- 1Department of Epidemiology and Biostatistics, School of Public Health, Anhui Medical University, Hefei, China
- 2Department of General Medicine, The First Affiliated Hospital of Anhui Medical University, Hefei, China
- 3Teaching Center for Preventive Medicine, School of Public Health, Anhui Medical University, Anhui, China
Objective: Emerging evidence has highlighted the possible links of environmental pollution with several cardiovascular diseases (CVDs). The current study aimed to explore the impact of short-term air pollution exposure on CHD hospitalization in Hefei.
Methods: Data about the daily number of CHD admissions (from 2014 to 2021) were retrieved from the First Affiliated Hospital of Anhui Medical University. Air pollutants and meteorological data were obtained from the China Environmental Monitoring Station and the China Meteorological Data Service Center, respectively. The correlation between air pollution and CHD hospitalization was assessed using distributed lag non-linear model (DLNM) and Poisson generalized linear regression.
Results: In the single-pollutant model, NO2, O3, and CO strongly correlated with CHD hospitalization rate. Specifically, exposure to NO2 (lag0, relative risk [RR]: 1.013, 95%CI: 1.002–1.024, per 10 μg/m3 increase) and CO (lag13, RR: 1.035, 95%CI: 1.001–1.071, per 1 μg/m3 increase) revealed a positive correlation with an increased rate of CHD hospitalization. Interestingly, O3 had a protective association with hospitalization of CHD (lag0, RR: 0.993, 95%CI: 0.988–0.999, per 10 μg/m3 increase). Similar results, to those of the single-pollutant model, were revealed following verification using two-pollutant models. Subgroup analyses indicated that young people, women, and people in hot seasons were more susceptible to NO2 exposure, while the elderly, women, and people in cold seasons were more susceptible to O3. Furthermore, the elderly were more susceptible to CO exposure.
Conclusion: Overall, exposure to NO2 and CO increases the rate of CHD hospitalization, but exposure to O3 shows a protective association with the rate of CHD hospitalization. Therefore, early preventive measures against air pollutants should be applied to protect vulnerable patients with CHD.
1. Introduction
Coronary heart disease (CHD) is a chronic heart disease that can cause myocardial ischemia, hypoxia, or necrosis and is usually caused by coronary atherosclerosis. As a typical cardiovascular disease (CVD), CHD is the leading cause of death worldwide (1). The American Heart Association (AHA) suggests that the global number of patients with CHD is likely to double by the year 2030 (2). In terms of prevalence, CHD ranks second among the world's leading causes of death. In China, the disease has been linked to the rising trend of death in the past two decades, from 1980 to 2016, when the average annual growth rate was 9.85%. Based on all these, it is clear that CHD is an important public health issue that negatively impacts people's health and quality of life.
Over the past two decades, a lot of research has profiled arterial hypertension, dyslipidemia, and smoking as risk factors associated with the increased incidence of CHD and related mortality (3). Lifestyle and diet patterns, as common influencing factors of chronic diseases, are related to the risk of CHD. In addition, temperature, relative humidity, barometric pressure, other meteorological factors, and air pollutants are also related to the risk of CHD (4–6). It is important to note that air pollution is harmful to human health in many ways. A growing number of studies have demonstrated that short-term exposure to air pollutants can cause acute and habitual damage to the CV and respiratory and immune systems (7–9). Complex components among air pollutants can induce systemic inflammation and disturb the balance of oxidative stress and the autonomic nervous system. This may cause injury to the CV system and promote the development of atherosclerosis (10, 11), eventually causing the onset and progression of CHD or stroke.
To date, a growing number of studies have shown that short-term exposure to air pollution is related to the rate of CHD (12–15). Nevertheless, most of these studies were conducted in developed countries, where climate conditions and the level of air pollution differ, in many aspects, from those in developing countries. First, comparative studies on climate characteristics and terrain conditions show that prevention and control of air pollution are more difficult in developing countries than in developed countries such as the United States and other European countries. Influenced by the topography of the Qinghai-Tibet Plateau, the Eastern part of China has a significant downdraft in winter, and the atmospheric stratification becomes more stable with less precipitation, which is not conducive to the diffusion of pollutants and wet removal. Second, global warming causes an interdecadal weakening of the East Asian winter monsoon. The average surface wind speed decreases, which is more conducive to the accumulation of pollutants. More importantly, meteorological conditions affect the transmission and diffusion of pollutants, chemical transformation, dry and wet deposition, and other processes. Even within the same country, differences in climate type and geographical location may also lead to differences in the role of atmospheric pollutants. Regardless of these, previous studies carried out in China have mainly reported on the association between PM and hospitalization of CHD (16–18), ignoring research on other pollutants. Therefore, in the present study, we carried out a time-series analysis to explore the relationship between air pollutants and CHD hospitalization in Hefei, and to identify the possible vulnerable sub-populations and meteorological factors. In addition, we employed the DLNM model, which is a modeling framework to flexibly estimate an exposure–time–response function. This modeling framework considers both the lagged effect of exposure factors and the exposure–response non-linear relationship.
2. Materials and methods
2.1. Study area
This study was carried out in Hefei, Anhui Province (31.87°N, 117°17E), which is among the central cities of the Yangtze River Delta region. Hefei is located in the middle latitude zone, and it is characterized by the sub-tropical monsoon humid climate conditions: obvious monsoon, distinct four seasons, relatively mild climate, and moderate annual rainfall (Figure 1).
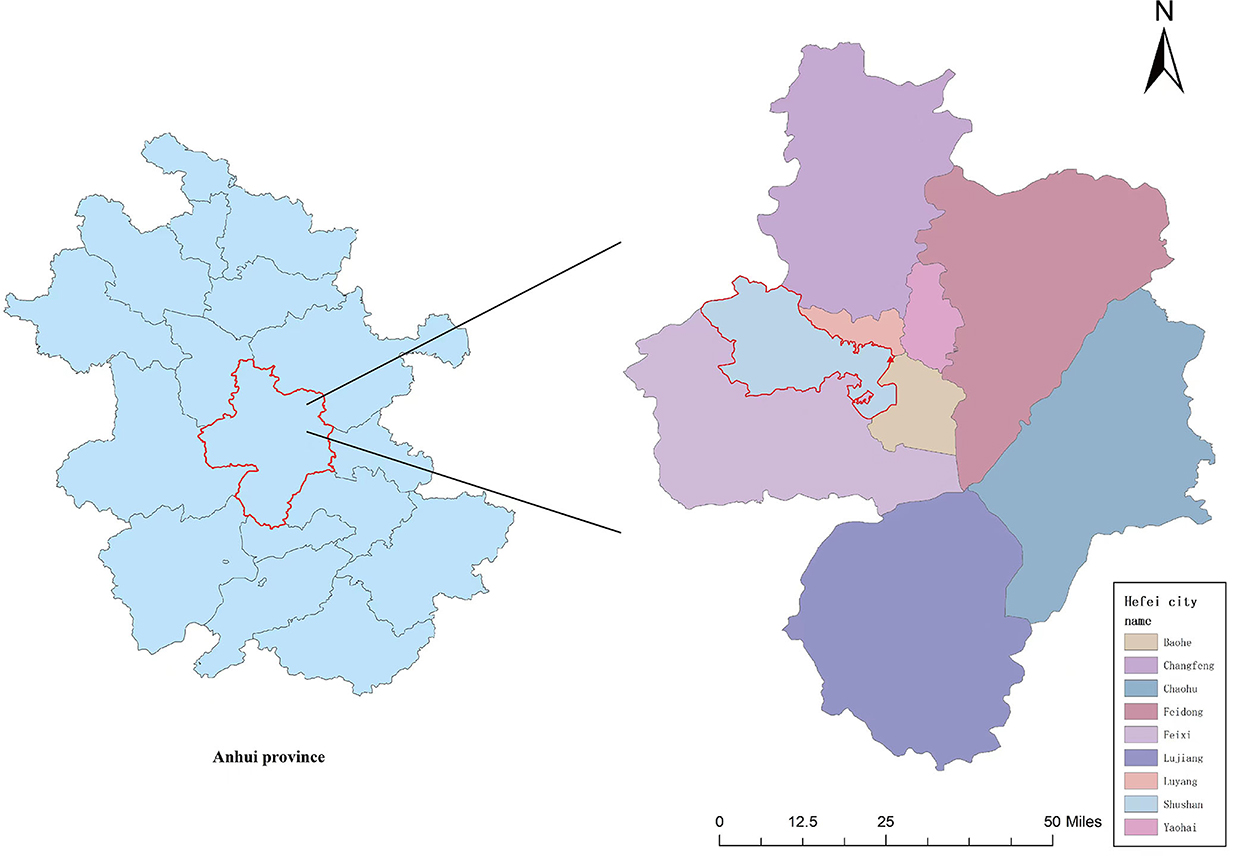
Figure 1. A map of the sample city and hospital (the city circled in red is Hefei, the city we studied, and the triangle represents the location of the hospital).
