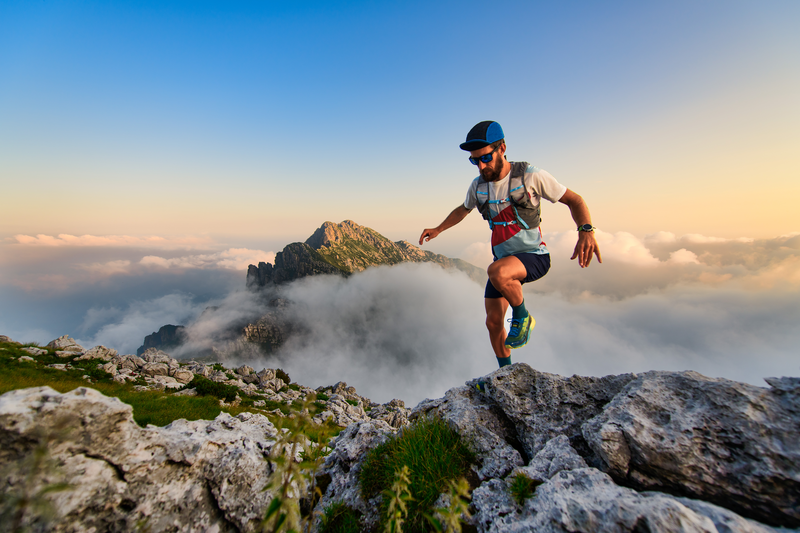
95% of researchers rate our articles as excellent or good
Learn more about the work of our research integrity team to safeguard the quality of each article we publish.
Find out more
SYSTEMATIC REVIEW article
Front. Public Health , 26 April 2022
Sec. Infectious Diseases – Surveillance, Prevention and Treatment
Volume 10 - 2022 | https://doi.org/10.3389/fpubh.2022.881412
This article is part of the Research Topic Novel Targets and State of the Art Therapies in ARDS and Sepsis View all 11 articles
Backgrounds: Hepcidin has been identified as a systemic iron-regulatory hormone. Recent studies have suggested that iron metabolism disorders may be involved in the pathogenesis of acute respiratory distress syndrome and multiple organ dysfunction in coronavirus disease 2019 (COVID-19).
Objectives: To re-evaluate the hepcidin-related iron metabolism parameters and explore the relationship between hepcidin-mediated iron dysmetabolism and COVID-19 severity.
Methods: COVID-19 is classified as mild and moderate as non-severe, severe and critical as severe. A meta-analysis was conducted. Four bibliographic databases were comprehensively searched up to December 31st 2021.
Results: Six unique studies with data from 477 COVID-19 patients were included. Compared to non-severe cases, severe cases had higher hepcidin (standardized mean difference (SMD), −0.39; 95% Confidence Interval (CI) [−0.76, −0.03]; P = 0.03) and ferritin (SMD, −0.84; 95% CI [−1.30, −0.38]; P = 0.0004). In five out of six studies, a total of 427 patients were tested for serum iron, and there were significant differences in their levels between severe and non-severe cases (SMD, 0.22; 95% CI [0.02, 0.41]; P = 0.03). A total of 320 patients from four out of six studies were tested for transferrin saturation, and the statistical difference was not significant (SMD, 0.06; 95% CI [−0.17, 0.28]; P = 0.64).
Conclusion: Severe COVID-19 cases had higher serum levels of hepcidin and ferritin, and lower serum iron, without significant differences in transferrin saturation. Further studies are needed to verify whether targeting the hepcidin-mediated iron metabolism axis may influence the outcome and treatment of COVID-19.
