- 1Department of Social Medicine, Graduate School of Medicine, Hirosaki University, Hirosaki, Japan
- 2Department of Human Ecology, Graduate School of Medicine, The University of Tokyo, Tokyo, Japan
- 3Pre-clinical Department, University of Medicine 2, Yangon, Myanmar
- 4Pre-clinical Department, University of Medicine Taunggyi, Taunggyi, Myanmar
- 5Department of Geography, University of Yangon, Yangon, Myanmar
Maintaining telomere length plays a crucial role in regulating cellular life span. Telomere lengthening or shortening is one of the important biomarkers which could predict the preceding or present diseases. Meanwhile, the impact of environmental arsenic exposure on telomere length has increasingly concerned. Although previous studies demonstrated the effects of arsenic on telomere length, the findings were unclear on whether telomere shortens or lengthens by arsenic exposure. Thus, this manuscript summarized and discussed the telomere length alteration following arsenic exposure and the possible does-response effect of arsenic on telomere length. The present review suggested that different age groups may respond differently to arsenic exposure, and the dose-response effect of arsenic could be a critical factor in its effect on telomere length. Moreover, speciation analysis of arsenic could be more informative in identifying the effect of arsenic on telomere length.
1. Introduction
Telomeres are repetitive DNA oligomer sequences (TTAGGG) located at the ends of the eukaryotic chromosomes (1, 2). They prevent chromosomal damage by protecting against chromosome-chromosome fusions and degradation, thereby maintaining genomic integrity (1, 2). Telomere length is largely maintained by the telomerase RNA protein complex and the shelterin proteins, whose level is regulated by the telomerase reverse transcriptase (hTERT) and telomerase RNA (hTR) genes (1–5). Telomere shortening occurs in normal somatic cells following each cell division (1, 2). Once they reach a certain minimum length, cellular apoptosis occurs as a result of chromosomal end fusion (2). Telomerase can add the telomere DNA sequences (TTAGGG repeats) to prevent chromosomal fusion, and restore DNA lost to some extent (1–4). Dysfunctional telomeres and telomerase activity, in turn, may lead to genomic instability and malignancy (6–9). Therefore, maintaining optimal telomere length plays a crucial role in the regulation of cellular life span. Meanwhile, telomere length alteration was found to be correlated with aging and increased risks of many chronic diseases and malignancies (10–15).
Arsenic is a well-known hazardous metal naturally present in the environment and is also considered as a genotoxic metal (16, 17). In general, among two types of arsenic, organic and inorganic, inorganic arsenic is more toxic to humans (18). Natural contamination of inorganic arsenic in the groundwater is increasingly concerning for the health of residents in many countries where the groundwater is the primary source of arsenic exposure (19). Inorganic arsenic is exposed to human beings mainly through the oral route by drinking arsenic-contaminated water and food. Additionally, individuals can get exposed to arsenic via smoking either in passive or active ways (17–19). Globally, at least 200 million people in 140 countries have been threatened by arsenic toxicity acquired through drinking arsenic-contaminated water (18). Chronic arsenic exposure can result in significant health outcomes in the exposed population, including several diseases and cancer.
The impact of environmental and occupational heavy metals exposure on telomere length has increasingly concerned. Not an exclusion, arsenic exposure appears to modify the telomere length, which may predispose to adverse health risks (17, 20, 21). Arsenic induces oxidative stress and alters DNA repair and DNA methylation patterns, which could potentially affect telomere maintenance (16, 22–24). So far, many possible underlying mechanisms of arsenic-induced telomere length alteration have been proposed. Arsenic was shown to be involved in telomere shortening through the mediation of arsenic-induced apoptosis (21, 25). It is also possible that arsenic-induced oxidative stress directly damages the telomeres and telomere-related proteins (21, 26). The effect of arsenic on telomerase hTERT gene expression is also being concerned since telomerase activation plays a crucial role in telomere maintenance (26, 27).
Regarding the previous findings of arsenic-induced telomere length, the results are conflicting as some studies showed a positive association while others demonstrated a negative association between arsenic exposure and telomere length. These contrary results call to explore the knowledge gap on the effect of arsenic on telomere maintenance. Therefore, this manuscript summarized and discussed the telomere length alteration following arsenic exposure and the possible dose-response effect of arsenic on telomere length.
2. Mechanistic insight of arsenic-induced telomere length alteration
2.1. Arsenic-induced telomere lengthening
In the mechanistic insight, arsenic-induced telomere lengthening is caused by either telomerase-dependent or independent pathways. In the case of telomerase (hTERT) dependent pathway, the up-regulation of hTERT triggers telomere lengthening by synthesizing new telomere DNA sequences, and it was positively correlated with arsenic exposure (28–30). In accordance, a study conducted in Mongolia reported that hTERT expression was highly associated the arsenic exposure in both in-vivo and in-vitro studies (27). Moreover, hTERT expression is strongly linked to arsenic exposure despite its non-significant association with telomere length (31). Meanwhile, according to a case-control study in India, telomere lengthening could be telomerase-independent and triggered through the up-regulation of shelterin complex proteins such as TRF1 and TRF2, resulting in “alternative telomere lengthening (ATL)” (31). Alteration in these gene expressions may cause telomere de-protection, contributing to the activation of ATL (32).
