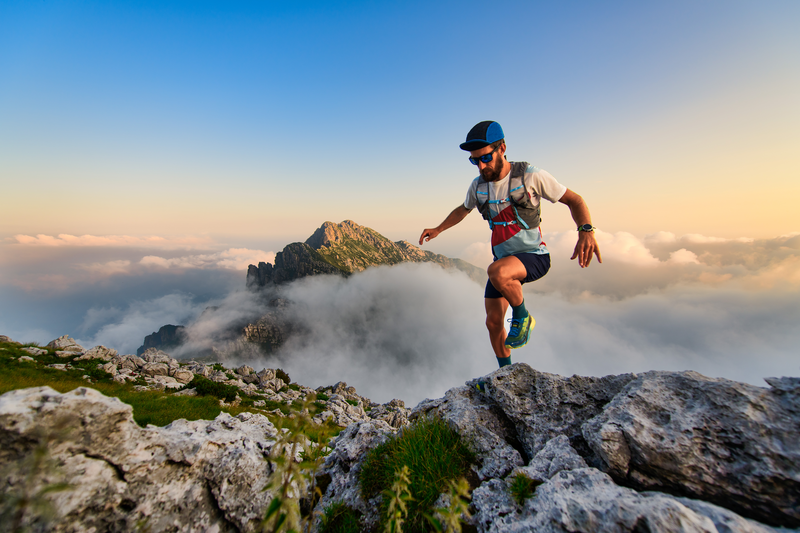
94% of researchers rate our articles as excellent or good
Learn more about the work of our research integrity team to safeguard the quality of each article we publish.
Find out more
ORIGINAL RESEARCH article
Front. Public Health , 09 June 2022
Sec. Infectious Diseases: Epidemiology and Prevention
Volume 10 - 2022 | https://doi.org/10.3389/fpubh.2022.850851
Dengue fever (DF) is endemic to Makkah and Jeddah, the Kingdom of Saudi Arabia (KSA). However, until recently, the circulation of dengue virus (DENV) in Aedes mosquitoes in these areas was unknown. Serological surveillance of DENV in Ae aegypti is a powerful tool for early detection of dengue outbreaks and essential for developing effective control strategies. Therefore, this research aimed to examine a sample of adult Ae aegypti mosquitoes from Makkah, KSA, to detect DENV. In total, 1295 Ae aegypti mosquitoes were collected from the field from target areas of Makkah with a high incidence and prevalence of DF. The samples were divided into 259 coded pools (five mosquitoes in each) and preserved in 1.5 mL plastic tubes. The tubes were labeled, capped, and stored at−86°C until use. RT-PCR was used to detect DENV in the samples. All positive pools were confirmed by RT-PCR. The RT-PCR products were analyzed by gel electrophoresis (1.5% agarose in Tris-acetate EDTA buffer), stained with ethidium bromide, and visualized. DENV was isolated from six female Ae Aegypti collected from six pools (out of 259 pools). No other viruses were detected. Only five of the nine target localities had positive pools. Samples from the remaining four localities yielded negative results. Four DENV-positive mosquitoes were collected at the aquatic stages, and two were collected at the adult stage. These results show the circulation of DENV in adult mosquitoes and offspring, indicating vertical transmission of DENV. In conclusion, this study found that, in Makkah, DENV is circulating in dengue vectors with a high significance rate, suggesting the possibility of a dengue outbreak in the future; therefore, a sensitive surveillance system is vital to predict the outbreak and for early intervention and control.
Dengue virus (DENV) is a single-stranded RNA virus of the family Flaviviridae. It is the most prominent mosquito-borne arbovirus worldwide (1, 2). The global incidence of dengue has dramatically increased in recent decades, and it is estimated that about half of the worldwide population is now at risk of dengue fever (DF) and dengue hemorrhagic fever. DENV is mainly transmitted by Aedes aegypti, a mosquito species that breeds and flourishes in stagnant water containers maintained by rain or human activity (3).
