- 1Sawyer Business School, Suffolk University, Boston, MA, United States
- 2California Environmental Protection Agency, Office of Environmental Health Hazard Assessment, Oakland, CA, United States
- 3Institute for Health and Aging, University of California, San Francisco, San Francisco, CA, United States
Evidence linking temperature with adverse perinatal and pregnancy outcomes is emerging. We searched for literature published until 30 January 2023 in PubMed, Web of Science, and reference lists of articles focusing on the outcomes that were most studied like preterm birth, low birth weight, stillbirth, and hypertensive disorders of pregnancy. A review of the literature reveals important gaps in knowledge and several methodological challenges. One important gap is the lack of knowledge of how core body temperature modulates under extreme ambient temperature exposure during pregnancy. We do not know the magnitude of non-modulation of body temperature during pregnancy that is clinically significant, i.e., when the body starts triggering physiologic counterbalances. Furthermore, few studies are conducted in places where extreme temperature conditions are more frequently encountered, such as in South Asia and sub-Saharan Africa. Little is also known about specific cost-effective interventions that can be implemented in vulnerable communities to reduce adverse outcomes. As the threat of global warming looms large, effective interventions are critically necessary to mitigate its effects.
1. Introduction
Global surface temperature has been on the rise since the Industrial Revolution due to the rapid increase in greenhouse gas emissions. The rising trend is likely to continue, increasingly exposing the world population to extreme climatic conditions, more frequently for longer duration and with greater intensity. The 2019 Global Burden of Disease study estimated the burden of extreme temperature, not including the effect of adverse pregnancy or perinatal outcomes because of the lack of causal evidence (1). This manuscript begins with a narrative presentation of the association between temperature and specific pregnancy or perinatal outcomes. In the following section, we discuss the methodological challenges in these types of studies. The final section highlights the gaps in the existing literature. Addressing these gaps should shed light on the nature of the relationship between temperature and adverse outcomes. This manuscript focused on highlighting the gaps and methodology issues in the current literature, rather than conducting a systematic or scoping review, because several of those already exist (2–4).
We focused on the outcomes that were most studied and searched for relevant literature using PubMed, Web of Science, published reviews, and reference lists. We used the following Medical Subject Headings (MeSH): “Temperature,” “Heat,” “Perinatal Outcomes,” “Pregnancy Outcomes,” “Preterm Birth,” “Low Birth Weight,” “Stillbirth,” “Hypertensive Disorders of Pregnancy.” The MeSH terms were combined with “and,” “or,” and “not” operators, and the search was restricted to articles on humans and published before 30 January 2023.
2. Preterm birth
We found 24 studies that examined short-term exposure to temperature and preterm birth (PTB) (5–28). The associations reported in these studies are quantitatively different [i.e., hazard ratio (HR), relative risk (RR), or odds ratio (OR)], vary in magnitude and precision, comparison groups are heterogeneous, and exposure ranges from a few individual days before delivery to cumulative average over a period. Eight studies were from the US, seven were conducted on different European populations, three each were from China and Australia, two were from Iran, and one was from Canada (Table 1). The studies vary in size ranging from a few thousand to over a million births, with percentages of PTB between 3% and 17% (Table 1). While many studies included births throughout the year, some included from one season. Four studies from California (9, 16–18) one from Minnesota (14), North Carolina (15), Italy (13), and Spain (6) examined the association separately by season. Most of the studies used birth record data employing retrospective cohort, case-crossover, or time series designs. Individual lag days (e.g., lag 1 representing the day before birth) or cumulative lags (e.g., lag 0–6 representing an average of 7 days, starting from the day of birth and including 6 preceding days), in the weeks and months prior to the day of birth, were used as exposure windows at risk. Additionally, various exposure contrasts were examined, e.g., for heat, 75th percentile or higher, and for cold, 10th percentile or lower temperatures were compared with the percentiles in between (e.g., median). Studies that examined heat waves defined in various ways, e.g., number of days above a threshold, and consecutive number of days above a threshold. The reported associations across studies broadly ranged from 1.01 (1.01, 1.02) to 2.5 (1.02, 6.15) (15, 24); the smallest association was reported by a US study for a 1-day lag temperature of 73–74°C compared with 71–72°C, and the largest was reported by a Swedish study for a 30-day cumulative lag at the 75th percentile (17.5°C) compared with the median (7.7°C). The study-specific associations are presented in Table 1. Notably, most of the studies reported that exposure–outcome associations were below 20%, i.e., the increased risk of PTB was below 20%. While heterogeneity between studies contributed to the diversity of evidence, summarizing results across studies becomes challenging without making additional assumptions. Many exposure windows were often combined with several exposure contrasts, and numerous tests of significance were conducted.
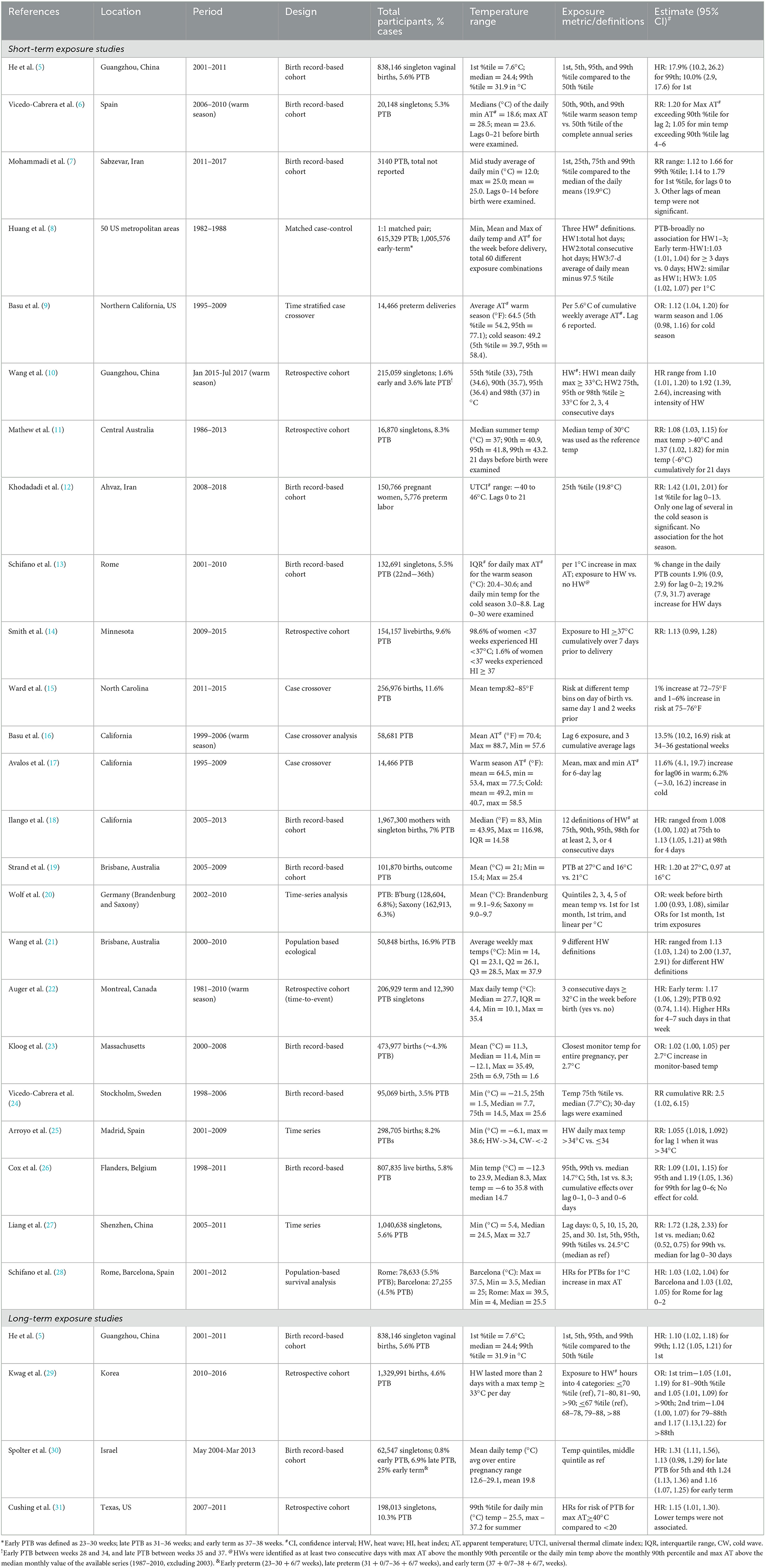
Table 1. Summary of studies conducted between 1982 and 2017 on short- and long-term exposure to temperature and preterm or early term births.
