- 1RECETOX, Faculty of Science, Masaryk University, Brno, Czechia
- 2Masaryk Memorial Cancer Institute, Brno, Czechia
Introduction: The exposures to hazardous antineoplastic drugs (AD) represent serious risks for health care personnel but the exposure limits are not commonly established because of the no-threshold effects (genotoxic action, carcinogenicity) of many ADs. In this study, we discussed and derived practically applicable technical guidance values (TGV) suitable for management of AD risks.
Methods: The long-term monitoring of surface contamination by eight ADs was performed in pharmacies and hospitals in the Czech Republic and Slovak Republic in 2008–2021; in total 2,223 unique samples were collected repeatedly in 48 facilities. AD contamination was studied by LC-MS/MS for cyclophosphamide, ifosfamide, methotrexate, irinotecan, paclitaxel, 5-fluorouracil and gemcitabine and by ICP-MS for total Pt as a marker of platinum-based ADs.
Results: The study highlighted importance of exposure biomarkers like 5-fluorouracil and especially carcinogenic and persistent cyclophosphamide, which should be by default included in monitoring along with other ADs. Highly contaminated spots like interiors of laminar biological safety cabinets represent a specific issue, where monitoring of contamination does not bring much added value, and prevention of staff and separated cleaning procedures should be priority. Rooms and surfaces in health care facilities that should be virtually free of ADs (e.g., offices, kitchenettes, daily rooms) were contaminated with lower frequency and concentrations but any contamination in these areas should be carefully examined.
Discussion and conclusions: For all other working places, i.e., majority of areas in pharmacies and hospitals, where ADs are being prepared, packaged, stored, transported, or administered to patients, the study proposes a generic TGV of 100 pg/cm2. The analysis of long-term monitoring data of multiple ADs showed that the exceedance of one TGV can serve as an indicator and trigger for improvement of working practices contributing thus to minimizing of unintended exposures and creating a safe work environment.
1. Introduction
A growing number of oncology patients as well as new types of therapy applications (1) leads to increasing use of antineoplastic drugs (ADs). In 2020, more than 19 million new cases of cancer were diagnosed (2). The therapeutic benefits of ADs with carcinogenic, mutagenic, and teratogenic properties outweigh the risks for patients but they represent a risk for health care workers. The long-term occupational exposures have been associated with adverse health outcomes including reproduction toxicity or cancer (3, 4). Acute adverse health effects in such as skin rashes and hair loss have been also reported (5, 6).
Occupational exposures of health care staff to ADs may occur in pharmacies and hospitals through direct dermal contact, inhalation, accidental ingestion, or indirectly via surfaces contaminated by ADs during their preparation, handling or administration to patients (3, 7). To minimize the occupational exposure and achieve maximum product safety, the preparation of ADs is regulated. Preparation of ADs is usually done in laminar or negative pressure boxes (3). In some countries, including Czech Republic, closed systems such as biohazard safety cabinets (BSC) are required by national regulation for AD preparation (8). However, other processes in handling of ADs are often less controlled and may lead to serious occupational exposures of nurses as well as sanitary staff (cleaning of contaminated floors or desktops/tables, handling and washing of contaminated beddings). Recently, exposures to ADs in home care settings have also been documented (9, 10).
The risks of hazardous medicinal products have recently been addressed by authorities around the world. The European Union updated in 2022 the 2004/37/EC Directive on the protection of workers from the risks related to exposure to carcinogens or mutagens at work (Directive (EU) 2022/431),1 and a detailed Guidance for the safe management of hazardous medicinal products at work was published in 2023 by the EU Agency for Safety and Health at Work (OSHA).2 In parallel, the European Trade Union Institute (ETUI) and the European Biosafety Network (EBN) released the updated list of hazardous medicinal products based on the Regulation (EC) 1272/2008 on the classification, labeling and packaging (CLP).3 The ongoing EU Partnership on Risk Assessment of Chemicals PARC (https://www.eu-parc.eu/) also runs the initiative on pan-European evaluation of ADs occupational risks. Also in the USA, the National Institute for Occupational Safety and Health released a detailed document on managing exposures to hazardous drugs in 2023 (11).
While some occupational exposure limit values have been provided in the EU for 58 industrial carcinogens, mutagens and reprotoxic substances (Annex III of EU Directive 2004/37/EC),4 no official limits for surface contamination by hazardous medicinal products have been established yet. Correspondingly, national regulations or protocols in health care facilities usually follow the “as low as reasonably achievable” principle (ALARA) to assure low occupational exposures (12). Nevertheless, despite the existing guidelines and prevention measures, monitoring studies still report AD contamination in health care facilities, and the external exposures were confirmed by detection of ADs or their metabolites in urine or blood of health care workers as well as family members of oncology patients (13–18).
Monitoring of contamination in workplaces is an important tool in risk management of these hazardous compounds (3, 10, 12, 19–22), and the standardized wipe sampling of the surfaces is the most broadly used approach to detect contamination by ADs (3). Monitoring results allow to prioritize hot spots, identify major sources, routes of release of ADs during handling, compare situations among health care facilities and positive results often trigger implementation of remedial, and preventive measures (3).
Nowadays, about 100 chemically diverse ADs with various mechanisms of action are used in cancer chemotherapy (3). The consumption of different ADs differ, and some compounds are highly relevant exposure markers with respect to their use and properties such as environmental persistence despite of cleaning procedures (23). For example, in the Czech Republic, about eight ADs are applied intra venously in large quantities including cyclophosphamide (CP), platinum-based drugs (Pt), 5-fluorouracil (FU), paclitaxel (PX), gemcitabine (GEM), irinotecan (IRI), ifosfamide (IF) and methotrexate (MET). These 8 ADs form 50% or more of the AD applications prepared in individual hospitals (9, 10). In agreement with other studies, this shows high importance of few ADs, namely CP, FU and Pt-based drugs as representative markers of occupational exposures (3, 15, 23–25).
The exposure levels in different pharmacy and hospital places may differ by orders of magnitude reaching up to hundreds ng/cm2 (documented e.g., for CP and FU) or tens ng/cm2 (Pt-based drugs) (15, 25–27). Most commonly, ADs are analyzed in wipe samples from the floors, desktops or various handles (26). Some sites, such as interior of laminar flow boxes are naturally highly contaminated due to open handling of ADs, lower levels are being found at other sites such as storage rooms, outpatient clinics etc. (21, 25, 28–34). On the other hand, these areas, where the procedures and staff are much less controlled represent higher risk to health care workers, e.g., via transdermal absorption (35, 36).
As mentioned above, individual occupational exposure limits for AD in work environment are not commonly established because of the “no-threshold effects” (genotoxic action of many ADs) and poorly understood links with adverse health effects in workers (3, 32). However, for practical reasons, risk managers seek for recommendations such as threshold guidance values (TGV) or hygiene guidance value (HGV). These have been proposed by some authors based on long-term monitoring data sets, e.g., as the 75th, 90th or 95th percentiles of the detected contamination (27, 30, 31, 34, 37, 38). Exceedance of TGVs (or HGVs) indicates that the exposures are not properly controlled and may trigger implementation of measures. Alternatively, a Dutch study (39) suggested a “traffic-light” model for CP considering correlations between the CP levels in the urine of healthcare workers and corresponding surface contamination. This study suggested that surface concentrations of CP < 0.1 ng/cm2 might be considered relatively safe (“green”), while CP values above 10 ng/cm2 are not acceptable and calls for immediate action (39). Numerically similar guidance values 0.1 ng/cm2 for CP and other ADs were suggested further by Connor et al. (20), Kiffmeyer et al. (31), Crul and Simons-Sanders (40), and Korczowska et al. (32) and this value was also highlighted in a document from the European Biosafety Network commenting on amendments of Directive 2004/37/EC on the protection of workers from the risks related to exposure to carcinogens or mutagens.5
Based on these evidences, national organizations continue to release recommendations for handling of hazardous drugs in health care sector (3) but debates on guidance values are still open and other important factors such as combined exposures to ADs mixtures remain to be addressed.
