ceji.2021.104412
41. de Kort, S, Keszthelyi, D, and Masclee, AA. Leaky gut and diabetes mellitus: what is the link? Obes Rev. (2011) 12:449–58. doi: 10.1111/j.1467-789X.2010.00845.x
42. van Baarlen, P, Troost, FJ, van Hemert, S, van der Meer, C, de Vos, WM, de Groot, PJ, et al. Differential NF-kappaB pathways induction by Lactobacillus plantarum in the duodenum of healthy humans correlating with immune tolerance. Proc Natl Acad Sci U S A. (2009) 106:2371–6. doi: 10.1073/pnas.0809919106
43. Karczewski, J, Troost, FJ, Konings, I, Dekker, J, Kleerebezem, M, Brummer, RJ, et al. Regulation of human epithelial tight junction proteins by Lactobacillus plantarum in vivo and protective effects on the epithelial barrier. Am J Physiol Gastrointest Liver Physiol. (2010) 298:G851–9. doi: 10.1152/ajpgi.00327.2009
44. Srutkova, D, Schwarzer, M, Hudcovic, T, Zakostelska, Z, Drab, V, Spanova, A, et al. Bifidobacterium longum CCM 7952 promotes epithelial barrier function and prevents acute DSS-induced colitis in strictly strain-specific manner. PLoS One. (2015) 10:e0134050. doi: 10.1371/journal.pone.0134050
45. Seminario-Amez, M, Lopez-Lopez, J, Estrugo-Devesa, A, Ayuso-Montero, R, and Jane-Salas, E. Probiotics and oral health: a systematic review. Med Oral Patol Oral Cir Bucal. (2017) 22:e282–8. doi: 10.4317/medoral.21494
46. Widyarman, AS, and Theodorea, CF. Novel indigenous probiotic Lactobacillus reuteri strain produces anti-biofilm Reuterin against pathogenic periodontal Bacteria. Eur J Dent. (2022) 16:96–101. doi: 10.1055/s-0041-1731591
47. Wilson, RM, Walker, JM, and Yin, K. Different concentrations of Lactobacillus acidophilus cell free filtrate have differing anti-biofilm and immunomodulatory effects. Front Cell Infect Microbiol. (2021) 11:737392. doi: 10.3389/fcimb.2021.737392
48. Sczepanik, FSC, Grossi, ML, Casati, M, Goldberg, M, Glogauer, M, Fine, N, et al. Periodontitis is an inflammatory disease of oxidative stress: we should treat it that way. Periodontol. (2000) 84:45–68. doi: 10.1111/prd.12342
49. Chang, YC, and Chuang, LM. The role of oxidative stress in the pathogenesis of type 2 diabetes: from molecular mechanism to clinical implication. Am J Transl Res. (2010) 2:316–31. doi: 10.4168/aair.2010.2.3.183
50. Lin, MY, and Chang, FJ. Antioxidative effect of intestinal bacteria Bifidobacterium longum ATCC 15708 and Lactobacillus acidophilus ATCC 4356. Digest Dis Sci. (2000) 45:1617–22. doi: 10.1023/A:1005577330695
51. Yokoji-Takeuchi, M, Takahashi, N, Yamada-Hara, M, Sulijaya, B, Tsuzuno, T, Aoki-Nonaka, Y, et al. A bacterial metabolite induces Nrf2-mediated anti-oxidative responses in gingival epithelial cells by activating the MAPK signaling pathway. Arch Oral Biol. (2020) 110:104602. doi: 10.1016/j.archoralbio.2019.104602
52. Dang, F, Jiang, Y, Pan, R, Zhou, Y, Wu, S, Wang, R, et al. Administration of Lactobacillus paracasei ameliorates type 2 diabetes in mice. Food Funct. (2018) 9:3630–9. doi: 10.1039/c8fo00081f
53. Zeng, Z, Yuan, Q, Yu, R, Zhang, J, Ma, H, and Chen, S. Ameliorative effects of probiotic Lactobacillus paracasei NL41 on insulin sensitivity, oxidative stress, and Beta-cell function in a type 2 diabetes mellitus rat model. Mol Nutr Food Res. (2019) 63:e1900457. doi: 10.1002/mnfr.201900457
54. Herrera, BS, Martins-Porto, R, Maia-Dantas, A, Campi, P, Spolidorio, LC, Costa, SK, et al. iNOS-derived nitric oxide stimulates osteoclast activity and alveolar bone loss in ligature-induced periodontitis in rats. J Periodontol. (2011) 82:1608–15. doi: 10.1902/jop.2011.100768