Increasing mobility within and between cities and countries has led to the introduction of DENV into new areas (4). This is illustrated very clearly in the Kingdom of Saudi Arabia (KSA) as Muslims from all over the world visit the KSA for Hajj and Umrah (pilgrimage). As many pilgrims come from endemic regions, dengue has been introduced into the Hajj and Umrah regions, including three major cities: Makkah, Jeddah and Al-Madinah. Furthermore, internal factors, including ecological changes, urbanization, and human behaviors that increase breeding sites, facilitate the spread of Ae aegypti (5, 6). All three cities in the Hajj and Umrah regions have had major dengue outbreaks from time to time (7–9). In 2009, the Saudi Ministry of Health (MOH) reported 3350 cases of DF in the KSA and estimated the case fatality rate to be 4.6 per 1000 (8).
One of the problems of epidemiological surveillance in DF in the KSA is the underestimation of DF cases. Dengue has been described as an iceberg with a tip presenting only a fraction of the symptomatic dengue cases reported (10). This demonstrates the alarming magnitude of silent virus transmission and the weakness of the current surveillance systems (11), which are responsible for multiple outbreaks.
Entomological surveillance can help detect DENV much earlier in Ae aegypti than in humans (12). DENVs have been detected in Ae aegypti mosquitoes from endemic and epidemic regions using surveillance systems based on clinical and laboratory diagnoses. During outbreaks, such detection help identify areas with a higher rate of transmission of the DENV, allowing vector control authorities to prioritize their efforts and focus on regions where people are at the most risk of disease (13, 14).
Effective vector control (a key component for dengue control) relies on the knowledge of local DENV species distribution and vector and human behaviors that may allow mosquitoes to avoid contact with interventions. Furthermore, surveillance of DENV-infected mosquitoes provides an early warning sign for the risk of transmission in an area and indicates the predominant circulating serotype in the vector population (15). This may enable the immediate implementation of vector control measures to prevent outbreaks. A more effective approach is to detect DENV in mosquitoes before its introduction into a human host. This approach enables the immediate implementation of vector control measures to prevent outbreaks. Furthermore, this approach provides an early warning sign for the risk of transmission in an area and indicates the predominant circulating serotype in the vector population (15).
We previously conducted a longitudinal survey of Aedes mosquitoes in 42,981 potential Aedes larval breeding sites in Makkah, KSA. We found a sizeable immature population and adaptation of Ae aegypti to the arid climate of Makkah (6). The objectives of the current study were to screen a sample of Ae aegypti mosquitoes from Makkah, KSA for DENV to identify the magnitude of DENV circulation in the Ae aegypti mosquito population among both adult and offspring mosquitos. This information will help the concerned authorities to develop guidelines for dengue surveillance programs in Makkah based on sensitive indicators that can facilitate preparedness, alertness, and early detection of outbreaks.
Between March 2016 and December 2016, 2343 houses from nine localities in Makkah, KSA, were inspected for adult and aquatic stages of DENV. According to the epidemiological surveillance of the vector-borne disease program in Makkah, these nine localities have high suspected and confirmed DF cases. The vector-borne disease program established a local protocol wherein using the locations of confirmed DF cases as center points (households), the area within a radius of 0.5 km was carefully inspected. Each house was inspected once during the study period. Adult mosquitoes were collected using either blacklight traps set up in the selected areas for 24 h and/or manually caught using hand nets or aspirators between 4 and 6 p.m. (before dusk).
For aquatic collection, larvae at different stages were collected from households with breeding sites. World Health Organization (WHO) standard entomological kits (mosquito inspection kit bags) were used to inspect and survey the mosquitoes in the aquatic stages. The sample containers were labeled and stored in a cool box at approximately 25°C. The samples were then transported to the Vector-Borne Disease Control Laboratory for species identification and further processing.
