- 1School of Public Health and Health Management, Gannan Medical University, Ganzhou, China
- 2Key Laboratory of Prevention and Treatment of Cardiovascular and Cerebrovascular Diseases of Ministry of Education, Gannan Medical University, Ganzhou, China
- 3Gannan Medical University First Affiliated Hospital, Ganzhou, China
Background: With modernization development, multiple studies of atmospheric particulate matter exposure conducted in China have confirmed adverse cardiovascular health effects. However, there are few studies on the effect of particulate matter on blood lipid levels in patients with cardiovascular disease, especially in southern China. The purpose of this study was to investigate the association between short- and long-term exposure to ambient particulate matter and the levels of blood lipid markers in hypertension inpatients in Ganzhou, China.
Methods: Data on admission lipid index testing for hypertension inpatients which were divided into those with and without arteriosclerosis disease were extracted from the hospital's big data center from January 1, 2016 to December 31, 2020, and air pollution and meteorology data were acquired from the China urban air quality real time release platform from January 1, 2015 to December 31, 2020 and climatic data center from January 1, 2016 to December 31, 2020, with data integrated according to patient admission dates. A semi-parametric generalized additive model (GAM) was established to calculate the association between ambient particulate matter and blood lipid markers in hypertension inpatients with different exposure time in 1 year.
Results: Long-term exposure to particulate matter was associated with increased Lp(a) in three kinds of people, and with increased TC and decreased HDL-C in total hypertension and hypertension with arteriosclerosis. But particulate matter was associated with increased HDL-C for hypertension inpatients without arteriosclerosis, at the time of exposure in the present study. It is speculated that hypertension inpatients without arteriosclerosis has better statement than hypertension inpatients with arteriosclerosis on human lipid metabolism.
Conclusion: Long-term exposure to ambient particulate matter is associated with adverse lipid profile changes in hypertension inpatients, especially those with arteriosclerosis. Ambient particulate matter may increase the risk of arteriosclerotic events in hypertensive patients.
1. Introduction
Air pollution has become an environmental risk factor that seriously affects health, bringing a huge economic burden of disease and health loss to society (1, 2). According to a Comment by the World Health Organization (WHO), the number of premature deaths caused by air pollution has exceeded 7 million each year (3). Among air pollutants, ambient particulate matter (fine particulate matter with aerodynamic diameter <2.5 μm [PM2.5] and respirable particulate matter with aerodynamic diameter <10 μm [PM10]) is widely recognized as an important toxic component of air pollution mixtures (4). Most studies have found that ambient particulate matter exposure can increase the incidence of cardiovascular disease events (5, 6), among which hypertension as a risk factor for most cardiovascular diseases has been confirmed by most studies to be related to the health hazard effects of ambient particulate matter exposure (7, 8).
Blood lipids are the general term for neutral fats (triglycerides) and lipids (phospholipids, glycolipids, sterols, steroids) in plasma (9). Adverse alterations in lipid levels in humans can cause deposition of lipids in the vessel wall, which in turn triggers vascular stiffening they are also recognized as a risk factor for cardio - and cerebrovascular disease (10–12), and play an important role in chronic diseases such as hypertension, myocardial infarction, and ischemic stroke (13–16). It has been found that long-term exposure to ambient particulate matter can change the lipid level in the population (17, 18), but there is no consistency about the lipid effects of air pollution described by different studies (19, 20).
Ganzhou City is located in the southern of China. It has a typical humid subtropical monsoon climate. The precipitation is concentrated in spring and summer, the climate is mild, the heat and rainfall are abundant, the duration of cold and hot air flow is short, and the frost-free period is long. In recent years, Ganzhou's rare earth mining industry, tourism, furniture construction and other industries have developed rapidly, driving the steady growth of the city's economy. With the influx of population, the continuous increase in traffic flow, and the urbanization, the urban vitality of Ganzhou has been greatly improved, but it has also increased pollution emissions, posing challenges to air governance. As the economy develops, there are no studies on associations between air pollution exposure and blood lipids in Ganzhou. Therefore, this study collected ambient particulate matter exposure concentrations in Ganzhou City and blood lipid detection data of hypertensive inpatients in a tertiary hospital, and explored the impacts of ambient particulate matter pollution on blood lipid levels in hypertensive populations.
2. Methods
2.1. Blood lipid markers in hypertensive hospitalized patients
The hospitalization records of cardiovascular medicine from January 1, 2016 to December 31, 2020 were extracted from the big data center of a tertiary hospital (two campuses) in Ganzhou City, including the patient's admission date, main diagnosis, age, gender, and biochemical tests. According to the 10th revised International Classification of Diseases (ICD-10), whose code is Hypertension (ICD-10: I10-I13), we screened the information of patients with “hypertension” as their discharge diagnosis and matched them with data of blood lipid markers collected during hospitalization. Included lipid markers included triglycerides (TG), total cholesterol (TC), high-density lipoprotein cholesterol (HDL-C), low-density lipoprotein cholesterol (LDL-C), and lipoprotein [Lp(a)]. Since LP(a) is skewed distribution data, we logarithmically transform LP(a). The hypertensive inpatients were divided into “hypertension with arteriosclerosis population” and “hypertension without arteriosclerosis population” according to whether other discharge diagnoses included arteriosclerosis. The sum of the two populations was called “total hypertension population.”
2.2. Air pollution and meteorology data of Ganzhou City
The air pollutant concentration data of Ganzhou City from January 1, 2015 to December 31, 2020 were obtained on the China Urban Air Quality Real-time Release Platform. Calculate and sort out the 24-h average concentrations of five air pollutants such as PM2.5, PM10, carbon monoxide (CO), Nitrogen dioxide (NO2), and sulfur dioxide (SO2) and the daily maximum 8-h average ozone (O3) concentration. Air pollution exposure concentrations were matched according to the patients' hospitalization dates to generate a total of 15 air pollution concentration data series including L0, L0-6, L0-13, L0-29, L0-59, L0-89, L0-119, L0-149, L0-179, L0-209, L0-239, L0-269, L0-299, L0-329, and L0-359. Where L0 is equivalent to the same day exposure concentration of the respective air pollutant and L0-6 are equivalent to the moving average exposure concentrations at a lag of 6 days between hospital admissions for patients with hypertension. Meteorological data comes from the National Climate Data Center, which collects indicators such as the daily average temperature (°C) and relative humidity (%) in Ganzhou from January 1, 2016 to December 31, 2020. The locations of air pollutant data monitoring sites and hospital admissions (two campuses) are shown in Figure 1.
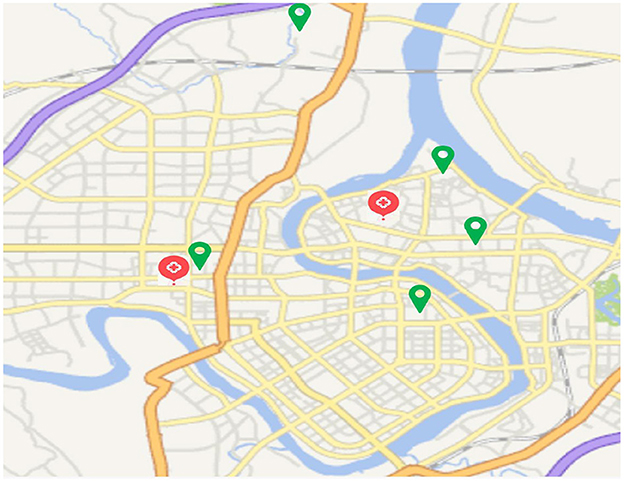
Figure 1. The locations of air pollutant data monitoring sites (green symbol) and hospital admissions (two campuses, red symbol).