The aim of the present study was to exploit a long-term monitoring data of AD contamination in pharmacies and hospitals in the Czech Republic and Slovakia to propose and discuss practically applicable technical guidance values (TGV). Our research shows that different TGVs may be relevant for different specific areas and places within health care facilities, and we discuss three categories. First, the strongly controlled areas where ADs are prepared (AD preparatory rooms). Second, other places in hospitals and pharmacies, where basic personal protective equipment is used such as storage, transport, administration to patients. Third, places expected to be without major contamination such as offices, daily rooms or kitchenettes. The TGVs derived in the present study support evidence-based and tailored risk management as well as benchmarking of surface AD contamination.
2. Materials and methods
2.1. Material
Analytical standards and solvents were obtained from Toronto Research Chemicals (TRC) or Sigma-Aldrich, British Pharmacopeia Chemical Reference Substances (BPCRS), Analytika (Czech Republic), Merck, and Biosolve BV. More details are provided in Supplementary material. Quality control sample for validation of extraction was prepared in methanol. Field blanks were regularly provided by participating hospitals.
2.2. Methods
2.2.1. Design of the monitoring programme
Monitoring program in the Czech Republic runs since 2008 with Slovak Republic added since 2018. It is organized in campaigns two times per year by RECETOX Center at Masaryk University. As of 11/05/2021 (November) the database used for the present paper contained total N = 9190 analyses (data points) covering period 2008–2021. This represented N = 2,223 unique samples, collected repeatedly in N = 48 different pharmacies and/or hospitals. During 2008–2014 only CP and Pt contamination was measured. In 2015, monitoring was further extended with FU, and since 2018–2019 eight ADs (Pt, CP, FU, PX, IF, IRI, GEM, MET) are covered in our monitoring with validated sampling and analytical procedures (21).
Hospitals and pharmacies are invited to voluntarily participate in monitoring, the costs are jointly covered by health care facilities and research grant projects of RECETOX. Participants are provided with standardized sampling kits (described below) and organize own wipe-sampling of surfaces according to the instructions and video manual (https://muni.cz/go/e00d53). Sampling is recommended at the end of a working day or before the next shift, usually before routine daily cleaning in hospitals but the actual sampling strategies reflect needs and decisions of individual participants. The collected wipe-samples are shipped by courier to RECETOX laboratories being responsible for further sample processing, instrumental measurements, and data analyses. The results from each campaign are provided to individual participant, and the participant data are compared with the overall statistics of the annual monitoring. This allows detailed comparing (ranking) of individual hospital/pharmacy within national-wide data. The AD handling procedures at various participants follow generic regulatory recommendations but they cannot be fully harmonized with respect to specific hygiene protocols in different health care providers in Czechia and Slovakia.
2.2.2. Wipe sampling and sample extraction
Surface wipe samples were obtained by standardized procedure (10, 21, 41). Surfaces samples from the pre-marked spots (30 × 30 cm) were obtained with moistened swabs (20 mM acetate buffer, pH 4) and stored at −20°C until extraction. The area of irregular surfaces (such as handles or phones) that could not be marked was calculated after dividing it into regular shapes (e.g., triangles, rectangles, circles) followed by summing up of individual areas. Field blanks (only moistened swab) and quality controls (swab spiked with quality control mixture; CP 3.6 ng/mL, Pt 3.6 ng/mL, FU 7.2 ng/mL, and PX 4.6 ng/mL) were extracted by sonication (45 min; 25 mL of 20 mM acetate buffer pH 4), centrifuged, and the supernatant was used for analyses of organic ADs by LC-MS/MS. For Pt, 0.4 mL aliquot of the supernatant was diluted with 2 ml of 3% hydrochloric acid and analyzed by ICP-MS.
The recoveries of the wipe and extraction procedures from different surfaces were validated in our previous studies (21, 41). Briefly, for CP, Pt, FU mean recovery for all tested surfaces was > 90%. For other monitored compounds - PX, IF, GEM, IRI, MET - mean recoveries were 80, 94, 94, 96, 47%, respectively, for the stainless-steel surface, and 67, 92, 88, 47, 26 %, respectively, for the benchtop material (see Supplementary Table 3 for details).
2.2.3. Instrumental analyses of ADs
Liquid chromatography/tandem mass spectrometry, LC-MS/MS Agilent 1200 coupled with Agilent 6410 Triple-Quad MS was used for analyses of CP between 2008 and 2015 (21). Since 2015, Waters Acquity LC chromatograph (Waters, Manchester, UK) and Xevo TQ-S quadrupole mass spectrometer (Waters, Manchester, UK) were used for multitarget analyses of cyclophosphamide CP, 5-fluorouracil FU, paclitaxel PX, irinotecan IRI, ifosphamide IF, methotrexate MET, and gemcitabine GEM using a recently described multitarget method (41). Analytes were detected in both positive and negative ion modes using tandem mass spectrometry. Settled parameters – i.e., collision energy, cone voltage, retention time as well as the lower limit of quantification, LLOQ, the lowest amount of analyte taken from a known area – 900 cm2 - in the sample matrix that can be repeatedly quantified (the signal to noise ratio > 10) are presented in Supplementary Table 1. Data were processed by MassLynxTM software (Waters, Manchester, U.K) and corrected to isotopically labeled standards (CP D4; FU 15N2 13C; PX D5; IRI D10; GEM 13C15N2; MET D3). The results of contamination were reported as picograms of AD per square centimeter of the tested surface (pg/cm2).
Inductively coupled plasma/mass spectrometry, ICP-MS used Agilent 7500ce or 7700x ICP-MS systems (Agilent Technologies Inc., Japan) for the analyses of total Pt concentration as a marker of Pt-based ADs (21, 41). Quantification was based on external calibration (194Pt and 195Pt isotopes) with the correction of signal drift and non-spectral interferences on internal standards (185Rh and 209Bi). Results are reported as pg of Pt per square centimeter of surface.
Although the sensitivity of the measurements of long-monitored substances (such as CP and Pt) improved during years because of new instruments, we decided to use the originally derived limits of quantification throughout the present study. This allowed us to assure consistency when comparing frequencies of contamination.
2.2.4. Data analyses
The analyses were done in Microsoft Excel and GraphPad (Boston, MA, USA) and included stratification of original contamination data into categories based on different places of sampling followed by visualization and calculation of basic statistics such as mean, median, min-max, standard deviation, etc.
3. Results and discussion
The present study investigated surface contamination in 40 pharmacies (N = 1,277 samples) and 43 hospitals (N = 946). In addition to this data set, monitoring covered also 17 patient homes (N = 133), three retirement houses (N = 19), and 2 hospices (N = 10) (9) but the data are not considered in the present paper.
From total N = 2,223 samples collected in hospitals and pharmacies (field blanks excluded), the most frequently sampled areas were desktops/tables and shelfs (N = 1,025) and floors (N = 716). Other types of collected samples included interiors of the BSCs, touch displays, handles, fridge doors, outpatient clinic chairs, phones, toilets, etc. The number of yearly AD preparations in participating hospitals varied and hospitals were categorized according to final report of European Commission (42). The monitoring covered small hospital units without own preparation of ADs (N = 5), hospitals with low number AD preparations per year (max 5 000; N = 17), medium size hospitals with max 15,000 preparation per year (N = 8) and large specialized oncology centers (N = 18) preparing between 15 000 – 58 000 applications of ADs per year.