The collected larvae and pupae were reared in the vector control rearing insectary following the Food and Agriculture Organization of the United Nations (FAO) and the International Atomic Energy Agency (IAEA) Guidelines for routine colony maintenance of Aedes mosquito species (16, 17). Mosquitoes caught as adults and adults developed from larvae were used for DENV detection.
A pictorial key to the Aedes (Stegomyia) mosquitoes of East Africa and a pictorial key for identification of subfamilies of Culicidae and genera of Culicidae as well as resources from the WHO were used to identify the Ae aegypti (18–22). Following labelling and coding, female Ae aegypti were placed, five to a tube, in 1.5 mL microcentrifuge tubes and stored at −86°C until further processing. Ae aegypti was the only Aedes species identified during this survey.
In all, 1295 collected mosquitoes were identified as Ae aegypti at the National Center for Vector-Borne Disease (Ministry of Health, Jazan). These were divided into 259 pools (five mosquitoes per pool) (23), preserved in 1.5 mL microcentrifuge tubes, labelled, capped, and stored at −86°C until use. Only female mosquitoes are tested in routine arbovirus surveillance programs (12).
A total RNA isolation kit (Promega, Germany) was used for RNA extraction. Following the manufacturer's instructions, the mosquito samples were homogenized using a mortar and pestle (mini borosilicate glass chamber length: 60 mm, pestle diameter: 9.0 mm, 3.0 mL, Fisherbrand) in 175 μL of RNA lysis solution buffer. Then, 350 μL of RNA dilution buffer was added to 175 μL of the lysate and mixed by inverting three to four times. The mixture was then placed in a heat block for three min at 70°C. After centrifugation at 10,000 rpm for 10 min, the clear lysate was transferred to a new 1.5 mL microcentrifuge tube containing 200 μL of 95% ethanol and mixed by pipetting. The mixture was transferred to a spin column inserted into a collection tube, and centrifuged for 1 min at 10,000 rpm. The collection tube was discarded, and the filter tube was placed in a new collection tube, 600 μL of RNA wash solution was added and then centrifuged for 1 min at 10,000 rpm. Following this, 50 μL of DNase incubation mix (40 μL yellow core buffer, 5 μL 0.09 M MnCl2, and 5 μL DNase I enzyme) was added into the collection tube, incubated for 15 min at 20–25°C, and then 200 μL of DNase stop solution was added and centrifuged for 1 min at 10,000 rpm. The filter tube was then washed twice by adding 450 μL of wash buffer under the same centrifugation conditions, followed by centrifugation for 15 s at 13,000 rpm to remove any residual wash buffer. The high filter tube was then inserted into a nuclease-free, sterile 1.5 mL centrifuge tube, and 100 μL of elution buffer was added to elute the viral RNA by centrifugation at 10,000 rpm for 1 min.
The detection of DENV in collected vectors was performed by RT-PCR (24–29). A one-step RT-PCR-based detection method was performed as described by Lanciotti (26) using D1 and D2 primers synthesized by Integrated DNA Technology (Belgium) (Table 1) to amplify 511 bp of the viral genome. The one-step RT-PCR was performed according to the Access RT-PCR system protocol (Promega, USA). The RT-PCR products were analyzed by gel electrophoresis (1.5% agarose in Tris-Acetate EDTA buffer), stained with ethidium bromide, and visualized using the Gel Doc XR Imaging System (Bio-Rad).
Ethical review and approval were granted by the Institutional Scientific Review Board of Faculty of Public Health and Health Informatics, Umm-Al-Qura University, Makkah, KSA (Centre of Medicine and Medical Science Research) (project #43409019).
The result of Ae aegypti mosquito collection is shown in Figure 1. In total, 1295 female adult Ae aegypti mosquitoes (232 caught as adults and 1063 developed from larvae into adults) were used for DENV detection.
DENV was detected in six of 259 pools (2.3%) (Table 2 and Supplementary 1).
Table 2. Localities with dengue cases, pools tested by RT-PCR and Ae aegypti larval breeding indices reported during the study period.