Four studies examined the association between long-term exposure to temperature and PTB (Table 1) (5, 29–31). Associations ranging from 1.05 (1.01, 1.19) to 1.31 (1.11, 1.56) were reported in studies conducted among Chinese, Korean, Israeli, and US populations (5, 29–31).
Effect modification by infant sex, race, ethnicity, maternal characteristics, and air pollution exposure has also been reported (8, 9, 26, 31). Three studies reported the associations with temperature were higher for non-Hispanic African Americans and Hispanics compared with non-Hispanic white people (8, 9, 31). Maternal smoking, alcohol consumption, hypertension, and diabetes have also been suggested to increase susceptibility to temperature (9). Preliminary evidence of synergism between heat waves and PM2.5 has been reported (10, 29). However, in another study, neither PM10 nor ozone modified the association between apparent temperature (AT) and PTB, although maternal age, education, and pre-existing chronic diseases modified the association (13).
3. Birth weight, low birth weight, and small for gestational age
We found 16 studies that examined the association of temperature with birth weight or low birth weight (LBW) (Table 2). Four of these studies were from the US, four from Europe, two from Israel, two from South America, two included global populations, and one each from Australia and Bangladesh (20, 23, 32–45). The majority of these studies included births occurring within the last two decades. However, the two global studies used World Health Organization (WHO) datasets and included births between 1980 and 1992 (41, 42). Most of the studies used retrospective cohort designs, except two, which were time-series (20) and prospective (37). Some studies reported associations of entire pregnancy exposures with LBW or birth weight (23, 35, 36, 38), while others reported associations with trimester-specific exposures (20, 23, 32–44). The associations for LBW broadly ranged between 18% (−5, 45%), per 2.4°C increase, and 148% (119, 181%) for temperature below the 5th percentile compared with 5th and 95th percentiles (Table 2) (35, 43). The study-specific associations along with the exposure contrasts are presented in Table 1. Notably, some studies included all LBW infants, regardless of gestational age, while others used term LBW (born at or after 37 completed weeks).
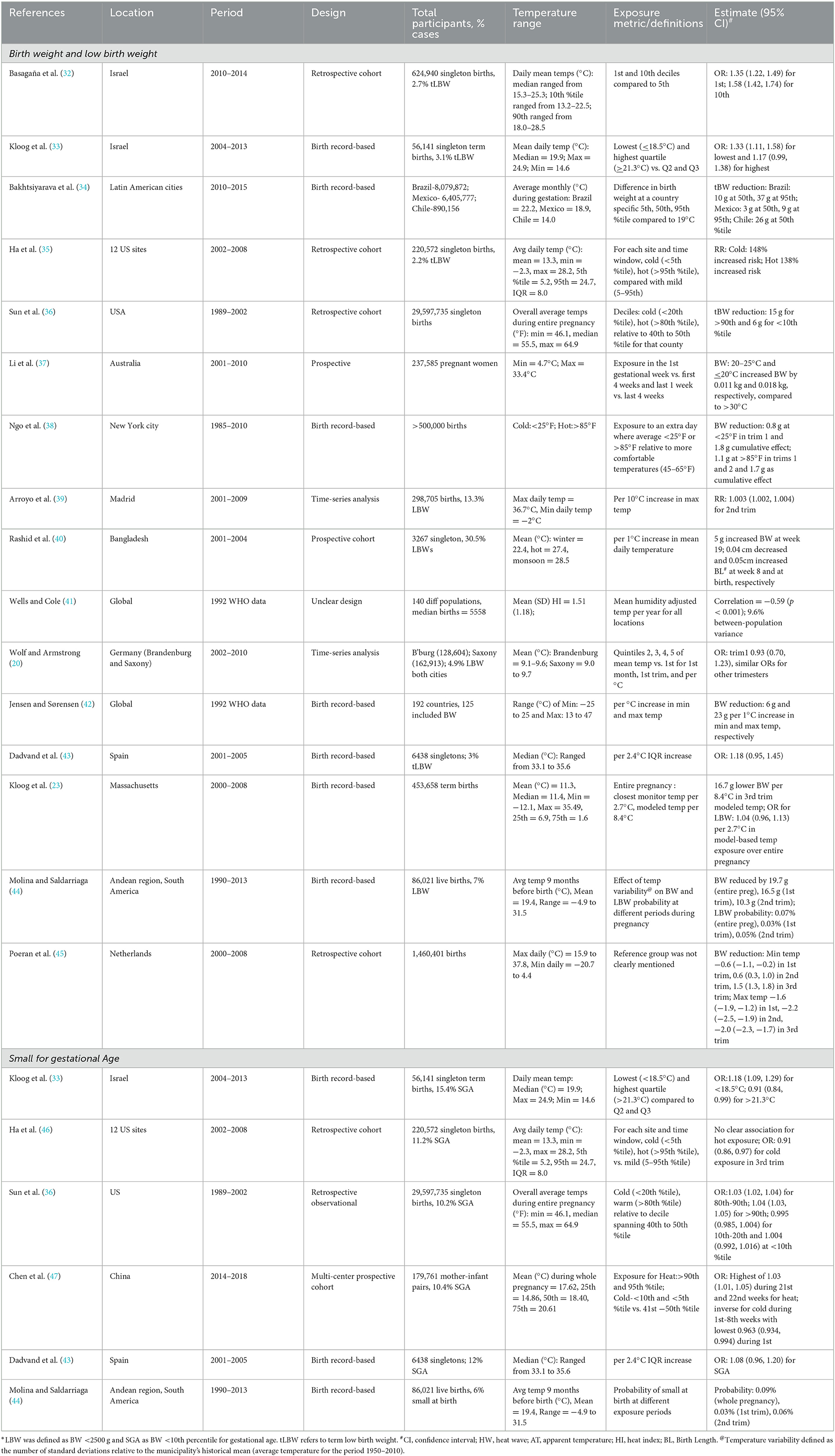
Table 2. Summary of studies conducted between 1985 and 2018 examining the association between temperature and birth weight, low birth weight or small-for-gestational age.