2.2. Data sources
The inpatient records of CHD hospitalization were obtained from the First Affiliated Hospital of Anhui Medical University, between 1 January 2014 to 31 December 2021. The First Affiliated Hospital of Anhui Medical University is a grade-A tertiary hospital, with a bed capacity of about 5,000 beds. As the largest hospital in Hefei, the hospital's inpatient data were representative. A total of 16,656 patients were enrolled after which 10,407 patients were eliminated following the data-cleaning process. The data contained variables such as age, sex, date of hospitalization, and residential address. At the same time, to ensure that the included patients were all local, with complete basic information, patients whose permanent addresses were not in Hefei or lacked demographic information (such as age or gender) were cast aside. All admissions were diagnosed according to the definition of CHD in the 10th edition of the International Classification of Diseases (ICD-10: I25.1) (19). The Ethics Committee of Anhui Medical University approved this research.
2.3. Air pollutants and meteorological data
Air pollution data (NO2, CO, SO2, PM2.5, PM10, and O3 8 h) from 2014 to 2021 were obtained from China's Environmental Monitoring stations. For each pollutant, we obtained the average concentration of 10 state-controlled air quality monitoring stations in Hefei. The daily data on relative humidity (RH) (%) and average temperature (MT) (°C), during the research period, were collected by the China Meteorological Data Service Center (http://data.cma.cn/).
2.4. Statistical analysis
The spearman analysis and the depicted scatter plot were used to determine the correlation coefficients between air pollutants, meteorological factors, and the number of CHD admissions. A distributed lag non-linear model (DLNM) was used to test the relationship between air pollution exposure and CHD hospitalization rates (20). Based on the exposure–response relationship, DLNM increases the lag effect because it considers both the lagged effect of exposure factors and the non-linear relationship of the exposure–response (21). Since CHD hospitalization is a probability event, we used the quasi-Poisson distribution generalized linear model (GLM) to explore the association between air pollutants and daily CHD hospitalization (22). In addition, this study brought the pollutants into the single pollutant model to explore the exposure–response relationship and the lag–response relationship. Since there may be possible interactions between various pollutants, the statistical correlations between pollutants were also conducted. To avoid multi-collinearity, a Spearman correlation coefficient of < 0.7 was selected as a covariate. Furthermore, to effectively control the effects of meteorological factors, long-running trends, the weekday effect, the holiday effect, and other potential confounding factors, these variables were incorporated into the control model. The quasi-Poisson Akaike Information Criterion (Q-AIC) was applied to evaluate the goodness of fit of the model and determine the lag time and degree of freedom (df). Finally, the time series model was constructed as below:
Yt quasi-Poisson (μt)
where t is the observation time (day), μt is the number of patients with CHD hospitalized on day t, α is the model's intercept, Xt, l is the cross-basis matrix of the DLNM model, l is the lag day, β is the vector of Xt, and ns (RH,3) is the natural cubic spline. In this study, a natural cubic spline of 7 dfs/year was applied to regulate seasonal and long-running trends (23). DOW is the dummy variable of a day of the week. We applied the binary variable Holidayt to regulate the holiday effect.
Further subgroup analyses were performed by age (<65 vs. ≥65 years) and gender (male vs. female) to identify the underlying vulnerable population. Moreover, the relationship between air pollution and CHD hospitalization throughout the hot season (April–September) and the cold season (October–March) was investigated.
All the statistical analyses and visualization, including “DLNM” and “Spline” packages, were performed using R software version 3.6.1 (http://www.R-project.org). P < 0.05 (bilateral) was considered statistically significant in statistical tests.
2.5. Sensitivity analysis
Based on the original single-pollutant model, we considered coexisting pollutants and built the two-pollutant model to evaluate the stability of the single-pollutant model developed in this study. In addition, to test the robustness of the model, sensitivity analysis was conducted by changing the dfs in the ns functions of air pollutants (3–5 dfs), meteorological variables (3–5 dfs), and time (6–8 dfs per year).
3. Results
3.1. Descriptive analysis
This study included 16,656 CHD cases from 1 January 2014 to 31 December 2021. A majority of the cases were male patients, at 9,967 (against 6,689 female patients), and people aged ≥65 years, at 11,190 (against 5,466 people aged <65 years). In addition, the proportion of CHD cases in the hot and cold seasons was 50.49 and 49.51%, respectively. The basic characteristics of patients with CHD and the data for meteorological factors and environmental pollutants are shown in Table 1.
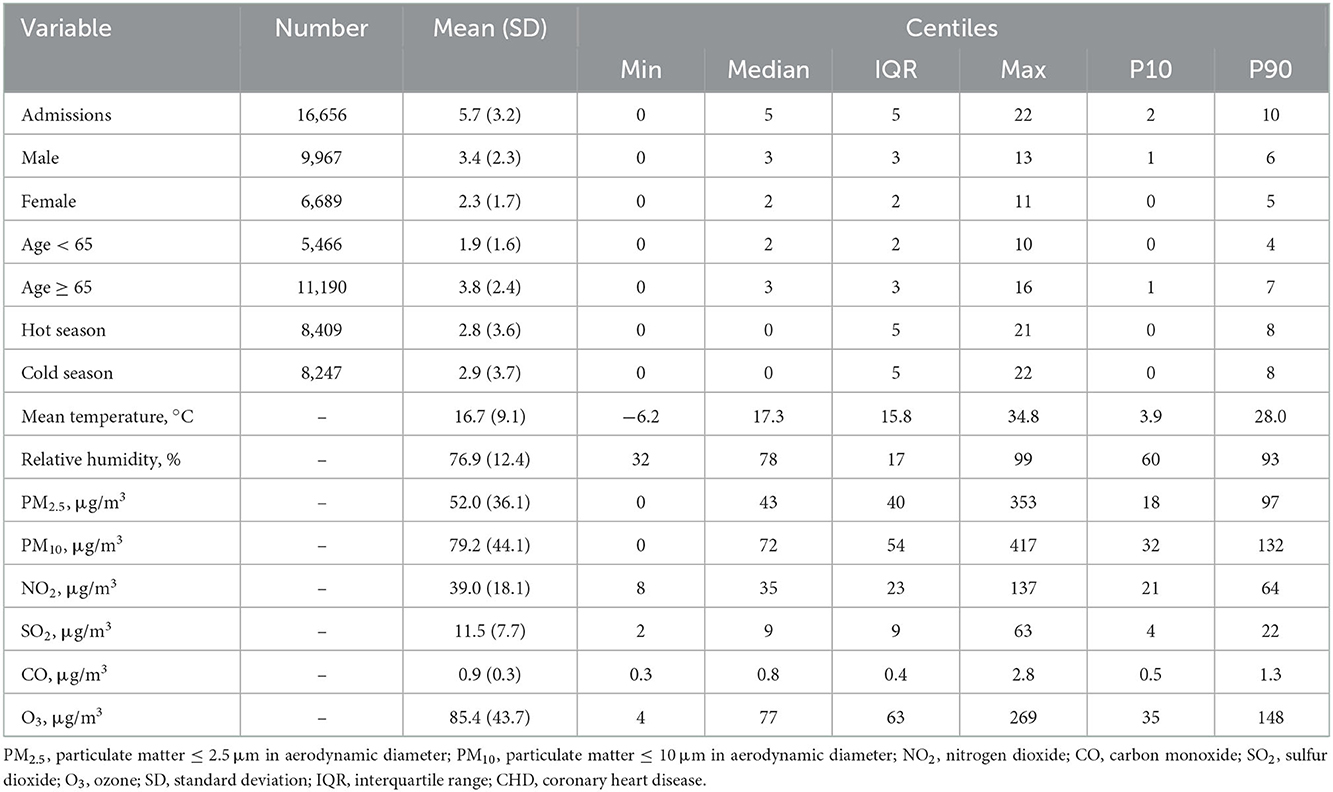
Table 1. Summary statistics of air pollutants, meteorological factors, and outpatients for CHD in Hefei, 2014–2021.
The daily average concentration of NO2 was 39.0 μg/m3 (8–137 μg/m3), PM2.5 was 52.0 μg/m3 (0–353 μg/m3), SO2 was 11.5 μg/m3 (2–63 μg/m3), O3 was 85.4 μg/m3 (4–269 μg/m3), and CO was 0.9 μg/m3 (0.3–2.8 ug/m3). The number of CHD hospitalizations per day, during the study, ranged from 0 to 22 (Figure 2). The time series distribution of air pollutants in Hefei is displayed in Figure 3, whereas, the Spearman rank correlation analysis and scatter diagram are shown in Supplementary Figure S1.