Four DENV-infected mosquitoes were collected at the aquatic stages and two were collected at the adult stage (Table 2). Results of RT-PCR for DENV are shown in Figure 2.
Figure 2. Agarose gel electrophoresis for the detection of amplified dengue virus RNA in Ae aegypti samples by RT-PCR using D1 and D2 primers. Lane (1) 100 bp DNA marker, lane (2-4) positive mosquito samples, lane (5-7) positive control, lane (8, 9) positive mosquito samples.
Five of the nine target localities (55.5%) yielded positive pools, while the other four yielded negative pools (Table 2 and Supplementary 2).
The number of dengue cases by localities and entomological surveillance data and indices of Ae aegypti shown in Table 2, while the number of dengue cases in relation to positive pools is shown in Figure 3. Both the dengue cases and positive pools peaked in May (Figure 2).
Our results confirm that DENV is prevalent in the Makkah region, and many studies have shown that DENV-2 is the most predominant in suspected dengue cases, followed by DENV-1 and DENV-3 (7, 30–32). Furthermore, we showed the circulation of DENV in adult mosquitoes and offspring, indicating vertical transmission of DENV. Moreover, when considering the distribution of the dengue cases by month, our results showed that the cases and positive pools peaked in May (Figure 3).
Increased air travel has contributed to dengue expansion, providing the means for viremic individuals to travel from one place to another (33–37). After the infection first appeared in Jeddah, continuous surveillance revealed a rapid increase in DF cases in Makkah city and Al-Madinah, pilgrimage sites. This may be due to the availability of favorable breeding sites (e.g., water storage containers and habitats). Furthermore, our study confirmed the transovarial transmission of DENV in Ae aegypti vectors, supporting this well-documented finding in endemic areas worldwide (38). Moreover, this provides information on the distribution and relative abundance of potential vector populations in Makkah city. These factors are essential components in combating mosquito-borne diseases (39–41).
Detection of DENVs in mosquitoes constitutes a reliable strategy for monitoring and evaluating the strategies and interventions currently in use to comprehend the types of viruses circulating in nature and help design vector-specific strategies as indicated elsewhere (23, 41, 42). Moreover, methods for detecting DENV in infected mosquitoes are one of the essential and powerful tools for the early detection of dengue outbreaks (23, 41, 43). Herein, RT-PCR was used for DENV detection. Chow et al. (12) demonstrated that by detecting DENV in adult mosquitoes using RT-PCR, an outbreak could be predicted6 weeks in advance of the occurrence of the first human cases. Further, PCR-based screening could be used to map locations with different levels of endemicity. In combination with the Geographical Information System (GIS), PCR-based screening will provide a practical tool for vector control, enabling the identification of high-risk areas. Further, it improves the ability of epidemiological surveillance systems to anticipate outbreaks and detect silent viruses, as reported by Méndez-Galván et al. (41).
Our study is not without limitations. Sequencing and serotyping of DENV were considered, but the technology was not readily available in our setting. Moreover, due to the method of data collection, we were unable to correlate the positivity of pools in relation to dengue houses vs. neighboring houses, as shown by Urdaneta et al. (23). Nevertheless, we showed the number of dengue cases reported during the study period in relation to tested pools and Ae aegypti entomological indices (Table 2).
In Makkah, DENV is circulating in dengue vectors with a high significance rate, indicating the possibility of a dengue outbreak in the future. This study also confirmed the transovarial transmission of DENV in the Ae aegypti vector. Overall, these results indicate that a sensitive surveillance system is key to predicting dengue outbreaks and facilitating early intervention and control. This information will help the concerned authorities develop guidelines for dengue surveillance programs in Makkah based on sensitive indicators that can promote preparedness, alertness, and early detection of outbreaks.
The raw data supporting the conclusions of this article will be made available by the authors, without undue reservation.