Two studies examined short-term exposure (Table 2). An Australian study reported the association between a daily maximum temperature of 20–25°C in the last week of pregnancy and 11 g (8, 18) higher estimated mean birth weight compared with > 30°C (37); another study from Spain reported an RR of 1.003 (1.002, 1.004) for LBW per 10°C increase in maximum temperature on the 14th week (39).
We found six studies from the US, Europe, Israel, China, and South America that investigated small for gestational age (SGA) (Table 2) (33, 35, 36, 43, 44, 47) with ORs ranging from 1.03 (1.01, 1.05) to 1.18 (1.09, 1.29).
4. Spontaneous abortion and stillbirth
We found 13 studies that examined the association of temperature with spontaneous abortions and stillbirths (12, 39, 46, 48–57) (Table 3). A unique study from Hungary reported that the likelihood of unobserved pregnancy loss is 0.22 (0.12, 0.33) per 100, 000 women aged 16–44 years if there is 1 day in the first 2 weeks of pregnancy with a mean temperature of >25°C compared with 15–20°C (48). Compared with the other outcomes, relatively more studies on stillbirth are from low- and middle-income countries, likely because of the higher incidence of stillbirth in these regions. These studies employed cross-sectional, case-crossover, retrospective, or time-series designs (Table 3). The associations for stillbirth ranged from 1.03 (1.00, 1.06), when the temperature was above 97.5th percentile for 4 consecutive days in the week prior to birth compared to 0 days above that level, to 3.7 (3.1, 4.5), per 1°C increase in the mean temperature in the week prior to birth in the warm season (46, 55).
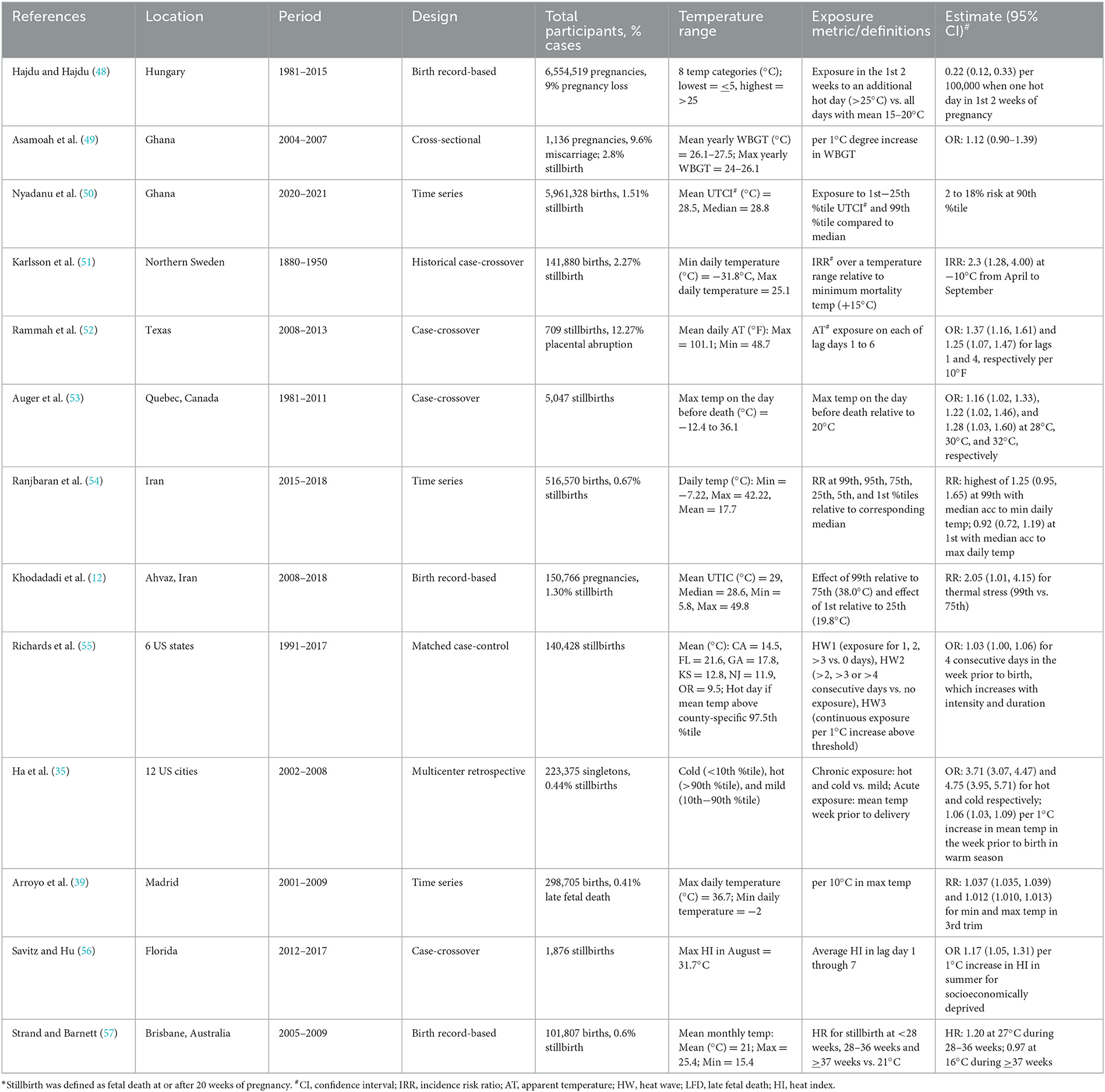
Table 3. Summary of studies conducted between 1981 and 2021 examining the association between temperature and stillbirth.
The association between temperature and stillbirth has been reported to differ by race, ethnicity, or socioeconomic status. Higher associations were reported for non-Hispanic African Americans and Hispanics compared with no association for non-Hispanic white (52). Higher association was also reported only in most socioeconomically deprived population but not in the overall study population (56).