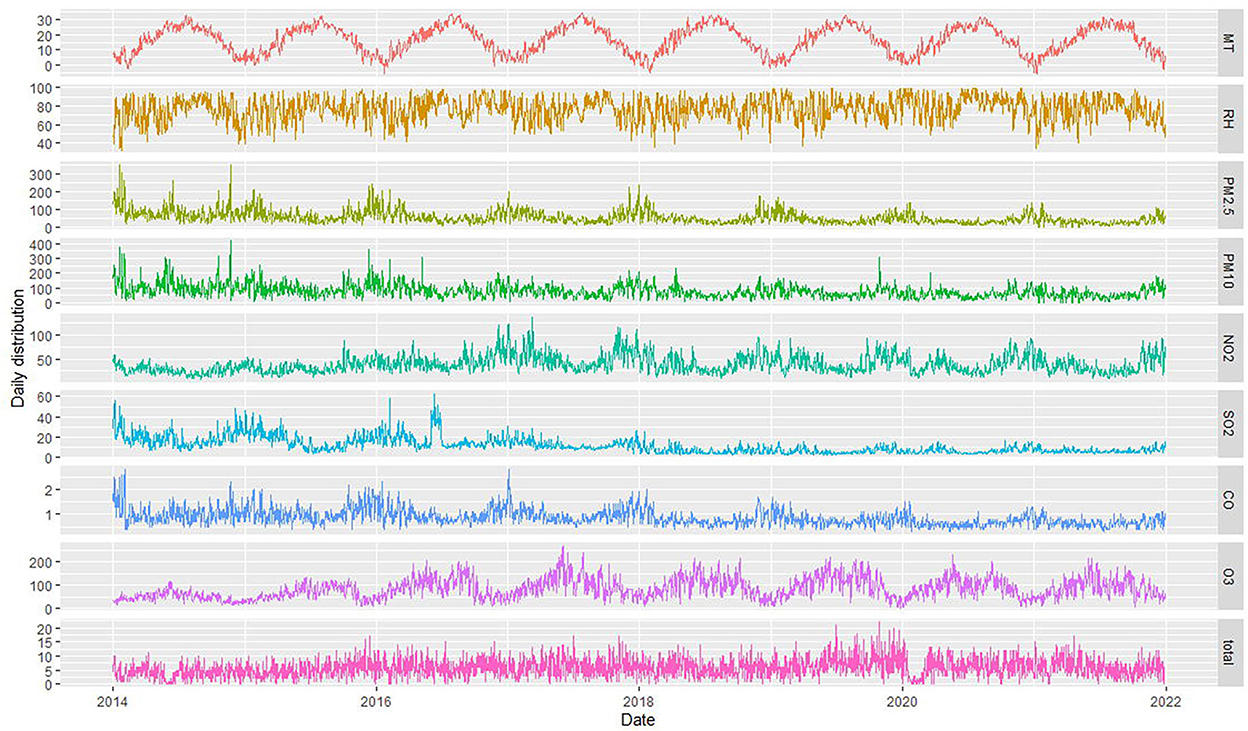
Figure 3. Time series of air pollutants, meteorological factors, and CHD patient hospitalizations in Hefei from 2014 to 2021 (MT represents mean temperature, RH represents relative humidity, and total represents the total number of CHD hospitalization).
3.2. The association between air pollution exposure and CHD hospitalization
The exposure–response relationships between exposure to air pollutants (NO2, CO, and O3) with different lag days and CHD admissions are shown in Figures 4A–C. The high concentrations of NO2 and CO exposures showed a strong correlation with CHD hospitalization (reference concentration 35 and 0.8 μg/m3, respectively). On the other hand, the elevation of O3 levels revealed a negative correlation with CHD hospitalization (reference concentration 77 μg/m3). The concentration–response relationship among NO2, CO, O3, and CHD admissions is shown in Supplementary Figure S2. Accordingly, to illustrate the association between air pollutant exposure and the rate of CHD hospitalization, we analyzed the specific lag effect of the rate of CHD hospitalization.
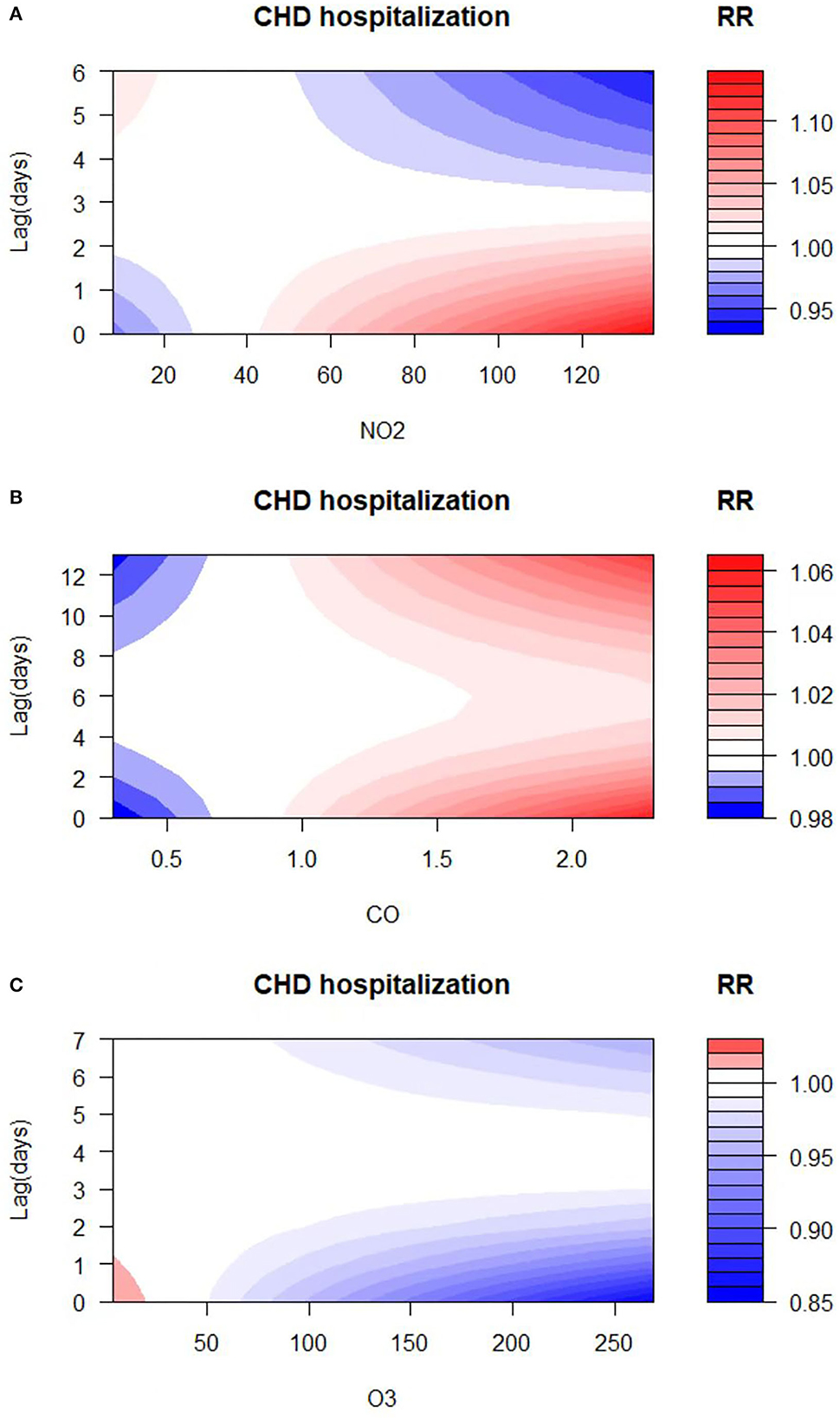
Figure 4. Relative risk (RR) contour plots for CHD hospitalization during the lag periods of NO2 (A), CO (B), and O3 (C) in Hefei, China, 2014–2021.
3.3. The association between NO2 exposure and CHD hospitalization
Results of the association between NO2 exposure and CHD hospitalization revealed a strong correlation between NO2 and the rate of CHD hospitalization in the single-pollutant model (lag0, RR: 1.013, 95%CI: 1.002–1.024; and lag1, RR: 1.007, 95%CI: 1.002–1.013, per 10 μg/m3 increase in NO2 concentration) (Figure 5A). The results of the exposure–response relationship and the lag–response relationship of NO2 are shown in Supplementary Table S1. Subgroup analyses showed that the association between NO2 exposure and the rate of CHD hospitalization increased in female patients (RR: 1.022, 95%CI: 1.005–1.040, lag0) and patients aged < 65 years (RR: 1.037, 95% CI: 1.006–1.069, lag3), while there was no statistical significance between NO2 exposure and the rate of CHD hospitalization among male patients (RR: 1.007, 95% CI: 0.993–1.021, lag0) and patients aged ≥65 years (RR: 1.016, 95% CI: 0.995–1.038, lag3). Moreover, exposure to NO2 during hot seasons was related to an increased rate of CHD hospitalization (RR: 1.037, 95%CI: 1.006–1.069, lag3). Interestingly, the relationship between exposure to NO2 during hot seasons and increased rate of CHD hospitalization was not statistically significant in the cold season (RR: 1.006, 95% CI: 0.988–1.024, lag3) (Figure 6A; Supplementary Table S2).
