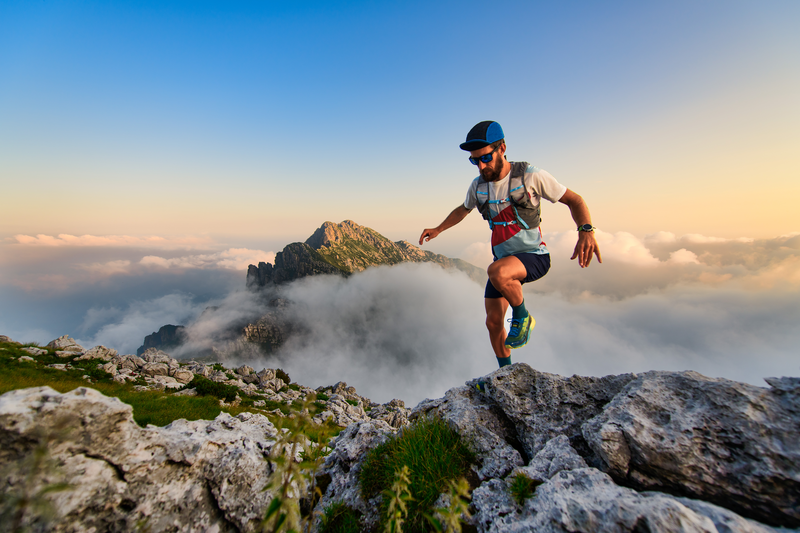
94% of researchers rate our articles as excellent or good
Learn more about the work of our research integrity team to safeguard the quality of each article we publish.
Find out more
REVIEW article
Front. Public Health , 08 August 2022
Sec. Infectious Diseases – Surveillance, Prevention and Treatment
Volume 10 - 2022 | https://doi.org/10.3389/fpubh.2022.945448
This article is part of the Research Topic COVID-19: From Bedside to Follow-up View all 11 articles
The unprecedented worldwide spread of SARS-CoV-2 has imposed severe challenges on global health care systems. The roll-out and widespread administration of COVID-19 vaccines has been deemed a major milestone in the race to restrict the severity of the infection. Vaccines have as yet not entirely suppressed the relentless progression of the pandemic, due mainly to the emergence of new virus variants, and also secondary to the waning of protective antibody titers over time. Encouragingly, an increasing number of antiviral drugs, such as remdesivir and the newly developed drug combination, Paxlovid® (nirmatrelvir/ritonavir), as well as molnupiravir, have shown significant benefits for COVID-19 patient outcomes. Pre-exposure prophylaxis (PrEP) has been proven to be an effective preventive strategy in high-risk uninfected people exposed to HIV. Building on knowledge from what is already known about the use of PrEP for HIV disease, and from recently gleaned knowledge of antivirals used against COVID-19, we propose that SARS-CoV-2 PrEP, using specific antiviral and adjuvant drugs against SARS-CoV-2, may represent a novel preventive strategy for high-risk populations, including healthcare workers, immunodeficient individuals, and poor vaccine responders. Herein, we critically review the risk factors for severe COVID-19 and discuss PrEP strategies against SARS-CoV-2. In addition, we outline details of candidate anti-SARS-CoV-2 PrEP drugs, thus creating a framework with respect to the development of alternative and/or complementary strategies to prevent COVID-19, and contributing to the global armamentarium that has been developed to limit SARS-CoV-2 infection, severity, and transmission.
Coronavirus disease 2019 (COVID-19), caused by severe acute respiratory syndrome coronavirus 2 (SARS-CoV-2), has, over the past 2 years, resulted in the death of millions of people globally (1). Moreover, COVID-19 has dramatically affected and altered the lives and livelihoods of people in every corner of the world due to its effects on local, regional, and global health care systems, economies, environments, and geopolitical posturing. During this period, various reactive, adaptive, and defensive coping strategies employed by government authorities, such as regional lockdowns, the use of SARS-CoV-2 vaccines, and antiviral drugs, have been implemented, which have influenced the evolution of the pandemic.
The roll-out of several different COVID-19 vaccines by pharmaceutical companies has been considered a major milestone in the global medical effort to prevent populations from developing severe disease from SARS-CoV-2 infection. With respect to the protective effects of these vaccines, data indicates that cellular immunity induced by COVID-19 vaccines protected against severe infection, even against new SARS-CoV-2 variants (2–4). Keeton et al. reported that the T-cell responses induced by COVID-19 vaccination or previous SARS-CoV-2 infection are cross-reactive with the Omicron variant of SARS-CoV-2, despite extensive mutation and reduced susceptibility to neutralizing antibodies of the Omicron variant (2). Nonetheless, the much anticipated protective effects of COVID-19 vaccines have been found to be limited and transient for two main reasons: (1) Protective and neutralizing antibody levels wane after a few months post vaccination, and (2) The SARS-CoV-2 virus undergoes active genomic mutation, rendering some COVID-19 vaccines functionally obsolete even before they have been utilized at a population level (5–9). One multicenter prospective study, conducted by Favresse et al. observed that vaccine-associated antibody titers decline post-vaccination with the BNT162b2 mRNA COVID-19 vaccine (Pfizer, BioNTech) (Comirnaty®), with an estimated antibody half-life of 55 and 80 days for seropositive and seronegative subjects, respectively (6). It has also been reported that vaccine-associated antibody titers achieve peak levels at 1 month after the second dose of the BNT162b2 mRNA COVID-19 vaccine, and subsequently rapidly decrease over time (9). Moreover, newer variants of SARS-CoV-2 may be evolving into virions capable of vaccine-breakthrough, containing several antibody-resistant mutations, such as the Omicron SARS-CoV-2 variant, which is a heavily-mutated virus variant, and is classified as a variant of concern (VOC) by the World Health Organization (WHO) (10). Based on three-dimensional (3D) renderings of its antibody-receptor-binding domain (RBD) complex structures, Chen et al. has claimed that the Omicron variant has an 88% likelihood of evading antibodies generated by current vaccines (11). The Omicron (B.1.1.529) variant could also increase the risk of SARS-CoV-2 reinfection, which is associated with immune evasion (12). Similarly, Hoffmann et al. have reported that the Omicron variant evades neutralization by antibodies from vaccinated individuals with up to 44-fold higher efficiency than the Delta variant (13). Also, microbial dysbiosis, gut barrier integrity loss, and/or microbial translocation are also thought to be involved in the milieu of COVID-19 disease and poor host immune responses secondary to vaccination (14, 15). This indicates that alternative strategies to vaccination to combat COVID-19, geared toward supplementing and consolidating the existing defensive arsenal, are warranted.
Pre-exposure prophylaxis (PrEP) refers to the utilization of medication/drugs before risk exposure, in order to prevent disease acquisition and transmission, for people at high risk to be infected. PrEP has typically referred to the prevention of HIV infection using specific antiviral agents by a person at risk of HIV acquisition. It has been deemed a cornerstone for HIV prevention. Convergent evidence from prospective clinical trials has demonstrated the efficacy of HIV PrEP in reducing the risk of HIV acquisition, which is known to be up to 98% effective when adherence to treatment is optimal (16–21). Several HIV PrEP drugs and drug combinations have been recommended by the WHO, and these have been employed for use in people at high risk of HIV infection, as a part of the combination HIV prevention approach (22).
With respect to SARS-CoV-2, several antiviral agents have now been investigated and developed that show inhibitory effects against SARS-CoV-2 both in vitro and in vivo, including remdesivir, molnupiravir, and Paxlovid® (nirmatrelvir/ritonavir). In this review, we propose that PrEP using the preceding antiviral drugs, as well as other potentially effective anti-SARS-CoV-2 agents, might be considered to prevent SARS-CoV-2 acquisition in high-risk populations, inspired by the unparalleled success of PrEP in preventing the acquisition of HIV.
Herein, we critically review the risk factors for severe COVID-19, discuss potentially viable SARS-CoV-2 PrEP strategies, as well as their limitations in targeted populations, thus paving the way for the development of an alternative or complementary strategy to prevent SARS-CoV-2 infection and secondary transmission.
The population that has a substantially higher probability to closely interact with SARS-CoV-2-infected individuals, and who would be considered as the group with the highest risk of exposure to SARS-CoV-2 infection, would be healthcare workers. A significantly large number of healthcare workers have already been infected by SARS-CoV-2, and some of these infected individuals experienced poor outcomes, especially at the early stages of the pandemic (23–25). In October 2021, the WHO estimated that, globally, between 80,000 and 180,000 healthcare workers died due to COVID-19 between January 2020 and May 2021 (26–28). Aside from close contact, there are multiple other risk factors, such as older age, presence of comorbidities, environmental factors, and poor vaccine response, which may facilitate SARS-CoV-2 infection and produce unfavorable outcomes, including long-COVID-19.
Convergent investigational observations indicate that susceptibility to and disease severity of COVID-19 are associated with older age, male gender, and ethnicity (29–31). Data from the early stages of the pandemic in US indicates that case-fatality rates increase with age, with <1% of deaths among people aged ≤ 54 years of age, 1.4–4.9% among people aged 55–74 years of age, 4.3–10.5% among people aged 75–84 years, and 10.4–27.3% among people aged ≥ 85 years old (29). Moreover, one global meta-analysis conducted in 2021, which included 59 studies and 36,470 patients, observed that males and the older population have a materially higher risk for SARS-CoV-2 infection, severe disease, and mortality (30). In concordance with these findings, another meta-analysis, which included 14 studies, 29,909 SARS-CoV-2-infected patients, and 1,445 cases of death, indicated that older age (≥65 years old) and male gender were associated with a greater risk of death from COVID-19 infection, with a pooled odds ratio (OR) of 4.59 [95% confidence interval (CI) = 2.61–8.04, ≥65 vs. <65 years old], and 1.50 (95% CI = 1.06–2.12, male vs. female) (31). Older age is unavoidably associated with various other comorbidities, poor immunity, and increased levels of circulating pro-inflammatory cytokines (32). Additional factors, such as differences in levels of circulating sex hormones between men and women, levels of ACE2 enzymes and receptors, the presence of the transmembrane serine protease 2 (TMPRSS2) enzyme, and lifestyle factors such as smoking, may also contribute to variable risks of severity and mortality of COVID-19 in afflicted persons (32, 33).