5. Hypertensive disorders of pregnancy
Temperature has been associated with hypertensive disorders of pregnancy. Two- to three-fold increase in the risk of preeclampsia per 9°C has been found for 1st, 2nd, and 3rd trimester averages of daily mean temperatures (58). Before 12 weeks of conception, colder temperature has been reported to increase the odds of preeclampsia or eclampsia (59). However, in the first 20 weeks of pregnancy, colder temperature reduced the odds, while hotter temperature increased the odds of preeclampsia or eclampsia (59). No association with preeclampsia was found for heat stress in the 3 weeks prior to delivery (12).
6. Methodological considerations
Choice of metric is an important consideration because each represents qualitatively different exposure. For example, AT incorporates humidity and reflects how hot it feels outdoors. High humidity in moderately warm conditions can cause discomfort leading to physiological stress (60). High minimum temperature over consecutive days reflects the lack of overnight cooling, which could cause sleep perturbations (61). High maximum outdoor temperature increases physical discomfort, but wind velocity may increase or decrease physiological stress. When the body is sweating, wind can considerably influence regulatory physiological mechanisms. The other less commonly used metrics to measure heat stress are excessive heat factor, physiological equivalent temperature (PET), and Universal Thermal Climate Index (UTCI) (12, 62, 63). Regardless of the primary choice of metric, at least a few (e.g., maximum and minimum AT) should be uniformly reported to allow comparisons of association across studies. Furthermore, if temperature thresholds or non-linear relationships are observed, additional results should be reported in a manner that will allow between study comparisons and pooling of estimates across studies.
The importance of the comparison group can be highlighted using the example of the tri-country Latin American study (34). In the study, the means of the monthly averages for Brazil, Mexico, and Chile were 22, 19, and 14°C, respectively. Because of the annual weather patterns in each country, for Brazil, the comparison between the 50th percentile (22) and the reference group (19°C, average across all study sites) reflects the effect of heat, while for Chile, the same comparison (14) reflects the effect of cold. Thus, based on the same exposure contrasts, the results are essentially different effects of temperature.
Measuring exposure accurately is an enduring challenge in environmental epidemiology. Most studies use fixed site or satellite measurements as proxies for true personal exposure (30, 33, 58). Small deviations (~0.5°C) in true exposure may have significant repercussions on physiological processes during critical windows of pregnancy, which, if missed, can produce misleading results. Residential mobility during pregnancy, which has been studied more in the context of air pollution, may also affect exposure to extreme temperature (64–66). The presence and extent of exposure misclassification depend on the proportion-changing residences during pregnancy, which vary by region (67, 68). The amount of time spent in temperature-controlled environments could also misclassify exposure, which requires prospective studies designed to measure the time activity patterns of the participants. This information is seldom available from routine sources such as birth registries or hospital records.
Studies should use standardized outcome definitions, especially when countries use different definitions, e.g., stillbirths are recorded at 20 (US), 24 (UK), or 28 (WHO) weeks of gestation (46, 49, 51–56). Another example is late fetal death defined in a study as “death within the first 24 h of life” (39), which is inconsistent with the definition by WHO that states the first 24 h after birth as the early neonatal period. The availability of reliable data on spontaneous abortion and stillbirth is a challenge across regions. Accuracy of gestational age determines the classification of PTB, especially when the last menstrual period-based assessment is not verified by first trimester ultrasound. Studies examining temperature and birth weight should conceptually consider gestational age because it has been suggested to be in the causal pathway and adjustment could produce biased estimates (69).
As temperature, air pollution, and season are inter-related, to understand the independent associations, studies should develop frameworks including all known and potential risk factors in the context of the temperature–outcome relationship (e.g., Directed Acyclic Graphs). Temperature and air pollution vary by season. Perinatal outcomes have also been observed to vary by season, as do births (70). Several studies adjusted for air pollution, while others examined effect modification (10, 13, 28, 35, 39, 54). Buckley et al. argued that temperature and some pollutants (e.g., ozone) likely share predecessors (71). The nature of the pollutant warrants careful consideration before inclusion with temperature in the same model. The same argument also applies to seasonality and temperature. Restricting births to a season is an option, though it limits generalizability and sample size.
Fixed cohort bias is a threat to validity, but it has generally been considered (19, 72). It occurs when births, only within a fixed period, is included. This results in shorter pregnancies being missed at study initiation, while longer pregnancies are missed at the end of the study (19). To avoid fixed cohort bias, studies have used conception dates within a fixed period or excluded pregnancies conceived before a fixed time prior to the starting date of the study (5, 8, 55).
7. Gaps in current knowledge
From the perspective of exposure to extreme temperatures, several questions remain unanswered. Several studies have reported associations with multiple exposures windows, separately. It remains unclear, which exposure(s) is(are) relatively more harmful - a single extreme, several consecutive extremes, several interspersed extremes or moderately high prolonged exposures? Examining the overlap of these extremes with stages of fetal development will advance the understanding of the pathophysiological pathways. Fetal growth is programmed around the first month, when the placental bed is formed, which could be sensitive to exogenous exposures and merits investigation. Conversely, lean body mass growth occurs in the third trimester, a period that is also vulnerable to environmental exposures. It will be analytically complex to examine more than one exposure simultaneously (e.g., short- and long-term exposures), especially when they are biologically relevant and statistically correlated. Models have been developed that can examine multiple correlated exposures (e.g., Bayesian Kernel Machine Regression and distributed linear and non-linear lag models) (73–75).
Studies have provided some insights into potential biological mechanisms. For example, heat has been shown to affect thermoregulation and blood pressure during pregnancy (46, 76–78). Around 37 weeks when thermoregulation becomes less efficient because of increased body mass and decreased ability to dissipate heat by sweating, heat-related uterine contraction has been observed (28, 79). Extreme temperature exposure during pregnancy has been linked with systemic inflammation, cell impermeability, upregulation of heat shock proteins, release of endotoxins (46, 80), and gene sequencing (81, 82). Nonetheless, we do not know how core body temperature modulates under extreme ambient temperature, which could be an intermediate link between exposure and biological mechanisms. The fetus is usually ~0.5°C hotter than the maternal core and can lose heat only through the umbilical artery and amniotic fluid (83). In the event of maternal core temperature increase, heat likely gets transferred to the fetus (84, 85). Any physiologic or systemic effects interfering with maternal—fetal exchange will likely affect fetal development, growth, and potential survival. We do not know the magnitude of non-modulation of body temperature that is clinically important, i.e., when the body starts triggering physiological counterbalances. Does non-modulation differ in different populations and what factors (e.g., lifestyle characteristics) influence it? None of these questions have been addressed to date. Bonell et al. (86) are taking an important stride in that direction. More studies are needed in different populations to understand the modulation of core body temperature during pregnancy, transfer of excess heat from the mother to the fetus, and its consequences. Heat sensing and pregnancy monitoring wearables have been feasibly implemented in various settings including in low- and middle-income countries (87–92). These wearable devices are small. Prices range from fifty to several hundred US Dollars and come in various forms such as watches, rings, bracelets, and small buttons. The devices can store a reasonable amount of data, and the frequency of measurement determines the duration of a cycle. Technology involved in transferring data from these devices for further use in research varies in complexity. In addition to body temperature, some of these devices can measure ambient temperature, sleep, physical activity, etc. Technology has opened up avenues for accurate measurement of environmental exposures and helped advance research, though it is necessary to test these devices for accuracy before extensive use.