2.3. Statistical analysis
SPSS was used to describe the mean ± standard deviation (Mean ± SD) of blood lipid indexes in three groups of “hypertension with arteriosclerosis,” “hypertension without arteriosclerosis” and “total hypertension.” Age, gender and hospitalization date were used as covariates for covariance analysis to compare the differences in the mean concentrations of blood lipid indexes among the three groups. If differences were statistically significant, Bonferroni's method was used for multiple comparisons (21). The central and discrete trends of air pollution and meteorological data were described, and the differences in air pollution concentrations between adjacent years were compared using the Kruskal-Wallis test (22). Spearman correlation analysis was used to evaluate the associations between air pollutants and meteorological data (23).
R4.0.4 was used to establish a semi-parametric generalized additive model (GAM) (24, 25) to adjust the temperature, atmospheric relative humidity, age and gender of patients and balance the time trend to calculate the effect of changes in the unit concentration of air pollution on blood lipids in the study population. On this basis, choose the lag time period in which the ambient particulate matter has the strongest correlation with the blood lipid concentration of the population in the lag of 1 year to establish a dual-pollutant model, and observe the impacts of ambient particulate matter exposure on the blood lipid concentration of the three groups of people after adjusting for the concentration of gaseous pollutants. The partial regression coefficient and 95% confidence interval of the effect of ambient particulate matter on the concentration of blood lipid markers were obtained, and converted into the change value of blood lipid concentration by exp[ (β × 10)-1] × 100. That is, the corresponding change in blood lipid concentration for every 10 μg/m3 increase in PM2.5 or PM10 concentration, and its 95% confidence interval.
3. Results
3.1. Characteristics of lipid markers in study subjects
Among the hypertensive hospitalized patients, 2,269 cases of TC and TG were matched respectively, among which 685 cases had arteriosclerosis, 2,268 cases of HDL-C had 685 cases of arteriosclerosis, 2,266 cases of LDL-C had 685 cases of arteriosclerosis, 2,241 cases of Lp(a) of which 674 had arteriosclerosis. As shown in Table 1, the mean values of the five lipid markers in the total hypertensive population were: TG 1.74 mmol/l, TC 4.45 mmol/l, HDL-C 1.1 mmol/l, LDL-C 2.69 mmol/l, and LP (a) 1.81 μmol/L. There were no significant differences in lipid concentrations among the three groups by covariance analysis.
3.2. Characteristics of air pollution and meteorological data
Table 2 shows that the average concentrations of CO, NO2, O3, PM10, PM2.5, and SO2 are 1.27 mg/m3, 23.171 μg/m3, 70.80 μg/m3, 59.89 μg/m3, 38.17 μg/m3 and 20.94 μg/m3, respectively. The daily average temperature and relative humidity were 19.72°C and 74.37%, respectively. Figure 2 shows the change in the annual average concentration of air pollutants from 2015 to 2020 compared with the previous year. From 2018 to 2020, the annual average concentrations of PM2.5, PM10, NO2 and CO showed a clear downward trend year by year. The annual mean concentration of SO2 decreased significantly in 2018 and 2019. The annual average concentration of O3 dropped significantly in 2016 and again in 2017 was significantly higher than the previous year.
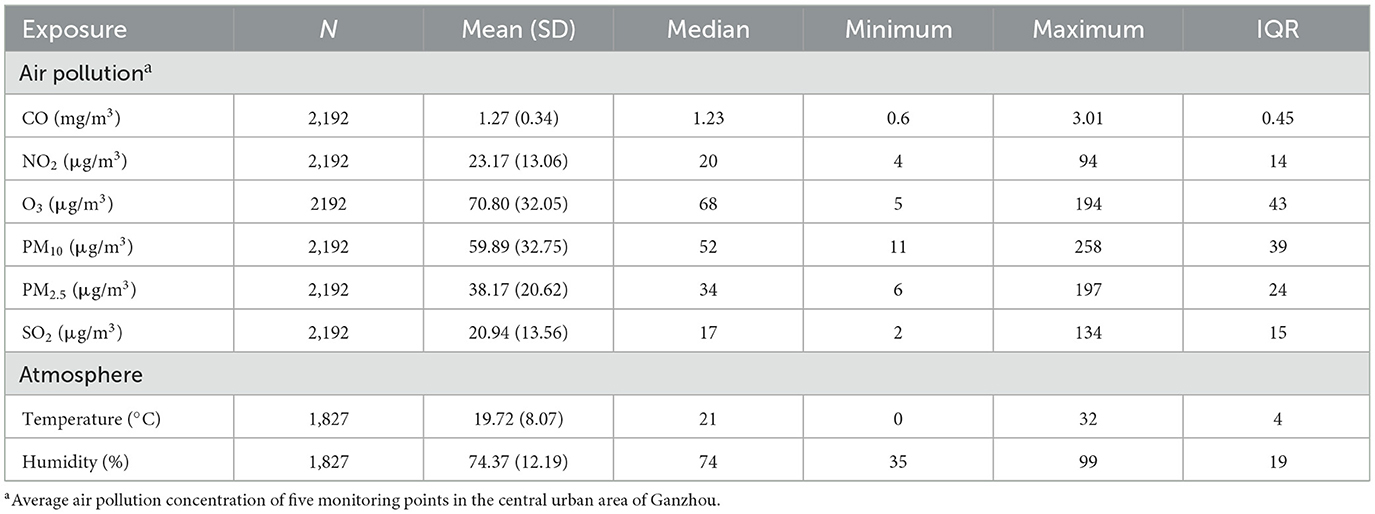
Table 2. General situation of air pollutant concentration in 2015–2020 and temperature and humidity in 2016–2020.