Generally, it has been considered that non-Caucasian races are associated with increased risk of SARS-CoV-2 infection, disease severity, and mortality, compared to people of Caucasian ancestry (34–36). One meta-analysis, which included 18,728,893 patients from 50 studies, observed that individuals of black and Asian ethnicity are at increased risk of SARS-CoV-2 infection compared to Caucasian individuals, and that Asian individuals are at higher risk of ICU admission and death (34). However, after adjusting for comorbidities, another meta-analysis reported that racial discrepancies observed in risk of SARS-CoV-2 infection rates may actually be attributable to higher comorbidity prevalence in certain racial groups (37).
ABO blood groups have also been found to be associated with COVID-19 susceptibility, severity, and mortality (38–43). Group A individuals showed an increased risk of becoming infected by SARS-CoV-2, compared to group O (39–43). With respect to severity and mortality, Muñiz-Diaz et al. reported that specific ABO blood groups were also seen to represent important risk factors for development of COVID-19, with the risk in Group A individuals being significantly higher than that in Group O individuals (38).
A large volume of published literature has reported that various comorbidities may predispose patients with COVID-19 to an unfavorable outcome, and a higher risk of death (44–51). Hernández-Garduño, after analyzing the data of 32,583 patients, showed that the presence of either obesity, diabetes, or hypertension were strong predictors for both the acquisition of SARS-CoV-2 infection and the development of severe disease (50). COVID-19 clinical guidance issued by The American College of Cardiology indicates that case fatality rates for comorbid COVID-19 patients are substantially higher than the average population, i.e., case fatality rates for comorbid cardiovascular disease (CVD) being 10.5%, diabetes (7.3%), chronic respiratory disease (6.3%), hypertension (6.0%), and cancer (5.6%) (51).
The United States (US) Centers for Disease Control and Prevention (CDC) has released a comorbid medical condition list for COVID-19, and has issued advice stating that having one of the listed conditions may make a person more likely to become severely ill from COVID-19 (52). This updated list includes cancer, chronic kidney disease, chronic liver disease, chronic lung diseases, dementia or other neurological conditions, diabetes, etc. (52). Similarly, several meta-analyses have now confirmed that the listed conditions do indeed predispose individuals to severe illness (53–57). For instance, Thakur et al. published a meta-analysis, which included 120 studies and 125 446 patients, which observed that the most prevalent COVID-19 comorbidities were hypertension (32%), obesity (25%), diabetes (18%), and cardiovascular disease (16%), while patients having renal comorbidities had the highest severity and mortality rates (53).
Accumulating evidence has shown that environmental and climatic factors have a significant effect on COVID-19 transmission and mortality. These factors include population density, temperature, ozone levels, sulfur dioxide levels, humidity, wind speed, and rainfall levels (58–65).
Yin et al. analyzed the data of cities in China and in the USA, and observed that a higher population density was associated with a higher percentage of morbidity related to COVID-19, indicating the importance of social distancing and travel/movement restrictions for the prevention of COVID-19 transmission (65). Sobral et al. investigated the association between climatic conditions and global SARS-CoV-2 transmission, and found that, aside from prevailing average temperature levels, countries with higher rainfall measurements showed an increase in COVID-19 transmission, with each average inch/day of rainfall equating to an additional 56.01 newly-identified COVID-19 cases/day (62). Generally, higher population density, air pollution, rainfall, and wind speed, as opposed to lower temperature, humidity and sulfur dioxide levels are associated with higher COVID-19 infection and severity rates (58–65).
The development of protective immunity after COVID-19 vaccination relies on long-term B- and T-cell memory responses to SARS-CoV-2 (66, 67). Immunosuppressed patients, such as those with immunodeficiency, organ transplant recipients, untreated HIV-infected patients, and cancer patients who have B- or T-cell deficiency are more likely to develop severe COVID-19 (68, 69). Goubet et al. reported that the lymphopenia was associated with prolonged SARS-CoV-2 RNA virus shedding and poor prognosis in cancer patients (70). In SARS-CoV-2 susceptible individuals, Fahrner et al. (67) observed a specific deficit in the TH1/Tc1 response against the receptor binding domain of the spike protein (S1-RBD), and vaccine-induced S1-RBD TH1 immunity is reduced in hematological malignancies. Fernandez Salinas et al. (71) reported that only 33% of patients with common variable immunodeficiency (CVID) showed an antibody response to the COVID-19 vaccine; moreover, CVID could not generate RBD-specific MBCs even after two vaccine doses, compared to healthy vaccinated individuals. Amodio et al. (69) reported that five patients with inborn errors of immunity (IEI) did not mount any cellular response, as is usually observed in healthy individuals, following the BNT162b2 mRNA COVID-19 vaccine, and one of these patients was also found to not be able to mount any humoral response. Thus, for these immunosuppressed populations, alternative or complementary strategies would be lifesaving.
Evidence indicates that HIV PrEP is extremely effective (up to 98% effective) in reducing the risk of HIV acquisition when adherence to PrEP is optimal (16–20). PrEP has been broadly utilized to prevent HIV spread for populations who have a higher risk of acquiring HIV, such as sex workers, people who engage in recreational injection drug use, and those who practice unprotected receptive anal intercourse. Two combinations of oral antiretroviral drugs have been approved and used for HIV PrEP, including the combination of tenofovir disoproxil and emtricitabine (Truvada®), and tenofovir alafenamide and emtricitabine (Descovy®). Additionally, in December 2021, the US Food and Drug Administration (FDA) approved the first injectable preparation (cabotegravir extended-release injectable suspension) for HIV PrEP, which is believed to greatly improve medication compliance as it is administered only every 2 months.
Concerns regarding long-term drug safety, cost, development of drug resistance, and risk compensation of HIV PrEP present ongoing challenges. Some adverse effects have been observed; however, cumulative evidence has revealed that HIV PrEP has an overall satisfactory safety profile (72). The main adverse effects are usually mild to moderate nausea, vomiting, and diarrhea. Kidney and liver toxicity are rare; however, regular monitoring of renal and liver functions are required (73). Other concerns exist, including cost, development of drug resistance, and risk compensation. One meta-analysis that included 18 studies and 19,491 participants demonstrated that PrEP was highly effective across populations, presented few adverse events and instances of drug resistance, and had no significant association with changes in sexual risk behavior (21). Overall, current evidence indicates that the benefit-risk profiles of available HIV PrEP regimes are strongly favorable for the targeted population at high risk of infection by HIV.
Drawing on knowledge gained from the use of PrEP for HIV disease, SARS-CoV-2 PrEP, using specific antiviral and adjuvant drugs against SARS-CoV-2, may represent a novel preventive strategy for COVID-19. However, unlike HIV, against which there is currently no available vaccine, SARS-CoV-2 PrEP probably will be given to individuals who have poor response to vaccination and have a high-risk of developing severe COVID-19. Moreover, SARS-CoV-2 PrEP differs from post-infection treatment, which relies on therapeutic interventions to be initiated after the patient has tested positive for COVID-19, as shown in Figure 1. We thus consider a few drugs (favoring oral administration as first choice) that could potentially be used as PrEP for SARS-CoV-2. The mechanisms of action of the drugs discussed are summarized in Figure 2.
Figure 1. Comparison of SARS-CoV-2 PrEP strategies and post infection therapy. With an effective PrEP strategy, the patient remains negative for COVID-19 as SARS-CoV-2 cannot replicate in the patient's body. Conversely, without a PrEP strategy, any exposure to SARS-CoV-2 can result in an infection, confirmed by a positive PCR test. In the optimal scenario, the patient will develop a mild infection which will be subsequently controlled and eliminated by their immune response. ICU, intensive care unit.
Figure 2. The mechanisms of action of specific drugs that could potentially serve as PrEP for SARS-CoV-2.
Molnupiravir (MK-4482) is an orally available prodrug of beta-D-N4-hydroxycytidine (NHC), a ribonucleoside that has broad antiviral activity against RNA viruses (74). Viral mutations and lethal mutagenesis results from NHC uptake by viral RNA-dependent RNA-polymerases. Molnupiravir has been found to be effective against SARS-CoV-2, as demonstrated by Zhou et al. (75) and Kabinger et al. (76). In a randomized, placebo-controlled, double-blind phase 2/3 trial, Arribas et al. observed that molnupiravir administration does not result in clinical benefit in patients hospitalized by COVID-19 (77). However, results gleaned from studies of molnupiravir administration to non-hospitalized patients indicate a much more favorable picture. Caraco et al. (78) (Phase 2 MOVe-OUT study) observed a lower incidence of hospitalization and/or death in the molnupiravir group vs. the placebo group in specific participants (especially those >60 years of age, and those with an increased risk for severe illness). The preceding research group subsequently concluded that molnupiravir administration can reduce hospitalizations and/or death in non-hospitalized patients with COVID-19. Furthermore, results from a study by Jayk Bernal et al. (79) (Phase 3 MOVe-OUT study) indicate that (i) the rate of hospitalization or death through day 29 is ~31% lower with molnupiravir than with placebo, and (ii) molnupiravir treatment is associated with greater reductions in mean viral load from baseline than placebo at days 3, 5 (end-of-treatment visit), and 10. Armed with the promising results gleaned from this study conducted in non-hospitalized COVID-19 patients, the authors concluded that early treatment with molnupiravir reduced the risk of hospitalization or death in at-risk and unvaccinated adults with COVID-19. Thus, proposing the use of molnupiravir in a pre- or post-exposure prophylaxis context seems reasonable upon reflection. Although the results from the MOVe-OUT trial indicate that molnupiravir is safe for human use, a theoretical risk with the use of molnupiravir has been postulated, as molnupiravir could possibly be processed by human host cells and could, conceivably, be incorporated into the host DNA, potentially leading to cellular mutations and cellular death (75).