Populations acclimatize to their natural surroundings. A maximum temperature that is heat wave in temperate zones will be perceived differently in tropical countries. Localized behavioral adaptations during extreme heat or cold, such as modifying outdoor activities and consumption of specific food types, help mitigate adverse effects, predisposing populations to varying levels of risks. Most of the existing studies were conducted in the Western Hemisphere. New studies should be conducted on populations exposed to extreme temperatures and in low- and middle-income countries to understand the global risk pattern.
Little is known about the cost-effective interventions that can be implemented in vulnerable communities to reduce adverse outcomes. Vulnerable communities are likely to have fewer mitigation measures (e.g., air conditioning and lack of access to health care), particularly in rural and socioeconomically deprived areas. These communities are also likely to have a higher prevalence of pre-existing conditions and high-risk lifestyle (e.g., substance use and drinking), disposing pregnant women to higher risk, regardless of heat exposure.
For a comprehensive understanding of the nature of the effect, studies should be conducted in places where populations are frequently exposed to extreme temperature. Several other gaps in the available literature exist, including no knowledge about modulation of core body temperature during pregnancy, when exposed to extreme ambient temperature, which can help understand the biological mechanisms. Even if the epidemiologic associations of temperature with PTB, LBW, SGA, and stillbirths are small, these are clinically relevant indicators of newborn health and are observed across populations in varying magnitude. Despite a small association, continuing global warming will potentially increase exposure prevalence, thereby increasing the death and disability burden attributable to temperature, as well as the associated healthcare cost of the affected infants.
Data availability statement
The original contributions presented in the study are included in the article/supplementary material, further inquiries can be directed to the corresponding author.
Ethics statement
Ethical approval was not required for the study involving humans in accordance with the local legislation and institutional requirements. Written informed consent to participate in this study was not required from the participants or the participants' legal guardians/next of kin in accordance with the national legislation and the institutional requirements.
Author contributions
MM involved in literature search, drafted, and revised the manuscript. RB provided expert critique and suggested revision of the manuscript. RG conceptualized the research ideas and revised the manuscript. All authors contributed to the article and approved the submitted version.
Conflict of interest
The authors declare that the research was conducted in the absence of any commercial or financial relationships that could be construed as a potential conflict of interest.
Publisher's note
All claims expressed in this article are solely those of the authors and do not necessarily represent those of their affiliated organizations, or those of the publisher, the editors and the reviewers. Any product that may be evaluated in this article, or claim that may be made by its manufacturer, is not guaranteed or endorsed by the publisher.
References
1. Murray CJL, Aravkin AY, Zheng P, Abbafati C, Abbas KM, Abbasi-Kangevari M, et al. Global burden of 87 risk factors in 204 countries and territories, 1990–2019: a systematic analysis for the global burden of disease study 2019. Lancet. (2020) 396:1223–49. doi: 10.1016/S0140-6736(20)30752-2
2. Dalugoda Y, Kuppa J, Phung H, Rutherford S, Phung D. Effect of elevated ambient temperature on maternal, foetal, and neonatal outcomes: a scoping review. Int J Environ Res Pub Health. (2022) 19:1771. doi: 10.3390/ijerph19031771
3. Kuehn L, McCormick S. Heat exposure and maternal health in the face of climate change. Int J Environ Res Pub Health. (2017) 14:853. doi: 10.3390/ijerph14080853
4. Bekkar B, Pacheco S, Basu R, DeNicola N. Association of air pollution and heat exposure with preterm birth, low birth weight, and stillbirth in the US: a systematic review. JAMA Netw Open. (2020) 3:e208243. doi: 10.1001/jamanetworkopen.2020.8243
5. He JR, Liu Y, Xia XY, Ma WJ, Lin HL, Kan HD, et al. Ambient temperature and the risk of preterm birth in Guangzhou, China (2001–2011). Environ Health Perspect. (2016) 124:1100–6. doi: 10.1289/ehp.1509778
6. Vicedo-Cabrera AM, Iñíguez C, Barona C, Ballester F. Exposure to elevated temperatures and risk of preterm birth in Valencia, Spain. Environ Res. (2014) 134:210–7. doi: 10.1016/j.envres.2014.07.021
7. Mohammadi D, Naghshineh E, Sarsangi A, Zare Sakhvidi MJ. Environmental extreme temperature and daily preterm birth in Sabzevar, Iran: a time-series analysis. Environ Health Prev Med. (2019) 24:5. doi: 10.1186/s12199-018-0760-x
8. Huang M, Strickland MJ, Richards M, Holmes HA, Newman AJ, Garn JV, et al. Acute associations between heatwaves and preterm and early-term birth in 50 US metropolitan areas: a matched case-control study. Environ Health. (2021) 20:47. doi: 10.1186/s12940-021-00733-y
9. Basu R. Chen Hong, Li DK, Avalos LA. The impact of maternal factors on the association between temperature and preterm delivery. Environ Res. (2017) 154:109–14. doi: 10.1016/j.envres.2016.12.017
10. Wang Q, Li B, Benmarhnia T, Hajat S, Ren M, Liu T, et al. Independent and Combined Effects of Heatwaves and PM2.5 on preterm birth in Guangzhou, China: a survival analysis. Environ Health Perspect. (2020) 128:017006. doi: 10.1289/EHP5117
11. Mathew S, Mathur D, Chang A, McDonald E, Singh G, Nur D, et al. Examining the effects of ambient temperature on pre-term birth in central Australia. Int J Environ Res Pub Health. (2017) 14:147. doi: 10.3390/ijerph14020147
12. Khodadadi N, Dastoorpoor M, Khanjani N, Ghasemi A. Universal thermal climate index (UTCI) and adverse pregnancy outcomes in Ahvaz, Iran. Reprod Health. (2022) 19:33. doi: 10.1186/s12978-022-01344-7