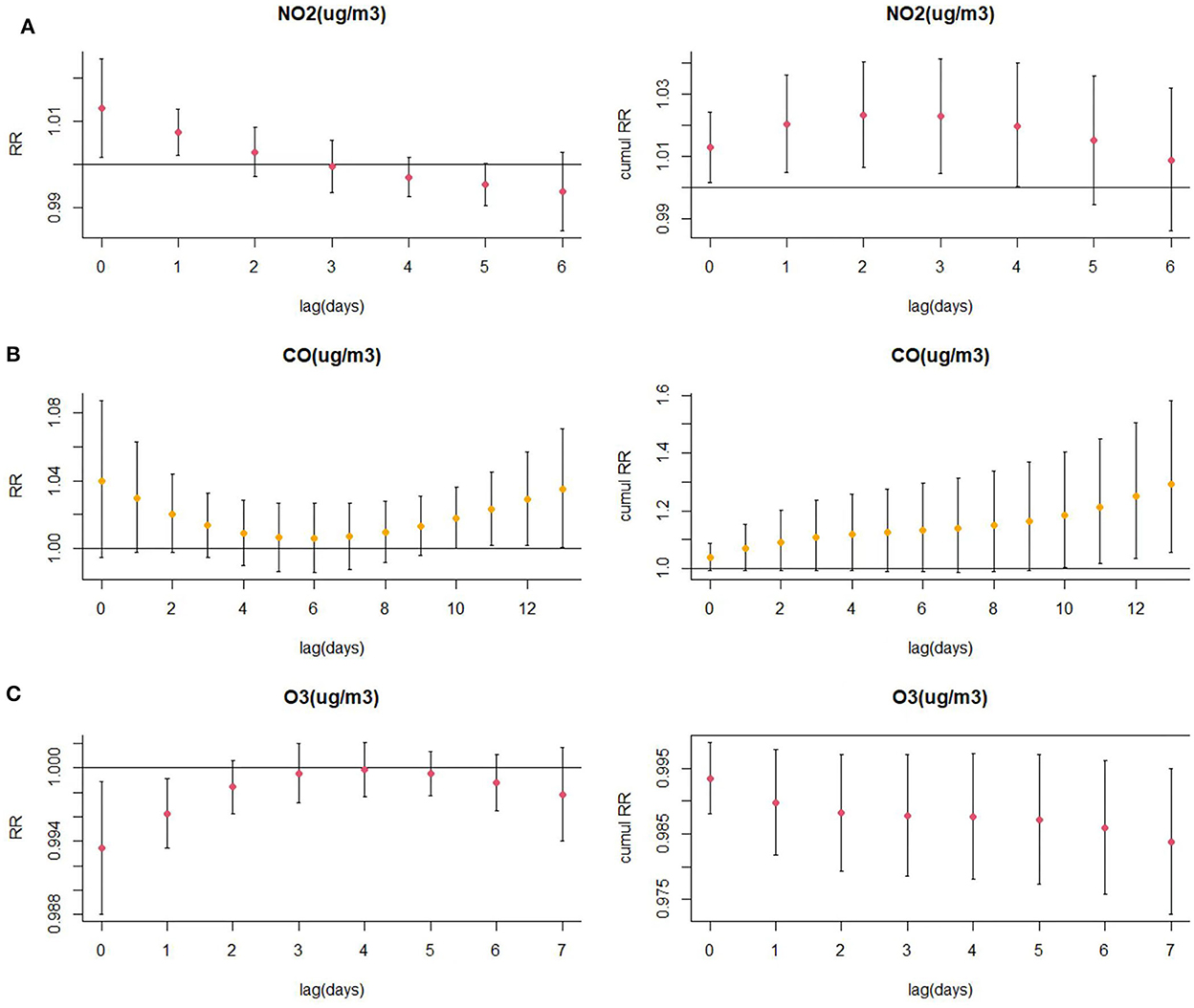
Figure 5. (A) Lagged specific relative risk (%) and (B, C) cumulative risk (%) of CHD hospitalization for each 10 (or 1) unit increase in daily average air pollution concentration in the model.
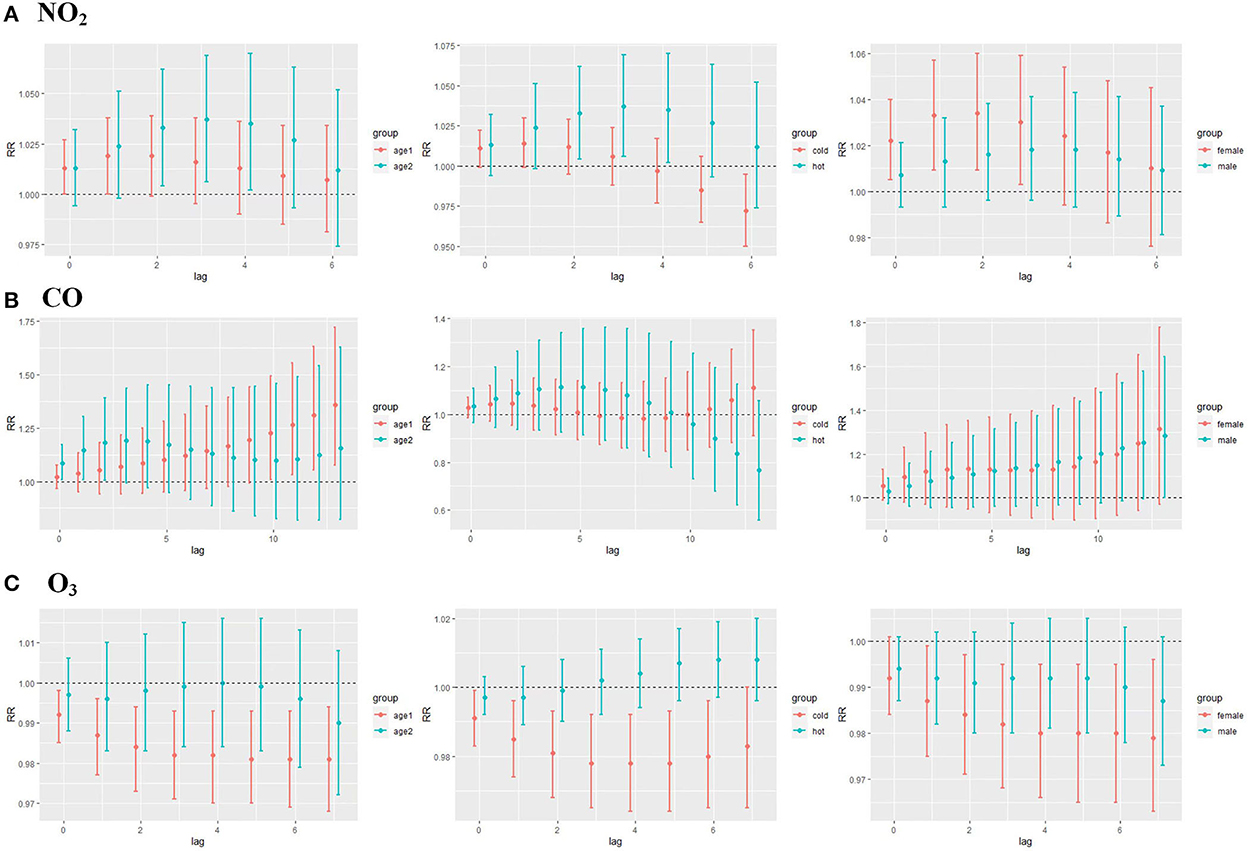
Figure 6. (A) CHD lag-specific relative risks (95% CI) per 10 unit increase in the daily concentrations of NO2 in models stratified by age, gender, and season; (B) CHD lag-specific relative risks (95% CI) per 1 unit increase in the daily concentrations of CO in models stratified by age, gender, and season; (C) CHD lag-specific relative risks (95% CI) per 10 unit increase in the daily concentrations of O3 in models stratified by age, gender, and season (age1 refers to people ≥65 years of age and age2 refers to people <65 years of age, cold season is from October to March, and the hot season is from April to September).