Since the outbreak of coronavirus disease 2019 (COVID-19) caused by severe acute respiratory syndrome coronavirus 2 (SARS-CoV-2) (1), the number of confirmed cases has increased rapidly around the world (2, 3). Acute respiratory distress syndrome (ARDS) and multiple organ dysfunction are the main clinical manifestations and leading causes of mortality in severe COVID-19 cases (4). Iron homeostasis is crucial for host immune defense and inflammatory response. Disorders of iron metabolism are mainly manifested as iron deficiency and/or overload, both of which can cause cellular and organ dysfunction (5). Low serum iron (SI) concentration restricts hemoglobin synthesis and causes anemia and systemic hypoxemia. Emerging data have found that hypoferremia is an independent risk factor for hypoxic respiratory failure and death in COVID-19 patients (6, 7). The rapid and excessive accumulation of intracellular iron, especially in macrophages, causes cell and tissue damage, presumably through the production of reactive oxygen species (ROS) catalyzed by iron. In addition, iron overload may also trigger a unique iron-dependent form of non-apoptotic cell death termed ferroptosis (8). Serum ferritin concentration has been proven to reflect the individual's iron storage status. Studies have found that increased serum ferritin levels are associated with adverse outcomes (9–11). Although studies on transferrin saturation (TSAT) have been far fewer than that of ferritin and hypoferritemia, low TSAT has been consistently reported in severe COVID-19, and significantly correlated to lung aeration loss, inflammatory markers and worse outcomes (7, 12–14).
The hepatic peptide hepcidin has been identified as the systemic iron-regulatory hormone and plays an important role in maintaining iron homeostasis (15). Hepcidin regulates intestinal iron absorption, SI concentrations, and tissue iron redistribution by inducing the degradation of its receptor, the sole iron exporter ferroportin (14, 16), and blocking ferroportin export activity (17). Serum hepcidin measurement may be a promising tool for assessing the status of iron metabolism (18), but has not been widely implemented in clinical practice (14, 19, 20). Notably, unlike serum ferritin testing that has been documented in guidelines, hepcidin has been rarely measured in COVID-19 patients (13, 21). As expected in severe inflammatory diseases, recent studies have reported that most COVID-19 patients showed varying degrees of upregulation of hepcidin levels (16, 21–25). More importantly, hepcidin levels have significantly negatively correlated with the ratio of arterial oxygen partial pressure (PaO2) and fraction of inspired oxygen (FiO2), which can be used to predict COVID-19 severity and mortality (22). To adopt the results for clinical use, these interesting findings need to be validated in wider cohorts, and the relationship between hepcidin-mediated iron dysmetabolism and COVID-19 severity also needs to be further analyzed. In view of the particularity of the prevention and control of the COVID-19 epidemic and the randomness of its incidence, large-scale, multi-center, prospective cohort studies are very difficult to achieve. Here we classify mild and moderate as non-severe, severe, and critical as severe, and conduct a meta-analysis to re-evaluate the hepcidin-related iron metabolism parameters (hepcidin, ferritin, iron, and TSAT) in COVID-19 patients and explore the relationship between iron dysmetabolism and severity.
This meta-analysis was performed following a recently published guideline and reported according to PRISMA (Preferred Reporting Items for Systematic Reviews and Meta-Analyses) guidelines (26). We searched comprehensively MEDLINE (National Library of Medicine, US), EMBASE (Elsevier, Netherlands), Cochrane (Cochrane Collaboration, UK), and the WHO COVID-19 database to identify relevant articles up to December 31, 2021. Keywords include “COVID-19,” “SARS-CoV-2,” and iron metabolism biomarkers, including “hepcidin,” “ferritin,” “serum iron,” “transferrin,” “soluble transferrin receptor,” “unsaturated iron binding capacity,” and “transferrin saturation,” The detailed search strategy is given in Supplementary File 1.
We follow the international guidelines for the diagnosis and treatment of COVID-19. The detailed severity classification criteria used in the evaluation of original studies and meta-analyses include: (1) Mild: asymptomatic or mild clinical symptoms, and no pneumonia on imaging, or outward treatment; (2) Moderate: fever, respiratory tract symptoms, pneumonia on imaging, and inward treatment; (3) Severe: dyspnea, or respiratory rate >30 breaths/min at rest, or oxygen saturation <93%, or PaO2/FiO2 < 300 mmHg; (4) Critical: respiratory failure and need for mechanical ventilation, or shock, or combined with other organ failure should be treated in the Intensive Care Unit. Severe and critical categories were defined as severe, mild, and moderate as non-severe in data analysis.
Inclusion criteria:
(1) All observational studies (e.g., cross-sectional, longitudinal, case-control, and cohort) with prospective or retrospective designs.
(2) Studies published in English, including preprints.
(3) Studies investigating hepcidin-mediated iron metabolism in COVID-19 cases.