The most frequently prepared drugs during 2018–2019 were FU (3 300 preparations per year, median within large specialized oncology hospitals), Pt based drugs (median 2 800 preparations), PX (median 1 004), CP (median 936), GEM (median 732), IRI (median 660), IF and MET (both median of 120 preparations per year) (For detail see Supplementary Table 2).
Table 1 and Figure 1 show occurrence and contamination by six ADs, i.e., CP and Pt (covering years 2008–2021), FU (2015–2021) and IF, GEM and PX (2018, 2019–2021). The two ADs included in our monitoring - IRI and MET (since 2018) - were only rarely detected with generally low concentrations (Table 1), and they were excluded from follow-up data analyses.
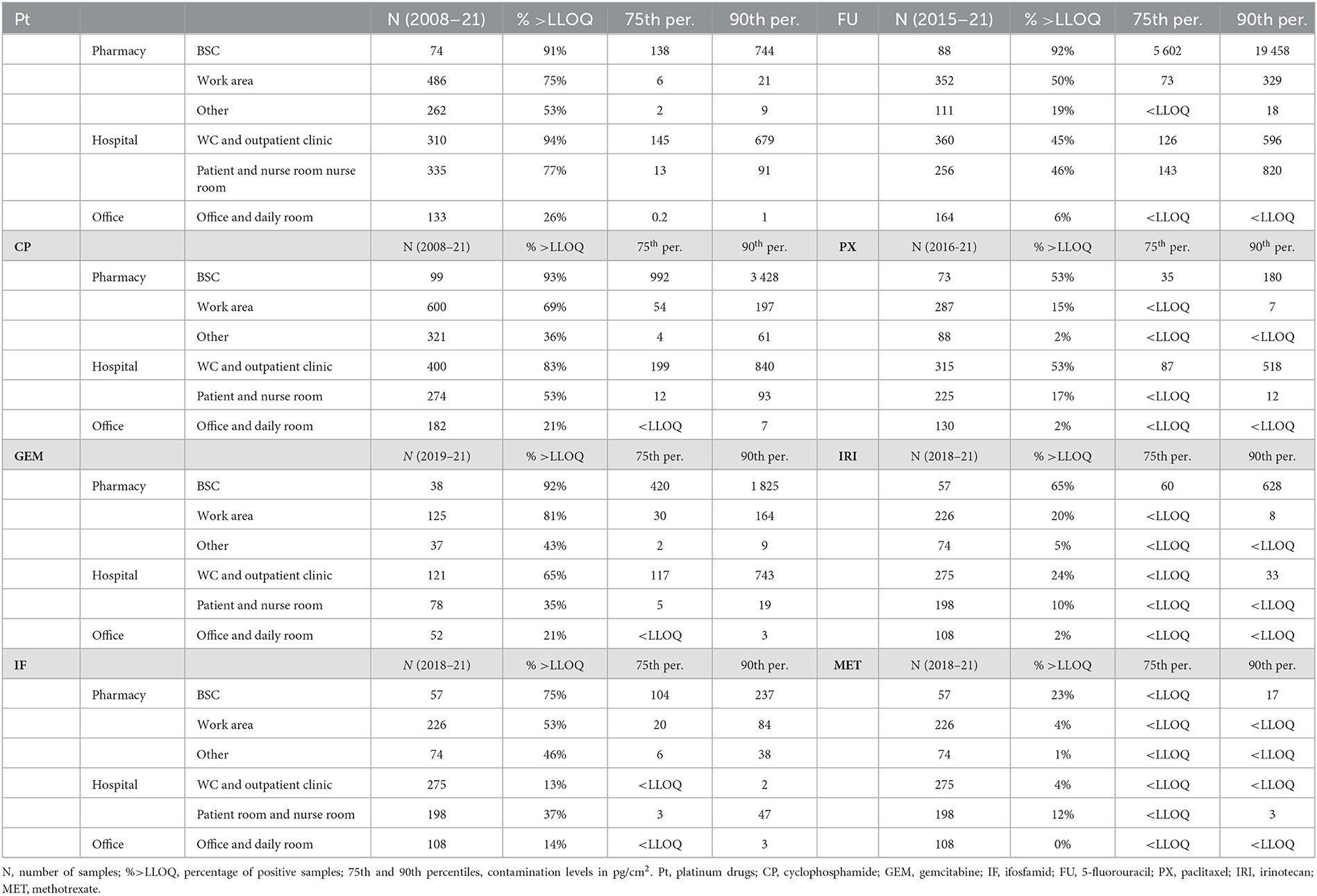
Table 1. Contamination of different areas (pharmacies, hospital, offices) and specific sites by six ADs in the Czech Republic.
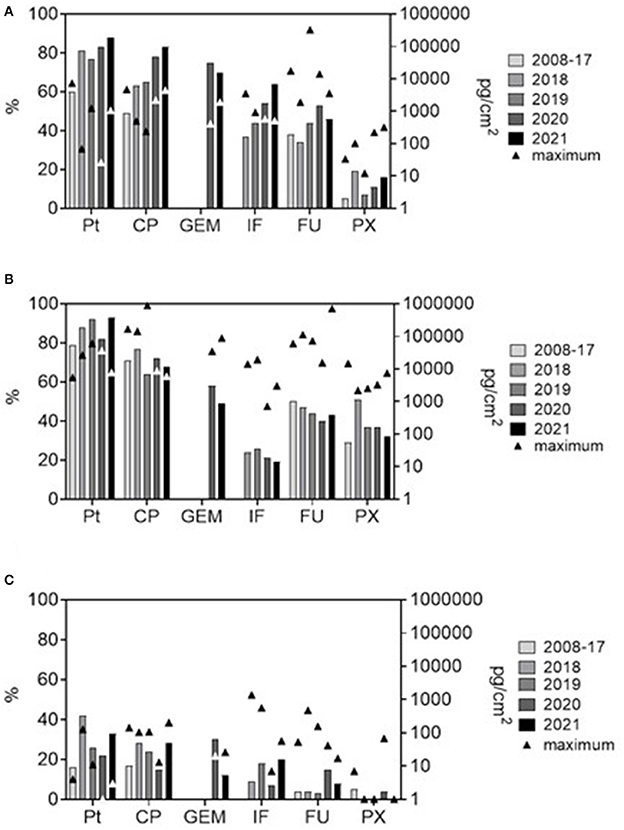
Figure 1. Frequencies of positive samples (%, bars; left Y-axis) and maximum contamination levels (pg/cm2, triangle symbols; right Y axis, log scale) for six antineoplastic drugs from the long-term monitoring. (A) pharmacies (excluding internal contamination of biological safety cabinets), (B) hospital areas (outpatient clinics and patient rooms), and (C) offices (offices, staff daily rooms, kitchenettes etc).
The data were first categorized by main areas with different working regimes (pharmacies, hospital patient areas, offices), and specific sites within these areas. Figure 1 shows the trends in contamination during the years. In Figure 1, specific sites within an area (i.e., within pharmacy and within hospital) were pooled for simplicity, and the most recent situation is highlighted (data collected during early 2008–2017 years are pooled and compared with individual years 2018, 2019, 2020, and 2021).
As apparent, Table 1 clearly shows that ADs were most frequently detected (and often in high concentrations – see 75th and 90th percentile concentrations in Table 1) on surfaces within interiors of BSCs where ADs are being openly handled and prepared for patients. This is in an agreement with other recent studies, where the highest obtained concentrations (up to 9.27 ng/cm2 of FU) in BSCs were reported by Sottani et al. (23). Comparably, in Canadian study, maximum contaminations were observed on the floor in front of the BSC (CP up to 120 ng/cm2) (19). BSCs thus may serve as an important source of contamination for other hospital areas. Namely in situations when cleaning staff is not well-trained and may spread the contamination from BSCs (23). However, under standard conditions, BSCs are likely to pose lower occupational risk because they are closed under-pressure systems, which minimizes potential impact on pharmacy staff, which is commonly well educated and uses extensive personal protective equipment. Contamination of BSCs thus represents a separate issue with respect to exposure scenario, and data of BSCs contamination were excluded and not used for further discussions of TGVs in hospitals.