3.4. The association between CO exposure and CHD hospitalization
As displayed in Figure 5B, there was a strong correlation between CO and CHD hospitalization rate in the single-pollutant model (lag13, RR: 1.035, 95%CI: 1.001–1.071, per 1 μg/m3 increase in CO concentration). We further explored the exposure–response relationship and the lag–response relationship of CO (Supplementary Table S3). Subgroup analyses indicated that CO exposure positively correlated with the rate of CHD hospitalization among patients aged above 65 years (RR: 1.360, 95%CI: 1.075–1.722, lag13). Yet, we found no association among CO exposure and other subgroups such as male patients (RR: 1.283, 95%CI: 1.000–1.246, lag13), female patients (RR: 1.315, 95%CI: 0.971–1.780, lag13), people < 65 years of age (RR: 1.156, 95%CI: 0.822–1.627, lag13), cold seasons (RR: 1.111, 95%CI: 0.911–1.353, lag13), and hot seasons (RR: 0.767, 95%CI: 0.558–1.056, lag13) (Figure 6B; Supplementary Table S4).
3.5. The association between O3 exposure and CHD hospitalization
Results of the single pollutant model showed that O3 correlated with the rate of CHD hospitalization (lag0, RR: 0.993, 95%CI: 0.988–0.999; and lag1, RR: 0.996, 95%CI: 0.993–0.999, per 10 μg/m3 increase in O3 concentration) (Figure 5C). We further explored the exposure–response relationship and the lag–response relationship of O3 and the results are presented in Supplementary Table S5. Further subgroup analyses revealed that O3 exposure negatively correlated with the rate of CHD hospitalization in subgroups of female patients (RR: 0.979, 95%CI: 0.963–0.996, lag7), people ≥65 years of age (RR: 0.981, 95%CI: 0.968–0.994, lag7), and during the cold season (RR: 0.978, 95%CI: 0.964–0.992, lag4). On the other hand, O3 exposure and the rate of CHD hospitalization among male patients (RR: 0.987, 95%CI: 0.973–1.001, lag7), people < 65 years of age (RR: 0.990, 95%CI: 0.972–1.008, lag7), and in hot seasons (RR: 1.004, 95%CI: 0.994–1.014, lag7) were not statistically significant (Figure 6C; Supplementary Table S6).
3.6. Sensitivity analysis
Considering that various pollutants may have collinearity with each other when a single pollutant model is used to assess the effect of a pollutant on the quantity of CHD hospitalization, another pollutant was included as an independent variable to explore the association between other coexisting pollutants and CHD hospitalization. The results of fitting the two-pollutant model showed that the results were unchanged (Tables 2–4). We also evaluated the stability of the model by varying degrees of freedom (4–6 dfs/year) and meteorological factors (4–6 dfs). The results demonstrated that the model fits well and produced reliable results (Supplementary Figures S3–S5).
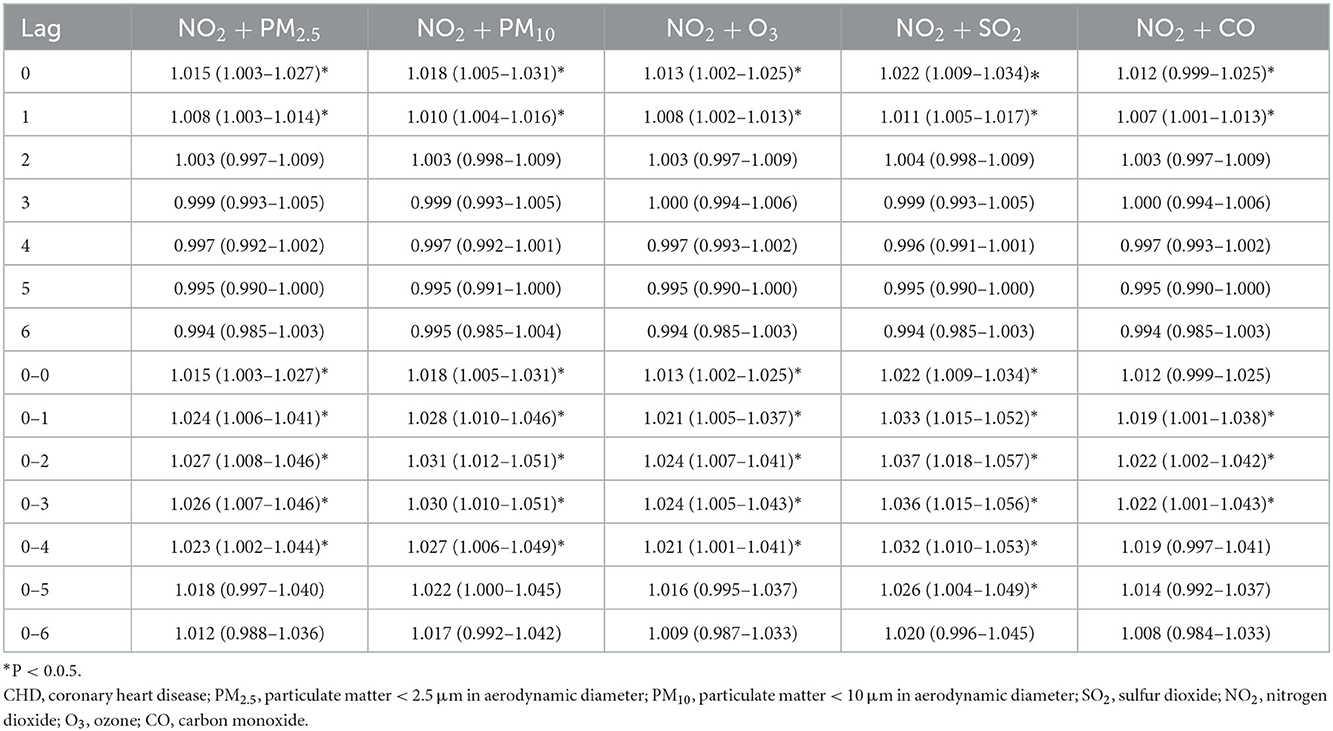
Table 2. The association between an increase of 10 μg/m3 of NO2 and CHD admission in Hefei, 2014–2021 (dual pollutant model).
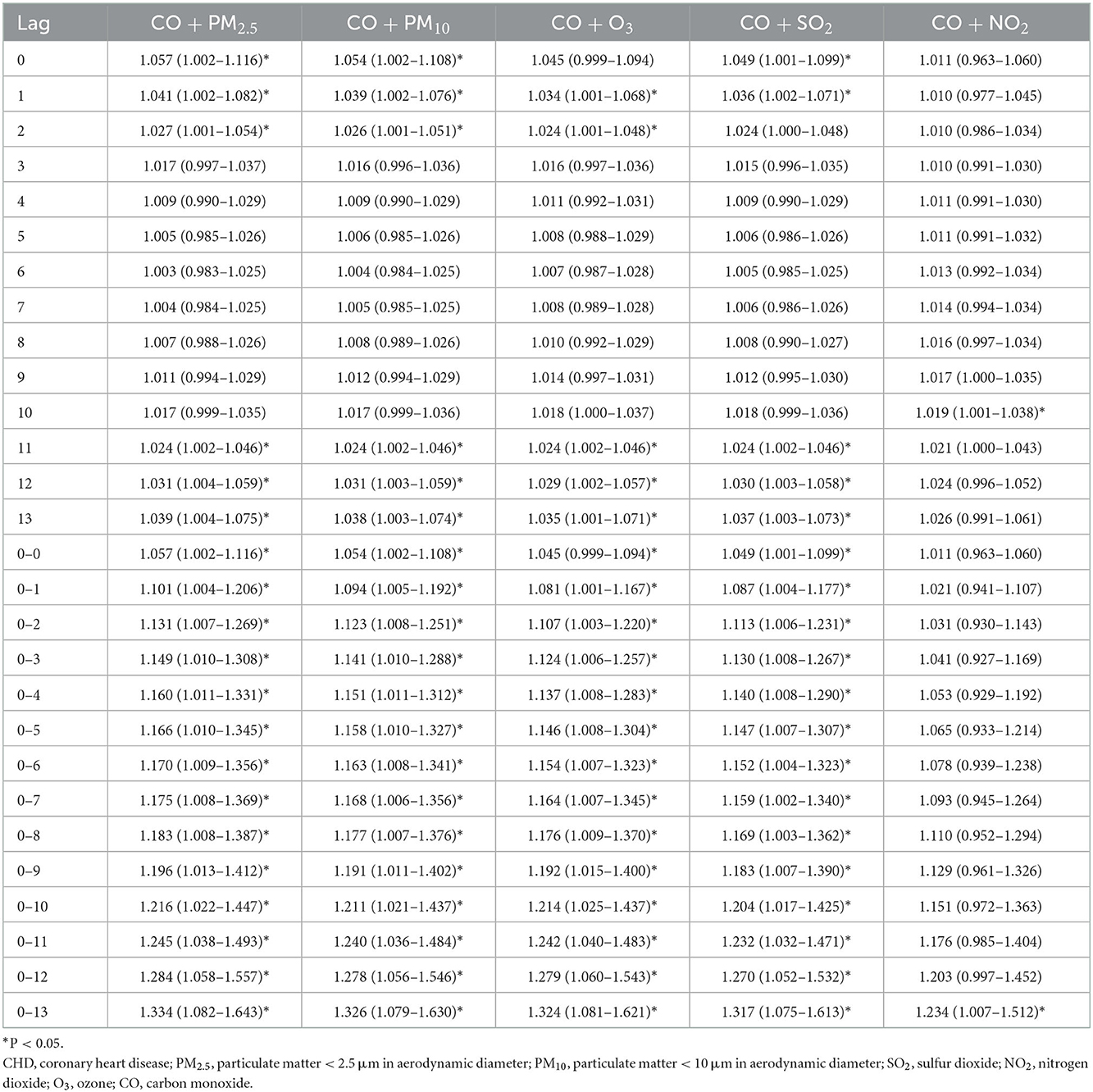
Table 3. The association between an increase of 1ug/m3 of CO and CHD admission in Hefei, 2014–2021 (dual pollutant model).