EA, AOB, and AOSB: conceptualization, project supervision, and funding acquisition. EA, AOB, AOSB, and Alhazmi, A.M.F: methodology. EA, SA-M, and MA-L: analysis. EA, AOSB, and SA-M: fieldwork. OD, IA-M, NM, and AA-Z: lab analysis and visualization. EA: writing—original draft preparation and project administration. AOB, AOSB, SA-M, and MA-L: writing, review and editing. All authors contributed to the article and approved the submitted version.
Scientific Research and Revival of Islamic Heritage at Umm Al-Qura University (project # 43409019).
The authors declare that the research was conducted in the absence of any commercial or financial relationships that could be construed as a potential conflict of interest.
All claims expressed in this article are solely those of the authors and do not necessarily represent those of their affiliated organizations, or those of the publisher, the editors and the reviewers. Any product that may be evaluated in this article, or claim that may be made by its manufacturer, is not guaranteed or endorsed by the publisher.
The authors would like to thank Gaber Abudo who helped collect mosquito larvae. We extend our gratitude to the staff and management of the National Center for Vector-Borne Disease (Ministry of Health, Jazan) for making their laboratory available for this study, especially to Dr. Adel Ali Alsheikh and Dr. Wheed Al-Deen Elsideeg for their immense contribution to the success of this work. The authors also wish to express their heartfelt gratitude to the Directorate-General for Health Affairs of Makkah Al-Mokarramah, particularly to the Department of Vector Control, for assistance in procuring reagents and equipment for larval processing and for participating in the fieldwork. We are incredibly grateful to the Department of Vector Control, without whose support, this study would not have been possible. Finally, the authors would like to thank the Institute of Scientific Research and Revival of Islamic Heritage at Umm Al-Qura University (project # 43409019) for financial support. We would like to thank Editage (www.editage.com) for English language editing.
The Supplementary Material for this article can be found online at: https://www.frontiersin.org/articles/10.3389/fpubh.2022.850851/full#supplementary-material
1. World Health Organization (WHO). Dengue Guidelines for Diagnosis, Treatment, Prevention and Control. Geneva, Switzerland: WHO (2009).
2. World Health Organization (WHO). Global Strategy for Dengue Prevention and Control 2012–2020. Geneva, Switzerland: WHO (2012).
3. Getachew D, Tekie H, Gebre-Michael T, Balkew M, Mesfin A. Breeding sites of Aedes aegypti: potential dengue vectors in Dire Dawa, East Ethiopia. Interdiscip Perspect Infect Dis. (2015) 2015:706276-. Epub 2015/09/07. doi: 10.1155/2015/706276
4. Heymann DL. Control of Communicable Diseases Manual: an Official Report of the American Public Health Association. 19 eds. Washington, DC: APHA Press. (2008).
5. Aziz AT, Dieng H, Ahmad AH, Mahyoub JA, Turkistani AM, Mesed H, et al. Household survey of container-breeding mosquitoes and climatic factors influencing the prevalence of Aedes aegypti (Diptera: Culicidae) in Makkah City, Saudi Arabia. Asian Pac J Trop Biomed. (2012) 2:849–57. doi: 10.1016/S2221-1691(12)60242-1
6. Ali EOM, Babalghith AO, Bahathig AOS, Toulah FHS, Bafaraj TG, Al-Mahmoudi SMY, et al. Prevalence of larval breeding sites and seasonal variations of Aedes aegypti mosquitoes (Diptera: Culicidae) in Makkah Al-Mokarramah, Saudi Arabia. Int J Environ Res Public Health. (2021) 18:7368. doi: 10.3390/ijerph18147368
7. Fakeeh M, Zaki A. Dengue in Jeddah, Saudi Arabia, 1994-2002. Dengue Bull. (2003) 27:13–8. Available online at: https://apps.who.int/iris/handle/10665/163900