Remdesivir is an antiviral nucleoside analog pro-drug which inhibits the RNA-dependent RNA polymerase non-structural protein 12 (NSP12) in SARS-CoV-2. It was originally developed to treat Ebola virus disease (80), but has shown positive outcomes when used in SARS-CoV and MERS-CoV infections in vitro and in preclinical in vivo animal models (81–83), and was also used in the treatment of the first reported case of COVID-19 in the United States of America (with no obvious adverse effects) (84). Ebola virus, SARS-CoV, MERS-CoV, and SARS-CoV-2 genomes obviously differ; however, remdesivir has a broad-spectrum of activity, and therefore has the capacity to effectively neutralize RNA polymerase, the structure and function of which is relatively similar in all RNA viruses (85, 86). Indeed, remdesivir, after a sequence of steps that is presumably initiated by esterase-mediated hydrolysis of the amino acid ester, is ultimately metabolized into the active nucleoside triphosphate analog form, which is utilized by the viral RNA-dependent RNA polymerase (RdRp) upon its diffusion into the cell. Then, utilization of that nucleoside triphosphate analog by RdRp inhibits viral replication, as it induces delayed chain termination (87, 88). It has now been established that remdesivir has potent in vitro activity against SARS-CoV-2 (89), and has been used and studied in several recent randomized clinical trials (90–94). Although authors arrive at differing conclusions regarding its efficacy for the treatment of hospitalized COVID-19 patients, a clinical benefit is suspected, especially when used in the early phase of the disease. Moreover, based on the manufacturer statement regarding remdesivir efficacy in preventing SARS-CoV-2 infection, the PINETREE study (NCT 04501952) showed positive effects of remdesivir on the course of COVID-19 in outpatients who were treated early, and was also shown to be safe, and well-tolerated (95). In order to circumvent the significant limitation to the use of remdesivir imposed by the requirement of intravenous administration (which may potentially limit its widespread use during the pandemic), the orally bioavailable nucleoside prodrug GS-621763, which has been shown to be metabolized into the same active nucleoside triphosphate formed by remdesivir, has now been developed, and has shown potent antiviral activity against SARS-CoV-2 in various cell models, with a similar therapeutic efficacy to intravenous remdesivir in a murine model of SARS-CoV-2 pathogenesis (96). Overall, it therefore seems reasonable to actively consider the use of remdesivir or its oral prodrug as potentially useful antiviral drugs for SARS-CoV-2 pre-exposure prophylaxis.
Favipiravir is a purine nucleoside analog which acts as a competitive inhibitor of RNA-dependent RNA polymerase (97). In other words, favipiravir has been shown to be a potent inhibitor of various different viral RNA-dependent RNA polymerases (RdRps), including in influenza A and B viruses, in several agents causing viral hemorrhagic fever, and also in SARS-CoV-2 in vitro (82, 97, 98). In a clinical context, favipiravir administration to COVID-19 patients has been shown to be capable of (i) reducing the window for viral clearance (from 11 to 4 days) and (ii) improving pulmonary inflammatory marker levels (91% of treated patients showed improvement vs. 62% in the control group) (99). Udwadia et al. in their randomized, comparative, open-label, multicenter, phase 3 clinical trial, have demonstrated that favipiravir administration can significantly shorten the time to clinical cure in COVID-19 patients (100). Importantly, Doi et al. have reported that early intervention with favipiravir is superior to late intervention in terms of viral clearance and time to defervescence. Favipiravir would thus be of potential benefit if administered in a SARS-CoV-2 PrEP context. Researchers conducting an ongoing clinical study in Canada are currently assessing the efficacy of favipiravir treatment over 25 days for the prevention of SARS-CoV-2 infection in residents and staff of nursing homes (among elderly, assisted-living patients, and healthcare professionals) (NCT04448119, Phase 2). Results and outcomes of this study are eagerly awaited.
In vitro investigations suggest that tenofovir (i) inhibits SARS-CoV-2 RdRp, although with weaker binding than remdesivir (91, 92, 101, 102) and (ii) possesses immunomodulatory effects as it demonstrates the ability to decrease both interleukin (IL)-8 and IL-10 production (103), which are both known to favor COVID-19 severity (104, 105). In addition, observations made in people living with HIV (PLWH) indicate that there is a little evidence that HIV infection increases COVID-19 risk in settings with good access to tenofovir-based antiretroviral therapy (ART) (106). The preceding intriguing observation initiated considered ruminations with respect to the potential activity of tenofovir disoproxil fumarate (TDF, now used worldwide for HIV treatment and HIV pre-exposure prophylaxis) against SARS-CoV-2. Indeed, del Amo et al. (106), in a Spanish cohort study of 77 590 PLWH taking ART, reported that the incidence (per 10,000 persons) of COVID-19 diagnosis among patients taking TDF/FTC was 16.9 [95% confidence interval (CI), 10.5–25.9], compared to 41.7 in the general population. Furthermore, a study by Boulle et al. (107) found that PLWH taking TDF/FTC experience 59% lower mortality from COVID-19 than those taking abacavir or zidovudine (aHR, 0.41; 95% CI, 0.21–0.78). Similarly, a third cohort study by Ayerdi et al. (108) has demonstrated that HIV PrEP (tenofovir/emtricitabine) users who tested positive for SARS-CoV-2 antibodies showed higher rates of asymptomatic infection, although the difference in asymptomatic rates of SARS-CoV-2 infection was not statistically significant (42.7 vs. 21.7% for non-PrEP users; p = 0.07). In light of the tenofovir-based treatment safety profile and its putative anti-SARS-CoV-2 effects, the tenofovir/emtricitabine combination (the combination present in DESCOVY® and TRUVADA® for example), is currently being studied as a SARS-CoV-2 prophylactic agent. As such, we can report that (i) a clinical trial assessing the efficacy of a 12 week SARS-CoV-2 prophylaxis course of the emtricitabine/tenofovir regimen (NCT04334928) in healthcare workers in Spain is ongoing, and (ii) several additional proposed studies intend to use this specific drug combination in a preventive manner for COVID-19 (examples: NCT04519125 and NCT04405271).
Another orally administered potentially prophylactic drug is nirmatrelvir, a specific inhibitor of the SARS-CoV-2 viral 3-chymotrypsin-like cysteine (3CL) protease (109, 110). To achieve adequate drug levels, nirmatrelvir is administered together with the CYP 3A4 inhibitor, ritonavir. The role of ritonavir, well-known as a pharmacological booster, is hypothetical in Figure 2, as it is based on theoretical evidence from several researchers (111–114) showing that lopinavir and ritonavir also inhibit the coronaviral 3CL1pro protease, although coronaviruses encode a different enzymatic class of protease. Knowing that 3CL1pro protease plays an essential role in processing the polyproteins that are translated from the viral RNA, we therefore are encouraged that ritonavir could possibly also inhibit the formation of mature virions of SARS-CoV-2. In the E,PIC-HR study (NCT04960202), 5 days of therapy with nirmatrelvir/ritonavir reduced the rate of hospitalization and/or death by 88% in COVID-19 outpatients with at least one risk factor for a severe course if therapy was started early (within 5 days) after the onset of symptoms. On the 22nd of December 2021, the US FDA endorsed and authorized nirmatrelvir/ritonavir (Paxlovid®) use for the treatment of COVID-19 (115). However, due to the required combination with ritonavir, drug interactions may occur, especially in high risk populations (116). Further study of this drug combination may provide a clearer picture of its benefits when administered for SARS-CoV-2 pre- or post-exposure prophylaxis.
Azvudine is a safe (117) nucleoside-based broad-spectrum anti-virus clinical candidate originally developed for HIV infection treatment and prevention (118, 119). As such, azvudine was approved by China FDA for AIDS treatment on July 21, 2021 (XZXK-2021-214) in view of its efficacy in treating AIDS and its favorable safety profile during the 48-week oral treatment (120). In vitro, azvudine has shown significant antiviral effects against HIV (121), HCV (122), human enterovirus 71 (123), and HBV (124). Furthermore, Ren and colleagues were the first to observe a potent antiviral activity against HCoV-OC43 and SARS-CoV-2, fostering speculation with respect to its anti-COVID-19 effect. Indeed, azvudine is known to inhibit viral RNA-dependent RNA polymerase (123, 125), and in a subsequent randomized, open-label, controlled clinical trial, Ren et al. have reported in 2020 that azvudine treatment may shorten the nucleic acid negative conversion time in the mild COVID-19 context (126). They therefore requested permission for investigation using a larger sample size, to confirm their findings. Recently (in December 2021), Zhang et al. (120) have demonstrated that oral administration of azvudine was able to (i) reduce the viral load in SARS-CoV-2 infected rhesus macaques and (ii) cure all COVID-19 patients in their treatment cohort (a randomized, single-arm clinical trial; n = 31). They observed that all study participants demonstrated 100% viral ribonucleic acid negative conversion in 3.29 days, with a 100% hospital discharge rate in 9 days, although minor and transient side-effects (dizziness and nausea) were noted in 16.12% (5/31) of patients. It is thus valid to state that the preceding findings favor the potential utilization of azvudine in future SARS-CoV-2 pre-exposure prophylaxis strategies.