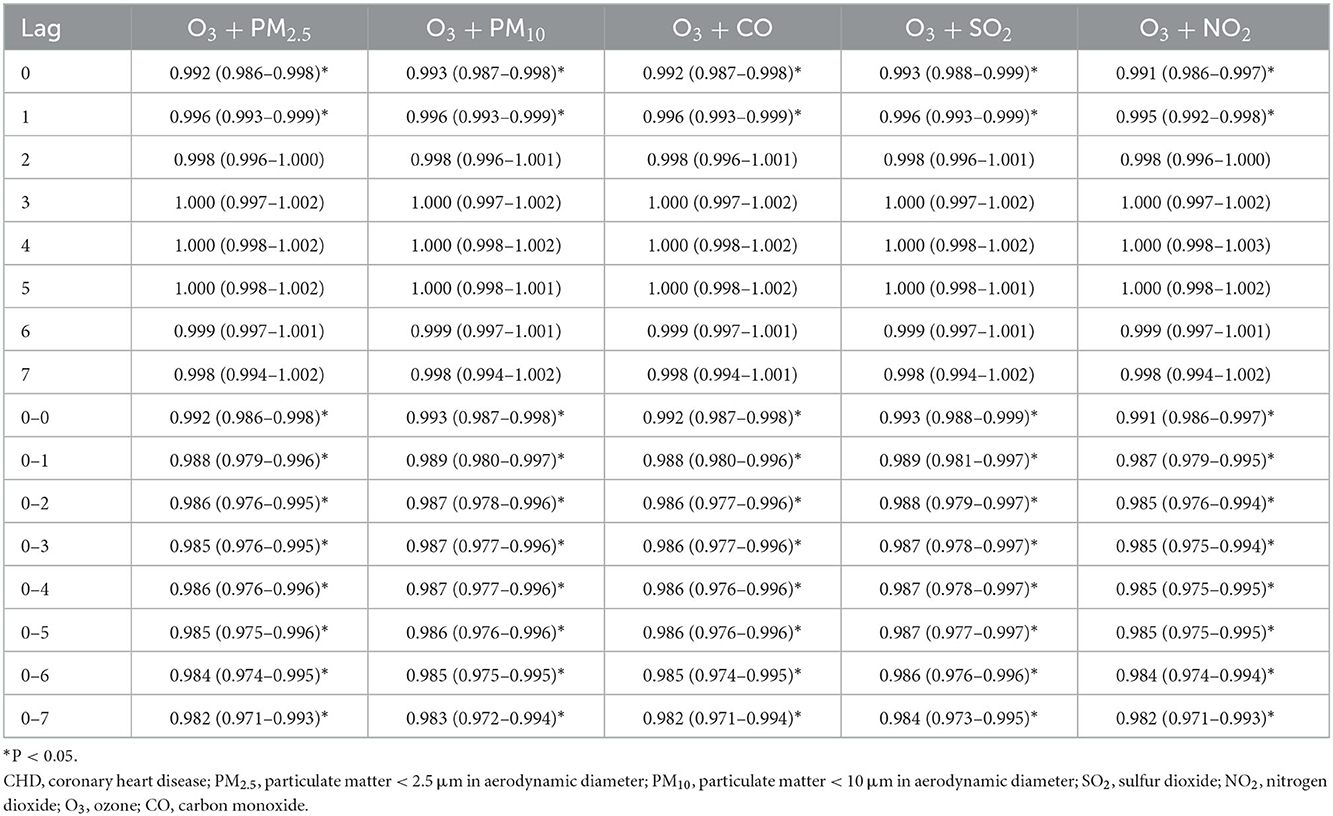
Table 4. The association between an increase of 10 μg/m3 of O3 and CHD admission in Hefei, 2014–2021 (dual pollutant model).
4. Discussion
Coronary heart disease (CHD) is a major chronic disease that threatens human health and negatively affects patients' quality of life globally. In severe cases of the disease, heart failure, myocardial infarction, and other adverse consequences may occur. More than 40% of deaths in China can be attributed to CHD (24). This implies that the potential contribution of air pollutants to the burden of CHD is significant in China. Nonetheless, the exact mechanism of air pollutants and CV systems remains inconclusive and inadequate. Previously, studies have reported the potential for air pollutants to affect the CV system directly or indirectly. The action mechanisms of air pollution may include epigenetic changes, inflammatory reactions, and other mechanisms, which can stimulate a series of pathological processes such as endothelial damage, vascular dysfunction, autonomic and neuroendocrine dysfunction, thrombosis, and atherosclerosis (25, 26). Exposure to air pollutants may worsen heart failure and reduce heart rate variability in the elderly due to decreased circulatory function and immunity (27).
With the accelerating process of industrialization and urbanization, air pollution has become a big threat to people's health, particularly in densely populated cities. In this study, we investigated the association between air pollutants and CHD hospitalization. The study's findings indicated that exposure to high concentrations of NO2 and CO correlated with an increased rate of CHD hospitalization. Furthermore, the findings revealed that exposure to O3 correlated with a lower rate of CHD hospitalization. Sensitivity analysis demonstrated that the model suited well and the outcomes were robust.
The current research found that exposure to NO2 increases the rate of CHD hospitalization. These findings are supported by previous epidemiological results (28–30). The NO2 is an index of traffic mixtures, such as ultrafine particles and diesel exhaust black carbon. Previously, studies involving experimental models have demonstrated the potential of diesel exhaust and ultrafine particles to cause noxious biological reactions (31). Thus, NO2 may promote the development of CHD via mechanisms such as the induction of inflammation and oxidative stress, coronary endothelial vasoconstriction, abnormal regulation of the cardiac autonomic nervous system, increased blood viscosity, and vasospasm. These may eventually contribute to plaque rupture, ischemia, and arrhythmias in CHD hospitalization (32, 33).
The present study revealed that O3 was negatively correlated with the rate of CHD hospitalization, which may be related to the newly discovered effects of O3. Studies have shown that O3 has effective antibacterial and antioxidant defense abilities (34) and is also related to the regulation of immune responses and accelerated wound healing (35, 36). Animal research has shown that O3 exposure can increase serum concentrations of cholesterol, triglycerides, and low-density (LDL) and very low-density (VLDL) lipoproteins. The risk of CHD increases with an increase in levels of lipid and lipoprotein concentrations in the human body, which is contrary to our findings. It is important to note that O3 is very active chemically and it can react with other air pollutants to form new compounds (37), making it unstable in the environment. Accordingly, we should be cautious in explaining the effects of O3 exposure. The mechanism of O3 in CHD remains to be further studied.
We revealed that CO exposure was related to an elevated rate of CHD hospitalization, which is consistent with previous epidemiological findings (38). Inhaled CO can combine with hemoglobin and form carboxyhemoglobin, thereby reducing the oxygen-carrying capacity of hemoglobin and leading to cellular hypoxia (39). Several possible biological mechanisms have been proposed that underlie the associations between low ambient CO concentrations and CVD. They include cardiac dysfunction (40), systemic inflammation (41), oxidative stress, and thrombotic reactions (42). Both animal models and population-based cohort studies confirm the cardiotoxicity of CO (40, 41). It has been proven that the cardiotoxicity of CO is caused by the dual effects of tissue hypoxia and direct effects on the myocardium. Chen et al. (42) carried out longitudinal research where they investigated 61 patients with CVD. The authors observed that the high-sensitivity c-reactive protein was elevated with short-term exposure to CO. They further reported that Fibrinogen and D-dimer were increased in response to environmental CO exposure. These biomarkers have been associated with thrombotic responses (43). Overall, the above evidence and our outcomes support a correlation between environmental CO exposure and CHD hospitalization risk.