A feasible telomerase-independent mechanism is the epigenetic modifications of subtelomeric DNA. In most cases, hypomethylation of subtelomeric DNA explains lengthening telomere, although some studies suggest hypermethylation in subtelomeric regions (33–36). A study in India revealed that chronic arsenic exposure lengthens telomere, and is correlated with subtelomeric methylation pattern changes (37). A couple of studies also demonstrated that telomere length is mainly altered by pattern changes in subtelomeric methylation, resulting in telomeric chromatin assemblage (35, 38). Thus, it is plausible for the distortion of telomere maintenance by the methylation pattern changes along with arsenic exposure and its effect on telomere length regulation.
2.2. Arsenic-induced telomere shortening
Arsenic-induced telomere shortening was well-known oxidative-stress-induced toxicity. Telomeres are made of high guanine content and are sensitive to oxidative stress; thus, high arsenic exposure may induce oxidative stress, rendering telomere attrition (21, 39–41). It was also reported that the primary mechanism behind the negative association between arsenic and telomere length was due to arsenic toxicity in the distortion of oxidative/anti-oxidant equilibrium (42, 43). This was probably facilitated by redox species overpowering anti-oxidant defenses, resulting in a shorter telomere, inflammation, and insufficient DNA repair mechanisms (17, 44–47).
Apart from the oxidative-stress-induced pathway, arsenic can trigger the fusion of chromosomal ends by downregulating telomere repeat binding factor in leukemia cell lines (26, 48). Some laboratory studies have also demonstrated that arsenic compound (As2O3) selectively suppresses the transcription of the hTERT gene by lowering the expression of some of the hTERT transcription factors in a dose-dependent manner (0.2-2 μM, 2.5 μM, 5 μM, and 10 μM, respectively) (29, 30). Further, arsenic can induce the up-regulation of heat shock proteins, oncogenes, and some cell growth factors that are also positive regulators of telomerase and cell proliferation (49).
3. Arsenic exposure and telomere length
3.1. Arsenic exposure and telomere length in adult populations
A couple of human population studies showed that the higher the arsenic exposure, the longer the telomere length (Table 1) (31, 32, 50, 52, 54, 55). For instance, a study conducted among Argentinian women supported the dose-related association (55). While the urinary arsenic levels of the participants varied from 0.1 to 1,251 μg/L, it is reported that an individual with an increased urinary arsenic tends to have longer telomeres (55). A case-control study, involving two groups of arsenic-exposed Indian adults—with skin lesions and without skin lesions in comparison with the control group, also revealed that the possible arsenic-induced lengthening was observed among the individuals with the skin-lesion (31). Likewise, 40 adults from India with neoplastic and surrounding normal tissues were recruited again 6 years later; not only was telomere length in tumor cells longer than that in normal cells, but higher arsenic levels also seemed to increase telomere length in that population (37). Based on the data from two large studies among the Bangladesh population, the same conclusion was drawn from 167 participants regarding the lengthening of telomeres in response to high arsenic concentration despite some controversial genetic results (32). The study in Mexico also casts light on arsenic′s role in lengthening telomere length (52). The study involved 188 Mexican males: 78 subjects with urine arsenic levels of 11.2–17.4 μg/L as an exposed group and 112 with lower concentrations as a control group (52). Similarly, in the study among Bolivians, the data from 193 recruited females reflected the correlation of arsenic exposure to telomere length as a biomarker of arsenic toxicity (50). The study also claimed that there is no gender difference in the associations between arsenic and telomere length (50).