Formulating, investigating, and proposing preventive strategies for COVID-19, such as SARS-CoV-2 PrEP, are likely to help prevent morbidity and mortality from COVID-19 in high-risk populations. The antiviral drugs and their combinations listed in the discussion should be considered to be theoretically and hypothetically proposed strategies for SARS-CoV-2 PrEP prevention. Even though some of the proposed therapeutic methods appear to be promising, multiple challenges remain for the future development of effective SARS-CoV-2 PrEP.
Firstly, drug adverse effects or toxicity are a primary concern. For example, there are specific host DNA mutational concerns with molnupiravir use which need to be addressed (75). Remdesivir, as a lyophilized powder or injectable solution, has been associated with renal and hepatic toxicity as a consequence of the accumulation of excipient sulfobutylether-β-cyclodextrin (SBECD) (127, 128). Moreover, most drugs listed have revealed their benefits in already-infected patients, while their efficacy and safety in preventing SARS-CoV-2 infection in uninfected and/or vaccinated individuals will warrant further studies.
Poor adherence to antiretroviral therapy against HIV has been shown to be a major determinant for the emergence of drug resistance (129). There would also be concerns regarding drug resistance development for SARS-CoV-2 PrEP. Monotherapy may well avoid drug-drug interactions; however, compared with combination therapy, monotherapy is more likely to result in the emergence of drug resistance (130, 131). Immunocompromised patients are more likely to develop high intra-host viral diversity (132–134), which further emphasizes their risk of developing drug resistance following monotherapy. Thus, further investigations should evaluate the possibility of co-administration of two or more drugs to potentially reduce the possibility of development of resistance. Thus, we believe that the US FDA-authorized nirmatrelvir/ritonavir (Paxlovid®) is one drug combination that can possibly be contemplated as an effective PrEP candidate.
A SARS-CoV-2 PrEP strategy may help prevent morbidity and mortality from COVID-19; however, it may also encourage the easing of the very effective preventive measures that attempt to decrease the spread of the virus, such as social distancing interventions and avoidance of exposure, thus potentially increasing infection risk. During the COVID-19 pandemic, risk compensation has been associated with vaccination and face mask use (135, 136). Risk compensation may also significantly impact the benefits of a SARS-CoV-2 PrEP strategy, especially if efficacy of SARS-CoV-2 PrEP in real-life is not sufficiently high.
Moreover, prior to the implementation of a SARS-CoV-2 PrEP strategy, the specific criteria for the likelihood of acquisition of infection after exposure to SARS-CoV-2 should also be studied and clearly defined, including the required exposure time for infection to occur (since the probability of being infected would increase when the exposure time exceeds specific time thresholds), the occurrence of new epidemic cases in the family or at the workplace, the physical distance from the potential infective spreader, and the duration of infection of the potential infective spreader (suspected infection or documented infection by PCR or rapid testing). Similarly, the follow-up of users of PrEP and the criteria evaluating the outcome of PrEP for COVID-19 remain to be clarified. A polymerase chain reaction (PCR) test performed 5 days after PrEP medication in parallel with a blood test evaluating toxicity of the drug(s) is recommended.
Furthermore, the PrEP concept excludes parenteral therapy, and should be available for high-risk patients at home, preferably before potential exposure. The dosage and the duration of treatment will depend on each drug used. Cost efficacy of PrEP should be considered with particular diligence and gravity, as such a preventive COVID-19 strategy, if effective, may circumvent ICU admission (where costs are known to be prohibitive), and extended hospital stays.
The PrEP for COVID-19 proposed in the preceding discussion involves the administration of the drugs listed above to only high-risk populations, particularly patients with an immunocompromised status, such as common variable immunodeficiency, lymphopenia, patients with organ transplants, and lymphoma. Additionally, the potential SARS-CoV-2 PrEP candidate drugs described herein have specific merit for use in patients who respond poorly to COVID-19 vaccination and who are more likely to develop severe COVID-19. Nevertheless, the entire SARS-CoV-2 PrEP remains a theoretical construct, and significant merits of and limitations to our proposed SARS-CoV-2 PrEP strategies exist (Figure 3). However, for selected categories of patients, PrEP for COVID-19 can likely represent a potentially viable course of action that should be carefully and impartially examined and studied.
Based on the perceived risk benefit ratio, we consider that potential SARS-CoV-2 PrEP strategies should be evaluated in the context of future SARS-CoV-2 infection waves in large populations.
Individual population health policies adopted by countries around the world have the potential to significantly challenge the PrEP strategy proposed in this article. For example, free rapid antigen testing kits are now widely available to individuals in countries such as Canada. This diagnostic test is not based the presence of SARS-CoV-2 in the test sample, but is based on specific parts of the SARS-CoV-2 virion (such as the nucleocapsid), and can thus potentially result in false positive results. The PrEP strategy that we propose is evaluated through PCR testing, which requires the presence of the virus in the test sample, and is therefore more accurate diagnostically. In Canada, based on a positive test with rapid antigen kits, pharmacists can initiate Paxlovid on the day of diagnosis, even without the approval of a physician. It is, thus, particularly difficult to initiate, follow-up, and/or evaluate the efficacy of PrEP in such a context. Another example is China with its dynamic zero COVID-19 policy. Indeed, this policy is ambitious; however, it does not favor implementation of strategies such as our proposed SARS-CoV-2 PrEP strategy as currently, (i) known cases are closely monitored, following stringent protocols, (ii) quarantine measures are largely implemented, (iii) and PCR tests are considered the gold standard. Perhaps, a PrEP strategy could be implemented if and when the prevailing COVID-19 situation in China becomes totally under control; however, the evaluation of its efficacy will remain difficult, as other associated measures aiming to reduce the exposure to SARS-CoV-2 (facemask use, decontamination measures) are ubiquitously and universally maintained.
HIV PrEP has been demonstrated to be an effective infection prevention strategy, with a significantly favorable benefit-risk profile for the prevention of HIV transmission to people at high risk. Based on this model, we propose the development of SARS-CoV-2 PrEP for use in high-risk populations, including healthcare workers who can induce secondary transmission, immunodeficient individuals, and poor vaccine responders. Much progress has been made in discerning the risk factors for acquiring COVID-19, which include close contact, demographic factors, presence of certain comorbidities, environmental factors, and vaccine response. Emergent drugs with beneficial effects are paving the way for development of possible PrEP strategies which could be utilized to prevent COVID-19 infection in high-risk populations. However, several challenges exist for the development of strategies for SARS-CoV-2 PrEP, such as drug toxicity and patient safety concerns, emergence of drug resistance, and the cost of drugs. We believe that collaborative efforts at conducting comprehensive assessments for ethical considerations related to SARS-CoV-2 PrEP, the benefit-risk profiles of SARS-CoV-2 PrEP, and strategic planning of implementation of SARS-CoV-2 PrEP in selected populations should be a research priority. Based on current evidence, we consider that PrEP for COVID-19 could be a potentially useful and practical adjunct to COVID-19 vaccination to prevent SARS-CoV-2 acquisition in selected at-risk patients.
JO and SZ wrote the first draft edition of the manuscript. VH and XL provided critical revisions of this manuscript. J-PR and YC conceived and designed the manuscript. All authors listed have made a substantial, direct, and intellectual contribution to the work and approved it for publication.
This work was supported by the Joint Medical Research Project (2020GDRC010) of Chongqing Science and Technology Bureau and Chongqing Health Commission and in part by réseau SIDA and maladies infectieuses du fonds de la recherche Québec en Santé (FRQ-S). J-PR is the holder of the Louis Lowenstein Chair in Hematology and Oncology, McGill University.
The authors declare that the research was conducted in the absence of any commercial or financial relationships that could be construed as a potential conflict of interest.
All claims expressed in this article are solely those of the authors and do not necessarily represent those of their affiliated organizations, or those of the publisher, the editors and the reviewers. Any product that may be evaluated in this article, or claim that may be made by its manufacturer, is not guaranteed or endorsed by the publisher.
1. Worldometer. COVID-19 Coronavirus Pandemic. (2020). Available online at: https://www.worldometers.info/coronavirus/ (accessed March 3, 2022).