Exclusion criteria:
(1) Duplicate studies, or repeated published studies.
(2) Studies in which accurate data cannot be obtained or some data are missing.
(3) Studies not strictly grouped by severity.
The extraction methodology was discussed and formulated by the team. Two of us (ZL and HW) independently screened the full text according to the selection criteria, and recorded information about the author's name, publication time, study location, study design, sample size, laboratory results of iron metabolism parameters, and COVID-19 severity in the data file. All laboratory values were converted to conventional units based on the US National Institute of Standards and Technology conversion factors. For studies that only reported the median and interquartile range, we converted these values into mean and standard deviation according to the methods reported by Hozo et al. (27) and Wan et al. (28).
Two authors (LZ and YG) independently used the Newcastle-Ottawa scale to assess the quality of the included studies (29). A third author (DP) ruled in case consensus was not reached. The scale was developed for non-random and observational studies including case-control, cross-sectional, longitudinal, and cohort studies (if applicable). The quality was assessed using a 9-star system. Studies rated ≥6 stars were defined as “acceptable quality” and proceeded to the final meta-analysis step.
This meta-analysis used RveMan5.4 (Cochrane Collaboration), with inverse variance as the statistical method to calculate standardized mean differences (SMDs) and corresponding 95% confidence intervals (CIs) for continuous variables. A P < 0.05 was determined to be statistically significant. The I2 statistic was used to assess the heterogeneity among the analyzed studies. An I2 > 50% or P < 0.10 indicated heterogeneity, for which the random effects model was used. Otherwise, the fixed effects model was used. In addition, visual inspection of funnel plots was used to evaluate publication bias.
Sixty-four unique citations were identified, of which 48 non-observational studies were excluded and 16 were selected for full-text eligibility assessment. Of these, six observational studies including 477 COVID-19 patients were included in the final data analysis and synthesis. The flow chart and details of study selection results can be found in Figure 1 and Supplementary File 1. The detailed characteristics of the included studies were shown in Table 1.
Six unique studies with data from 477 COVID-19 patients were included. Hepcidin and ferritin were assessed in six out of six (100%) studies. Heterogeneity was assessed at P = 0.006, I2 = 70% and P = 0.0001, I2 = 80%, respectively (Figures 2A,B). Both of them had significant heterogeneity, and the random effects model was used. Compared to non-severe cases, severe cases have higher hepcidin (SMD, −0.39; 95% CI [−0.76, −0.03]; P = 0.03) and ferritin (SMD, −0.84; 95% CI [−1.30, −0.38]; P = 0.0004). In five out of six (83.3%) studies, a total of 427 patients were tested for SI, and there were significant differences in their levels between severe and non-severe cases (SMD, 0.22; 95% CI [0.02, 0.41]; P = 0.03). A total of 320 patients from four out of six (66.7%) studies were tested for TSAT, and the statistical difference was insignificant (SMD, 0.06; 95% CI [−0.17, 0.28]; P = 0.64). Heterogeneity was assessed at P = 0.38, I2 = 4% and P = 0.66, I2 = 0%, respectively (Figures 2C,D). Neither of them was significantly heterogenous, and the fixed effects model was used.
Figure 2. Comparison of iron metabolism parameters between non-severe and severe COVID-19 cases. (A) Hepcidin; (B) Ferritin; (C) Serum iron; (D) Transferrin saturation (TSAT).
All six included studies had NOS scores ≥6 stars, and their quality was acceptable. The funnel plots of SMDs in hepcidin and ferritin (both included six studies) were asymmetric, suggesting the possible presence of publication bias. For SI and TAST, five and four studies were included, respectively, and we found no evidence of publication bias (Supplementary File 1).