As predicted, the results clearly showed that areas, where AD contamination should be virtually avoided (offices, kitchenettes, daily rooms) were, indeed, generally less contaminated. In the offices and related areas, only about 20% of samples were positive for few ADs such as Pt, CP and GEM (see Table 1).
Nevertheless, the overall frequencies of occurrence (Table 1) in pharmacies (BSCs excluded) and hospitals were comparable for most ADs and showed high detection rates namely for Pt, CP, GEM and FU (with overall more than 50% of samples positive). Percent positivity (i.e., % above LLOQ) is a useful parameter to characterize contamination, namely when LLOQs of the analytes are within the same range (43), which was the case also in the present study (see details on LC-MS/MS method in Supplementary Table 1). High positivity in our monitoring is comparable to another recent study from Italy that showed 44% positives in pharmacies and 59% in patient care units for CP, FU, GEM and Pt (23). Importance of carcinogenic CP as a major indicator of surface contamination is confirmed also in recent studies from Canadian hospitals (19) or France (44).
Importantly, our data showed differing time trends. While apparent declines in % positives over the time were observed in hospitals (Figure 1B), there was an opposite trend of increasing positivity in pharmacies (Figure 1A). Further, there were specific differences between hospitals and pharmacies for PX (higher % positive in hospitals) or IF (more frequently found in pharmacies; Table 1). Although decreasing contamination might be expected with regards to long term recognition of the problem and implementation of remedial measures (19, 28, 32, 39), this is not generally confirmed in all reports. Similarly to the present long-term study, variable and non-systematic trends were also reported for FU contamination in Italian hospitals and pharmacies (23) or for GEM, CP and PX in oncology centers in Canada (43). This variability could be related to complexity of health care services including factors such as workload, cleaning regime, national regulatory requirements, solubility of individual drugs, their metabolization or degradation, etc. (https://ec.europa.eu/social/main.jsp?langId=en&catId=89&newsId=10564&furtherNews=yes&).
As a next step, we analyzed data from 2018–2021 to capture the most recent contamination, and, correspondingly, to derive TGVs reflecting the current situation.
Considering different levels of protection of staff in pharmacies and hospitals, areas were categorized into three groups. First, (i) the AD preparation areas, i.e., the isolated room within a pharmacy, where ADs are being prepared for patients, and staff is well protected (usage of whole body coveralls, goggles, face masks and durable gloves). As described above, data on the inner contamination of BSC were excluded. Second, (ii) other AD handling areas such as delivery and storage areas, dispatch rooms in pharmacies as well as outpatient clinics or patient rooms in hospitals including toilets. Within this second category, certain level of staff protection is usually required and used, typically medical gloves. The third category were (iii) the offices, daily rooms, kitchenettes etc., where workers do not use any protective equipment.
Figure 2 presents the aggregated 2018–2021 data of contamination, and several generic conclusions could be derived. First, the contamination in areas (i) AD preparation and (ii) other AD handling does not substantially differ, the ranges of contamination for most ADs overlap, the 25th-75th quantile range is between 1 and 100 pg/cm2. Some specific differences, such as higher PX contamination in hospitals, were discussed above. For the category (iii) offices, contamination was lower with maxima exceptionally exceeding 100 pg/cm2. Nevertheless, data revealed AD contamination even in these areas that are used by completely unprotected staff, and periodic monitoring should be recommended to check potential exposures. Any contamination in this category (iii) offices (i.e., surface concentrations above LLOQ) should call for case by case examination and implementation of corresponding measures. Overall, this analysis shows that separate technical guidance values (TGVs) might relevant for different areas corresponding to different exposure scenarios of workers.
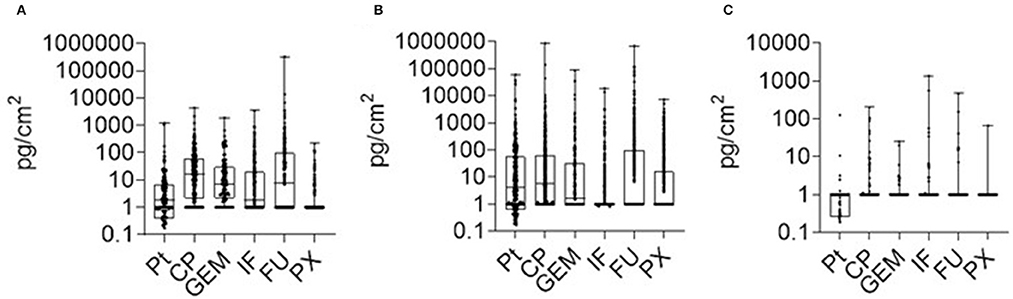
Figure 2. AD contamination (2018–2021) in three categories of areas within health care facilities. (A) preparation AD areas (inner parts of BSC excluded); (B) other AD handling areas, (C) offices and daily rooms. Data show median (line) with 25–75 percentile range (box) and minimum-maximum range.
With regards to previously derived TGVs, authors used different approaches but a value of 100 pg/cm2 (0.1 ng/cm2) was repeatedly suggested (20, 31, 32, 39, 40). Table 2 shows the comparison of this threshold with the contamination of (i) AD preparation and (ii) AD handling areas in Czechia and Slovakia. In Table 2, data are additionally categorized to tables and working desktops (i.e., spots commonly touched by hands, i.e., higher risk for workers), and the floors (lower risk of direct contact for most of the workers). The exceedance of 0.1 ng/cm2 threshold ranged between 2% of samples from all surfaces (see Pt in category (i) AD preparatory rooms) to 25% exceedance for FU in both (i) AD preparatory and (ii) other AD handling areas. The most frequent exceedances were – in both categories of areas – observed at FU followed by CP and PX. More specifically, within the (i) AD preparatory rooms (upper part of the table), the most contaminated were packaging desktops and transfer carriages (FU and CP followed by GEM and IF). On the contrary, in the (ii) other AD handling areas, threshold was mostly exceeded on the floors, specifically under the administration IV poles in outpatient clinics and around the toilets (FU and CP followed by Pt, GEM and PX).