2. Keeton R, Tincho MB, Ngomti A, Baguma R, Benede N, Suzuki A, et al. T cell responses to SARS-CoV-2 spike cross-recognize Omicron. Nature. (2022) 603:488–92. doi: 10.1038/s41586-022-04460-3
3. Mistry P, Barmania F, Mellet J, Peta K, Strydom A, Viljoen IM, et al. SARS-CoV-2 variants, vaccines, and host immunity. Front Immunol. (2021) 12:809244. doi: 10.3389/fimmu.2021.809244
4. Tabilo Valenzuela PB, Flores Balter G, Saint-Pierre Contreras G, Conei Valencia D, Moreno Calderón C, Bohle Venegas C, et al. Cellular immune response in patients immunized with three vaccine doses of different vaccination schemes authorized by the chilean ministry of health in January 2022. Life. (2022) 12:534. doi: 10.3390/life12040534
5. Doria-Rose N, Suthar MS, Makowski M, O'Connell S, McDermott AB, Flach B, et al. Antibody persistence through 6 months after the second dose of mRNA-1273 vaccine for Covid-19. N Engl J Med. (2021) 384:2259–61. doi: 10.1056/NEJMc2103916
6. Favresse J, Bayart JL, Mullier F, Elsen M, Eucher C, Van Eeckhoudt S, et al. Antibody titres decline 3-month post-vaccination with BNT162b2. Emerg Microbes Infect. (2021) 10:1495–8. doi: 10.1080/22221751.2021.1953403
7. Pegu A, O'Connell SE, Schmidt SD, O'Dell S, Talana CA, Lai L, et al. Durability of mRNA-1273 vaccine-induced antibodies against SARS-CoV-2 variants. Science. (2021) 373:1372–7. doi: 10.1126/science.abj4176
8. Naaber P, Tserel L, Kangro K, Sepp E, Jürjenson V, Adamson A, et al. Dynamics of antibody response to BNT162b2 vaccine after six months: a longitudinal prospective study. Lancet Regional Health Europe. (2021) 10:100208. doi: 10.1016/j.lanepe.2021.100208
9. Khoury J, Najjar-Debbiny R, Hanna A, Jabbour A, Abu Ahmad Y, Saffuri A, et al. COVID-19 vaccine - Long term immune decline and breakthrough infections. Vaccine. (2021) 39:6984–9. doi: 10.1016/j.vaccine.2021.10.038
10. Gao SJ, Guo H, Luo G. Omicron variant (B.1.1.529) of SARS-CoV-2, a global urgent public health alert! J Med Virol. (2022) 94:1255–6. doi: 10.1002/jmv.27491
11. Chen J, Wang R, Gilby NB, Wei GW. Omicron variant (B.1.1.529): infectivity, vaccine breakthrough, and antibody resistance. J Chem Inform Model. (2022) 62:412–22. doi: 10.1021/acs.jcim.1c01451
12. Pulliam JRC, van Schalkwyk C, Govender N, von Gottberg A, Cohen C, Groome MJ, et al. Increased risk of SARS-CoV-2 reinfection associated with emergence of Omicron in South Africa. Science. (2022) 376:eabn4947. doi: 10.1126/science.abn4947
13. Hoffmann M, Krüger N, Schulz S, Cossmann A, Rocha C, Kempf A, et al. The Omicron variant is highly resistant against antibody-mediated neutralization: implications for control of the COVID-19 pandemic. Cell. (2022) 185:447–56.e11. doi: 10.1016/j.cell.2021.12.032
14. Tomalka JA, Suthar MS, Deeks SG, Sekaly RP. Fighting the SARS-CoV-2 pandemic requires a global approach to understanding the heterogeneity of vaccine responses. Nat Immunol. (2022) 23:360–70. doi: 10.1038/s41590-022-01130-4
15. Yeoh YK, Zuo T, Lui GC, Zhang F, Liu Q, Li AY, et al. Gut microbiota composition reflects disease severity and dysfunctional immune responses in patients with COVID-19. Gut. (2021) 70:698–706. doi: 10.1136/gutjnl-2020-323020
16. Grant RM, Anderson PL, McMahan V, Liu A, Amico KR, Mehrotra M, et al. Uptake of pre-exposure prophylaxis, sexual practices, and HIV incidence in men and transgender women who have sex with men: a cohort study. Lancet Infect Dis. (2014) 14:820–9. doi: 10.1016/S1473-3099(14)70847-3
17. Liu AY, Cohen SE, Vittinghoff E, Anderson PL, Doblecki-Lewis S, Bacon O, et al. Preexposure prophylaxis for HIV infection integrated with municipal- and community-based sexual health services. JAMA Intern Med. (2016) 176:75–84. doi: 10.1001/jamainternmed.2015.4683
18. McCormack S, Dunn DT, Desai M, Dolling DI, Gafos M, Gilson R, et al. Pre-exposure prophylaxis to prevent the acquisition of HIV-1 infection (PROUD): effectiveness results from the pilot phase of a pragmatic open-label randomised trial. Lancet. (2016) 387:53–60. doi: 10.1016/S0140-6736(15)00056-2
19. Marcus JL, Hurley LB, Nguyen DP, Silverberg MJ, Volk JE. Redefining human immunodeficiency virus (HIV) preexposure prophylaxis failures. Clin Infect Dis. (2017) 65:1768–9. doi: 10.1093/cid/cix593
20. Volk JE, Marcus JL, Phengrasamy T, Blechinger D, Nguyen DP, Follansbee S, et al. No new HIV infections with increasing use of hiv preexposure prophylaxis in a clinical practice setting. Clin Infect Dis. (2015) 61:1601–3. doi: 10.1093/cid/civ778
21. Fonner VA, Dalglish SL, Kennedy CE, Baggaley R, O'Reilly KR, Koechlin FM, et al. Effectiveness and safety of oral HIV preexposure prophylaxis for all populations. AIDS. (2016) 30:1973–83. doi: 10.1097/QAD.0000000000001145
22. WHO. WHO Implementation Tool for Pre-exposure Prophylaxis (PrEP) of HIV Infection. Module 5: Monitoring and Evaluation. Geneva: WHO (2018).
23. Zhan M, Qin Y, Xue X, Zhu S. Death from Covid-19 of 23 health care workers in China. N Engl J Med. (2020) 382:2267–8. doi: 10.1056/NEJMc2005696
24. Kluytmans-van den Bergh MFQ, Buiting AGM, Pas SD, Bentvelsen RG, van den Bijllaardt W, van Oudheusden AJG, et al. Prevalence and clinical presentation of health care workers with symptoms of coronavirus disease 2019 in 2 Dutch hospitals during an early phase of the pandemic. JAMA Netw Open. (2020) 3:e209673. doi: 10.1001/jamanetworkopen.2020.9673
25. Nguyen LH, Drew DA, Graham MS, Joshi AD, Guo CG, Ma W, et al. Risk of COVID-19 among front-line health-care workers and the general community: a prospective cohort study. Lancet Public health. (2020) 5:e475–e83. doi: 10.1016/S2468-2667(20)30164-X
26. Health and Care Worker Deaths During COVID-19. World Health Organization (2021). Available online at: https://www.who.int/news/item/20-10-2021-health-and-care-worker-deaths-during-covid-19 (accessed March 3, 2022).
27. Up to 180 000 Health Workers May Have Died From COVID-19. (2021). Available online at: https://news.un.org/en/story/2021/10/1103642 (accessed March 3, 2022).
28. Bandyopadhyay S, Baticulon RE, Kadhum M, Alser M, Ojuka DK, Badereddin Y, et al. Infection and mortality of healthcare workers worldwide from COVID-19: a systematic review. BMJ Global Health. (2020) 5:e003097. doi: 10.1136/bmjgh-2020-003097
29. Severe outcomes among patients with coronavirus disease 2019 (COVID-19) - United States February 12-March 16 2020. MMWR Morb Mortal Wkly Rep. (2020) 69:343–6. doi: 10.15585/mmwr.mm6912e2
30. Pijls BG, Jolani S, Atherley A, Derckx RT, Dijkstra JIR, Franssen GHL, et al. Demographic risk factors for COVID-19 infection, severity, ICU admission and death: a meta-analysis of 59 studies. BMJ Open. (2021) 11:e044640. doi: 10.1136/bmjopen-2020-044640
31. Parohan M, Yaghoubi S, Seraji A, Javanbakht MH, Sarraf P, Djalali M. Risk factors for mortality in patients with Coronavirus disease 2019 (COVID-19) infection: a systematic review and meta-analysis of observational studies. Aging Male. (2020) 23:1416–24. doi: 10.1080/13685538.2020.1774748
32. Gao YD, Ding M, Dong X, Zhang JJ, Kursat Azkur A, Azkur D, et al. Risk factors for severe and critically ill COVID-19 patients: a review. Allergy. (2021) 76:428–55. doi: 10.1111/all.14657
33. Papadopoulos V, Li L, Samplaski M. Why does COVID-19 kill more elderly men than women? Is there a role for testosterone? Andrology. (2021) 9:65–72. doi: 10.1111/andr.12868
34. Sze S, Pan D, Nevill CR, Gray LJ, Martin CA, Nazareth J, et al. Ethnicity and clinical outcomes in COVID-19: a systematic review and meta-analysis. EClinicalMedicine. (2020) 29:100630. doi: 10.1016/j.eclinm.2020.100630
35. Tian T, Zhang J, Hu L, Jiang Y, Duan C, Li Z, et al. Risk factors associated with mortality of COVID-19 in 3125 counties of the United States. Infect Dis Poverty. (2021) 10:3. doi: 10.1186/s40249-020-00786-0
36. Williamson EJ, Walker AJ, Bhaskaran K, Bacon S, Bates C, Morton CE, et al. Factors associated with COVID-19-related death using OpenSAFELY. Nature. (2020) 584:430–6. doi: 10.1038/s41586-020-2521-4
37. Raharja A, Tamara A, Kok LT. Association between ethnicity and severe COVID-19 disease: a systematic review and meta-analysis. J Racial Ethnic Health Disparities. (2021) 8:1563–72. doi: 10.1007/s40615-020-00921-5
38. Muñiz-Diaz E, Llopis J, Parra R, Roig I, Ferrer G, Grifols J, et al. Relationship between the ABO blood group and COVID-19 susceptibility, severity and mortality in two cohorts of patients. Blood Transfusion. (2021) 19:54–63. doi: 10.2450/2020.0256-20
39. Zhao J, Yang Y, Huang H, Li D, Gu D, Lu X, et al. Relationship between the ABO blood group and the coronavirus disease 2019 (COVID-19) susceptibility. Clin Infect Dis. (2021) 73:328–31. doi: 10.1093/cid/ciaa1150
40. Zietz M, Zucker J, Tatonetti NP. Associations between blood type and COVID-19 infection, intubation, and death. Nat Commun. (2020) 11:5761. doi: 10.1038/s41467-020-19623-x
41. Liu N, Zhang T, Ma L, Zhang H, Wang H, Wei W, et al. The impact of ABO blood group on COVID-19 infection risk and mortality: a systematic review and meta-analysis. Blood Rev. (2021) 48:100785. doi: 10.1016/j.blre.2020.100785
42. Li J, Wang X, Chen J, Cai Y, Deng A, Yang M. Association between ABO blood groups and risk of SARS-CoV-2 pneumonia. Br J Haematol. (2020) 190:24–7. doi: 10.1111/bjh.16797
43. Wu Y, Feng Z, Li P, Yu Q. Relationship between ABO blood group distribution and clinical characteristics in patients with COVID-19. Clin Chim Acta. (2020) 509:220–3. doi: 10.1016/j.cca.2020.06.026
44. Zhou F, Yu T, Du R, Fan G, Liu Y, Liu Z, et al. Clinical course and risk factors for mortality of adult inpatients with COVID-19 in Wuhan, China: a retrospective cohort study. Lancet. (2020) 395:1054–62. doi: 10.1016/S0140-6736(20)30566-3
45. Huang C, Wang Y, Li X, Ren L, Zhao J, Hu Y, et al. Clinical features of patients infected with 2019 novel coronavirus in Wuhan, China. Lancet. (2020) 395:497–506. doi: 10.1016/S0140-6736(20)30183-5
46. Cecconi M, Piovani D, Brunetta E, Aghemo A, Greco M, Ciccarelli M, et al. Early predictors of clinical deterioration in a cohort of 239 patients hospitalized for Covid-19 infection in Lombardy, Italy. J Clin Med. (2020) 9:1548. doi: 10.3390/jcm9051548
47. Chen T, Wu D, Chen H, Yan W, Yang D, Chen G, et al. Clinical characteristics of 113 deceased patients with coronavirus disease 2019: retrospective study. BMJ. (2020) 368:m1091. doi: 10.1136/bmj.m1091
48. Huang S, Wang J, Liu F, Liu J, Cao G, Yang C, et al. COVID-19 patients with hypertension have more severe disease: a multicenter retrospective observational study. Hypertens Res. (2020) 43:824–31. doi: 10.1038/s41440-020-0485-2
49. Guo W, Li M, Dong Y, Zhou H, Zhang Z, Tian C, et al. Diabetes is a risk factor for the progression and prognosis of COVID-19. Diabetes Metab Res Rev. (2020) 36:e3319. doi: 10.1002/dmrr.3319
50. Hernández-Garduño E. Obesity is the comorbidity more strongly associated for Covid-19 in Mexico. A case-control study. Obesity Res Clin Pract. (2020) 14:375–9. doi: 10.1016/j.orcp.2020.06.001
51. ACC. COVID-19 Clinical Guidance For the Cardiovascular Care Team. (2020). Available online at: https://www.acc.org/~/media/665AFA1E710B4B3293138D14BE8D1213.pdf (accessed July 26, 2022).