55. Han, CY. Roles of reactive oxygen species on insulin resistance in adipose tissue. Diabetes Metab J. (2016) 40:272–9. doi: 10.4093/dmj.2016.40.4.272
56. Liu, WC, Yang, MC, Wu, YY, Chen, PH, Hsu, CM, and Chen, LW. Lactobacillus plantarum reverse diabetes-induced Fmo3 and ICAM expression in mice through enteric dysbiosis-related c-Jun NH2-terminal kinase pathways. PLoS One. (2018) 13:e0196511. doi: 10.1371/journal.pone.0196511
57. Zhang, Y, Guo, X, Guo, J, He, Q, Li, H, Song, Y, et al. Lactobacillus casei reduces susceptibility to type 2 diabetes via microbiota-mediated body chloride ion influx. Sci Rep. (2014) 4:5654. doi: 10.1038/srep05654
58. Harisa, IG, Taha, IE, Khalil, FA, and Salem, MM. Oral Administration of Lactobacillus Acidophilus Restores Nitric Oxide Level in diabetic rats. Austral J Basic Applied Sci. (2009)
59. Yao, K, Zeng, L, He, Q, Wang, W, Lei, J, and Zou, X. Effect of probiotics on glucose and lipid metabolism in type 2 diabetes mellitus: a Meta-analysis of 12 randomized controlled trials. Med Sci Monit. (2017) 23:3044–53. doi: 10.12659/msm.902600
60. Yan, F, Li, N, Shi, J, Li, H, Yue, Y, Jiao, W, et al. Lactobacillus acidophilus alleviates type 2 diabetes by regulating hepatic glucose, lipid metabolism and gut microbiota in mice. Food Funct. (2019) 10:5804–15. doi: 10.1039/c9fo01062a
61. Farida, E, Nuraida, L, Giriwono, PE, and Jenie, BSL. Lactobacillus rhamnosus reduces blood glucose level through downregulation of gluconeogenesis gene expression in Streptozotocin-induced diabetic rats. Int J Food Sci. (2020) 2020:6108575. doi: 10.1155/2020/6108575
62. Yadav, R, Dey, DK, Vij, R, Meena, S, Kapila, R, and Kapila, S. Evaluation of anti-diabetic attributes of Lactobacillus rhamnosus MTCC: 5957, Lactobacillus rhamnosus MTCC: 5897 and Lactobacillus fermentum MTCC: 5898 in streptozotocin induced diabetic rats. Microb Pathog. (2018) 125:454–62. doi: 10.1016/j.micpath.2018.10.015
63. Zhang, L, Zhou, W, Zhan, L, Hou, S, Zhao, C, Bi, T, et al. Fecal microbiota transplantation alters the susceptibility of obese rats to type 2 diabetes mellitus. Aging (Albany NY). (2020) 12:17480–502. doi: 10.18632/aging.103756
64. Li, X, Wang, E, Yin, B, Fang, D, Chen, P, Wang, G, et al. Effects of Lactobacillus casei CCFM419 on insulin resistance and gut microbiota in type 2 diabetic mice. Benef Microbes. (2017) 8:421–32. doi: 10.3920/BM2016.0167
65. Tabuchi, M, Ozaki, M, Tamura, A, Yamada, N, Ishida, T, Hosoda, M, et al. Antidiabetic effect of Lactobacillus GG in streptozotocin-induced diabetic rats. Biosci Biotechnol Biochem. (2003) 67:1421–4. doi: 10.1271/bbb.67.1421
66. Honda, K, Moto, M, Uchida, N, He, F, and Hashizume, N. Anti-diabetic effects of lactic acid bacteria in normal and type 2 diabetic mice. J Clin Biochem Nutr. (2012) 51:96–101. doi: 10.3164/jcbn.11-07
67. Lihn, AS, Pedersen, SB, and Richelsen, B. Adiponectin: action, regulation and association to insulin sensitivity. Obes Rev. (2005) 6:13–21. doi: 10.1111/j.1467-789X.2005.00159.x
68. Kim, SW, Park, KY, Kim, B, Kim, E, and Hyun, CK. Lactobacillus rhamnosus GG improves insulin sensitivity and reduces adiposity in high-fat diet-fed mice through enhancement of adiponectin production. Biochem Biophys Res Commun. (2013) 431:258–63. doi: 10.1016/j.bbrc.2012.12.121
69. Newsholme, P, Cruzat, VF, Keane, KN, Carlessi, R, and de Bittencourt, PI Jr. Molecular mechanisms of ROS production and oxidative stress in diabetes. Biochem J. (2016) 473:4527–50. doi: 10.1042/BCJ20160503C
70. Matsuzaki, T, Yamazaki, R, Hashimoto, S, and Yokokura, T. Antidiabetic effects of an oral administration of Lactobacillus casei in a non-insulin-dependent diabetes mellitus (NIDDM) model using KK-ay mice. Endocr J. (1997) 44:357–65. doi: 10.1507/endocrj.44.357