To our knowledge, this study is the first meta-analysis of the relationship between hepcidin-mediated iron dysmetabolism and COVID-19 severity. However, there is significant heterogeneity among six studies included in the data synthesis of hepcidin and ferritin. The possible reasons are analyzed as follows: (a) The time point and time course of laboratory testing were different. Some studies collected blood samples at admission (21–23), another 2 months after onset (16), and others throughout the hospital stay (24, 25). In addition, the detection methods and the reagents used were not the same. Enzyme-linked immunosorbent assay (ELISA) kits (16, 21–24) or competitive enzyme immunoassay (EIA) kits (25) were used to detect hepcidin. (b) Iron metabolism is strongly influenced by gender, with females generally having lower SI and hepcidin levels than males (22). Also, males have a higher ratio of severe COVID-19 cases. There were potential effects on heterogeneity due to differences in the composition of males and females between studies. (c) Our study classified mild and moderate as non-severe, severe and critical as severe. Differences in the proportions and sample sizes of cases of distinct severity between studies contributed to heterogeneity. Unfortunately, hepcidin and its related iron metabolism parameters are rarely tested simultaneously in COVID-19, and there are even fewer original studies analyzing the relationship between hepcidin and disease severity. Also, subgroup analysis could not be performed due to the lack of access to raw data. Furthermore, only the Sonnweber 2020 study tested hepcidin and ferritin 2 months after onset. We removed this study and performed a new meta-analysis and found no significant change in the final overall effect (Supplementary Figure 1). Therefore, from the results of the meta-analysis, differences in blood collection at different stages of COVID-19 between studies may not affect the fact that severe cases have higher levels of hepcidin and ferritin. This also suggests that hepcidin-mediated iron dysmetabolism throughout the course of COVID-19 may be some very promising therapeutic targets. Comprehensively, these studies met rigid criteria for inclusion and were homogeneous in terms of study design and objectives, as well as cohort settings. Therefore, the final overall effect generated by the random effects model brings novel insights into the biological effects of COVID-19.
Hepcidin, the key iron metabolism regulatory hormone, sequesters iron and prevents iron efflux in enterocytes and macrophages, resulting in increased intracellular ferritin and hypoferremia (30, 31). Physiologically, hepcidin synthesis by hepatocytes is reactively upregulated or downregulated by high or low SI, respectively. Other hepcidin agonists are inflammatory cytokines represented by interleukin-6 (IL-6). Conversely, hepcidin is antagonized by hypoxemia, with hypoxia induced factors (HIF) release (15, 32). Our study found that severe COVID-19 cases had higher serum levels of hepcidin and ferritin, and lower SI, without significant differences in TSAT.
These findings were consistent with most of other studies. Unexpectedly, hepcidin levels in severe COVID-19 cases did not appear to be downregulated by hypoferremia in a feedback manner and antagonized by systemic hypoxemia. Hyperferritinemia inevitably promotes the production of ROS and lipoperoxidation, which ultimately leads to extensive cell and tissue damage possibly through the ferroptosis pathway, and cascade-amplified inflammation (32, 33). In turn, hyperinflammation will transcriptionally upregulate hepcidin, further exacerbating iron dysmetabolism. We speculate that the potential intervention targets for this vicious circle may lie in the hepcidin-mediated iron metabolism axis.
Cytokine storm is a hallmark of the hyperinflammatory state of COVID-19 and is closely linked to disturbances in iron metabolism (30, 34). A growing body of studies have suggested that several components of elevated inflammatory status may be effective therapeutic targets, notably the administration of IL-6 receptor antagonists such as tocilizumab, which significantly reduces the mortality of severe COVID-19 cases (35, 36). However, the effective suppression of the excessive inflammatory response has also raised concerns about prolonged SARS-CoV-2 clearance. Furthermore, data on whether IL-6 receptor antagonists can correct hepcidin-mediated iron dysmetabolism in COVID-19 cases have been lacking to date.
Ehsani highlighted the striking similarity between the amino acid sequence of the SARS-CoV-2 spike glycoprotein and the hepcidin protein (37). This observation provides ideas for vaccine design and bioengineered antibody development for SARS-CoV-2. Hepcidin-mimetic action of SARS-CoV-2 may markedly increase circulating and tissue ferritin, while inducing SI deficiency (32, 33). However, whether SARS-CoV-2 utilizes ferroportin on the cell membrane as another binding receptor for the spike protein to invade host cells requires further analysis. Moreover, whether SARS-CoV-2 directly degrades ferroportin through mimicking hepcidin or indirectly upregulates hepcidin through inflammation to mediate iron dysmetabolism also needs to be carefully investigated. In animal models, hepcidin-neutralizing monoclonal antibodies have been shown to reverse inflammatory anemia (38). Recently, an antibody targeting ferroportin has been described and hypothesized to reduce ferroportin degradation by interfering with hepcidin binding, thereby increasing SI (39). The application of hepcidin antibodies to block viral entry and correct iron metabolism disturbances in COVID-19 cases may be a very promising therapeutic approach in the future.