To elucidate the correlation between the four aforementioned air pollutants and the rate of CHD hospitalization, further subgroup analyses regarding varied age, sex, and season groups were conducted. The results showed a correlation between NO2 exposure and an elevated CHD hospitalization rate in young, female patients with CHD during the hot season. This may be because NO2 pollution mainly comes from car exhaust. Young people can stay in such an environment longer than the elderly because they engage in more outdoor activities. Women's immunity is weaker than that of men, and the reason that the effect is greater in hot seasons may be that the rise in temperature accelerates the spread of NO2 in the air and reacts with other pollutants. However, the association between O3 and CHD hospitalization was more obvious in female patients, patients aged over 65 years, and during the cold season. These findings may be attributed to the fact that women and the elderly are more sensitive to the external environment. Moreover, the photoreaction rate is relatively high in the summer, and the second-generation concentration of O3 is higher than that in the winter. In terms of CO, it was revealed that CO exposure and CHD hospitalization was more common among patients aged above 65 years. This result implies that age-related serious brain systemic inflammatory environment and lower cerebrovascular hemodynamics might cause CHD cases among the elderly who are more sensitive to the mediators of CO.
Noteworthy, the current study is not without shortcomings. First, we did not consider the spatial heterogeneity of urban air pollution and we calculated the average value of 10 fixed sites by considering the exposure index of NO2, O3, and CO. Anyway, almost all the families of hospitalized patients with CHD are within the detection range of urban monitoring stations, which avoids significant spatial differences to some extent. Second, the time-series study methodology is naturally an ecological design, which may be vulnerable to ecological fallacies. Third, the lack of detailed individual information for patients with CHD, such as exercise, mental and mental stress, smoking, and heavy drinking, may have limited subgroup analyses. Fourth, we, unfortunately, failed to obtain specific individual diagnostic data to distinguish between first CHD outpatient visits and recurrent CHD outpatient visits, thus restricting the potential clinical impact of the study results. Finally, this is a single city study. Because of geographical and climatic divergence, the results need to be further verified when extrapolated to other regions.
Despite the shortcomings, our study also has some advantages. Since previous studies have focused more on the health effects of particulate matter on CHD, we have investigated the meteorological factors of NO2, CO, and O3 on the risk of CHD hospitalization. In addition, we have identified that the correlation between air pollution exposure and hospitalization risk of CHD may be influenced by season, gender, and age. This information may form a basis upon which healthcare practitioners may formulate novel protective measures to minimize the adverse health effects of air pollution on CHD risk earlier.
5. Conclusion
Overall, this time-series study shows that short-term exposures to NO2 and CO positively correlate with the risk of CHD hospitalization. However, O3 exposure is related to a decreased risk of CHD. Pollutant effects are different in different populations, according to stratified analysis. Consequently, to effectively reduce the risk of CHD admission, it is essential to construct a sound environmental monitoring and feedback system. For susceptible populations, appropriate preventive measures should be developed to avoid unnecessary and harmful exposure.
Data availability statement
The original contributions presented in the study are included in the article/Supplementary material, further inquiries can be directed to the corresponding authors.
Author contributions
Y-TF: conceptualization, methodology, and software. C-FL: data curation and writing—original draft preparation. MH, YF, Y-SH, and YC: writing—reviewing and editing. CC: visualization and investigation. YF, R-DZ, and XF: supervision. L-QJ: software and validation. All authors contributed to the article and approved the submitted version.
Conflict of interest
The authors declare that the research was conducted in the absence of any commercial or financial relationships that could be construed as a potential conflict of interest.
Publisher's note
All claims expressed in this article are solely those of the authors and do not necessarily represent those of their affiliated organizations, or those of the publisher, the editors and the reviewers. Any product that may be evaluated in this article, or claim that may be made by its manufacturer, is not guaranteed or endorsed by the publisher.
Supplementary material
The Supplementary Material for this article can be found online at: https://www.frontiersin.org/articles/10.3389/fpubh.2022.1090443/full#supplementary-material
Abbreviations
CHD, coronary heart disease; CVD, cardiovascular disease; PM2.5, particulate matter < 2.5 μm in aerodynamic diameter; PM10, particulate matter < 10 μm in aerodynamic diameter; SO2, sulfur dioxide; NO2, nitrogen dioxide; O3, ozone; CO, carbon monoxide; RR, relative risk; CI, confidence interval; IQR, interquartile range; DLNM, distributed lag non-linear model; ns, natural cubic spline; GLM, generalized linear model; MT, mean temperature; RH, relative humidity; Q-AIC, quasi-poisson akaike information criterion.
References
1. Lozano R, Naghavi M, Foreman K, Lim S, Shibuya K, Aboyans V, et al. Global and regional mortality from 235 causes of death for 20 age groups in 1990 and 2010: a systematic analysis for the Global Burden of Disease Study 2010. Lancet. (2012) 380:2095–128. doi: 10.1016/S0140-6736(12)61728-0
2. Benjamin EJ, Muntner P, Alonso A, Bittencourt MS, Callaway CW, Carson AP, et al. Heart disease and stroke statistics-2019 update: a report from the American heart association. Circulation. (2019) 139:e56–e528. doi: 10.1161/CIR.0000000000000659
3. Johansson S, Rosengren A, Young K, Jennings E. Mortality and morbidity trends after the first year in survivors of acute myocardial infarction: a systematic review. BMC Cardiovasc Disord. (2017) 17:53. doi: 10.1186/s12872-017-0482-9
4. Chen K, Breitner S, Wolf K, Hampel R, Meisinger C, Heier M, et al. Temporal variations in the triggering of myocardial infarction by air temperature in Augsburg, Germany, 1987–2014. Eur Heart J. (2019) 40:1600–8. doi: 10.1093/eurheartj/ehz116
5. Fiordelisi A, Piscitelli P, Trimarco B, Coscioni E, Iaccarino G, Sorriento D. The mechanisms of air pollution and particulate matter in cardiovascular diseases. Heart Fail Rev. (2017) 22:337–47. doi: 10.1007/s10741-017-9606-7
6. Verberkmoes NJ, Hamad MAS, Woorst JFT, Tan MESH, Peels CH, van Straten AHM. Impact of temperature and atmospheric pressure on the incidence of major acute cardiovascular events. Neth Heart J. (2012) 20:193–6. doi: 10.1007/s12471-012-0258-x
7. Liu W, Xu Z, Yang T. Health effects of air pollution in China. Int J Environ Res Public Health. (2018) 15:1471. doi: 10.3390/ijerph15071471
8. Losacco C, Perillo A. Particulate matter air pollution and respiratory impact on humans and animals. Environ Sci Pollut Res Int. (2018) 25:33901–10. doi: 10.1007/s11356-018-3344-9
9. Thompson JE. Airborne particulate matter: human exposure and health effects. J Occup Environ Med. (2018) 60:392–423. doi: 10.1097/JOM.0000000000001277
10. Miller MR. Oxidative stress and the cardiovascular effects of air pollution. Free Radic Biol Med. (2020) 151:69–87. doi: 10.1016/j.freeradbiomed.2020.01.004
11. Rajagopalan S, Al-Kindi SG, Brook RD. Air pollution and cardiovascular disease: JACC state-of-the-art review. J Am Coll Cardiol. (2018) 72:2054–70. doi: 10.1016/j.jacc.2018.07.099
12. Carugno M, Consonni D, Randi G, Catelan D, Grisotto L, Bertazzi PA, et al. Air pollution exposure, cause-specific deaths and hospitalizations in a highly polluted Italian region. Environ Res. (2016) 147:415–24. doi: 10.1016/j.envres.2016.03.003