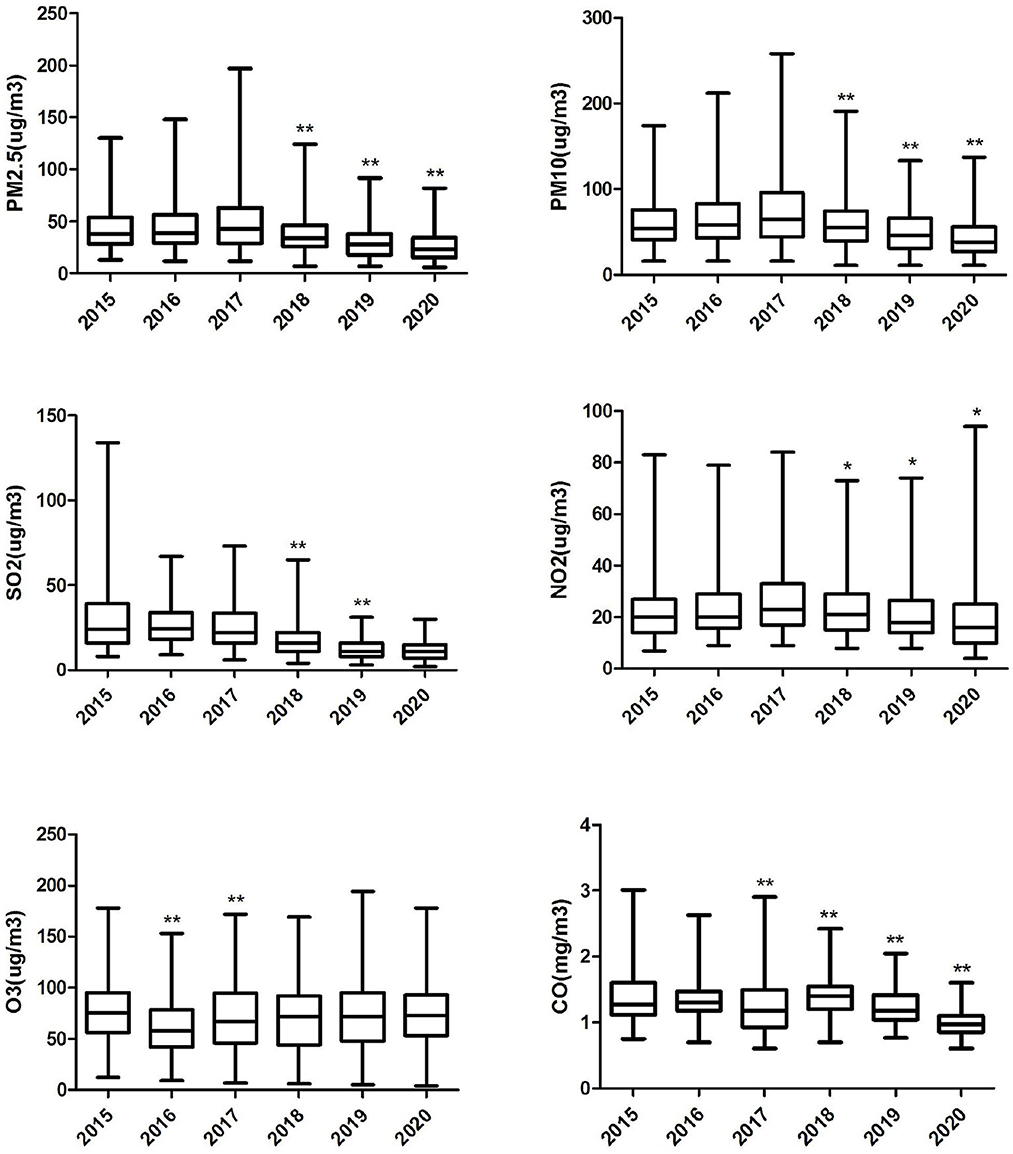
Figure 2. Box plot of annual air pollution concentration in Ganzhou City, 2015–2020. “*” Differences between pollutant concentrations in the marker year and the previous year 0.001≤P< 0.05. “**” Differences between pollutant concentrations in the marker year and the previous year P < 0.001.
As shown in Table 3, positive correlations were observed among all air pollutants except O3, for which ambient particulate matter had stronger correlations with NO2 and SO2. There was a negative correlation between both air temperature and humidity and with ambient particulate matter, but positive correlations between air temperature and O3 or SO2.as well as humidity and CO were observed.
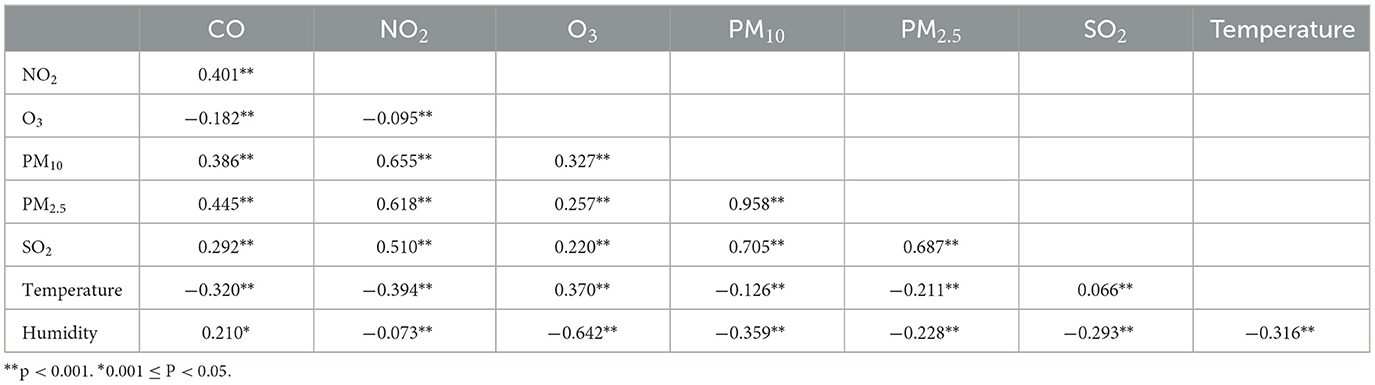
Table 3. Spearman correlation analysis between air pollutants and meteorological factors in Ganzhou City.
3.3. Blood lipids effects on hypertension inpatients with arteriosclerosis
As shown in Figure 3, it was observed in the hypertensive population with arteriosclerosis that PM2.5 and PM10 maintained a significant positive correlation with TC starting from lag0-59. At lag0-209, every 10 μg/m3 increase in PM2.5 and PM10 caused 41.8% (95%CI: 19.39, 68.42) and 25.25% (95%CI: 12.67, 39.23) increases in TC concentration, respectively. From lag 0-179, HDL-C maintained a long negative correlation with PM2.5 and PM10. At lag 0-239, ambient particulate matter had the most significant effect on HDL-C, and every 10μg/m3 increase in PM2.5 and PM10 caused 5.61% (95%CI: 1.76, 9.31) and 3.42 (95%CI: 1.04, 5.74) decrease in HDL-C, respectively. The effects of PM2.5 and PM10 on LDL-C and TG in hospitalized patients with hypertension and arteriosclerosis were not observed with a lag of 359 days in the moving average of ambient particulate matter exposure. Significant positive correlations between PM2.5 and PM10 and Lp(a) were observed only at lag0-359. Lp(a) increased by 38.52% (95%CI: 5.09, 82.59) and 26.47% (95%CI: 6.03, 50.84) for each 10 μg/m3 increase in PM2.5 and PM10, respectively.
3.4. Blood lipids effects on hypertension inpatients without arteriosclerosis
TC and TG in hypertension inpatients without arteriosclerosis were not associated with ambient particulate matter exposure during the study period. As show in Figure 4, HDL-C and LDL-C were both significantly positively correlated with ambient particulate matter at lag0-6, and HDL-C showed a positive correlation with ambient particulate matter again at lag0-89 to lag0-209. During the study period, PM2.5 was positively correlated with Lp(a) concentration in hypertensive population without arteriosclerosis only at lag 0-359. Lp(a) increased by 29.19% (95%CI: 8.75, 53.46) for every 10 μg/m3 increase in PM2.5. PM10 showed a positive correlation with Lp(a) concentrations in this population at various time periods, including lag0-6, lag0-59 to lag0-89, and lag0-299 days later. These results revealed that hypertension inpatients without arteriosclerosis has better statement than hypertension inpatients with arteriosclerosis on human lipid metabolism.