Hyperferritinemia in COVID-19 lies downstream of the hepcidin-mediated iron metabolism axis. Iron chelation has been shown to reduce viral replication and exert anti-inflammatory effects in viral infections (32, 40, 41). Multiple evidences have shown that deferoxamine reduces the levels of IL-6, the main inflammatory mediator that triggers cytokine storm, mimics HIF, and downregulates hepcidin (40, 42). However, the application of iron chelators in COVID-19 has brought great controversy (42–44). The iron dysmetabolism of COVID-19 is essentially abnormal iron distribution; the coexistence of low SI and local intracellular iron overload, and total iron in the body may not increase. Therefore, the safety and efficacy of systemic iron chelator administration has been challenged (43), and hepcidin antagonists may be preferred as supportive treatments for COVID-19 compared with iron chelators. Four clinical trials of iron chelation are currently underway (NCT04333550, NCT04361032, NCT04389801, IRCT20200506047323N4) and the results are pending.
There are some limitations to this study that need to be noted. First, most of the studies were single-center, retrospective, with small sample sizes, and may be subject to confounding and bias. Second, the six studies used for hepcidin and ferritin data synthesis were heterogeneous, subgroup analyses were not performed, and the results from random-effects models may be inaccurate. Third, the literature search may not be completely comprehensive, resulting in the omission of a few relevant studies. Fourth, this included preprint is a preliminary manuscript version. There are certain risks to the validity and applicability of the data it provides.
Severe COVID-19 cases had higher serum levels of hepcidin and ferritin, and lower SI, without significant differences in TSAT. Compared with other clinically applied therapeutic options targeting the iron metabolism axis, such as IL-6 receptor antagonists and iron chelators, hepcidin antibody may be more promising, but further studies are needed to verify whether targeting the hepcidin-mediated iron metabolism axis may influence the outcome and treatment of COVID-19.
The original contributions presented in the study are included in the article/Supplementary Material, further inquiries can be directed to the corresponding author/s.
DP was responsible for methodology, investigation, formal analysis, data curation, writing the original draft, and visualization. YG and LZ for investigation, formal analysis, and data curation. YL for conceptualization, investigation, review and editing, and supervision. ZL and HW worked on conceptualization, formal analysis, investigation, and data curation. All authors have read and approved the final manuscript version to be submitted.
The authors declare that the research was conducted in the absence of any commercial or financial relationships that could be construed as a potential conflict of interest.
All claims expressed in this article are solely those of the authors and do not necessarily represent those of their affiliated organizations, or those of the publisher, the editors and the reviewers. Any product that may be evaluated in this article, or claim that may be made by its manufacturer, is not guaranteed or endorsed by the publisher.
The authors thank Professor Hai Rao, School of Medicine, Southern University of Science and Technology, China, and Cindy Acon Chen, USA, for reviewing this manuscript.
The Supplementary Material for this article can be found online at: https://www.frontiersin.org/articles/10.3389/fpubh.2022.881412/full#supplementary-material
ARDS, acute respiratory distress syndrome; CI, confidence interval; COVID-19, coronavirus disease 2019; FiO2, fraction of inspired oxygen; HIF, hypoxia induced factor; NOS, Newcastle-Ottawa quality assessment scale; PaO2, arterial oxygen partial pressure; SARS-CoV-2, severe acute respiratory syndrome coronavirus 2; SMD, standardized mean difference; SI, serum iron; TSAT, transferrin saturation.