71. Cani, PD, Lecourt, E, Dewulf, EM, Sohet, FM, Pachikian, BD, Naslain, D, et al. Gut microbiota fermentation of prebiotics increases satietogenic and incretin gut peptide production with consequences for appetite sensation and glucose response after a meal. Am J Clin Nutr. (2009) 90:1236–43. doi: 10.3945/ajcn.2009.28095
72. Simon, MC, Strassburger, K, Nowotny, B, Kolb, H, Nowotny, P, Burkart, V, et al. Intake of Lactobacillus reuteri improves incretin and insulin secretion in glucose-tolerant humans: a proof of concept. Diabetes Care. (2015) 38:1827–34. doi: 10.2337/dc14-2690
73. Yadav, H, Jain, S, and Sinha, PR. Oral administration of dahi containing probiotic Lactobacillus acidophilus and Lactobacillus casei delayed the progression of streptozotocin-induced diabetes in rats. J Dairy Res. (2008) 75:189–95. doi: 10.1017/S0022029908003129
74. Puddu, A, Sanguineti, R, Montecucco, F, and Viviani, GL. Evidence for the gut microbiota short-chain fatty acids as key pathophysiological molecules improving diabetes. Mediat Inflamm. (2014) 2014:162021. doi: 10.1155/2014/162021
75. Hintao, J, Teanpaisan, R, Chongsuvivatwong, V, Ratarasan, C, and Dahlen, G. The microbiological profiles of saliva, supragingival and subgingival plaque and dental caries in adults with and without type 2 diabetes mellitus. Oral Microbiol Immunol. (2007) 22:175–81. doi: 10.1111/j.1399-302X.2007.00341.x
76. Koll-Klais, P, Mandar, R, Leibur, E, Marcotte, H, Hammarstrom, L, and Mikelsaar, M. Oral lactobacilli in chronic periodontitis and periodontal health: species composition and antimicrobial activity. Oral Microbiol Immunol. (2005) 20:354–61. doi: 10.1111/j.1399-302X.2005.00239.x
77. Imlay, JA. The molecular mechanisms and physiological consequences of oxidative stress: lessons from a model bacterium. Nat Rev Microbiol. (2013) 11:443–54. doi: 10.1038/nrmicro3032
78. Cornacchione, LP, Klein, BA, Duncan, MJ, and Hu, LT. Interspecies inhibition of Porphyromonas gingivalis by yogurt-derived Lactobacillus delbrueckii requires active pyruvate oxidase. Appl Environ Microbiol. (2019) 85:e01271–19. doi: 10.1128/AEM.01271-19
79. Ding, Q, Sun, X, Cao, S, Zhao, C, Wang, Y, and Wang, X. Heat-killed Lactobacillus acidophilus mediates Fusobacterium nucleatum induced pro-inflammatory responses in epithelial cells. FEMS Microbiol Lett. (2021) 368:160. doi: 10.1093/femsle/fnaa160
80. Suzuki, N, Tanabe, K, Takeshita, T, Yoneda, M, Iwamoto, T, Oshiro, S, et al. Effects of oil drops containing Lactobacillus salivarius WB21 on periodontal health and oral microbiota producing volatile sulfur compounds. J Breath Res. (2012) 6:017106. doi: 10.1088/1752-7155/6/1/017106
81. Mayanagi, G, Kimura, M, Nakaya, S, Hirata, H, Sakamoto, M, Benno, Y, et al. Probiotic effects of orally administered Lactobacillus salivarius WB21-containing tablets on periodontopathic bacteria: a double-blinded, placebo-controlled, randomized clinical trial. J Clin Periodontol. (2009) 36:506–13. doi: 10.1111/j.1600-051X.2009.01392.x
82. Jabri, B, Iken, M, Ait-Ou-Amar, S, Rida, S, Bouziane, A, and Ennibi, OK. Candida albicans and Candida dubliniensis in periodontitis in adolescents and young adults. Int J Microbiol. (2022) 2022:4625368–8. doi: 10.1155/2022/4625368
83. Payne, S, Gibson, G, Wynne, A, Hudspith, B, Brostoff, J, and Tuohy, K. In vitro studies on colonization resistance of the human gut microbiota to Candida albicans and the effects of tetracycline and Lactobacillus plantarum LPK. Curr Issues Intest Microbiol. (2003) 4:1–8.