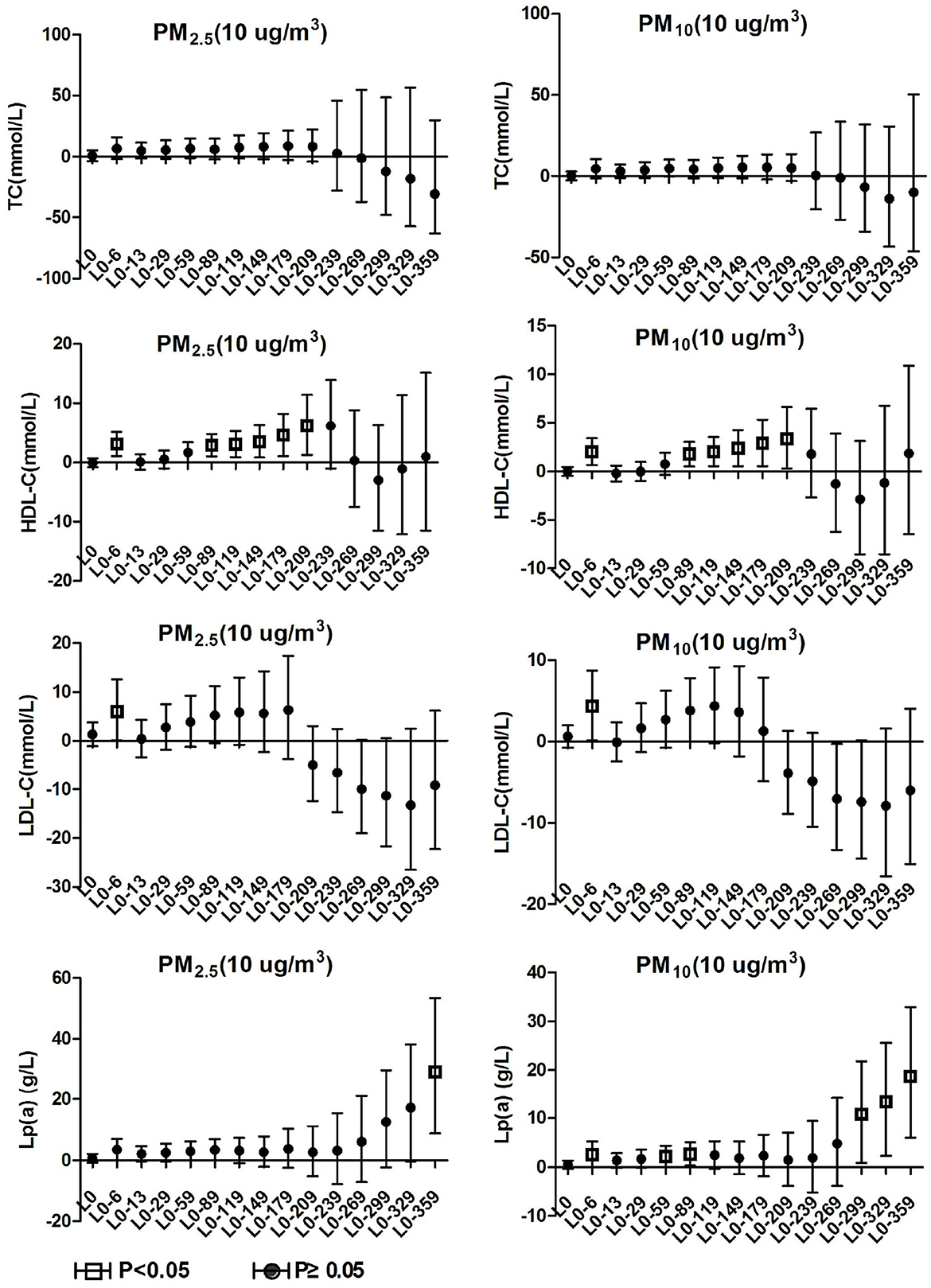
Figure 4. Effect of PM2.5 and PM10 on blood lipid in hypertension inpatients without arteriosclerosis.
3.5. Blood lipids effects on total hypertension inpatients
As show in Figure 5, PM2.5 and PM10 were significantly positively correlated with TC levels in total hypertensive patients at lag0-119 to lag0-239 and lag0-89 to lag0-239, respectively. For every 10 μg/m3 increase in PM2.5 and PM10 at lag 0-209, TC in total hypertensive patients increased by 16.77% (95%CI: 5.52, 9.22) and 10.54% (95%CI: 3.60, 17.94), respectively. PM2.5 and PM10 exposures were positively correlated with Lp(a) concentrations in hypertensive patients from lag0-29 to lag0-119, the correlation disappeared at lag0-149, and then reappeared from lag0-359 and lag0-229, respectively. Lp(a) concentrations in patients with a 10μg/m3 increase in PM2.5 and PM10 at lag0-359 increased by 31.41% (95%CI: 10.16, 56.76) and 22.25% (95%CI: 9.16, 36.91), respectively. PM2.5 and PM10 were negatively correlated with HDL-C levels in this population at lag0-239 to lag0-229 and lag0-209 to lag0-229, respectively. For each 10 μg/m3 increase in PM2.5 and PM10 at lag 0-239, HDL-C concentrations in patients decreased by 3.63% (95%CI: 1.71, 5.50) and 2.49% (95%CI: 1.26, 3.69), respectively. No correlation was observed between ambient particulate matter exposure and LDL-C and TG in the total hypertensive population.
3.6. Blood lipids effects after adjusting for gaseous pollutants
The positive correlation between ambient particulate matter and TC in the population with total hypertension and hypertension with arteriosclerosis remained unchanged after adjusting for gaseous pollutants, but it disappeared significantly after adjusting for NO2 concentration. The positive correlation between particulate matter and Lp(a) of the three groups of people remained unchanged after adjusting for the effects of gaseous pollutants, but the significant effects of particulate matter disappeared after adjusting for SO2, O3 and NO2. In the total hypertensive population, ambient particulate matter was negatively correlated with HDL-C, and the correlation changed positively after CO adjustment. The correlation between PM10 and HDL-C changed from positive to negative after adjusting for NO2 and SO2 in the hypertensive population without arteriosclerosis. The positive correlation between ambient particulate matter and LDL-C levels in hypertensive patients without arteriosclerosis was reversed after adjusting for CO and NO2, but there was not significant. The detailed results are shown in Figure 6. These results revealed that long-term exposure to ambient particulate matter is associated with adverse lipid profile changes in hypertension inpatients, especially those with arteriosclerosis
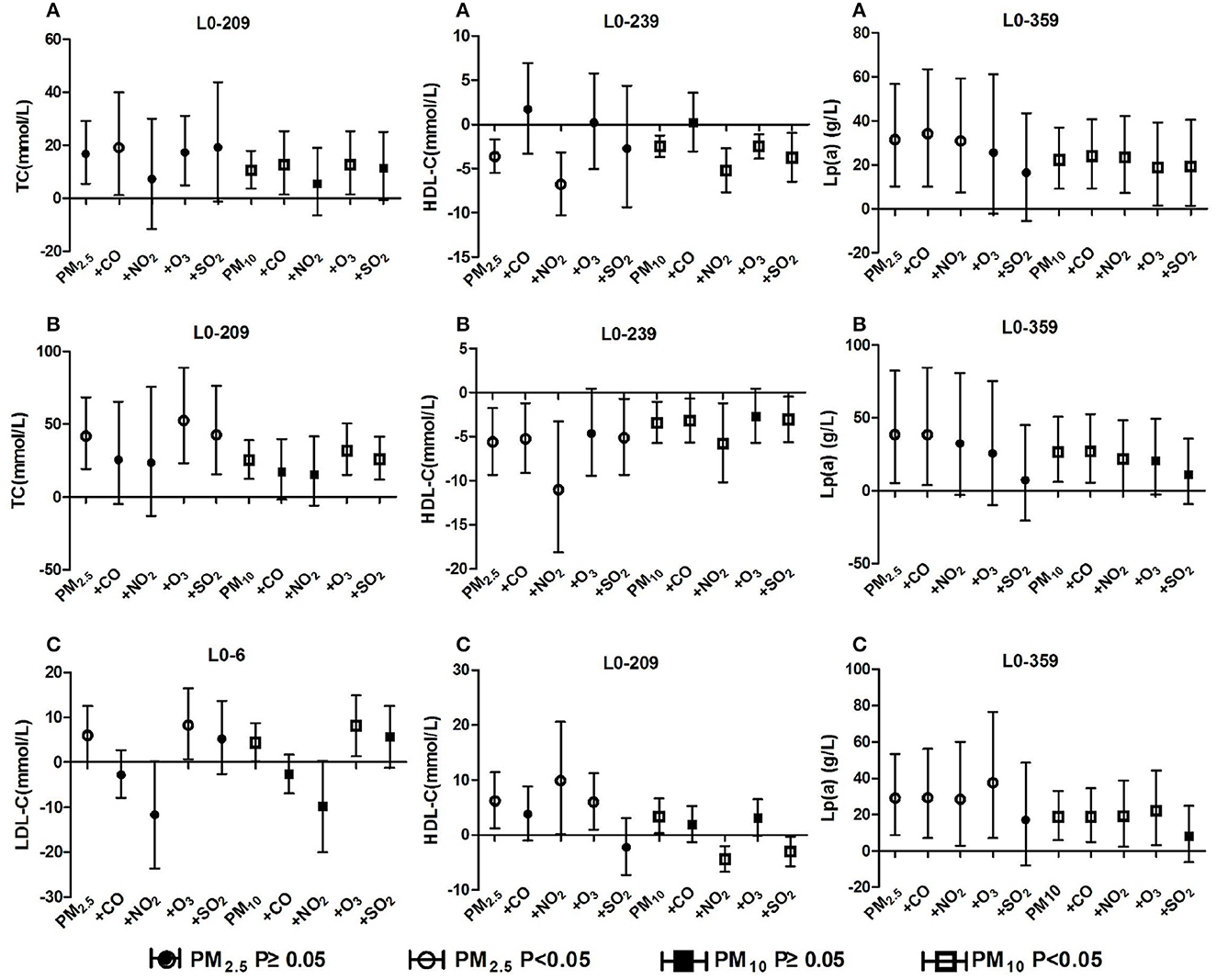
Figure 6. Effect of ambient particulate matter on blood lipid after adjusting for gaseous pollutants. (A) Effect of ambient particulate matter on blood lipid after adjusting for gaseous pollutants in total hypertensive inpatients, (B) Effect of ambient particulate matter on blood lipid after adjusting for gaseous pollutants in hypertension inpatients with arteriosclerosis, (C) Effect of ambient particulate matter on blood lipid after adjusting for gaseous pollutants in hypertension inpatients without arteriosclerosis. +CO, +NO2, +O3, +SO2 two-pollutant model after adjusting for the concentration of CO, NO2, O3, and SO2 respectively.
4. Discussion
In recent years, Ganzhou has stepped up its air pollution control actions. Since 2017, the concentration of ambient particulate matter has decreased significantly year by year. Ganzhou City belongs to southern China and has not established a unified heating system. However, in the cold winter, residents will generally have individual combustion or energy-consuming heating behaviors, resulting in an increase in the emission of ambient particulate matter and a negative correlation between the concentration of particulate matter and meteorological factors. In addition, the low temperatures in winter may cause poor diffusion of pollutants in the air, leading to widespread peaks of pollutants in the cold season (26). Similar to most southern cities, the main sources of ambient particulate matter emissions in Ganzhou are construction dust and vehicle exhaust from urbanization. From 2015 to 2020, the average daily concentration of PM10 in Ganzhou was 59.89 μg/m3, which was higher than the first-level standard of the national ambient air quality standard (GB3095-2012), but lower than the second-level standard (the first-level standard was 40 μg/m3, and the second-level standard was 70 μg/m3). The average daily concentration of PM2.5 was 38.17 μg/m3, which was higher than the NAQS secondary standard (15 μg/m3 for the primary standard and 35 μg/m3 for the secondary standard).