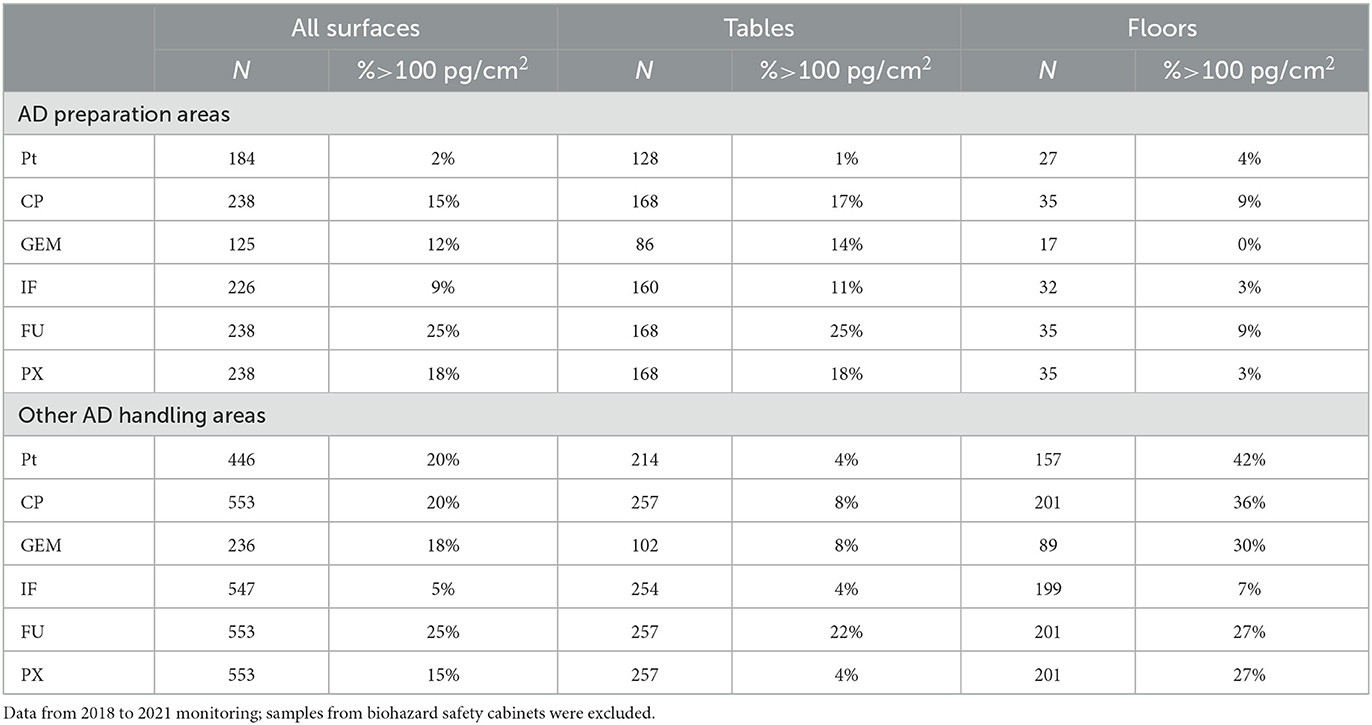
Table 2. Exceedance of 100 pg/cm2 threshold originally suggested for CP by Sessink (39) in hospital and pharmacy samples in (i) AD preparation areas, and (ii) other AD handling and drug administration areas.
Another derivation of TGVs considers statistical analyses and percentiles based on monitoring data. The exceedance of certain value, such as 90th percentile, indicates that the sample is among the top 10% highest contaminated, which calls for immediate investigation and remedial actions. From the management perspective, a single TGV (75th, 90th or 95th percentile) is another approach and two TGV levels were also discussed in the literature. For example, Schierl et al. (27) reported monitoring of 102 pharmacies in Germany and proposed that contamination of FU and Pt below the 50th percentile indicates a good working practice, while the values higher than 75th percentile called for adaptation of working procedures.
Detailed analysis of percentiles of our monitoring data is shown in Figure 3 and Table 3. The 90th percentile for all ADs was found to be highly variable in different years which is expected for higher percentiles (e.g., compare Figure 3), while the 75th percentile was more stable in time, and, it thus appeared to be more suitable for derivation of a threshold for the workers and their possible exposure to ADs.
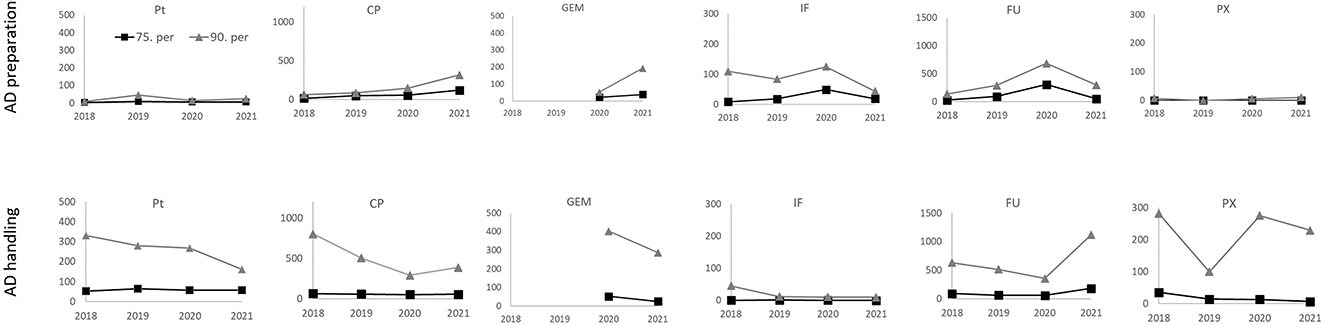
Figure 3. Surface contamination (pg/cm2; 75th and 90th percentiles) by 6 antineoplastic drugs from the 2018–2021 monitoring. Results for two categories of areas with differing protection level, i.e., (i) AD preparation areas - upper panels, and (ii) other AD handling areas - lower panels. Results for biological safety cabinets, BSCs, are excluded.
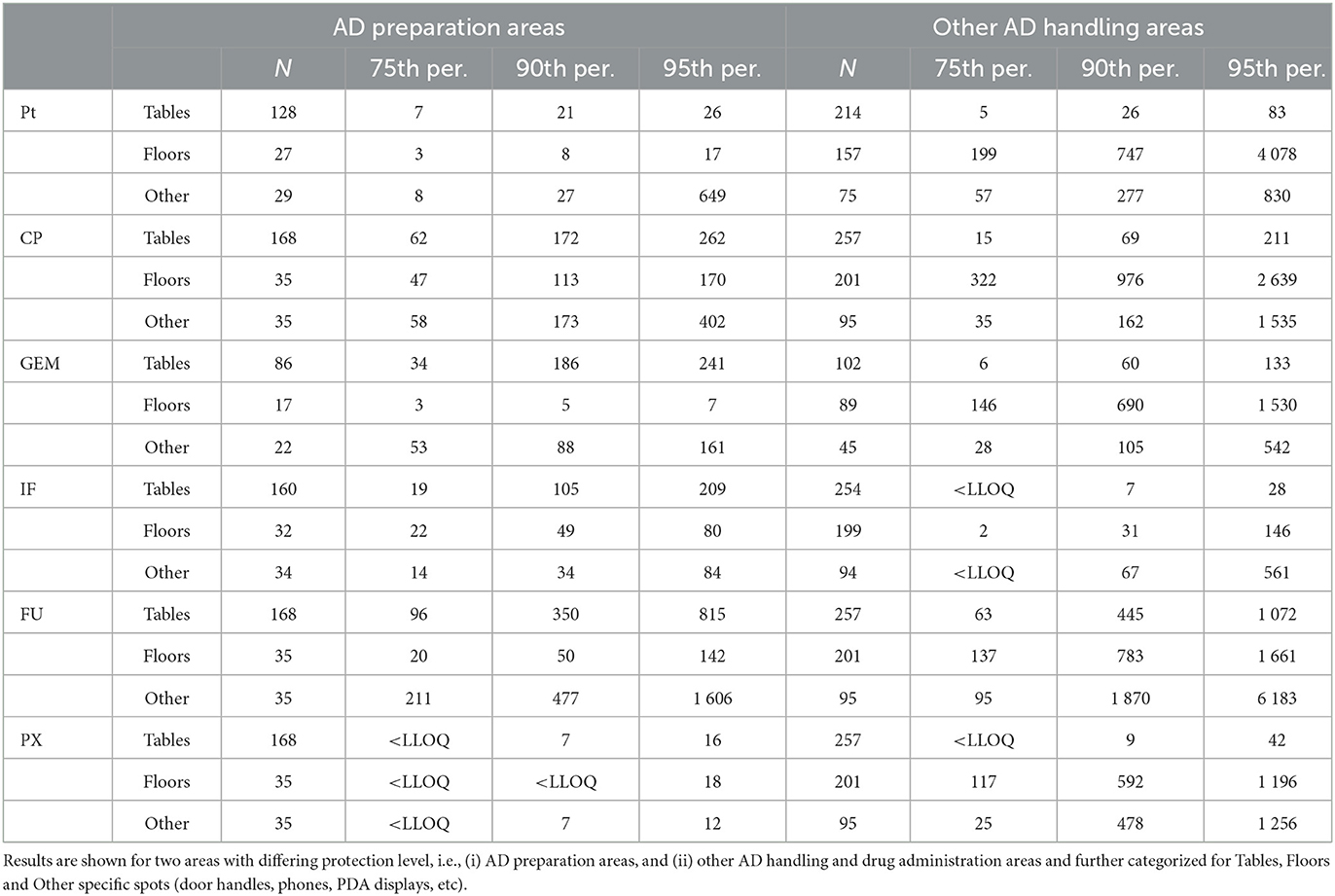
Table 3. Statistics for surface contamination (pg/cm2) by six antineoplastic drugs from the 2018–2021 monitoring.