8. Ministry of Health (Saudi Arabia). Saudi Arabia Health Statistical Yearbook 2006. Riyadh: Ministry of Health (2006).
9. Melebari S, Bakri R, Hafiz A, Qabbani F, Khogeer A, Alharthi I, et al. The Epidemiology and incidence of dengue in Makkah, Saudi Arabia, during 2017-2019. Saudi Med J. (2021) 42:1173–9. doi: 10.15537/smj.2021.42.11.20210124
10. Kyle JL, Harris E. Global spread and persistence of dengue. Annu Rev Microbiol. (2008) 62:71–92. doi: 10.1146/annurev.micro.62.081307.163005
11. Aziz AT, Al-Shami SA, Mahyoub JA, Hatabbi M, Ahmad AH, Rawi CSM. An update on the incidence of dengue gaining strength in Saudi Arabia and current control approaches for its vector mosquito. Parasitvectors. (2014) 7:258-. doi: 10.1186/1756-3305-7-258
12. Chow V, Chan YC, Yong R, Lee KM, Lim LK, Chung YK, et al. Monitoring of dengue viruses in field-caught Aedes aegypti and Aedes albopictus mosquitoes by a type-specific polymerase chain reaction and cycle sequencing. Am J Trop Med Hyg. (1998) 58:578–86. doi: 10.4269/ajtmh.1998.58.578
13. Achee NL, Gould F, Perkins TA, Reiner RC. Jr., Morrison AC, Ritchie SA, et al. A critical assessment of vector control for dengue prevention. PLOS Negl Trop Dis. (2015) 9:e0003655. doi: 10.1371/journal.pntd.0003655
14. Sanchez-Casas RM, Alpuche-Delgado RH, Blitvich BJ, Diaz-Gonzalez EE, Ramirez-Jimenez R, Zarate-Nahon EA, et al. Detection of dengue virus serotype 2 in Aedes aegypti in Quintana Roo, Mexico, 2011. Southwestern Entomol. (2013) 38:109-17, 9. doi: 10.3958/059.038.0115
15. Haninah AU, Vasan SS, Ravindran T, Chandru A, Lee HL, Devi SS. Development and evaluation of a one-step SYBR-Green I-based real-time RT-PCR assay for the detection and quantification of Chikungunya virus in human, monkey and mosquito samples. Trop Biomed. (2010) 27:611–23.
16. Puggioli A, Balestrino F, Damiens D, Lees RS, Soliban SM, Madakacherry O, et al. Efficiency of three diets for larval development in mass rearing aedes albopictus (Diptera: Culicidae). J Med Entomol. (2013) 50:819–25. doi: 10.1603/ME13011
17. The Food and Agriculture Organization of the United Nations (FAO), The International Atomic Energy Agency (IAEA). Guidelines for Routine Colony Maintenance of Aedes Mosquito Species - Version 10; FAO: Rome, Italy; IAEA: Vienna, Austria. (2017) Availableonline at: https://www.iaea.org/resources/manual/guidelines-for-routine-colony-maintenance-of-aedes-mosquito-species (accessed February 24, 2021).
18. Harbach RE. Mosquito Taxonomic Inventory (M.T.I.). Available online at: http://mosquito-taxonomic-inventory.info/ (accessed February 24, 2021).
19. Huang YM, A. Pictorial Key for the identification of the subfamilies of Culicidae, Genera of Culicinae, and subgenera of Aedes mosquitoes of the afrotropical region (Diptera: Culicidae). Proceedings- Entomological Society of Washington. (2001) 103:1–53.
20. Rueda L. Pictorial keys for the identification of mosquitoes (Diptera: Culicidae) associated with dengue virus transmission. Zootaxa. (2004) 589. doi: 10.11646/zootaxa.589.1.1
21. Gerberg EJ, van Someren ECC. Pictorial Key to the Mosquitoes Aedes (Stegomyia) of East Africa. Geneva, Switzerland: WHO. (1970).