Total cholesterol (TC) refers to the sum of cholesterol contained by all lipoproteins in the blood, including free cholesterol and cholesterol esters. Most of the cholesterol is mainly synthesized by the body itself and from food sources as a minor supplement. There are these lipid transfer proteins which are HDL-C, LDL-C, and very low-density lipoprotein cholesterol. High density lipoprotein (HDL) can uptake low-density lipoprotein, cholesterol, triglycerides, sedimented from the intimal lining of the vessel wall for excretion to the liver, which helps to resist the stiffening of blood vessels caused by hyperlipidemia (27) and maintain cardiovascular health (28–30). Low density lipoprotein (LDL) is a lipoprotein particle that carries cholesterol into tissue cells and carries cholesterol accumulation across the arterial wall to cause arteriosclerosis (31). Lipoprotein [Lp (a)] is synthesized by the liver and is a specialized cholesterol rich macromolecular lipoprotein that promotes atherosclerosis (32).
This study found differences in the effects of ambient particulate matter on TC, HDL-C, LDL-C, and Lp (a) content among hypertension with or without arteriosclerosis. Combined with the analysis of biochemical associations among lipid markers to identify differences in the associations of each lipid marker with ambient particulate matter among different populations, we raise several conjectures.
Ambient particulate matter exposure exhibited health hazard effects of increasing TC levels and decreasing HDL-C levels for both the hypertensive with arteriosclerosis population and the total hypertensive population. However, in the hypertensive without arteriosclerosis population, there are no association with TC levels was present but increasing HDL-C levels emerged with ambient particulate matter exposure. There was a similar trend for Lp (a) levels to increase with higher ambient particulate matter concentrations in the three groups. A positive association with short-term particulate matter exposure was observed only in people with hypertension without arteriosclerosis. It is speculated that ambient particulate matter exposure mainly cause increased cholesterol and lipoprotein synthesis and decreased high-density lipoprotein synthesis in hypertensive patients. But in patients without arteriosclerosis, the regulatory mechanisms of lipid homeostasis are normal, so that lipid transfer proteins such as HDL-C and LDL-C were temporarily increases to maintain stable total cholesterol levels. When cholesterol synthesis continues increasing with long-term exposure, beneficial HDL-C is more producted to metabolize excess cholesterol. Meanwhile LDL-C is inhibited temporarily to protect blood vessels. The phenomenon of compensatory increases in HDL-C and LDL-C with ambient particulate matter exposure has thus emerged in hypertensive population without vascular stiffening.
Long-term exposure to air pollution was found to be associated with increased TC and LP (a) levels in the elderly, obese adolescents and cardiovascular disease (CVD) patients in other studies on associations between air pollution and lipid concentrations (33–35), and additional reports showed that lower HDL-C levels were associated with particulate matter exposure (19, 34, 36). Two studies of the association between air pollution and blood lipids in adults in China both showed that particulate matter had a deleterious effect on blood lipid markers, and this effect was more pronounced in people who were overweight or obese (37). These studies are similar to the results of ambient particulate matter and lipid-related changes that we observed in hypertensive patients with arteriosclerosis and total hypertension. Particulate matter exposure may have a stronger effect on raising TC and lowering HDL-C in individuals already at high risk, a study of older U.S. men suggests (38). This corresponds to our findings and conjectures.