Detailed statistics (Table 3) show that within the (i) AD preparatory rooms, the 75th percentile was in most cases below the suggested 100 pg/cm2. For the second category - (ii) other AD handling areas - contamination of floors was higher with the 75th percentiles exceeding the 100 pg/cm2. Desktops/tables and “other” spots (such as door handles) had lower 75th percentiles ranging from <LLOQ to 95 pg/cm2 for all six ADs. Similar observations of higher floor contamination with 75th percentiles exceeding the 100 pg/cm2 threshold were also reported by other authors such as Hedmer et al. (37) for CP and IF contamination in Sweden or Labrèche et al. (38) for FU in Canada. Similar conclusions were recently published by Dugheri et al. (45) who observed higher floor contamination (compared to desktops), and suggested the new surface exposure level of 100 pg/cm2 (with the exception of bathrooms).
Although the floor contamination by ADs is high, direct exposures via skin contact for most of the health care workers is less likely (37). However, this route of exposure is of specific concern for hospital cleaning staff (38), which should be properly trained how to remain protected, and how to avoid spread of ADs from highly contaminated places such as floors or interiors of biosafety cabinets (44). Although decreasing of the floor contamination may be theoretically achievable, e.g., by repeated applications of strong oxidation cleaning products (41), it is challenging and highly demanding considering common hospital practices.
Finally, a potential effort how to better protect health care workers might be using of TGVs that are annually updated based on periodic contamination monitoring. These could further be “tailored” for different places (e.g., floors vs. desktops) or different ADs (AD-specific TGV). Correspondingly, our study suggests that TGVs for floors should be higher than 100 pg/cm2 for some ADs so it can be realistically achieved. Such a detailed approach is, however, not very practical for regular hygiene management as many different trigger values might bring uncertainty and confusion. Having one TGV is further supported from our monitoring data, where the 75th percentile for two most important contaminants (i.e., CP and FU) was sufficiently high to serve also as a trigger for other ADs, i.e., Pt, PX, IF and GEM.
4. Conclusions
The thorough analysis of the long-term monitoring data of AD contamination in Czech and Slovak hospitals revealed following conclusions and recommendations summarized also in Figure 4.
First, it confirmed high relevance of traditional exposure biomarkers such as CP and FU (19). Especially, CP is frequently detected in high concentrations, it is persistent on surfaces (41, 46, 47), and represents a long-term concern considering its carcinogenicity (https://eur-lex.europa.eu/legal-content/EN/TXT/?uri=OJ:L:2022:088:TOC).
Second, highly contaminated spots, namely interiors of laminar biological safety cabinets (BSC) or flow boxes but also contaminated floors represent a major and separate issue. This should be specifically handled by implementing careful cleaning procedures that are separated from other areas preventing thus potential spread of AD contamination. Cleaning and prevention are priority, and monitoring of AD contamination in BSC interiors does not bring much added value, it might be recommended only case by case.
Third, hospital and pharmacy areas that should be virtually free of AD contamination, i.e., offices, kitchenettes, daily rooms, etc., are indeed less contaminated. However, staff is usually not protected in these areas at all, and periodic monitoring should be performed. Any positive contamination by ADs (i.e., samples >LLOQ) should call for immediate examination and adaptation of preventive measures.
Fourth, for the areas in pharmacies and hospitals, where ADs are being prepared, stored, transported and administered to patients, periodic monitoring is needed. A single value of 100 pg/cm2 could be suggested as a generic TGV based on the long-term monitoring data of many studies. For most ADs and most exposure situations, this value is close to the 75th percentile (the samples with contamination >100 pg/cm2 are among the top 25% contaminated). A TGV of 100 pg/cm2 is thus a “warning” or “trigger” value that calls for investigation and improvement of practices, which may be considered during the implementation of new regulations such as the EU Directive 2022/431.
In conclusion, long-life exposures of health care staff to ADs represent a major issue, and routine monitoring along with implementation of proper measures help to implement the “as low as reasonably achievable” principle (ALARA) (12) minimizing thus occupational risks. Challenging problems that require research attention are the take-home anticancer therapies (48), veterinary clinics or research facilities that might contribute to spread of AD contamination to other environments such as patient homes (9).
Data availability statement
The raw data supporting the conclusions of this article will be made available by the authors, without undue reservation.
Author contributions
LBlaho contributed to the study design, validated methods, performed analyses of organic drugs, collected data, performed statistical analyses, and drafted the manuscript. JK developed methods, analyzed PT in studied samples, and contributed to manuscript writing. LD contributed to the design of the study, sampling, and manuscript writing. TH contributed to sample extractions, analyses, and to manuscript writing. LBlaho contributed to the study design, method development, data processing, interpretation, and manuscript writing. All authors contributed to the article and approved the submitted version.
Funding
The results of the project were created with the financial support of the provider Ministry of Health of the Czech Republic, within the project no. NV18–09–00188. Authors thank the RECETOX Research Infrastructure (No. LM2018121) financed by the Ministry of Education, Youth and Sports, and the Operational Programme Research, Development and Education (the CETOCOEN EXCELLENCE project no. CZ.02.1.01/0.0/0.0/17_043/0009632) for supportive background. This work was also supported from the European Union's Horizon 2020 research and innovation program under grant agreement no. 857560.
Acknowledgments
Authors wish to thank to partners from cooperating pharmacies and hospitals for sharing of samples and information, to Roswita Lišková (RECETOX) for technical support during processing of monitoring samples and to Dr. Šárka Kozáková (University Hospital Brno) for expert advises and thorough support to monitoring programme.
Conflict of interest
The authors declare that the research was conducted in the absence of any commercial or financial relationships that could be construed as a potential conflict of interest.
Publisher's note
All claims expressed in this article are solely those of the authors and do not necessarily represent those of their affiliated organizations, or those of the publisher, the editors and the reviewers. Any product that may be evaluated in this article, or claim that may be made by its manufacturer, is not guaranteed or endorsed by the publisher.
Author disclaimer
This publication reflects only the author's view and the European Commission is not responsible for any use that may be made of the information it contains.