1. Huang C, Wang Y, Li X, Ren L, Zhao J, Hu Y, et al. Clinical features of patients infected with 2019 novel coronavirus in Wuhan, China. Lancet. (2020) 395:497–506. doi: 10.1016/S0140-6736(20)30183-5
2. Li Q, Guan X, Wu P, Wang X, Zhou L, Tong Y, et al. Early Transmission dynamics in Wuhan, China, of novel coronavirus-infected pneumonia. N Engl J Med. (2020) 382:1199–207. doi: 10.1056/NEJMoa2001316
3. Wu C, Chen X, Cai Y, Xia J, Zhou X, Xu S, et al. Risk factors associated with acute respiratory distress syndrome and death in patients with coronavirus disease 2019 pneumonia in Wuhan, China. JAMA Intern Med. (2020) 180:934–43. doi: 10.1001/jamainternmed.2020.0994
4. Ruan Q, Yang K, Wang W, Jiang L, Song J. Clinical predictors of mortality due to COVID-19 based on an analysis of data of 150 patients from Wuhan, China. Intensive Care Med. (2020) 46:846–48. doi: 10.1007/s00134-020-05991-x
5. Ganz T, Nemeth E. Iron homeostasis in host defence and inflammation. Nat Rev Immunol. (2015) 15:500–10. doi: 10.1038/nri3863
6. Shah A, Frost JN, Aaron L, Donovan K, Drakesmith H. Systemic hypoferremia and severity of hypoxemic respiratory failure in COVID-19. Crit Care. (2020) 24:320. doi: 10.1186/s13054-020-03051-w
7. Zhao K, Huang J, Dai D, Feng Y, Liu L, Nie S. Serum iron level as a potential predictor of coronavirus disease 2019 severity and mortality: a retrospective study. Open Forum Infect Dis. (2020) 7:ofaa250. doi: 10.1093/ofid/ofaa250
8. Stockwell BR, Friedmann Angeli JP, Bayir H, Bush AI, Conrad M, Dixon SJ, et al. Ferroptosis: a regulated cell death nexus linking metabolism, redox biology, and disease. Cell. (2017) 171:273–85. doi: 10.1016/j.cell.2017.09.021
9. Lv Y, Chen L, Liang X, Liu X, Gao M, Wang Q, et al. Association between iron status and the risk of adverse outcomes in COVID-19. Clin Nutr. (2021) 40:3462–9. doi: 10.1016/j.clnu.2020.11.033
10. Huang I, Pranata R, Lim MA, Oehadian A, Alisjahbana B. C-reactive protein, procalcitonin, D-dimer, and ferritin in severe coronavirus disease-2019: a meta-analysis. Ther Adv Respir Dis. (2020) 14:1753466620937175. doi: 10.1177/1753466620937175
11. Kappert K, Jahić A, Tauber R. Assessment of serum ferritin as a biomarker in COVID-19: bystander or participant? Insights by comparison with other infectious and non-infectious diseases. Biomarkers. (2020) 25:616–25. doi: 10.1080/1354750X.2020.1797880
12. Bolondi G, Russo E, Gamberini E, Circelli A, Meca MCC, Brogi E, et al. Iron metabolism and lymphocyte char- acterisation during Covid-19 infection in ICU patients: an observational cohort study. World J Emerg Surg. (2020) 15:41. doi: 10.1186/s13017-020-00323-2
13. Hippchen T, Altamura S, Muckenthaler MU, Merle U. Hypoferremia is associated with increased hospitalization and oxygen demand in COVID-19 patients. Hemasphere. (2020) 4:e492. doi: 10.1097/HS9.0000000000000492
14. Girelli D, Marchi G, Busti F, Vianello A. Iron metabolism in infections: focus on COVID-19. Semin Hematol. (2021) 58:182–7. doi: 10.1053/j.seminhematol.2021.07.001
15. Ganz T, Nemeth E. Hepcidin and iron homeostasis. Biochim Biophys Acta. (2012) 1823:1434–43. doi: 10.1016/j.bbamcr.2012.01.014
16. Sonnweber T, Boehm A, Sahanic S, Pizzini A, Aichner M, Sonnweber B, et al. Persisting alterations of iron homeostasis in COVID-19 are associated with non-resolving lung pathologies and poor patients' performance: a prospective observational cohort study. Respir Res. (2020) 21:276. doi: 10.1186/s12931-020-01546-2
17. Aschemeyer S, Qiao B, Stefanova D, Valore EV, Sek AC, Ruwe TA, et al. Structure-function analysis of ferroportin defines the binding site and an alternative mechanism of action of hepcidin. Blood. (2018) 131:899–910. doi: 10.1182/blood-2017-05-786590