22. Huang YM. A pictorial key to the mosquito genera of the world, including subgenera of Aedes and Ochlerotatus (Diptera: Culicidae). Insecta Koreana. (2002) 19:1–130.
23. Urdaneta L, Herrera F, Pernalete M, Zoghbi N, Rubio-Palis Y, Barrios R, et al. Detection of dengue viruses in field-caught Aedes aegypti (Diptera: Culicidae) in Maracay, Aragua State, Venezuela by type-specific polymerase chain reaction. Infect Genet Evol. (2005) 5:177–84. doi: 10.1016/j.meegid.2004.09.004
24. Balm M, Lee C, Lee H, Chiu L, Koay ES, Tang J, et al. Diagnostic polymerase chain reaction assay for zika virus. J Med Virol. (2012) 84:1501–5. doi: 10.1002/jmv.23241
25. Chen C-F, Shu P-Y, Teng H-J, Su C-L, Wu J-W, Wang J-H, et al. Screening of dengue virus in field-caught Aedes aegypti and Aedes albopictus (Diptera: Culicidae) by one-step SYBR green-based reverse transcriptase-polymerase chain reaction assay during 2004–2007 in Southern Taiwan. Vector Borne Zoonotic Dis. (2010) 10:1017–25. doi: 10.1089/vbz.2008.0069
26. Lanciotti RS, Calisher C, Gubler D, Chang G-J, Vorndam AV. Rapid detection and typing of dengue viruses from clinical samples by using reverse transcriptase-polymerase chain reaction. J Clin Microbiol. (1992) 30:545–51. doi: 10.1128/jcm.30.3.545-551.1992
27. Laurent P, Roux K, Grivard P, Bertil G, Naze F, Picard M, et al. Development of a sensitive real-time reverse transcriptase PCR assay with an internal control to detect and quantify chikungunya virus. Clin Chem. (2007) 53:1408–14. doi: 10.1373/clinchem.2007.086595
28. Chien L-J, Liao T-L, Shu P-Y, Huang J-H, Gubler D, Chang G-J. Development of real-time reverse transcriptase PCR assays to detect and serotype dengue viruses. J Clin Microbiol. (2006) 44:1295–304. doi: 10.1128/JCM.44.4.1295-1304.2006
29. Hanlim L, Ahmad R, Pahang J. Transovarial transmission of dengue virus in Aedes aegypti and Aedes albopictus in relation to dengue outbreak in an urban area in Malaysia. Dengue Bull. (2005) 29.
30. Ahmad R, Ismail A, Saat Z, Lim L. Detection of dengue virus from field Aedes aegypti and Aedes albopictus adults and larvae. Southeast Asian J Trop Med Public Health. (1997) 28:138–42.
31. Azhar E, Kao M, Niedrig M, Masri B, Godus A, Badierah R, et al. Virological diagnosis of dengue fever in Jeddah, Saudi Arabia: comparison between RT-PCR and virus isolation in cell culture. J Inf Dis Immunity. (2010) 2.
32. Ashshi A. Serodetection of dengue virus and its antibodies among blood donors in the western region of Saudi Arabia: a preliminary study. Blood Transfus. (2014) 13:1–4. doi: 10.2450/2014.0134-14
33. Bhatt S, Gething P, Brady O, Messina J, Farlow A, Moyes C, et al. the global distribution and burden of dengue. Nature. (2013) 496:504–7. doi: 10.1038/nature12060
34. [Dataset] Brady O, Gething P, Bhatt S, Messina J, Brownstein J, Hoen A, et al. refining the global spatial limits of dengue virus transmission by evidence-based consensus. - PLoS ONE. (2012):8-10. doi: 10.1371/journal.pntd.0001760
35. Chan YYG KT, Heng BH, Tan BH. Recent dengue outbreaks in Singapore. In: Sim TS, Singh, M, editor. Microbiology in the Ninetie. Singapore: World Scientific and Global Publications (1994).