13. Schifano P, Lallo A, Asta F, De Sario M, Davoli M, Michelozzi P. Effect of ambient temperature and air pollutants on the risk of preterm birth, Rome 2001–2010. Environ Int. (2013) 61:77–87. doi: 10.1016/j.envint.2013.09.005
14. Smith ML, Hardeman RR. Association of summer heat waves and the probability of preterm birth in minnesota: an exploration of the intersection of race and education. Int J Environ Res Public Health. (2020) 17:6391. doi: 10.3390/ijerph17176391
15. Ward A, Clark J, McLeod J, Woodul R, Moser H, Konrad C. The impact of heat exposure on reduced gestational age in pregnant women in North Carolina, 2011–2015. Int J Biometeorol. (2019) 63:1611–20. doi: 10.1007/s00484-019-01773-3
16. Basu R, Malig B, Ostro B. High ambient temperature and the risk of preterm delivery. Am J Epidemiol. (2010) 172:1108–17. doi: 10.1093/aje/kwq170
17. Avalos LA, Chen H, Li DK, Basu R. The impact of high apparent temperature on spontaneous preterm delivery: a case-crossover study. Environ Health. (2017) 16:5. doi: 10.1186/s12940-017-0209-5
18. Ilango SD, Weaver M, Sheridan P, Schwarz L, Clemesha RES, Bruckner T, et al. Extreme heat episodes and risk of preterm birth in California, 2005–2013. Environ Int. (2020) 137:105541. doi: 10.1016/j.envint.2020.105541
19. Strand LB, Barnett AG, Tong S. Methodological challenges when estimating the effects of season and seasonal exposures on birth outcomes. BMC Med Res Methodol. (2011) 11:49. doi: 10.1186/1471-2288-11-49
20. Wolf J, Armstrong B. The association of season and temperature with adverse pregnancy outcome in two German states, a time-series analysis. PLoS ONE. (2012) 7:e40228. doi: 10.1371/journal.pone.0040228
21. Wang J, Williams G, Guo Y, Pan X, Tong S. Maternal exposure to heatwave and preterm birth in Brisbane, Australia. BJOG Int J Obstet Gynaecol. (2013) 120:1631–41. doi: 10.1111/1471-0528.12397
22. Auger N, Naimi AI, Smargiassi A, Lo E, Kosatsky T. Extreme heat and risk of early delivery among preterm and term pregnancies. Epidemiology. (2014) 25:344–50. doi: 10.1097/EDE.0000000000000074
23. Kloog I, Melly SJ, Coull BA, Nordio F, Schwartz JD. Using satellite-based spatiotemporal resolved air temperature exposure to study the association between ambient air temperature and birth outcomes in Massachusetts. Environ Health Perspect. (2015) 123:1053–8. doi: 10.1289/ehp.1308075
24. Vicedo-Cabrera A, Olsson D, Forsberg B. Exposure to seasonal temperatures during the last month of gestation and the risk of preterm birth in Stockholm. Int J Environ Res Public Health. (2015) 12:3962–78. doi: 10.3390/ijerph120403962
25. Arroyo V, Díaz J, Ortiz C, Carmona R, Sáez M, Linares C. Short term effect of air pollution, noise and heat waves on preterm births in Madrid (Spain). Environ Res. (2016) 145:162–8. doi: 10.1016/j.envres.2015.11.034
26. Cox B, Vicedo-Cabrera AM, Gasparrini A, Roels HA, Martens E, Vangronsveld J, et al. Ambient temperature as a trigger of preterm delivery in a temperate climate. J Epidemiol Commun Health. (2016) 70:1191–9. doi: 10.1136/jech-2015-206384
27. Liang Z, Lin Y, Ma Y, Zhang L, Zhang X, Li L, et al. The association between ambient temperature and preterm birth in Shenzhen, China: a distributed lag non-linear time series analysis. Environ Health. (2016) 15:84. doi: 10.1186/s12940-016-0166-4
28. Schifano P, Asta F, Dadvand P, Davoli M, Basagana X, Michelozzi P. Heat and air pollution exposure as triggers of delivery: a survival analysis of population-based pregnancy cohorts in Rome and Barcelona. Environ Int. (2016) 88:153–9. doi: 10.1016/j.envint.2015.12.013
29. Kwag Y, Kim M, Oh J, Shah S, Ye S, Ha EH. Effect of heat waves and fine particulate matter on preterm births in Korea from 2010 to 2016. Environ Int. (2021) 147:106239. doi: 10.1016/j.envint.2020.106239
30. Spolter F, Kloog I, Dorman M, Novack L, Erez O, Raz R. Prenatal exposure to ambient air temperature and risk of early delivery. Environ Int. (2020) 142:105824. doi: 10.1016/j.envint.2020.105824
31. Cushing L, Morello-Frosch R, Hubbard A. Extreme heat and its association with social disparities in the risk of spontaneous preterm birth. Paediatr Perinat Epidemiol. (2022) 36:13–22. doi: 10.1111/ppe.12834
32. Basagaña X, Michael Y, Lensky IM, Rubin L, Grotto I, Vadislavsky E, et al. Low and high ambient temperatures during pregnancy and birth weight among 624,940 singleton term births in Israel (2010–2014): an investigation of potential windows of susceptibility. Environ Health Perspect. (2021) 129:107001. doi: 10.1289/EHP8117
33. Kloog I, Novack L, Erez O, Just AC, Raz R. Associations between ambient air temperature, low birth weight and small for gestational age in term neonates in southern Israel. Environ Health. (2018) 17:76. doi: 10.1186/s12940-018-0420-z
34. Bakhtsiyarava M, Ortigoza A, Sánchez BN, Braverman-Bronstein A, Kephart JL, Rodríguez López S, et al. Ambient temperature and term birthweight in Latin American cities. Environ Int. (2022) 167:107412. doi: 10.1016/j.envint.2022.107412
35. Ha S, Zhu Y, Liu D, Sherman S, Mendola P. Ambient temperature and air quality in relation to small for gestational age and term low birthweight. Environ Res. (2017) 155:394–400. doi: 10.1016/j.envres.2017.02.021
36. Sun S, Spangler KR, Weinberger KR, Yanosky JD, Braun JM, Wellenius GA. Ambient temperature and markers of fetal growth: a retrospective observational study of 29 million U.S singleton births. Environ Health Perspect. (2019) 127:067005. doi: 10.1289/EHP4648
37. Li S, Wang J, Xu Z, Wang X, Xu G, Zhang J, et al. Exploring associations of maternal exposure to ambient temperature with duration of gestation and birth weight: a prospective study. BMC Preg Childb. (2018) 18:513. doi: 10.1186/s12884-018-2100-y
38. Ngo NS, Horton RM. Climate change and fetal health: the impacts of exposure to extreme temperatures in New York City. Environ Res. (2016) 144:158–64. doi: 10.1016/j.envres.2015.11.016