18. Stoffel NU, Lazrak M, Bellitir S, Mir NE, Hamdouchi AE, Barkat A, et al. The opposing effects of acute inflammation and iron deficiency anemia on serum hepcidin and iron absorption in young women. Haematologica. (2019) 104:1143–9. doi: 10.3324/haematol.2018.208645
19. Girelli D, Nemeth E, Swinkels DW. Hepcidin in the diagnosis of iron disorders. Blood. (2016) 127:2809–13. doi: 10.1182/blood-2015-12-639112
20. Lasocki S, Lefebvre T, Mayeur C, Mebazaa A, Gayat E; FROG-ICU study group. Iron deficiency diagnosed using hepcidin on critical care discharge is an independent risk factor for death and poor quality of life at one year: an observational prospective study on 1161 patients. Crit Care. (2018) 22:314. doi: 10.1186/s13054-018-2253-0
21. Zhou C, Chen Y, Ji Y, He X, Xue D. Increased serum levels of hepcidin and ferritin are associated with severity of COVID-19. Med Sci Monit. (2020) 26:e926178. doi: 10.12659/MSM.926178
22. Nai A, Lore NI, Pagani A, De Lorenzo R, Di Modica S, Saliu F, et al. Hepcidin levels predict Covid-19 severity and mortality in a cohort of hospitalized Italian patients. Am J Hematol. (2021) 96:E32–5. doi: 10.1002/ajh.26027
23. Yagci S, Serin E, Acicbe Ö, Zeren MI, Odabaşi MS. The relationship between serum erythropoietin, hepcidin, and haptoglobin levels with disease severity and other biochemical values in patients with COVID-19. Int J Lab Hematol. (2021) 43(Suppl. 1):142–51. doi: 10.1111/ijlh.13479
24. Chakurkar V, Rajapurkar M, Lele S, Mukhopadhyay B, Lobo V, Injarapu R, et al. Increased serum catalytic iron may mediate tissue injury and death in patients with COVID-19. Sci Rep. (2021) 11:19618. doi: 10.1038/s41598-021-99142-x
25. Duca L, Nava I, Vallisa D, Vadacca G, Magnacavallo A, Vercelli A . COVID-19, inflammatory response, iron homeostasis and toxicity: a prospective cohort study in the Emergency Department of Piacenza (Italy). Research Square (2021) (preprint). Available online: https://www.researchsquare.com/article/rs-1085949/v1
26. Muka T, Glisic M, Milic J, Verhoog S, Bohlius J, Bramer W, et al. A 24-step guide on how to design, conduct, and successfully publish a systematic review and meta-analysis in medical research. Eur J Epidemiol. (2020) 35:49–60. doi: 10.1007/s10654-019-00576-5
27. Hozo SP, Djulbegovic B, Hozo I. Estimating the mean and variance from the median, range, and the size of a sample. BMC Med Res Methodol. (2005) 5:13. doi: 10.1186/1471-2288-5-13
28. Wan X, Wang W, Liu J, Tong T. Estimating the sample mean and standard deviation from the sample size, median, range and/or interquartile range. BMC Med Res Methodol. (2014) 14:135. doi: 10.1186/1471-2288-14-135
29. Stang A. Critical evaluation of the Newcastle-Ottawa scale for the assessment of the quality of nonrandomized studies in meta-analyses. Eur J Epidemiol. (2010) 25:603–5. doi: 10.1007/s10654-010-9491-z
30. Edeas M, Saleh J, Peyssonnaux C. Iron: innocent bystander or vicious culprit in COVID-19 pathogenesis? Int J Infect Dis. (2020) 97:303–5. doi: 10.1016/j.ijid.2020.05.110
31. Daher R, Manceau H, Karim Z. Iron metabolism and the role of the iron-regulating hormone hepcidin in health and disease. Presse Med. (2017) 46(12 Pt 2):e272–8. doi: 10.1016/j.lpm.2017.10.006
32. Cavezzi A, Troiani E, Corrao S. COVID-19: hemoglobin, iron, and hypoxia beyond inflammation. A narrative review. Clin Pract. (2020) 10:1271. doi: 10.4081/cp.2020.1271
33. Hirschhorn T, Stockwell BR. The development of the concept of ferroptosis. Free Radic Biol Med. (2019) 133:130–43. doi: 10.1016/j.freeradbiomed.2018.09.043