The cardiovascular harm of ambient particulate matter exposure has been widely confirmed, and it has been proposed that oxidative stress (39, 40), inflammatory response (41, 42), and DNA methylation (43, 44) are the main damage mechanisms. Dyslipidemia is the most sensitive metabolic risk factor to air pollution exposure (45). Ambient particulate matter can cause systemic inflammation and oxidative stress, inducing adverse lipid metabolism and oxidation (46). The inflammatory response further mediates a lipid compensatory response against the invasion of hazardous substances from ambient particulate matter and aids tissue repair. When the repair of injury is saturated by lipid compensatory responses, cascading repetitive stimulation of the inflammatory response enhances atherosclerotic lesion formation (47). Additionally ambient particulate matter can also lead to specific gene methylation related to lipid metabolism by reducing the activity of DNA methyltransferases (48). The above studies exhibited different mechanistic pathways by which ambient particulate matter altered blood lipids, which could directly provoke adverse lipid metabolism and also provoke lipid compensatory responses in the pre hazard period, corresponding to the differences in relevant lipid changes among different populations in this study and the speculation raised previously.
Two-pollutant model calculation found that the correlations between PM10 and PM2.5 exposures and adverse changes in blood lipid markers in the total hypertensive population and the hypertensive patients with arteriosclerosis remained basically stable after adjusting for the four types of gaseous pollutants respectively. Moreover, the blood lipid changes related to PM10 exposure were more significant than those of PM2.5 in the dual-pollutant model. Therefore, we believe that compared with gaseous pollutants, ambient particulate matter exposure is more strongly associated with increased TC, Lp(a) and decreased HDL-C, and the harmful effect of PM10 is greater than that of PM2.5. A longitudinal study in Shijiazhuang, China also suggests that ambient particulate matter may have a greater impact on lipid health than gaseous pollutants (49). In a hypertensive population with arteriosclerosis, the positive associations between ambient particulate matter exposure and HDL-C and LDL-C were partially reversed after adjustment for gaseous pollutants. Based on the results of the dual-pollutant model, we believe that ambient particulate matter exposure is not the main pollutant affecting the elevated HDL-C and LDL-C concentrations in this population. Combined with the previous conjecture, we suggest that the phenomenon of higher HDL-C associated with particulate matter exposure in people with hypertension without vascular stiffness, on the one hand, may be due to compensatory increases in HDL-C caused by the body's own protective mechanisms against lipid homeostasis. On the other hand, gaseous pollutants, which were positively correlated with each other and with particulate matter, exhibited stronger associations with HDL-C elevation. Based on the above results, we speculate that ambient particulate matter exposure can cause adverse changes in blood lipid levels in hypertensive patients and increase the risk of arteriosclerosis events.
This study has several strengths. First, we applied the detailed blood lipid detection data of inpatients collected by the hospital's big data center, and the real-time monitoring data of air pollution concentration collected by the environmental protection department to ensure the accuracy of the data source. We selected hypertensive patients as research subjects and grouped them according to whether they were accompanied by arteriosclerosis, so as to study the correlation between ambient particulate matter exposure and the risk of blood lipids in hypertensive patients with different disease states. Second, the data analysis applied a semiparametric generalized additive model. After adjusting for the effects of gender, age, weather, and time, the correlation between ambient particulate matter pollution and blood lipids in different lag time periods in a year was more accurately explored, and the relationship between each blood lipid index and air pollution exposure was calculated. Third, this study is the first to explore the relationship between air pollution and human blood lipid concentrations in southern Jiangxi, China, and its findings can provide reference for other regions with similar development levels and geographic latitudes.
This study also has some limitations. First, in addition to environmental factors, blood lipid levels are affected by a variety of factors, including genetics, behavior, and medication (50, 51). This study was not able to collect this information, so the results ignore the role of some valuable confounding factors and may be biased. Secondly, the air pollution concentration is the average of the data of the five monitoring points in Ganzhou City, and it is impossible to accurately understand the actual exposure concentration of air pollution of each research object. This exposed misclassification is likely to reduce the significance of the association (52). In addition, our study only analyzed the lipid effects associated with particulate matter exposure during a one-year period, and did not investigate the effect of a longer lag. Significant associations of LP (a) with ambient PM generally appeared at lag 359 days. Looking at the trends of LP (a) changes associated with exposure at different lag times, more significant associations with LP (a) increases may occur at longer exposure times.
5. Conclusions
Ambient particulate matter exposure was associated with higher TC, LP (a) and lower HDL-C in hypertensive patients, and PM10 exposure was more strongly associated with changes in lipid markers than PM2.5. There are association of long-term exposure to ambient particulate matter with the risk of arteriosclerosis in hypertensive patients, and such impacts have stronger harmful effects in patients with arteriosclerosis.
Data availability statement
The raw data supporting the conclusions of this article will be made available by the authors, without undue reservation.
Ethics statement
The studies involving human participants were reviewed and approved by the Ethics Committee of Gannan Medical University. The patients/participants provided their written informed consent to participate in this study. Written informed consent was obtained from the individual(s) for the publication of any potentially identifiable images or data included in this article.
Author contributions
YG: conceptualization, writing–reviewing and editing, and funding acquisition. CL and LH: methodology, software, data curation, formal analysis, writing–original draft, review, and editing. XZho: software, formal analysis, investigation, and data curation. KH and MG: sensitivity analysis. XZha: conceptualization, data acquisition, supervision, and funding acquisition. All authors contributed to the article and approved the submitted version.
Funding
This project was supported by the National Natural Science Foundation of China (No. 81903364); the National Natural Science Foundation of Jiangxi Province (20202BABL206123); the Open Project of Key Laboratory of Prevention and Treatment of Cardiovascular and Cerebrovascular Diseases, Ministry of Education (No. XN201918). This work was funded by Gannan Medical University (QD201901) and Department of Education of Jiangxi Province (GJJ190786).
Conflict of interest
The authors declare that the research was conducted in the absence of any commercial or financial relationships that could be construed as a potential conflict of interest.
Publisher's note
All claims expressed in this article are solely those of the authors and do not necessarily represent those of their affiliated organizations, or those of the publisher, the editors and the reviewers. Any product that may be evaluated in this article, or claim that may be made by its manufacturer, is not guaranteed or endorsed by the publisher.