Supplementary material
The Supplementary Material for this article can be found online at: https://www.frontiersin.org/articles/10.3389/fpubh.2023.1235496/full#supplementary-material
Footnotes
1. ^https://eur-lex.europa.eu/eli/dir/2022/431/oj
2. ^https://osha.europa.eu/en/publications/guidance-safe-management-hazardous-medicinal-products-work
3. ^https://www.europeanbiosafetynetwork.eu/wp-content/uploads/2022/10/The-ETUIs-list-of-hazardous-medicinal-products-HMPs_2022.pdf
4. ^https://echa.europa.eu/carcinogens-mutagens-oels
5. ^https://www.europeanbiosafetynetwork.eu/wp-content/uploads/2019/03/Amendments-to-CMD3-and-implications-1.pdf
References
1. Kümmerer K, Haiß A, Schuster A, Hein A, Ebert I. Antineoplastic compounds in the environment—substances of special concern. EnvironSci Pollut Res. (2016) 23:14791–14804. doi: 10.1007/s11356-014-3902-8
2. Sung H, Ferlay J, Siegel RL, Laversanne M, Soerjomataram I, Jemal A, et al. and Bray F. Global cancer statistics 2020: GLOBOCAN estimates of incidence and mortality worldwide for 36 cancers in 185 countries. CA Cancer J Clin. (2021) 71:209–49. doi: 10.3322/caac.21660
3. Alexander M, Connor TH, Bauters T, Alexander MC, Mackenzie BA, Vandenbroucke J, et al. ISOPP standards for the safe handling of cytotoxics. J Oncol Pharm Pract. (2022) 28:S1–S126. doi: 10.1177/10781552211070933
4. Cherrie JW, Hutchings S, Gorman Ng M, Mistry R, Corden C, Lamb J, et al. Prioritising action on occupational carcinogens in Europe: a socioeconomic and health impact assessment. Br J Cancer. (2017) 117:274–81. doi: 10.1038/bjc.2017.161
5. Valanis BG, Vollmer WM, Labuhn KT, Glass AG. Acute symptoms associated with antineoplastic drug handling among nurses. Cancer Nurs. (1993) 16:288–95. doi: 10.1097/00002820-199308000-00005
6. Krstev S, Perunicic B, Vidakovic A. Work practice and some adverse health effects in nurses handling antineoplastic drugs. Med Lav. (2003) 94:432–9.
7. Hon CY, Chua PPS, Danyluk Q, Astrakianakis G. Examining factors that influence the effectiveness of cleaning antineoplastic drugs from drug preparation surfaces: a pilot study. J Oncol Pharm Pract. (2014) 20:210–216. doi: 10.1177/1078155213497070
8. Decree 84/2008 Coll. Vyhlaska o spravne lekarenske praxi, blizsích podminkach zachazeni s lecivy v lekarnach, zdravotnickych zarizenich a u dalsich provozovatelu a zarizeni vydavajicich lecive pripravky. Vyhlaska c. 84/2008 Sb. [in Czech] [Good pharmaceutical practice drugs handling conditions for pharmacies, healthcare facilities other entities emitting pharmaceutical products to final consumers; Decree 84/2008 Coll.] (2008). Available online at: https://www.psp.cz/sqw/sbirka.sqw?cz=84&r=2008
9. Bláhová L, Kuta J, DoleŽalová L, Kozáková Š, Hojdarová T, Bláha L, et al. Levels and risks of antineoplastic drugs in households of oncology patients, hospices and retirement homes. Environ Sci Eur. (2021) 33:1–14. doi: 10.1186/s12302-021-00544-5
10. Doležalová L, Bláhová L, Kuta J, Hojdarová T, Kozáková Š, Bláha L, et al. Levels and risks of surface contamination by thirteen antineoplastic drugs in the Czech and Slovak hospitals and pharmacies. Environ Sci Pollut Res. (2022) 29:26810–9. doi: 10.1007/s11356-021-17607-y
11. NIOSH. Managing Hazardous Drug Exposures: Information for Healthcare Settings. Cincinnati, OH: U.S. Department of Health and Human Services, Centers for Disease Control and Prevention, National Institute for Occupational Safety and Health, DHHS (NIOSH) Publication No. 2023–130. (2023).
12. Mathias PI, MacKenzie BA, Toennis CA, Connor TH. Survey of guidelines and current practices for safe handling of antineoplastic and other hazardous drugs used in 24 countries. J Oncol Pharm Pract. (2019) 25:148–62. doi: 10.1177/1078155217726160
13. Fransman W, Huizer D, Tuerk J, Kromhout H. Inhalation and dermal exposure to eight antineoplastic drugs in an industrial laundry facility. Int Arch Occup Environ Health. (2007) 80:396–403. doi: 10.1007/s00420-006-0148-x
14. Santos AN, Oliveira RJ, Pessatto LR, Gomes R, Freitas CAF. Biomonitoring of pharmacists and nurses at occupational risk from handling antineoplastic agents. Int J Pharm Pract. (2020) 28:506–511. doi: 10.1111/ijpp.12590
15. Turci R, Sottani C, Spagnoli G, Minoia C. Biological and environmental monitoring of hospital personnel exposed to antineoplastic agents: a review of analytical methods. J Chromatogr B. (2003) 789:169–209. doi: 10.1016/S1570-0232(03)00100-4
16. Villa A, Tremolet K, Martinez B, Petit M, Dascon X, Stanek J, et al. Urine biomonitoring of occupational exposure to methotrexate using a highly sensitive uhplc-ms/ms method in mrm3 mode. SSRN Electron J. (2022) 1209:1–8. doi: 10.1016/j.jchromb.2022.123411
17. Villa A, Molimard M, Sakr D, Lassalle R, Bignon E, Martinez B, et al. Nurses' internal contamination by antineoplastic drugs in hospital centers: a cross-sectional descriptive study. Int Arch Occup Environ Health. (2021) 94:1839–50. doi: 10.1007/s00420-021-01706-x
18. Yuki M, Sekine S, Takase K, Ishida T, Sessink PJM. Exposure of family members to antineoplastic drugs via excreta of treated cancer patients. J Oncol Pharm Pract. (2013) 19:208–17. doi: 10.1177/1078155212459667
19. Chabut C, Tanguay C, Gagné S, Caron N, Bussières JF. Surface contamination with nine antineoplastic drugs in 109 canadian centers; 10 years of a monitoring program. J. Oncol. Pharm. Pract. (2021) 28:343–352. doi: 10.1177/1078155221992103
20. Connor TH, Zock MD, Snow AH. Surface wipe sampling for antineoplastic (chemotherapy) and other hazardous drug residue in healthcare settings: Methodology and recommendations. J Occup Environ Hyg. (2016) 13:658–67. doi: 10.1080/15459624.2016.1165912
21. Odraska P, Dolezalova Pharm D, Kuta L, Oravec J, Piler M, Synek Pharm P, et al. Association of surface contamination by antineoplastic drugs with different working conditions in hospital pharmacies association of surface contamination by antineoplastic drugs with different working conditions in hospital pharmacies. Arch Environ Occup Health. (2014) 693:148–58. doi: 10.1080/19338244.2013.763757
22. Palamini M, Gagné S, Caron N, Bussières JF. Cross-sectional evaluation of surface contamination with 9 antineoplastic drugs in 93 Canadian healthcare centers: 2019 results. J. Oncol. Pharm. Pract. (2020) 26:1921–1930. doi: 10.1177/1078155220907125
23. Sottani C, Grignani E, Cornacchia M, Negri S, Saverio F, Cottica D, et al. Occupational exposure assessment to antineoplastic drugs in nine italian hospital centers over a 5-year survey program. Int J Environ Res Public Health. (2022) 19:8601–18. doi: 10.3390/ijerph19148601
24. Jeronimo M, Colombo M, Astrakianakis G, Hon CY. A surface wipe sampling and LC-MS/MS method for the simultaneous detection of six antineoplastic drugs commonly handled by healthcare workers. Anal Bioanal Chem. (2015) 407:7083–92. doi: 10.1007/s00216-015-8868-y
25. Kopp B, Schierl R, Nowak D. Evaluation of working practices and surface contamination with antineoplastic drugs in outpatient oncology health care settings. Int Arch Occup Environ Health. (2013) 86:47–55. doi: 10.1007/s00420-012-0742-z