34. Mehta P, McAuley DF, Brown M, Sanchez E, Tattersall RS, Manson JJ. COVID-19: consider cytokine storm syndromes and immunosuppression. Lancet. (2020) 395:1033–4. doi: 10.1016/S0140-6736(20)30628-0
35. Moore JB, June CH. Cytokine release syndrome in severe COVID-19. Science. (2020) 368:473–4. doi: 10.1126/science.abb8925
36. Zhang C, Wu Z, Li JW, Zhao H, Wang GQ. Cytokine release syndrome in severe COVID-19: interleukin-6 receptor antagonist tocilizumab may be the key to reduce mortality. Int J Antimicrob Agents. (2020) 55:105954. doi: 10.1016/j.ijantimicag.2020.105954
37. Ehsani S. COVID-19 and iron dysregulation: distant sequence similarity between hepcidin and the novel coronavirus spike glycoprotein. Biol Direct. (2020) 15:19. doi: 10.1186/s13062-020-00275-2
38. Sasu BJ, Cooke KS, Arvedson TL, Plewa C, Ellison AR, Sheng J, et al. Antihepcidin antibody treatment modulates iron metabolism and is effective in a mouse model of inflammation-induced anemia. Blood. (2010) 115:3616–24. doi: 10.1182/blood-2009-09-245977
39. Sheetz M, Barrington P, Callies S, Berg PH, McColm J, Marbury T, et al. Targeting the hepcidin-ferroportin pathway in anaemia of chronic kidney disease. Br J Clin Pharmacol. (2019) 85:935–48. doi: 10.1111/bcp.13877
40. Liu W, Zhang S, Nekhai S, Liu S. Depriving iron supply to the virus represents a promising adjuvant therapeutic against viral survival. Curr Clin Microbiol Rep. (2020) 20:1–7. doi: 10.1007/s40588-020-00140-w
41. Abobaker A. Can iron chelation as an adjunct treatment of COVID-19 improve the clinical outcome? Eur J Clin Pharmacol. (2020) 76:1619–20. doi: 10.1007/s00228-020-02942-9
42. Perricone C, Bartoloni E, Bursi R, Cafaro G, Guidelli GM, Shoenfeld Y, et al. COVID-19 as part of the hyperferritinemic syndromes: the role of iron depletion therapy. Immunol Res. (2020) 68:213–24. doi: 10.1007/s12026-020-09145-5
43. Garrick MD, Ghio AJ. Iron chelation may harm patients with COVID-19. Eur J Clin Pharmacol. (2021) 77:265–6. doi: 10.1007/s00228-020-02987-w
Keywords: COVID-19, ferritin, hepcidin, iron metabolism, severity
Citation: Peng D, Gao Y, Zhang L, Liu Z, Wang H and Liu Y (2022) The Relationship Between Hepcidin-Mediated Iron Dysmetabolism and COVID-19 Severity: A Meta-Analysis. Front. Public Health 10:881412. doi: 10.3389/fpubh.2022.881412
Received: 22 February 2022; Accepted: 30 March 2022;
Published: 26 April 2022.
Edited by:
Daniel O'Toole, National University of Ireland Galway, IrelandReviewed by:
Alessia Pagani, San Raffaele Hospital (IRCCS), ItalyCopyright © 2022 Peng, Gao, Zhang, Liu, Wang and Liu. This is an open-access article distributed under the terms of the Creative Commons Attribution License (CC BY). The use, distribution or reproduction in other forums is permitted, provided the original author(s) and the copyright owner(s) are credited and that the original publication in this journal is cited, in accordance with accepted academic practice. No use, distribution or reproduction is permitted which does not comply with these terms.
*Correspondence: Yingxia Liu, eWluZ3hpYWxpdUBob3RtYWlsLmNvbQ==
Disclaimer: All claims expressed in this article are solely those of the authors and do not necessarily represent those of their affiliated organizations, or those of the publisher, the editors and the reviewers. Any product that may be evaluated in this article or claim that may be made by its manufacturer is not guaranteed or endorsed by the publisher.
Research integrity at Frontiers
Learn more about the work of our research integrity team to safeguard the quality of each article we publish.