13. Li G, Zeng Q, Pan X. Disease burden of ischaemic heart disease from short-term outdoor air pollution exposure in Tianjin, 2002–2006. Eur J Prev Cardiol. (2016) 23:1774–82. doi: 10.1177/2047487316651352
14. Wang, L., Wu X., Du J., Cao W., Sun S. (2021). Global burden of ischemic heart disease attributable to ambient PM (2.5) pollution from 1990 to 2017. Chemosphere. 263, 128134. doi: 10.1016/j.chemosphere.2020.128134
15. Zhou M, Wang H, Zeng X, Yin P, Zhu J, Chen W, et al. Mortality, morbidity, and risk factors in China and its provinces, 1990–2017: a systematic analysis for the global burden of disease study 2017. Lancet. (2019) 394:1145–58. doi: 10.1016/S0140-6736(19)30427-1
16. Jiang W, Chen H, Liao J, Yang X, Yang B, Zhang Y, et al. The short-term effects and burden of particle air pollution on hospitalization for coronary heart disease: a time-stratified case-crossover study in Sichuan, China. Environ Health. (2022) 21:19. doi: 10.1186/s12940-022-00832-4
17. Yao C, Wang Y, Williams C, Xu C, Kartsonaki C, Lin Y, et al. The association between high particulate matter pollution and daily cause-specific hospital admissions: a time-series study in Yichang, China. Environ Sci Pollut Res Int. (2020) 27:5240–50. doi: 10.1007/s11356-019-06734-2
18. Ye X, Peng L, Kan H, Wang W, Geng F, Mu Z, et al. Acute effects of particulate air pollution on the incidence of coronary heart disease in Shanghai, China. PLoS ONE. (2016) 11:e0151119. doi: 10.1371/journal.pone.0151119
19. Seo H, Yoon S-J, Yoon J, Kim D, Gong Y, Kim AR, et al. Recent trends in economic burden of acute myocardial infarction in South Korea. PLoS ONE. (2015) 10:e0117446. doi: 10.1371/journal.pone.0117446
20. Gasparrini A. Modeling exposure-lag-response associations with distributed lag non-linear models. Stat Med. (2014) 33:881–99. doi: 10.1002/sim.5963
21. Gasparrini A, Armstrong B, Kenward MG. Distributed lag non-linear models. Stat Med. (2010) 29:2224–34. doi: 10.1002/sim.3940
22. Huang K, Ding K, Yang X-J, Hu C-Y, Jiang W, Hua X-G, et al. Association between short-term exposure to ambient air pollutants and the risk of tuberculosis outpatient visits: a time-series study in Hefei, China. Environ Res. (2020) 184:109343. doi: 10.1016/j.envres.2020.109343
23. Bhaskaran K, Gasparrini A, Hajat S, Smeeth L, Armstrong B. Time series regression studies in environmental epidemiology. Int J Epidemiol. (2013) 42:1187–95. doi: 10.1093/ije/dyt092
24. Weiwei C, Runlin G, Lisheng L, Manlu Z, Wen W, Yongjun W, et al. Outline of the report on cardiovascular diseases in China, 2014. Eur Heart J Suppl. (2016) 18:F2–f11. doi: 10.1093/eurheartj/suw030
25. Kodavanti UP. Stretching the stress boundary: linking air pollution health effects to a neurohormonal stress response. Biochim Biophys Acta. (2016) 1860:2880–90. doi: 10.1016/j.bbagen.2016.05.010
26. Pope CA, Verrier RL, Lovett EG, Larson AC, Raizenne ME, Kanner RE, et al. Heart rate variability associated with particulate air pollution. Am Heart J. (1999) 138:890–9.
27. Shah ASV, Langrish JP, Nair H, McAllister DA, Hunter AL, Donaldson K, et al. Global association of air pollution and heart failure: a systematic review and meta-analysis. Lancet. (2013) 382:1039–48. doi: 10.1016/S0140-6736(13)60898-3
28. Atkinson RW, Carey IM, Kent AJ, van Staa TP, Anderson HR, Cook DG. Long-term exposure to outdoor air pollution and incidence of cardiovascular diseases. Epidemiology. (2013) 24:44–53. doi: 10.1097/EDE.0b013e318276ccb8
29. Huang J, Yang Z. Correlation between air temperature, air pollutants, and the incidence of coronary heart disease in Liaoning Province, China: a retrospective, observational analysis. Ann Palliat Med. (2021) 10:12412–9. doi: 10.21037/apm-21-3212
30. Klompmaker JO, Hart JE, James P, Sabath MB, Wu X, Zanobetti A, et al. Air pollution and cardiovascular disease hospitalization: are associations modified by greenness, temperature and humidity? Environ Int. (2021) 156:106715. doi: 10.1016/j.envint.2021.106715
31. Araujo JA, Nel AE. Particulate matter and atherosclerosis: role of particle size, composition and oxidative stress. Part Fibre Toxicol. (2009) 6:24. doi: 10.1186/1743-8977-6-24
32. Brook RD, Rajagopalan S, Pope CA, Brook JR, Bhatnagar A, Diez-Roux AV, et al. Particulate matter air pollution and cardiovascular disease: an update to the scientific statement from the American Heart Association. Circulation. (2010) 121:2331–78. doi: 10.1161/CIR.0b013e3181dbece1
33. Mustafic H, Jabre P, Caussin C, Murad MH, Escolano S, Tafflet M, et al. Main air pollutants and myocardial infarction: a systematic review and meta-analysis. JAMA. (2012) 307:713–21. doi: 10.1001/jama.2012.126
34. Delgado-Roche L, Riera-Romo M, Mesta F, Hernández-Matos Y, Barrios JM, Martínez-Sánchez G, et al. Medical ozone promotes Nrf2 phosphorylation reducing oxidative stress and pro-inflammatory cytokines in multiple sclerosis patients. Eur J Pharmacol. (2017) 811:148–54. doi: 10.1016/j.ejphar.2017.06.017
35. Elvis AM, Ekta JS. Ozone therapy: a clinical review. J Nat Sci Biol Med. (2011) 2:66–70. doi: 10.4103/0976-9668.82319
36. Mirowsky JE, Dailey LA, Devlin RB. Differential expression of pro-inflammatory and oxidative stress mediators induced by nitrogen dioxide and ozone in primary human bronchial epithelial cells. Inhal Toxicol. (2016) 28:374–82. doi: 10.1080/08958378.2016.1185199
37. Borges GÁ, Elias ST, da Silva SMM, Magalhães PO, Macedo SB, Ribeiro APD, et al. In vitro evaluation of wound healing and antimicrobial potential of ozone therapy. J Craniomaxillofac Surg. (2017) 45:364–70. doi: 10.1016/j.jcms.2017.01.005
38. Smith GS, Van Den Eeden SK, Garcia C, Shan J, Baxter R, Herring AH, et al. Air pollution and pulmonary tuberculosis: a nested case-control study among members of a Northern California health plan. Environ Health Perspect. (2016) 124:761–8. doi: 10.1289/ehp.1408166
39. Li H, Wu J, Wang A, Li X, Chen S, Wang T, et al. Effects of ambient carbon monoxide on daily hospitalizations for cardiovascular disease: a time-stratified case-crossover study of 460,938 cases in Beijing, China from 2013 to 2017. Environ Health. (2018) 17:82. doi: 10.1186/s12940-018-0429-3
40. Andre L, Boissière J, Reboul C, Perrier R, Zalvidea S, Meyer G, et al. Carbon monoxide pollution promotes cardiac remodeling and ventricular arrhythmia in healthy rats. Am J Respir Crit Care Med. (2010) 181:587–95. doi: 10.1164/rccm.200905-0794OC
41. Satran D, Henry CR, Adkinson C, Nicholson CI, Bracha Y, Henry TD. Cardiovascular manifestations of moderate to severe carbon monoxide poisoning. J Am Coll Cardiol. (2005) 45:1513–6. doi: 10.1016/j.jacc.2005.01.044
42. Chen S-Y, Chan C-C, Su T-C. Particulate and gaseous pollutants on inflammation, thrombosis, and autonomic imbalance in subjects at risk for cardiovascular disease. Environ Pollut. (2017) 223:403–8. doi: 10.1016/j.envpol.2017.01.037
Keywords: air pollutants, CHD, NO2, CO, O3, disease risk
Citation: Feng Y-T, Lang C-F, Chen C, Harry Asena M, Fang Y, Zhang R-D, Jiang L-Q, Fang X, Chen Y, He Y-S, Wang P and Pan H-F (2023) Association between air pollution exposure and coronary heart disease hospitalization in a humid sub-tropical region of China: A time-series study. Front. Public Health 10:1090443. doi: 10.3389/fpubh.2022.1090443
Received: 05 November 2022; Accepted: 16 December 2022;
Published: 11 January 2023.
Edited by:
Zengliang Ruan, Southeast University, ChinaReviewed by:
Xiaowen Wang, Peking University, ChinaMiao Cai, School of Public Health, Sun Yat-sen University, China
Wangjian Zhang, Sun Yat-sen University, China
Copyright © 2023 Feng, Lang, Chen, Harry Asena, Fang, Zhang, Jiang, Fang, Chen, He, Wang and Pan. This is an open-access article distributed under the terms of the Creative Commons Attribution License (CC BY). The use, distribution or reproduction in other forums is permitted, provided the original author(s) and the copyright owner(s) are credited and that the original publication in this journal is cited, in accordance with accepted academic practice. No use, distribution or reproduction is permitted which does not comply with these terms.
*Correspondence: Peng Wang, wangpeng19910318@sina.com; Hai-Feng Pan,
panhaifeng@ahmu.edu.cn
†These authors have contributed equally to this work and share first authorship