References
1. Nemery B, Hoet PH, Nemmar A. The meuse valley fog of 1930: an air pollution disaster. Lancet. (2001) 357:704–8. doi: 10.1016/S0140-6736(00)04135-0
2. Brauer M, Amann M, Burnett RT, Cohen A, Dentener F, Ezzati M, et al. Exposure assessment for estimation of the global burden of disease attributable to outdoor air pollution. Environ Sci Technol. (2012) 46:652–60. doi: 10.1021/es2025752
3. Neira MP. Air pollution and human health: a comment from the World Health Organization. Annals of Global Health. (2019) 85:141. doi: 10.5334/aogh.2712
4. Wang X, Leng M, Liu Y, Qian ZM, Zhang J, Li Z, et al. Different sized particles associated with all-cause and cause-specific emergency ambulance calls: a multicity time-series analysis in China. Sci Total Environ. (2021) 783:147060. doi: 10.1016/j.scitotenv.2021.147060
5. Zeng Q, Li P, Ni Y, Li GX, Wang DZ, Pan XC, et al. Research on the relationship between atmospheric inhalable particulate matter and cardiovascular diseases burden in Tianjin] Zhonghua Xin Xue Guan Bing Za Zhi. (2018) 46:50–5. doi: 10.3760/cma.j.issn.0253-3758.2018.01.009
6. Tian Y, Liu H, Wu Y, Si Y, Song J, Cao Y, et al. Association between ambient fine particulate pollution and hospital admissions for cause specific cardiovascular disease: time series study in 184 major Chinese cities. BMJ. (2019) 367:l6572. doi: 10.1136/bmj.l6572
7. Soldevila BN, Vinyoles BE, Agudo UJ, Camps VL. Air pollution, cardiovascular risk and hypertension. Hipertens Riesgo Vasc. (2018) 35:177–84. doi: 10.1016/j.hipert.2018.03.001
8. Mannucci PM, Ancona C. Noise and air pollution as triggers of hypertension. Eur Heart J. (2021) 42:2085–7. doi: 10.1093/eurheartj/ehab104
9. Mao S, Chen G, Liu F, Li N, Wang C, Liu Y, et al. Long-term effects of ambient air pollutants to blood lipids and dyslipidemias in a Chinese rural population. Environ Pollut. (2020) 256:113403. doi: 10.1016/j.envpol.2019.113403
10. Islam T, Gauderman WJ, Berhane K, McConnell R, Avol E, Peters JM, et al. Relationship between air pollution, lung function and asthma in adolescents. Thorax. (2007) 62:957–63. doi: 10.1136/thx.2007.078964
11. Hwang BF, Chen YH, Lin YT, Wu XT, Leo LY. Relationship between exposure to fine particulates and ozone and reduced lung function in children. Environ Res. (2015) 137:382–90. doi: 10.1016/j.envres.2015.01.009
12. Spyratos D, Sioutas C, Tsiotsios A, Haidich AB, Chloros D, Triantafyllou G, et al. Effects of particulate air pollution on nasal and lung function development among Greek children: a 19-year cohort study. Int J Environ Health Res. (2015) 25:480–9. doi: 10.1080/09603123.2014.979775
13. Franssen R, Monajemi H, Stroes ES, Kastelein JJ. Obesity and dyslipidemia. Med Clin North Am. (2011) 95:893–902. doi: 10.1016/j.mcna.2011.06.003
14. Lee MH, Kim HC, Ahn SV, Hur NW, Choi DP, Park CG, et al. Prevalence of dyslipidemia among Korean adults: Korea National Health and Nutrition Survey 1998-2005. Diabetes Metabol J. (2012) 36:43–55. doi: 10.4093/dmj.2012.36.1.43
15. Kulkarni H, Mamtani M, Blangero J, Curran JE. Lipidomics in the study of hypertension in metabolic syndrome. Curr Hypertens Rep. (2017) 19:7. doi: 10.1007/s11906-017-0705-6
16. Da SC, Di Domenico M, Hilario NSP, Ramos RC. Subchronic air pollution exposure increases highly palatable food intake, modulates caloric efficiency and induces lipoperoxidation. Inhal Toxicol. (2018) 30:370–80. doi: 10.1080/08958378.2018.1530317
17. Heydari H, Abroudi M, Adli A, Pirooznia N, Najafi ML, Pajohanfar NS, et al. Maternal exposure to ambient air pollution during pregnancy and lipid profile in umbilical cord blood samples; a cross-sectional study. Environ Pollut. (2020) 261:114195. doi: 10.1016/j.envpol.2020.114195
18. Kim KN, Ha B, Seog W, Hwang IU. Long-term exposure to air pollution and the blood lipid levels of healthy young men. Environ Int. (2022) 161:107119. doi: 10.1016/j.envint.2022.107119
19. Poursafa P, Mansourian M, Motlagh ME, Ardalan G, Kelishadi R. Is air quality index associated with cardiometabolic risk factors in adolescents? The CASPIAN-III study. Environ Res. (2014) 134:105–9. doi: 10.1016/j.envres.2014.07.010
20. Sorensen M, Hjortebjerg D, Eriksen KT, Ketzel M, Tjonneland A, Overvad K, et al. Exposure to long-term air pollution and road traffic noise in relation to cholesterol: a cross-sectional study. Environ Int. (2015) 85:238–43. doi: 10.1016/j.envint.2015.09.021
21. Hong X, Zhang B, Liang L, Zhang Y, Ji Y, Wang G, et al. Postpartum plasma metabolomic profile among women with preeclampsia and preterm delivery: implications for long-term health. BMC Med. (2020) 18:277. doi: 10.1186/s12916-020-01741-4
22. Mura MC, De Felice M, Morlino R, Fuselli S. Short-term monitoring of benzene air concentration in an urban area: a preliminary study of application of Kruskal-Wallis non-parametric test to assess pollutant impact on global environment and indoor. Ann Ist Super Sanita. (2010) 46:444–50. doi: 10.4415/ANN.10.04.13
23. Tian H, Xu B, Wang X, Wang J, Zhong C. Study on the correlation between ambient environment-meteorological factors and the number of visits of acute otitis media, Lanzhou, China. J Otol. (2020) 15:86–94. doi: 10.1016/j.joto.2020.01.002
24. Forbes LJ, Patel MD, Rudnicka AR, Cook DG, Bush T, Stedman JR, et al. Chronic exposure to outdoor air pollution and markers of systemic inflammation. Epidemiology. (2009) 20:245–53. doi: 10.1097/EDE.0b013e318190ea3f
25. Chen SY, Chan CC, Su TC. Particulate and gaseous pollutants on inflammation, thrombosis, and autonomic imbalance in subjects at risk for cardiovascular disease. Environ Pollut. (2017) 223:403–8. doi: 10.1016/j.envpol.2017.01.037
26. Masson O, Steinhauser G, Wershofen H, Mietelski JW, Fischer HW, Pourcelot L, et al. Potential source apportionment and meteorological conditions involved in airborne (131)I Detections in January/February 2017 in Europe. Environ Sci Technol. (2018) 52:8488–500. doi: 10.1021/acs.est.8b01810
27. van Capelleveen JC, Bochem AE, Motazacker MM, Hovingh GK, Kastelein JJ. Genetics of HDL-C: A causal link to atherosclerosis? Curr Atheroscler Rep. (2013) 15:326. doi: 10.1007/s11883-013-0326-8
28. Collins P, Webb CM, de Villiers TJ, Stevenson JC, Panay N, Baber RJ. Cardiovascular risk assessment in women - an update. Climacteric. (2016) 19:329–36. doi: 10.1080/13697137.2016.1198574
29. Jabir NR, Siddiqui AN, Firoz CK, Ashraf GM, Zaidi SK, Khan MS, et al. Current updates on therapeutic advances in the management of cardiovascular diseases. Curr Pharm Des. (2016) 22:566–71. doi: 10.2174/1381612822666151125000746