39. Arroyo V, Díaz J, Carmona R, Ortiz C, Linares C. Impact of air pollution and temperature on adverse birth outcomes: Madrid, 2001–2009. Environ Pollut. (2016) 218:1154–61. doi: 10.1016/j.envpol.2016.08.069
40. Rashid H, Kagami M, Ferdous F, Ma E, Terao T, Hayashi T, et al. Temperature during pregnancy influences the fetal growth and birth size. Trop Med Health. (2017) 45:1. doi: 10.1186/s41182-016-0041-6
41. Wells JCK, Cole TJ. Birth weight and environmental heat load: a between-population analysis. Am J Phys Anthropol. (2002) 119:276–82. doi: 10.1002/ajpa.10137
42. Jensen PM, Sørensen M. Differences in human birth weight and corollary attributes as a result of temperature regime. Ann Hum Biol. (2013) 40:385–95. doi: 10.3109/03014460.2013.786757
43. Dadvand P, Ostro B, Figueras F, Foraster M, Basagaña X, Valentín A, et al. Residential proximity to major roads and term low birth weight: the roles of air pollution, heat, noise, and road-adjacent trees. Epidemiology. (2014) 25:518–25. doi: 10.1097/EDE.0000000000000107
44. Molina O, Saldarriaga V. The perils of climate change: In utero exposure to temperature variability and birth outcomes in the Andean region. Econ Hum Biol. (2017) 24:111–24. doi: 10.1016/j.ehb.2016.11.009
45. Poeran J, Birnie E, Steegers EAP. The impact of extremes in outdoor temperature and sunshine exposure on birth weight. J Environ Health. (2016) 78:92–101. Available online at: https://www.jstor.org/stable/26330398
46. Ha S, Liu D, Zhu Y, Soo Kim S, Sherman S, Grantz KL, et al. Ambient temperature and stillbirth: a multi-center retrospective cohort study. Environ Health Perspect. (2017) 125:067011. doi: 10.1289/EHP945
47. Chen X, Chen S, Zhu Z, Luo J, Wang H, Wulayin M, et al. Identifying the critical windows and joint effects of temperature and PM2.5 exposure on small for gestational age. Environ Int. (2023) 173:107832. doi: 10.1016/j.envint.2023.107832
48. Hajdu T, Hajdu G. Post-conception heat exposure increases clinically unobserved pregnancy losses. Sci Rep. (2021) 11:1987. doi: 10.1038/s41598-021-81496-x
49. Asamoah B, Kjellstrom T, Östergren PO. Is ambient heat exposure levels associated with miscarriage or stillbirths in hot regions? A cross-sectional study using survey data from the Ghana maternal health survey 2007. Int J Biometeorol. (2018) 62:319–30. doi: 10.1007/s00484-017-1402-5
50. Nyadanu SD, Tessema GA, Mullins B, Kumi-Boateng B, Ofosu AA, Pereira G. Prenatal exposure to long-term heat stress and stillbirth in Ghana: a within-space time-series analysis. Environ Res. (2023) 222:115385. doi: 10.1016/j.envres.2023.115385
51. Karlsson L, Junkka J, Lundevaller EH, Schumann B. Ambient temperature and stillbirth risks in northern Sweden, 1880–1950. Environ Epidemiol. (2021) 5:e176. doi: 10.1097/EE9.0000000000000176
52. Rammah A, Whitworth KW, Han I, Chan W, Hess JW, Symanski E. Temperature, placental abruption and stillbirth. Environ Int. (2019) 131:105067. doi: 10.1016/j.envint.2019.105067
53. Auger N, Fraser WD, Smargiassi A, Bilodeau-Bertrand M, Kosatsky T. Elevated outdoor temperatures and risk of stillbirth. Int J Epidemiol. (2016) 9:200. doi: 10.1093/ije/dyw077
54. Ranjbaran M, Mohammadi R, Yaseri M, Kamari M, Habibelahi A, Yazdani K. Effect of ambient air pollution and temperature on the risk of stillbirth: a distributed lag nonlinear time series analysis. J Environ Health Sci Eng. (2020) 18:1289–99. doi: 10.1007/s40201-020-00547-z
55. Richards M, Huang M, Strickland MJ, Newman AJ, Warren JL, D'Souza R, et al. Acute association between heatwaves and stillbirth in six US states. Environ Health. (2022) 21:59. doi: 10.1186/s12940-022-00870-y
56. Savitz DA, Hu H. Ambient heat and stillbirth in Northern and Central Florida. Environ Res. (2021) 199:111262. doi: 10.1016/j.envres.2021.111262
57. Strand LB, Barnett AG, Tong S. Maternal exposure to ambient temperature and the risks of preterm birth and stillbirth in Brisbane, Australia. Am J Epidemiol. (2012) 175:99–107. doi: 10.1093/aje/kwr404
58. Shashar S, Kloog I, Erez O, Shtein A, Yitshak-Sade M, Sarov B, et al. Temperature and preeclampsia: epidemiological evidence that perturbation in maternal heat homeostasis affects pregnancy outcome. PLoS ONE. (2020) 15:e0232877. doi: 10.1371/journal.pone.0232877
59. Xiong T, Chen P, Mu Y, Li X, Di B, Li J, et al. Association between ambient temperature and hypertensive disorders in pregnancy in China. Nat Commun. (2020) 11:2925. doi: 10.1038/s41467-020-16775-8
60. Heal G, Park J. Reflections—temperature stress and the direct impact of climate change: a review of an emerging literature. Rev Environ Econ Policy. (2016) 10:347–62. doi: 10.1093/reep/rew007
61. Lu Q, Zhang X, Wang Y, Li J, Xu Y, Song X, et al. Sleep disturbances during pregnancy and adverse maternal and fetal outcomes: a systematic review and meta-analysis. Sleep Med Rev. (2021) 58:101436. doi: 10.1016/j.smrv.2021.101436
62. Dastoorpoor M, Khanjani N, Khodadadi N. Association between physiological equivalent temperature (PET) with adverse pregnancy outcomes in Ahvaz, southwest of Iran. BMC Preg Childb. (2021) 21:415. doi: 10.1186/s12884-021-03876-5
63. Nairn JR, Fawcett RJB. The excess heat factor: a metric for heatwave intensity and its use in classifying heatwave severity. Int J Environ Res Pub Health. (2015) 12:227–53. doi: 10.3390/ijerph120100227
64. Fell DB, Dodds L, King WD. Residential mobility during pregnancy. Paediatr Perinat Epidemiol. (2004) 18:408–14. doi: 10.1111/j.1365-3016.2004.00580.x
65. Pennington AF, Strickland MJ, Klein M, Zhai X, Russell AG, Hansen C, et al. Measurement error in mobile source air pollution exposure estimates due to residential mobility during pregnancy. J Expo Sci Environ Epidemiol. (2017) 27:513–20. doi: 10.1038/jes.2016.66
66. Blanchard O, Deguen S, Kihal-Talantikite W, François R, Zmirou-Navier D. Does residential mobility during pregnancy induce exposure misclassification for air pollution? Environ Health. (2018) 17:72. doi: 10.1186/s12940-018-0416-8
67. Warren JL, Son JY, Pereira G, Leaderer BP, Bell ML. Investigating the impact of maternal residential mobility on identifying critical windows of susceptibility to ambient air pollution during pregnancy. Am J Epidemiol. (2018) 187:992–1000. doi: 10.1093/aje/kwx335