52. CDC. COVID-19 Information for Specific Groups of People. Centers for Disease Control and Prevention (2021). Available online at: https://www.cdc.gov/coronavirus/2019-ncov/need-extra-precautions/people-with-medical-conditions.html (accessed January 30, 2021).
53. Thakur B, Dubey P, Benitez J, Torres JP, Reddy S, Shokar N, et al. A systematic review and meta-analysis of geographic differences in comorbidities and associated severity and mortality among individuals with COVID-19. Sci Rep. (2021) 11:8562. doi: 10.1038/s41598-021-88130-w
54. Bhattacharyya A, Seth A, Srivast N, Imeokparia M, Rai S. Coronavirus (COVID-19): a systematic review and meta-analysis to evaluate the significance of demographics and comorbidities. Res Sq. (2021). doi: 10.21203/rs.3.rs-144684/v1
55. Gold MS, Sehayek D, Gabrielli S, Zhang X, McCusker C, Ben-Shoshan M. COVID-19 and comorbidities: a systematic review and meta-analysis. Postgrad Med. (2020) 132:749–55. doi: 10.1080/00325481.2020.1786964
56. Singh AK, Gillies CL, Singh R, Singh A, Chudasama Y, Coles B, et al. Prevalence of co-morbidities and their association with mortality in patients with COVID-19: a systematic review and meta-analysis. Diabetes Obes Metab. (2020) 22:1915–24. doi: 10.1111/dom.14124
57. Nandy K, Salunke A, Pathak SK, Pandey A, Doctor C, Puj K, et al. Coronavirus disease (COVID-19): A systematic review and meta-analysis to evaluate the impact of various comorbidities on serious events. Diabetes Metab Synd. (2020) 14:1017–25. doi: 10.1016/j.dsx.2020.06.064
58. Ma Y, Zhao Y, Liu J, He X, Wang B, Fu S, et al. Effects of temperature variation and humidity on the death of COVID-19 in Wuhan, China. Sci Total Environ. (2020) 724:138226. doi: 10.1016/j.scitotenv.2020.138226
59. Pirouz B, Shaffiee Haghshenas S, Pirouz B, Shaffiee Haghshenas S, Piro P. Development of an assessment method for investigating the impact of climate and urban parameters in confirmed cases of COVID-19: a new challenge in sustainable development. Int J Environ Res Public Health. (2020) 17:2801. doi: 10.3390/ijerph17082801
60. Prata DN, Rodrigues W, Bermejo PH. Temperature significantly changes COVID-19 transmission in (sub)tropical cities of Brazil. Sci Total Environ. (2020) 729:138862. doi: 10.1016/j.scitotenv.2020.138862
61. Sahin M. Impact of weather on COVID-19 pandemic in Turkey. Sci Total Environ. (2020) 728:138810. doi: 10.1016/j.scitotenv.2020.138810
62. Sobral MFF, Duarte GB, da Penha Sobral AIG, Marinho MLM, de Souza Melo A. Association between climate variables and global transmission oF SARS-CoV-2. Sci Total Environ. (2020) 729:138997. doi: 10.1016/j.scitotenv.2020.138997
63. Zhu Y, Xie J, Huang F, Cao L. Association between short-term exposure to air pollution and COVID-19 infection: evidence from China. Sci Total Environ. (2020) 727:138704. doi: 10.1016/j.scitotenv.2020.138704
64. Shakil MH, Munim ZH, Tasnia M, Sarowar S. COVID-19 and the environment: a critical review and research agenda. Sci Total Environ. (2020) 745:141022. doi: 10.1016/j.scitotenv.2020.141022
65. Yin H, Sun T, Yao L, Jiao Y, Ma L, Lin L, et al. Association between population density and infection rate suggests the importance of social distancing and travel restriction in reducing the COVID-19 pandemic. Environ Sci Pollut Res Int. (2021) 28:40424–30. doi: 10.1007/s11356-021-12364-4
66. Walsh EE, Frenck RW Jr., Falsey AR, Kitchin N, Absalon J, et al. Safety and immunogenicity of two RNA-based Covid-19 vaccine candidates. N Engl J Med. (2020) 383:2439–50. doi: 10.1056/NEJMoa2027906
67. Fahrner JE, Lahmar I, Goubet AG, Haddad Y, Carrier A, Mazzenga M, et al. The polarity and specificity of antiviral T lymphocyte responses determine susceptibility to SARS-CoV-2 infection in cancer patients and healthy individuals. Cancer Discov. (2022) 12:958–83. doi: 10.1158/2159-8290.CD-21-1441
68. Ho HE, Mathew S, Peluso MJ, Cunningham-Rundles C. Clinical outcomes and features of COVID-19 in patients with primary immunodeficiencies in New York City. J Allergy Clin Immunol Pract. (2021) 9:490–3.e2. doi: 10.1016/j.jaip.2020.09.052
69. Amodio D, Ruggiero A, Sgrulletti M, Pighi C, Cotugno N, Medri C, et al. Humoral and cellular response following vaccination with the BNT162b2 mRNA COVID-19 vaccine in patients affected by primary immunodeficiencies. Front Immunol. (2021) 12:727850. doi: 10.3389/fimmu.2021.727850
70. Goubet AG, Dubuisson A, Geraud A, Danlos FX, Terrisse S, Silva CAC, et al. Prolonged SARS-CoV-2 RNA virus shedding and lymphopenia are hallmarks of COVID-19 in cancer patients with poor prognosis. Cell Death Differ. (2021) 28:3297–315. doi: 10.1038/s41418-021-00817-9
71. Fernandez Salinas A, Piano Mortari E, Terreri S, Milito C, Zaffina S, Perno CF, et al. Impaired memory B-cell response to the Pfizer-BioNTech COVID-19 vaccine in patients with common variable immunodeficiency. J Allergy Clin Immunol. (2022) 149:76–7. doi: 10.1016/j.jaci.2021.08.031
72. Tetteh RA, Yankey BA, Nartey ET, Lartey M, Leufkens HG, Dodoo AN. Pre-exposure prophylaxis for HIV prevention: safety concerns. Drug Safety. (2017) 40:273–83. doi: 10.1007/s40264-017-0505-6
73. Bloch M. Prescribing pre-exposure prophylaxis for HIV. Aust Prescriber. (2020) 43:200–3. doi: 10.18773/austprescr.2020.057
74. NIH. The COVID-19 Treatment Guidelines Panel's Statement on Therapies for High-Risk, Nonhospitalized Patients With Mild to Moderate COVID-19. (2022). Available online at: https://www.covid19treatmentguidelines.nih.gov/therapies/statement-on-therapies-for-high-risk-nonhospitalized-patients/ (accessed March 3, 2022).