36. Norman G, Theodre A, Joseph A. An insular outbreak of dengue fever in a rural south Indian village. J Commun Dis. (1991) 23:185–90.
37. Getis A, Morrison A, Gray K, Scott T. characteristics of the spatial pattern of the dengue vector, Aedes aegypti, in Iquitos, Peru. Am J Trop Med Hyg. (2003) 69:494–505. doi: 10.4269/ajtmh.2003.69.494
38. Rohani A, Zamree I, Lee HL, Mustafakamal I, Norjaiza MJ, Kamilan D. Detection of transovarial dengue virus from field-caught Aedes aegypti and Ae. Albopictus Larvae Using C6/36 Cell Culture and Reverse Transcriptase-Polymerase Chain Reaction (RT-PCR) Techniques. Dengue Bull. (2007) 31:47–57.
39. Aziz A, Dieng H, Hassan A, Mahyoub J, Mesed H, Koshike S, et al. Temporal variations on abundance, blood feeding activity of Aedes aegypti (Diptera: Culicidae) and dengue incidence in Makkah city, Saudi Arabia Ministry of Municipal, kingdom of Saudi Arabia. Asian Pac J Trop Biomed (2012):1.
40. European Centre for Disease Prevention and Control. Technical Report Guidelines for the Surveillance of Invasive Mosquitoes in Europe. Available online at: https://www.ecdc.europa.eu/en (acessed February 27, 2021).
41. Méndez-Galván J, Sánchez-Casas RM, Gaitan-Burns A, Díaz-González EE, Ibarra-Juarez LA., Garza CEMdl, et al. Detection of Aedes aegypti mosquitoes infected with dengue virus as a complementary method for increasing the sensitivity of surveillance: identification of serotypes 1, 2, and 4 by RT-PCR in Quintana Roo, Mexico. Southwestern Entomol. (2014) 39:307–16. doi: 10.3958/059.039.0208
42. Samuel PP, Tyagi BK. Diagnostic methods for detection & isolation of dengue viruses from vector mosquitoes. Indian J Med Res. (2006) 123:615–28.
Keywords: larval ecology, Makkah, dengue fever, dengue virus, vectors, Aedes
Citation: Ali EOM, Babalghith AO, Bahathig AOS, Dafalla OM, Al-Maghamsi IW, Mustafa NEAG, AL-Zahrani AAA, Al-Mahmoudi SMY and Abdel-Latif ME (2022) Detection of Dengue Virus From Aedes aegypti (Diptera, Culicidae) in Field-Caught Samples From Makkah Al-Mokarramah, Kingdom of Saudi Arabia, Using RT-PCR. Front. Public Health 10:850851. doi: 10.3389/fpubh.2022.850851
Received: 08 January 2022; Accepted: 08 April 2022;
Published: 09 June 2022.
Edited by:
Sunil Dhiman, Defence Research & Development Establishment (DRDE), IndiaReviewed by:
Rina Tilak, Armed Forces Medical College, IndiaCopyright © 2022 Ali, Babalghith, Bahathig, Dafalla, Al-Maghamsi, Mustafa, AL-Zahrani, Al-Mahmoudi and Abdel-Latif. This is an open-access article distributed under the terms of the Creative Commons Attribution License (CC BY). The use, distribution or reproduction in other forums is permitted, provided the original author(s) and the copyright owner(s) are credited and that the original publication in this journal is cited, in accordance with accepted academic practice. No use, distribution or reproduction is permitted which does not comply with these terms.
*Correspondence: Elfadol Obeid Mohamed Ali, ZmFkb2xvYmVpZEB5YWhvby5jb20=
Disclaimer: All claims expressed in this article are solely those of the authors and do not necessarily represent those of their affiliated organizations, or those of the publisher, the editors and the reviewers. Any product that may be evaluated in this article or claim that may be made by its manufacturer is not guaranteed or endorsed by the publisher.
Research integrity at Frontiers
Learn more about the work of our research integrity team to safeguard the quality of each article we publish.