30. Townsend N, Wilson L, Bhatnagar P, Wickramasinghe K, Rayner M, Nichols M. Cardiovascular disease in Europe: epidemiological update 2016. Eur Heart J. (2016) 37:3232–45. doi: 10.1093/eurheartj/ehw334
31. Karagiannis AD, Mehta A, Dhindsa DS, Virani SS, Orringer CE, Blumenthal RS, et al. How low is safe? The frontier of very low (<30 mg/dL) LDL cholesterol. Eur Heart J. (2021) 42:2154–69. doi: 10.1093/eurheartj/ehaa1080
32. Jang AY, Han SH, Sohn IS, Oh PC, Koh KK. Lipoprotein(a) and Cardiovascular Diseases- Revisited. Circ J. (2020) 84:867–74. doi: 10.1253/circj.CJ-20-0051
33. Chuang KJ, Yan YH, Chiu SY, Cheng TJ. Long-term air pollution exposure and risk factors for cardiovascular diseases among the elderly in Taiwan. Occup Environ Med. (2011) 68:64–8. doi: 10.1136/oem.2009.052704
34. Ghosh R, Gauderman WJ, Minor H, Youn HA, Lurmann F, Cromar KR, et al. Air pollution, weight loss and metabolic benefits of bariatric surgery: a potential model for study of metabolic effects of environmental exposures. Pediatr Obes. (2018) 13:312–20. doi: 10.1111/ijpo.12210
35. McGuinn LA, Schneider A, McGarrah RW, Ward-Caviness C, Neas LM, Di Q, et al. Association of long-term PM2.5 exposure with traditional and novel lipid measures related to cardiovascular disease risk. Environ Int. (2019) 122:193–200. doi: 10.1016/j.envint.2018.11.001
36. Chuang KJ, Yan YH, Cheng TJ. Effect of air pollution on blood pressure, blood lipids, and blood sugar: a population-based approach. J Occup Environ Med. (2010) 52:258–62. doi: 10.1097/JOM.0b013e3181ceff7a
37. Yang BY, Bloom MS, Markevych I, Qian ZM, Vaughn MG, Cummings-Vaughn LA, et al. Exposure to ambient air pollution and blood lipids in adults: The 33 Communities Chinese Health Study. Environ Int. (2018) 119:485–92. doi: 10.1016/j.envint.2018.07.016
38. Bind MA, Peters A, Koutrakis P, Coull B, Vokonas P, Schwartz J. Quantile regression analysis of the distributional effects of air pollution on blood pressure, heart rate variability, blood lipids, and biomarkers of inflammation in elderly American men: the normative aging study. Environ Health Perspect. (2016) 124:1189–98. doi: 10.1289/ehp.1510044
39. Zhao L, Zhang L, Chen M, Dong C, Li R, Cai Z. Effects of Ambient Atmospheric PM2.5, 1-Nitropyrene and 9-Nitroanthracene on DNA Damage and Oxidative Stress in Hearts of Rats. Cardiovasc Toxicol. (2019) 19:178–190. doi: 10.1007/s12012-018-9488-5
40. Long YM, Yang XZ, Yang QQ, Clermont AC, Yin YG, Liu GL, et al. PM2.5 induces vascular permeability increase through activating MAPK/ERK signaling pathway and ROS generation. J Hazard Mater. (2020) 386:121659. doi: 10.1016/j.jhazmat.2019.121659
41. Xu X, Wang H, Liu S, Xing C, Liu Y, Zhou W, et al. TP53-dependent autophagy links the ATR-CHEK1 axis activation to proinflammatory VEGFA production in human bronchial epithelial cells exposed to fine particulate matter (PM2.5). Autophagy. (2016) 12:1832–48. doi: 10.1080/15548627.2016.1204496
42. Hu H, Asweto CO, Wu J, Shi Y, Feng L, Yang X, et al. Gene expression profiles and bioinformatics analysis of human umbilical vein endothelial cells exposed to PM2.5. Chemosphere. (2017) 183:589–98. doi: 10.1016/j.chemosphere.2017.05.153
43. Jiang Y, Li J, Ren F, Ji C, Aniagu S, Chen T. PM2.5-induced extensive DNA methylation changes in the heart of zebrafish embryos and the protective effect of folic acid. Environ Pollut. (2019) 255:113331. doi: 10.1016/j.envpol.2019.113331
44. Zhao L, Zhang M, Bai L, Zhao Y, Cai Z, Yung K, et al. Real-world PM2.5 exposure induces pathological injury and DNA damage associated with miRNAs and DNA methylation alteration in rat lungs. Environ Sci Pollut Res Int. (2022). 29:28788–803. doi: 10.1007/s11356-021-17779-7
45. Yang BY, Guo Y, Markevych I, Qian ZM, Bloom MS, Heinrich J, et al. Association of long-term exposure to ambient air pollutants with risk factors for cardiovascular disease in China. JAMA Netw Open. (2019) 2:e190318. doi: 10.1001/jamanetworkopen.2019.0318
46. Shanley RP, Hayes RB, Cromar KR, Ito K, Gordon T, Ahn J. Particulate air pollution and clinical cardiovascular disease risk factors. Epidemiology. (2016) 27:291–8. doi: 10.1097/EDE.0000000000000426
47. Esteve E, Ricart W, Fernandez-Real JM. Dyslipidemia and inflammation: an evolutionary conserved mechanism. Clin Nutr. (2005) 24:16–31. doi: 10.1016/j.clnu.2004.08.004
48. Chen R, Meng X, Zhao A, Wang C, Yang C, Li H, et al. DNA hypomethylation and its mediation in the effects of fine particulate air pollution on cardiovascular biomarkers: a randomized crossover trial. Environ Int. (2016) 94:614–9. doi: 10.1016/j.envint.2016.06.026
49. Zhang K, Wang H, He W, Chen G, Lu P, Xu R, et al. The association between ambient air pollution and blood lipids: a longitudinal study in Shijiazhuang, China. Sci Total Environ. (2021) 752:141648. doi: 10.1016/j.scitotenv.2020.141648
50. Schoeler M, Caesar R. Dietary lipids, gut microbiota and lipid metabolism. Rev Endocr Metab Disord. (2019) 20:461–72. doi: 10.1007/s11154-019-09512-0
51. Felzer-Kim IT, Visker JR, Ferguson DP, Hauck JL. Infant blood lipids: a systematic review of predictive value and influential factors. Expert Rev Cardiovasc Ther. (2020) 18:381–94. doi: 10.1080/14779072.2020.1782743
Keywords: ambient particulate matter, blood lipid markers, hypertension, arteriosclerosis, generalized additive model (GAM)
Citation: Gao Y, Li C, Huang L, Huang K, Guo M, Zhou X and Zhang X (2023) Effects of ambient particulate exposure on blood lipid levels in hypertension inpatients. Front. Public Health 11:1106852. doi: 10.3389/fpubh.2023.1106852
Received: 25 November 2022; Accepted: 31 January 2023;
Published: 21 February 2023.
Edited by:
Tao Liu, Jinan University, ChinaReviewed by:
Zhi-Hao Li, Southern Medical University, ChinaXiaobo Liu, National Institute for Communicable Disease Control and Prevention (China CDC), China
Copyright © 2023 Gao, Li, Huang, Huang, Guo, Zhou and Zhang. This is an open-access article distributed under the terms of the Creative Commons Attribution License (CC BY). The use, distribution or reproduction in other forums is permitted, provided the original author(s) and the copyright owner(s) are credited and that the original publication in this journal is cited, in accordance with accepted academic practice. No use, distribution or reproduction is permitted which does not comply with these terms.
*Correspondence: Xiaokang Zhang, emhhbmd4aWFva2FqdSYjeDAwMDQwOzE2My5jb20=