75. Zhou S, Hill CS, Sarkar S, Tse LV, Woodburn BMD, Schinazi RF, et al. β-d-N4-hydroxycytidine inhibits SARS-CoV-2 through lethal mutagenesis but is also mutagenic to mammalian cells. J Infect Dis. (2021) 224:415–9. doi: 10.1093/infdis/jiab247
76. Kabinger F, Stiller C, Schmitzová J, Dienemann C, Kokic G, Hillen HS, et al. Mechanism of molnupiravir-induced SARS-CoV-2 mutagenesis. Nat Struct Mol Biol. (2021) 28:740–6. doi: 10.1038/s41594-021-00651-0
77. Arribas JR, Bhagani S, Lobo SM, Khaertynova I, Mateu L, Fishchuk R, et al. Randomized trial of molnupiravir or placebo in patients hospitalized with Covid-19. NEJM Evid. (2022) 1:EVIDoa2100044. doi: 10.1056/EVIDoa2100044
78. Caraco Y, Crofoot GE, Moncada PA, Galustyan AN, Musungaie DB, Payne B, et al. Phase 2/3 trial of molnupiravir for treatment of Covid-19 in nonhospitalized adults. NEJM Evid. (2022) 1:EVIDoa2100043. doi: 10.1056/EVIDoa2100043
79. Jayk Bernal A, Gomes da Silva MM, Musungaie DB, Kovalchuk E, Gonzalez A, Delos Reyes V, et al. Molnupiravir for oral treatment of Covid-19 in nonhospitalized patients. New Engl J Med. (2021) 386:509–20. doi: 10.1056/NEJMoa2116044
80. Mulangu S, Dodd LE, Davey RT Jr., Tshiani Mbaya O, Proschan M, et al. A randomized, controlled trial of ebola virus disease therapeutics. New Engl J Med. (2019) 381:2293–303. doi: 10.1056/NEJMoa1910993
81. de Wit E, Feldmann F, Cronin J, Jordan R, Okumura A, Thomas T, et al. Prophylactic and therapeutic remdesivir (GS-5734) treatment in the rhesus macaque model of MERS-CoV infection. Proc Natl Acad Sci USA. (2020) 117:6771–6. doi: 10.1073/pnas.1922083117
82. Wang M, Cao R, Zhang L, Yang X, Liu J, Xu M, et al. Remdesivir and chloroquine effectively inhibit the recently emerged novel coronavirus (2019-nCoV) in vitro. Cell Res. (2020) 30:269–71. doi: 10.1038/s41422-020-0282-0
83. Sheahan TP, Sims AC, Leist SR, Schäfer A, Won J, Brown AJ, et al. Comparative therapeutic efficacy of remdesivir and combination lopinavir, ritonavir, and interferon beta against MERS-CoV. Nat Commun. (2020) 11:222. doi: 10.1038/s41467-019-13940-6
84. Holshue ML, DeBolt C, Lindquist S, Lofy KH, Wiesman J, Bruce H, et al. First Case of 2019 novel coronavirus in the United States. New Engl J Med. (2020) 382:929–36. doi: 10.1056/NEJMoa2001191
85. Tchesnokov EP, Feng JY, Porter DP, Götte M. Mechanism of inhibition of ebola virus RNA-dependent RNA polymerase by remdesivir. Viruses. (2019) 11:326. doi: 10.3390/v11040326
86. De Clercq E. New nucleoside analogues for the treatment of hemorrhagic fever virus infections. Chem Asian J. (2019) 14:3962–8. doi: 10.1002/asia.201900841
87. Eastman RT, Roth JS, Brimacombe KR, Simeonov A, Shen M, Patnaik S, et al. Remdesivir: a review of its discovery and development leading to emergency use authorization for treatment of COVID-19. ACS Central Sci. (2020) 6:672–83. doi: 10.1021/acscentsci.0c00489
88. Sheahan TP, Sims AC, Graham RL, Menachery VD, Gralinski LE, Case JB, et al. Broad-spectrum antiviral GS-5734 inhibits both epidemic and zoonotic coronaviruses. Sci Trans Med. (2017) 9: eaal3653. doi: 10.1126/scitranslmed.aal3653
89. Simonis A, Theobald SJ, Fätkenheuer G, Rybniker J, Malin JJ. A comparative analysis of remdesivir and other repurposed antivirals against SARS-CoV-2. EMBO Mol Med. (2021) 13:e13105. doi: 10.15252/emmm.202013105
90. Ader F, Bouscambert-Duchamp M, Hites M, Peiffer-Smadja N, Poissy J, Belhadi D, et al. Remdesivir plus standard of care versus standard of care alone for the treatment of patients admitted to hospital with COVID-19 (DisCoVeRy): a phase 3, randomised, controlled, open-label trial. Lancet Infect Dis. (2022) 22:209–21. doi: 10.1016/S1473-3099(21)00485-0
91. Beigel JH, Tomashek KM, Dodd LE, Mehta AK, Zingman BS, Kalil AC, et al. Remdesivir for the treatment of Covid-19 - final report. New Engl J Med. (2020) 383:1813–26. doi: 10.1056/NEJMoa2007764
92. Pan H, Peto R, Henao-Restrepo AM, Preziosi MP, Sathiyamoorthy V, Abdool Karim Q, et al. Repurposed antiviral drugs for Covid-19 - interim WHO solidarity trial results. New Engl J Med. (2021) 384:497–511. doi: 10.1056/NEJMoa2023184
93. Spinner CD, Gottlieb RL, Criner GJ, Arribas López JR, Cattelan AM, Soriano Viladomiu A, et al. Effect of remdesivir vs standard care on clinical status at 11 days in patients with moderate COVID-19: a randomized clinical trial. JAMA. (2020) 324:1048–57. doi: 10.1001/jama.2020.16349
94. Wang Y, Zhang D, Du G, Du R, Zhao J, Jin Y, et al. Remdesivir in adults with severe COVID-19: a randomised, double-blind, placebo-controlled, multicentre trial. Lancet. (2020) 395:1569–78. doi: 10.1016/S0140-6736(20)31022-9
95. Gilead. Veklury® (Remdesivir) Significantly Reduced Risk of Hospitalization in High-Risk Patients with COVID-19. (2021). Available online at: https://www.gilead.com/news-and-press/press-room/press-releases/2021/9/veklury-remdesivir-significantly-reduced-risk-of-hospitalization-in-highrisk-patients-with-covid19 (accessed March 4, 2022).
96. Schäfer A, Martinez DR, Won JJ, Meganck RM, Moreira FR, Brown AJ, et al. Therapeutic treatment with an oral prodrug of the remdesivir parental nucleoside is protective against SARS-CoV-2 pathogenesis in mice. Sci Transl Med. (2022) 14:eabm3410. doi: 10.1126/scitranslmed.abm3410
97. Furuta Y, Gowen BB, Takahashi K, Shiraki K, Smee DF, Barnard DL. Favipiravir (T-705), a novel viral RNA polymerase inhibitor. Antiviral Res. (2013) 100:446–54. doi: 10.1016/j.antiviral.2013.09.015
98. Shiraki K, Daikoku T. Favipiravir, an anti-influenza drug against life-threatening RNA virus infections. Pharmacol Therap. (2020) 209:107512. doi: 10.1016/j.pharmthera.2020.107512
99. Cai Q, Yang M, Liu D, Chen J, Shu D, Xia J, et al. Experimental treatment with favipiravir for COVID-19: an open-label control study. Engineering. (2020) 6:1192–8. doi: 10.1016/j.eng.2020.03.007
100. Udwadia ZF, Singh P, Barkate H, Patil S, Rangwala S, Pendse A, et al. Efficacy and safety of favipiravir, an oral RNA-dependent RNA polymerase inhibitor, in mild-to-moderate COVID-19: a randomized, comparative, open-label, multicenter, phase 3 clinical trial. Int J Infect Dis. (2021) 103:62–71. doi: 10.1016/j.ijid.2020.11.142
101. Jockusch S, Tao C, Li X, Anderson TK, Ju J. Triphosphates of the two components in DESCOVY and TRUVADA are inhibitors of the SARS-CoV-2 polymerase. bioRxiv [Preprint]. (2020). doi: 10.1101/2020.04.03.022939
102. Elfiky AA. Ribavirin, remdesivir, sofosbuvir, galidesivir, and tenofovir against SARS-CoV-2 RNA dependent RNA polymerase (RdRp): a molecular docking study. Life Sci. (2020) 253:117592. doi: 10.1016/j.lfs.2020.117592
103. Melchjorsen J, Risør MW, Søgaard OS, O'Loughlin KL, Chow S, Paludan SR, et al. Tenofovir selectively regulates production of inflammatory cytokines and shifts the IL-12/IL-10 balance in human primary cells. J Acquired Immune Deficiency Synd. (2011) 57:265–75. doi: 10.1097/QAI.0b013e3182185276
104. Liu QQ, Cheng A, Wang Y, Li H, Hu L, Zhao X, et al. Cytokines and their relationship with the severity and prognosis of coronavirus disease 2019 (COVID-19): a retrospective cohort study. BMJ Open. (2020) 10:e041471. doi: 10.1136/bmjopen-2020-041471
105. Lu L, Zhang H, Dauphars DJ, He YW. A potential role of interleukin 10 in COVID-19 pathogenesis. Trends Immunol. (2021) 42:3–5. doi: 10.1016/j.it.2020.10.012
106. Del Amo J, Polo R, Moreno S, Díaz A, Martínez E, Arribas JR, et al. Incidence and severity of COVID-19 in HIV-positive persons receiving antiretroviral therapy : a cohort study. Ann Internal Med. (2020) 173:536–41. doi: 10.7326/M20-3689
107. Risk factors for coronavirus disease 2019 (COVID-19) death in a population cohort study from the Western Cape Province South Africa. Clin Infect Dis. (2021) 73:e2005–15. doi: 10.1093/cid/ciaa1198
108. Ayerdi O, Puerta T, Clavo P, Vera M, Ballesteros J, Fuentes ME, et al. Preventive efficacy of tenofovir/emtricitabine against severe acute respiratory syndrome coronavirus 2 among pre-exposure prophylaxis users. Open Forum Infect Dis. (2020) 7:ofaa455. doi: 10.1093/ofid/ofaa455
109. Nirmatrelvir. Drugs and Lactation Database (LactMed). Bethesda, MD: National Library of Medicine (US) (2006).
110. Owen DR, Allerton CMN, Anderson AS, Aschenbrenner L, Avery M, Berritt S, et al. An oral SARS-CoV-2 M(pro) inhibitor clinical candidate for the treatment of COVID-19. Science. (2021) 374:1586–93. doi: 10.1126/science.abl4784
111. Chu CM, Cheng VC, Hung IF, Wong MM, Chan KH, Chan KS, et al. Role of lopinavir/ritonavir in the treatment of SARS: initial virological and clinical findings. Thorax. (2004) 59:252–6. doi: 10.1136/thorax.2003.012658
112. de Wilde AH, Jochmans D, Posthuma CC, Zevenhoven-Dobbe JC, van Nieuwkoop S, Bestebroer TM, et al. Screening of an FDA-approved compound library identifies four small-molecule inhibitors of Middle East respiratory syndrome coronavirus replication in cell culture. Antimicrob Agents Chemother. (2014) 58:4875–84. doi: 10.1128/AAC.03011-14
113. Chan JF, Yao Y, Yeung ML, Deng W, Bao L, Jia L, et al. Treatment with lopinavir/ritonavir or interferon-β1b improves outcome of MERS-CoV infection in a nonhuman primate model of common marmoset. J Infect Dis. (2015) 212:1904–13. doi: 10.1093/infdis/jiv392
114. Chan KS, Lai ST, Chu CM, Tsui E, Tam CY, Wong MM, et al. Treatment of severe acute respiratory syndrome with lopinavir/ritonavir: a multicentre retrospective matched cohort study. Hong Kong Med J. (2003) 9:399–406.