26. Astrakianakis G, Jeronimo M, Griffiths A, Colombo M, Kramer D, Demers PA, et al. The application of novel field measurement and field evaluation protocols for assessing health care workers' exposure risk to antineoplastic drugs. J Occup Environ Hyg. (2020) 17:373–82. doi: 10.1080/15459624.2020.1777296
27. Schierl R, Böhlandt A, Nowak D. Guidance values for surface monitoring of antineoplastic drugs in german pharmacies. Ann Occup Hyg. (2009) 53:703–11. doi: 10.1093/annhyg/mep050
28. Blahova L, Dolezalova L., Kuta J., Kozáková Š., Bláha L. (2020). Hospitals and Pharmacies as Sources of Contamination by Cytostatic Pharmaceuticals: Long-Term Monitoring in the Czech Republic, in:Heath, E., Isidori, M., Kosjek, T., Filipič M., (,Eds.), Fate and Effects of Anticancer Drugs in the Environment. Springer Nature Switzerland AG, pp. 57–70. doi: 10.1007/978-3-030-21048-9_3
29. Chauchat L, Tanguay C, Caron N, Gagné S, Labrèche F, Bussières J, et al. Surface contamination with ten antineoplastic drugs in 83 Canadian centers. J Oncol Pharm Pract. (2019) 25:1089–1098. doi: 10.1177/1078155218773862
30. Fleury-Souverain S, Mattiuzzo M, Mehl F, Nussbaumer S, Bouchoud L, Falaschi L, et al. Evaluation of chemical contamination of surfaces during the preparation of chemotherapies in 24 hospital pharmacies. Eur J Hosp Pharm. (2015) 22:333–41. doi: 10.1136/ejhpharm-2014-000549
31. Kiffmeyer TK, Tuerk J, Hahn M, Stuetzer H, Hadtstein C, Heinemann A, et al. Application and assessment of a regular environmental monitoring of the antineoplastic drug contamination level in pharmacies-the MEWIP project. Ann Occup Hyg. (2013) 57:444–55. doi: 10.1093/annhyg/mes081
32. Korczowska E, Crul M, Tuerk J, Meier K. Environmental contamination with cytotoxic drugs in 15 hospitals from 11 European countries—results of the MASHA project. Eur J Oncol Pharm. (2020) 3:e24. doi: 10.1097/OP9.0000000000000024
33. Roland C, Caron N, Bussières JF. Multicenter study of environmental contamination with cyclophosphamide, ifosfamide, and methotrexate in 66 canadian hospitals: a 2016 follow-up study. J Occup Environ Hyg. (2017) 14:650–8. doi: 10.1080/15459624.2017.1316389
34. Sottani C, Grignani E, Oddone E, Dezza B, Negri S, Villani S, et al. Monitoring surface contamination by antineoplastic drugs in Italian hospitals: Performance-based hygienic guidance values (HGVs) Project. Ann Work Expo Heal. (2017) 61:1–9. doi: 10.1093/annweh/wxx065
35. Kromhout H, Hoek F, Uitterhoeve R, Huijbers R, Overmars RF, Anzion R, et al. Erratum: Postulating a dermal pathway for exposure to anti-neoplastic drugs among hospital workers. Applying a conceptual model to the results of three workplace surveys. Ann Occup Hyg 44. (2000) 551–60. doi: 10.1016/S0003-4878(00)00050-8
36. Power LA, Coyne JW, Hawkins B. ASHP guidelines on handling hazardous drugs. Am J Heal Pharm. (2018) 75:1996–2031. doi: 10.2146/ajhp180564
37. Hedmer M, Wohlfart G. Hygienic guidance values for wipe sampling of antineoplastic drugs in Swedish hospitals. J Environ Monit. (2012) 14:1968–75. doi: 10.1039/c2em10704j
38. Labrèche F, Ouellet C, Roberge B, Caron NJ, Yennek A, Bussières JF, et al. Occupational exposure to antineoplastic drugs: what about hospital sanitation personnel? Int Arch Occup Environ Health. (2021) 94:1877–88. doi: 10.1007/s00420-021-01731-w
39. Sessink PJ. Environmental contamination with cytostatic drugs: past, present and future. Saf Considerations Oncol Pharm Special Ed. (2011) 12:3–5.
40. Crul M, Simons-Sanders K. Carry-over of antineoplastic drug contamination in Dutch hospital pharmacies. J Oncol Pharm Pract. (2018) 24:483–9. doi: 10.1177/1078155217704990
41. Bláhová L, Kuta J, Doležalová L, Kozáková Š, Krovová T, Bláha L, et al. The efficiency of antineoplastic drug contamination removal by widely used disinfectants–laboratory and hospital studies. Int Arch Occup Environ Health. (2021) 24:5. doi: 10.1007/s00420-021-01671-5
42. European Commission Directorate-General for Employment Social Affairs Inclusion Sand, Jespersen M, Lassen C, Madsen P. et al. Study Supporting the Assessment of Different Options Concerning the Protection of Workers from Exposure to Hazardous Medicinal Products, including Cytotoxic Medicinal Products - Final Report, Publications Office (2021). Available online at: https://data.europa.eu/doi/10.2767/17127
43. Jeronimo M, Arnold S, Astrakianakis G, Lyden G, Stewart Q, Petersen A, et al. Spatial and temporal variability in antineoplastic drug surface contamination in cancer care centers in Alberta and Minnesota. Ann Work Expo Heal. (2021) 65:760–74. doi: 10.1093/annweh/wxab013
44. Acramel A, Foquet J, Blondeel-Gomes S, Huguet S, Rezai K, Madar O, et al. Application of an environmental monitoring to assess the practices and control the risk of occupational exposure to cyclophosphamide in two sites of a french comprehensive cancer center. Annal Work Exp Health. (2021) 2:wxac035. doi: 10.1093/annweh/wxac035
45. Dugheri S, Mucci N, Bucaletti E, Squillaci D, Cappelli G, Trevisani L, et al. Monitoring of surface contamination for thirty antineoplastic drugs: a new proposal for surface exposure levels (SELs). Med. Pr. (2022) 73:383–96. doi: 10.13075/mp.5893.01288
46. Adé A, Chauchat L, Frève JFO, Gagné S, Caron N, Bussières JF, et al. Comparison of decontamination efficacy of cleaning solutions on a biological safety cabinet workbench contaminated by cyclophos- phamide. Can J Hosp Pharm. (2017) 70:407–14. doi: 10.4212/cjhp.v70i6.1708
47. Simon N, Odou P, Decaudin B, Bonnabry P, Fleury-Souverain S. Efficiency of degradation or desorption methods in antineoplastic drug decontamination: a critical review. J Oncol Pharm Pract. (2019) 25:929–46. doi: 10.1177/1078155219831427
Keywords: hazardous drugs, surface contamination, antineoplastic drugs, monitoring, technical guidance values
Citation: Bláhová L, Bláha L, Doležalová L, Kuta J and Hojdarová T (2023) Proposals of guidance values for surface contamination by antineoplastic drugs based on long term monitoring in Czech and Slovak hospitals and pharmacies. Front. Public Health 11:1235496. doi: 10.3389/fpubh.2023.1235496
Received: 06 June 2023; Accepted: 22 August 2023;
Published: 14 September 2023.
Edited by:
Susana Viegas, New University of Lisbon, PortugalReviewed by:
Antonio Baldassarre, University of Florence, ItalyMatteo Creta, National Health Laboratory, Luxembourg
Cristina Sottani, Scientific Clinical Institute Maugeri (ICS Maugeri), Italy
Copyright © 2023 Bláhová, Bláha, Doležalová, Kuta and Hojdarová. This is an open-access article distributed under the terms of the Creative Commons Attribution License (CC BY). The use, distribution or reproduction in other forums is permitted, provided the original author(s) and the copyright owner(s) are credited and that the original publication in this journal is cited, in accordance with accepted academic practice. No use, distribution or reproduction is permitted which does not comply with these terms.
*Correspondence: Luděk Bláha, blaha@recetox.muni.cz