68. Madsen C, Gehring U, Erik Walker S, Brunekreef B, Stigum H, Næss Ø, et al. Ambient air pollution exposure, residential mobility and term birth weight in Oslo, Norway. Environ Res. (2010) 110:363–71. doi: 10.1016/j.envres.2010.02.005
69. Wilcox AJ, Weinberg CR, Basso O. On the pitfalls of adjusting for gestational age at birth. Am J Epidemiol. (2011) 174:1062–8. doi: 10.1093/aje/kwr230
70. Strand LB, Barnett AG, Tong S. The influence of season and ambient temperature on birth outcomes: a review of the epidemiological literature. Environ Res. (2011) 111:451–62. doi: 10.1016/j.envres.2011.01.023
71. Buckley JP, Samet JM, Richardson DB. Commentary: does air pollution confound studies of temperature? Epidemiology. (2014) 25:242. doi: 10.1097/EDE.0000000000000051
72. Auger N, Siemiatycki J, Bilodeau-Bertrand M, Healy-Profitós J, Kosatsky T. Ambient temperature and risk of preeclampsia: biased association? Paediatr Perinat Epidemiol. (2017) 31:267–71. doi: 10.1111/ppe.12362
73. Bobb JF, Valeri L, Claus Henn B, Christiani DC, Wright RO, Mazumdar M, et al. Bayesian kernel machine regression for estimating the health effects of multi-pollutant mixtures. Biostat Oxf Engl. (2015) 16:493–508. doi: 10.1093/biostatistics/kxu058
74. Chen YH, Mukherjee B, Berrocal VJ. Distributed lag interaction models with two pollutants. J R Stat Soc Ser C Appl Stat. (2019) 68:79–97. doi: 10.1111/rssc.12297
75. Gasparrini A. Distributed lag linear and non-linear models in R: the package DLNM. J Stat Softw. (2011) 43:1–20. doi: 10.18637/jss.v043.i08
76. Bruckner TA, Modin B, Vågerö D. Cold ambient temperature in utero and birth outcomes in Uppsala, Sweden, 1915–1929. Ann Epidemiol. (2014) 24:116–21. doi: 10.1016/j.annepidem.2013.11.005
77. Khamis Y, Shaala S, Damarawy H, Romia A, Toppozada M. Effect of heat on uterine contractions during normal labor. Int J Gynecol Obstet. (1983) 21:491–3. doi: 10.1016/0020-7292(83)90041-3
78. Vähä-Eskeli K, Erkkola R. The effect of short-term heat stress on uterine contractility, fetal heart rate and fetal movements at late pregnancy. Eur J Obstet Gynecol Reprod Biol. (1991) 38:9–14. doi: 10.1016/0028-2243(91)90200-5
79. He S, Kosatsky T, Smargiassi A, Bilodeau-Bertrand M, Auger N. Heat and pregnancy-related emergencies: risk of placental abruption during hot weather. Environ Int. (2018) 111:295–300. doi: 10.1016/j.envint.2017.11.004
80. Samuels L, Nakstad B, Roos N, Bonell A, Chersich M, Havenith G, et al. Physiological mechanisms of the impact of heat during pregnancy and the clinical implications: review of the evidence from an expert group meeting. Int J Biometeorol. (2022) 66:1505–13. doi: 10.1007/s00484-022-02301-6
81. Edwards M. Hyperthermia and birth defects. Reprod Toxicol. (1995) 9:411–25. doi: 10.1016/0890-6238(95)00043-A
82. Edwards MJ. Review: Hyperthermia and fever during pregnancy. Birt Defects Res A Clin Mol Teratol. (2006) 76:507–16. doi: 10.1002/bdra.20277
83. McMurray RG, Katz VL. Thermoregulation in Pregnancy. Sports Med. (1990) 10:146–58. doi: 10.2165/00007256-199010030-00002
84. Mann TP. Observations on temperatures of mothers and babies in the perinatal period*. BJOG Int J Obstet Gynaecol. (1968) 75:316–21. doi: 10.1111/j.1471-0528.1968.tb02085.x
85. Walker D, Walker A, Wood C. Temperature of the human fetus. BJOG Int J Obstet Gynaecol. (1969) 76:503–11. doi: 10.1111/j.1471-0528.1969.tb05870.x
86. Bonell A, Hirst J, Vicedo-Cabrera AM, Haines A, Prentice AM, Maxwell NS, et al. protocol for an observational cohort study of heat strain and its effect on fetal wellbeing in pregnant farmers in The Gambia. Wellcome Open Res. (2020) 5:32. doi: 10.12688/wellcomeopenres.15731.2
87. Garbern SC, Mbanjumucyo G, Umuhoza C, Sharma VK, Mackey J, Tang O, et al. Validation of a wearable biosensor device for vital sign monitoring in septic emergency department patients in Rwanda. Digit Health. (2019) 5:2055207619879349. doi: 10.1177/2055207619879349
88. Shahil Feroz A, Afzal N, Seto E. Exploring digital health interventions for pregnant women at high risk for pre-eclampsia and eclampsia in low-income and-middle-income countries: a scoping review. BMJ Open. (2022) 12:e056130. doi: 10.1136/bmjopen-2021-056130
89. Ryu D, Kim DH, Price JT, Lee JY, Chung HU, Allen E, et al. Comprehensive pregnancy monitoring with a network of wireless, soft, and flexible sensors in high- and low-resource health settings. Proc Natl Acad Sci U S A. (2021) 118:e2100466118. doi: 10.1073/pnas.2100466118
90. Dhivya MR, Ananthalakshmi A, Harini TR, Lavanya M. Monitoring and shaping the future of pregnant Women in rural areas using IoT. Int Res J Mod Eng Technol Sci. (2021) 3:2482–8. doi: 10.1109/ICACCS51430.2021.9441677
91. Baste VS, Gaikar S, Borkar M, Gatade H, Bhoyar R. Survey on development of convenient health monitoring system for pregnant women in rural area. Int J Comput Artif Intell. (2021) 2:53–6. doi: 10.33545/27076571.2021.v2.i1a.26
Keywords: temperature, birth outcomes, pregnancy outcomes, heat, climate change
Citation: Mehta M, Basu R and Ghosh R (2023) Adverse effects of temperature on perinatal and pregnancy outcomes: methodological challenges and knowledge gaps. Front. Public Health 11:1185836. doi: 10.3389/fpubh.2023.1185836
Received: 14 March 2023; Accepted: 19 September 2023;
Published: 01 November 2023.
Edited by:
Weiwei Wu, Shanxi Medical University, ChinaReviewed by:
Bruce Bekkar, Climate for Health, ecoAmerica, United StatesMarie O'Neill, University of Michigan, United States
Copyright © 2023 Mehta, Basu and Ghosh. This is an open-access article distributed under the terms of the Creative Commons Attribution License (CC BY). The use, distribution or reproduction in other forums is permitted, provided the original author(s) and the copyright owner(s) are credited and that the original publication in this journal is cited, in accordance with accepted academic practice. No use, distribution or reproduction is permitted which does not comply with these terms.
*Correspondence: Rakesh Ghosh, rakesh.ghosh@ucsf.edu