115. USFDA. Coronavirus (COVID-19) Update: FDA Authorizes First Oral Antiviral for Treatment of COVID-19. (2021). Available online at: https://www.fda.gov/news-events/press-announcements/coronavirus-covid-19-update-fda-authorizes-first-oral-antiviral-treatment-covid-19 (accessed March 6, 2022).
116. Lange NW, Salerno DM, Jennings DL, Choe J, Hedvat J, Kovac DB, et al. Nirmatrelvir/ritonavir use: managing clinically significant drug-drug interactions with transplant immunosuppressants. Am J Trans. (2022) 22:1925–6. doi: 10.1111/ajt.16955
117. A Drug Safety and Dose-exploratory Clinical Study of Azvudine Tablets in Patients Who Have Not Received Anti-HIV Treatment (FNC). Available online at: https://clinicaltrials.gov/ct2/show/NCT04109183?term=AZVUDINE&draw=2&rank=2term=NCT04257656&draw=2&rank=1 (accessed March 6, 2022).
118. Sun L, Peng Y, Yu W, Zhang Y, Liang L, Song C, et al. Mechanistic insight into antiretroviral potency of 2'-deoxy-2'-β-fluoro-4'-azidocytidine (FNC) with a long-lasting effect on HIV-1 prevention. J Med Chem. (2020) 63:8554–66. doi: 10.1021/acs.jmedchem.0c00940
119. Wang RR, Yang QH, Luo RH, Peng YM, Dai SX, Zhang XJ, et al. Azvudine, a novel nucleoside reverse transcriptase inhibitor showed good drug combination features and better inhibition on drug-resistant strains than lamivudine in vitro. PLoS ONE. (2014) 9:e105617. doi: 10.1371/journal.pone.0105617
120. Zhang JL, Li YH, Wang LL, Liu HQ, Lu SY, Liu Y, et al. Azvudine is a thymus-homing anti-SARS-CoV-2 drug effective in treating COVID-19 patients. Signal Transduction Targeted Ther. (2021) 6:414. doi: 10.1038/s41392-021-00835-6
121. Tyack PL, Calambokidis J, Friedlaender A, Goldbogen J, Southall B., Formal Comment on Schorr GS, Falcone EA, Moretti DJ, Andrews RD (2014) first long-term behavioral records from Cuvier's Beaked Whales (Ziphius cavirostris) reveal record-breaking dives. PLoS ONE 9(3): e92633. PLoS ONE. (2015) 10:e0142287. doi: 10.1371/journal.pone.0142287
122. Smith DB, Kalayanov G, Sund C, Winqvist A, Maltseva T, Leveque VJ, et al. The design, synthesis, and antiviral activity of monofluoro and difluoro analogues of 4'-azidocytidine against hepatitis C virus replication: the discovery of 4'-azido-2'-deoxy-2'-fluorocytidine and 4'-azido-2'-dideoxy-2',2'-difluorocytidine. J Med Chem. (2009) 52:2971–8. doi: 10.1021/jm801595c
123. Xu N, Yang J, Zheng B, Zhang Y, Cao Y, Huan C, et al. The pyrimidine analog FNC potently inhibits the replication of multiple enteroviruses. J Virol. (2020) 94:e00204–20. doi: 10.1128/JVI.00204-20
124. Zhou Y, Zhang Y, Yang X, Zhao J, Zheng L, Sun C, et al. Novel nucleoside analogue FNC is effective against both wild-type and lamivudine-resistant HBV clinical isolates. Antiviral Ther. (2012) 17:1593–9. doi: 10.3851/IMP2292
125. Klumpp K, Kalayanov G, Ma H, Le Pogam S, Leveque V, Jiang WR, et al. 2'-deoxy-4'-azido nucleoside analogs are highly potent inhibitors of hepatitis C virus replication despite the lack of 2'-alpha-hydroxyl groups. J Biol Chem. (2008) 283:2167–75. doi: 10.1074/jbc.M708929200
126. Ren Z, Luo H, Yu Z, Song J, Liang L, Wang L, et al. A randomized, open-label, controlled clinical trial of azvudine tablets in the treatment of mild and common COVID-19, a pilot study. Adv Sci. (2020) 7:2001435. doi: 10.1002/advs.202001435
127. Shah S, Ackley TW, Topal JE. Renal and hepatic toxicity analysis of remdesivir formulations: does what is on the inside really count? Antimicrob Agents Chemother. (2021) 65:e0104521. doi: 10.1128/AAC.01045-21
128. Pettit NN, Pisano J, Nguyen CT, Lew AK, Hazra A, Sherer R, et al. Remdesivir use in the setting of severe renal impairment: a theoretical concern or real risk? Clin Infect Dis. (2021) 73:e3990–e5. doi: 10.1093/cid/ciaa1851
129. Nachega JB, Marconi VC, van Zyl GU, Gardner EM, Preiser W, Hong SY, et al. HIV treatment adherence, drug resistance, virologic failure: evolving concepts. Infect Disord Drug Targets. (2011) 11:167–74. doi: 10.2174/187152611795589663
130. Schmid A, Wolfensberger A, Nemeth J, Schreiber PW, Sax H, Kuster SP. Monotherapy versus combination therapy for multidrug-resistant Gram-negative infections: systematic review and meta-analysis. Sci Rep. (2019) 9:15290. doi: 10.1038/s41598-019-51711-x
131. Rockett R, Basile K, Maddocks S, Fong W, Agius JE, Johnson-Mackinnon J, et al. Resistance mutations in SARS-CoV-2 delta variant after sotrovimab use. N Engl J Med. (2022) 386:1477–9. doi: 10.1056/NEJMc2120219
132. Choi B, Choudhary MC, Regan J, Sparks JA, Padera RF, Qiu X, et al. Persistence and evolution of SARS-CoV-2 in an immunocompromised host. N Engl J Med. (2020) 383:2291–3. doi: 10.1056/NEJMc2031364
133. Hoffmann M, Arora P, Groß R, Seidel A, Hörnich BF, Hahn AS, et al. SARS-CoV-2 variants B.1.351 and P.1 escape from neutralizing antibodies. Cell. (2021) 184:2384–93.e12. doi: 10.1016/j.cell.2021.03.036
134. Starr TN, Greaney AJ, Addetia A, Hannon WW, Choudhary MC, Dingens AS, et al. Prospective mapping of viral mutations that escape antibodies used to treat COVID-19. Science. (2021) 371:850–4. doi: 10.1126/science.abf9302
135. Trogen B, Caplan A. Risk compensation and COVID-19 vaccines. Ann Intern Med. (2021) 174:858–9. doi: 10.7326/M20-8251
Keywords: COVID-19, pre-exposure prophylaxis (PrEP), high-risk population, molnupiravir, remdesivir
Citation: Ouyang J, Zaongo SD, Harypursat V, Li X, Routy J-P and Chen Y (2022) SARS-CoV-2 pre-exposure prophylaxis: A potential COVID-19 preventive strategy for high-risk populations, including healthcare workers, immunodeficient individuals, and poor vaccine responders. Front. Public Health 10:945448. doi: 10.3389/fpubh.2022.945448
Received: 16 May 2022; Accepted: 19 July 2022;
Published: 08 August 2022.
Edited by:
Jesper Damsgaard Gunst, Aarhus University Hospital, DenmarkReviewed by:
Rishad Robin, The Leprosy Mission International Bangladesh, BangladeshCopyright © 2022 Ouyang, Zaongo, Harypursat, Li, Routy and Chen. This is an open-access article distributed under the terms of the Creative Commons Attribution License (CC BY). The use, distribution or reproduction in other forums is permitted, provided the original author(s) and the copyright owner(s) are credited and that the original publication in this journal is cited, in accordance with accepted academic practice. No use, distribution or reproduction is permitted which does not comply with these terms.
*Correspondence: Yaokai Chen, eWFva2FpY2hlbkBob3RtYWlsLmNvbQ==; Jean-Pierre Routy, amVhbi1waWVycmUucm91dHlAbWNnaWxsLmNh
†These authors have contributed equally to this work
Disclaimer: All claims expressed in this article are solely those of the authors and do not necessarily represent those of their affiliated organizations, or those of the publisher, the editors and the reviewers. Any product that may be evaluated in this article or claim that may be made by its manufacturer is not guaranteed or endorsed by the publisher.
Research integrity at Frontiers
Learn more about the work of our research integrity team to safeguard the quality of each article we publish.