84. Koliarakis, I, Messaritakis, I, Nikolouzakis, TK, Hamilos, G, Souglakos, J, and Tsiaoussis, J. Oral Bacteria and intestinal Dysbiosis in colorectal Cancer. Int J Mol Sci. (2019) 20:4146. doi: 10.3390/ijms20174146
85. Larsen, N, Vogensen, FK, van den Berg, FW, Nielsen, DS, Andreasen, AS, Pedersen, BK, et al. Gut microbiota in human adults with type 2 diabetes differs from non-diabetic adults. PLoS One. (2010) 5:e9085. doi: 10.1371/journal.pone.0009085
86. Gao, H, Wen, JJ, Hu, JL, Nie, QX, Chen, HH, Xiong, T, et al. Polysaccharide from fermented Momordica charantia L. with Lactobacillus plantarum NCU116 ameliorates type 2 diabetes in rats. Carbohydr Polym. (2018) 201:624–33. doi: 10.1016/j.carbpol.2018.08.075
87. Silva, DNA, Cruz, NTS, Martins, AA, Silva, RCM, Almeida, HC, Costa, HES, et al. Probiotic Lactobacillus rhamnosus EM1107 prevents hyperglycemia, alveolar bone loss, and inflammation in a rat model of diabetes and periodontitis. J Periodontol. (2023) 94:376–88. doi: 10.1002/JPER.22-0262
88. Elsadek, MF, Ahmed, BM, Alkhawtani, DM, and Zia, SA. A comparative clinical, microbiological and glycemic analysis of photodynamic therapy and Lactobacillus reuteri in the treatment of chronic periodontitis in type-2 diabetes mellitus patients. Photodiagn Photodyn Ther. (2020) 29:101629. doi: 10.1016/j.pdpdt.2019.101629
89. Lu, M, Zhang, Y, Yuan, X, Zhang, Y, Zhou, M, Zhang, T, et al. Increased serum alpha-tocopherol acetate mediated by gut microbiota ameliorates alveolar bone loss through the STAT3 signalling pathway in diabetic periodontitis. J Clin Periodontol. (2023) 50:1539–52. doi: 10.1111/jcpe.13862
90. Malyshev, ME, Iordanishvili, AK, Prisyazhnyuk, OV, and Bumai, AO. The effect of probiotics on the secretory immunity of saliva in patients with type 2 diabetes. Stomatologiia. (2019) 98:26–9. doi: 10.17116/stomat20199806126
91. Bauer, PV, Duca, FA, Waise, TMZ, Dranse, HJ, Rasmussen, BA, Puri, A, et al. Lactobacillus gasseri in the upper small intestine impacts an ACSL3-dependent fatty acid-sensing pathway regulating whole-body glucose homeostasis. Cell Metab. (2018) 27:572–587.e6. doi: 10.1016/j.cmet.2018.01.013
92. Feizollahzadeh, S, Ghiasvand, R, Rezaei, A, Khanahmad, H, Sadeghi, A, and Hariri, M. Effect of probiotic soy Milk on serum levels of adiponectin, inflammatory mediators, lipid profile, and fasting blood glucose among patients with type II diabetes mellitus. Probiot Antimicrob Prot. (2017) 9:41–7. doi: 10.1007/s12602-016-9233-y
Keywords: periodontitis, type II diabetes, Lactobacillus , mechanism, application
Citation: Chen S and Zhang Y (2023) Mechanism and application of Lactobacillus in type 2 diabetes-associated periodontitis. Front. Public Health. 11:1248518. doi: 10.3389/fpubh.2023.1248518
Edited by:
Mohd Ashraf Ganie, Sher-I-Kashmir Institute of Medical Sciences, IndiaReviewed by:
Giorgio Giraffa, Council for Agricultural and Economics Research (CREA), ItalyGiulia Matacchione, Università Politecnica delle Marche, Italy
Copyright © 2023 Chen and Zhang. This is an open-access article distributed under the terms of the Creative Commons Attribution License (CC BY). The use, distribution or reproduction in other forums is permitted, provided the original author(s) and the copyright owner(s) are credited and that the original publication in this journal is cited, in accordance with accepted academic practice. No use, distribution or reproduction is permitted which does not comply with these terms.
*Correspondence: Yuhan Zhang, 835061684@qq.com