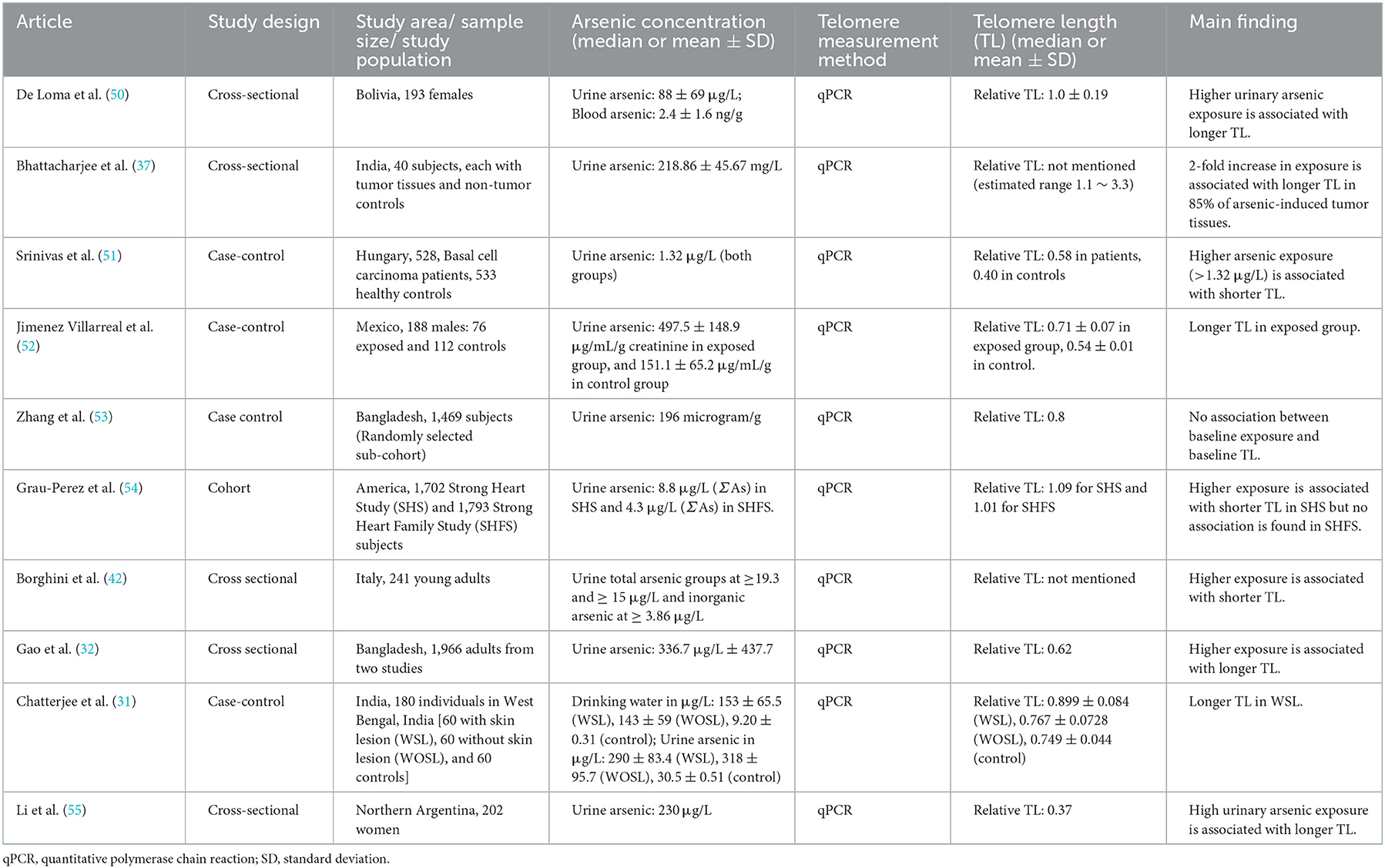
Table 1. Summarized studies regarding the associations between arsenic exposure and telomere length in adults.
Meanwhile, there are some inconsistencies while the speciation analysis of arsenic is considered. In a large population study of the American Indian tribe, higher arsenic exposure tends to shorten telomere length among men and women (54). The average level of arsenic, calculated as the sum of inorganic and metabolite forms, was 8.8 μg/g creatinine. Whilst the negative association was more robust in the over-50 group, it was negligible among the middle-aged group (54). Another report investigating young male Italians from four arsenic-polluted regions also stated that long-term exposure to arsenic was associated with telomere attrition through DNA damage, resulting in shorter telomere length (42). The study evaluated the effects of arsenic metabolites on telomere length rather than the effect of total arsenic exposure. A case-control study with a lower arsenic exposure claimed a negative association between arsenic and telomere length (51). In addition, some studies reported no significant association between arsenic exposure and telomere length (53, 54). In summary, the previous literature among the human adult populations is conflicting in demonstrating the effect of arsenic on telomere length.
3.2. Early life arsenic exposure and telomere length in children and adolescents
Although the positive relationship was mainly observed in the adult population studies, there are some conflicting results in other age groups (Table 2). For example, from the study of 476 Bangladesh primary schoolers, urinary arsenic exposure (mean = 143.85 μg/L) was negatively associated with leucocyte telomere length (43). Specifically, with a 2-fold increase in urinary arsenic, telomere length was shortened by 0.017 relatively (43). Meanwhile, the results among childhood or adolescent populations are inconsistent. For instance, arsenic exposure was significantly and positively associated with telomere elongation in Mexican children (57). On the other hand, some studies showed no significant association between arsenic and telomere length as in Nepal and Indian populations (62, 63). In terms of exposure dose, Mexican elementary children had a mean urinary arsenic level of 54.78 μg/L, while the Indian and Nepal adolescents were in the higher range of 74.7 ± 34.9 μg/L and 114.5 ± 241.3 μg/L, respectively (62, 63). The exposure dose was lower than that of the previous study among Bangladesh children of similar age (43).
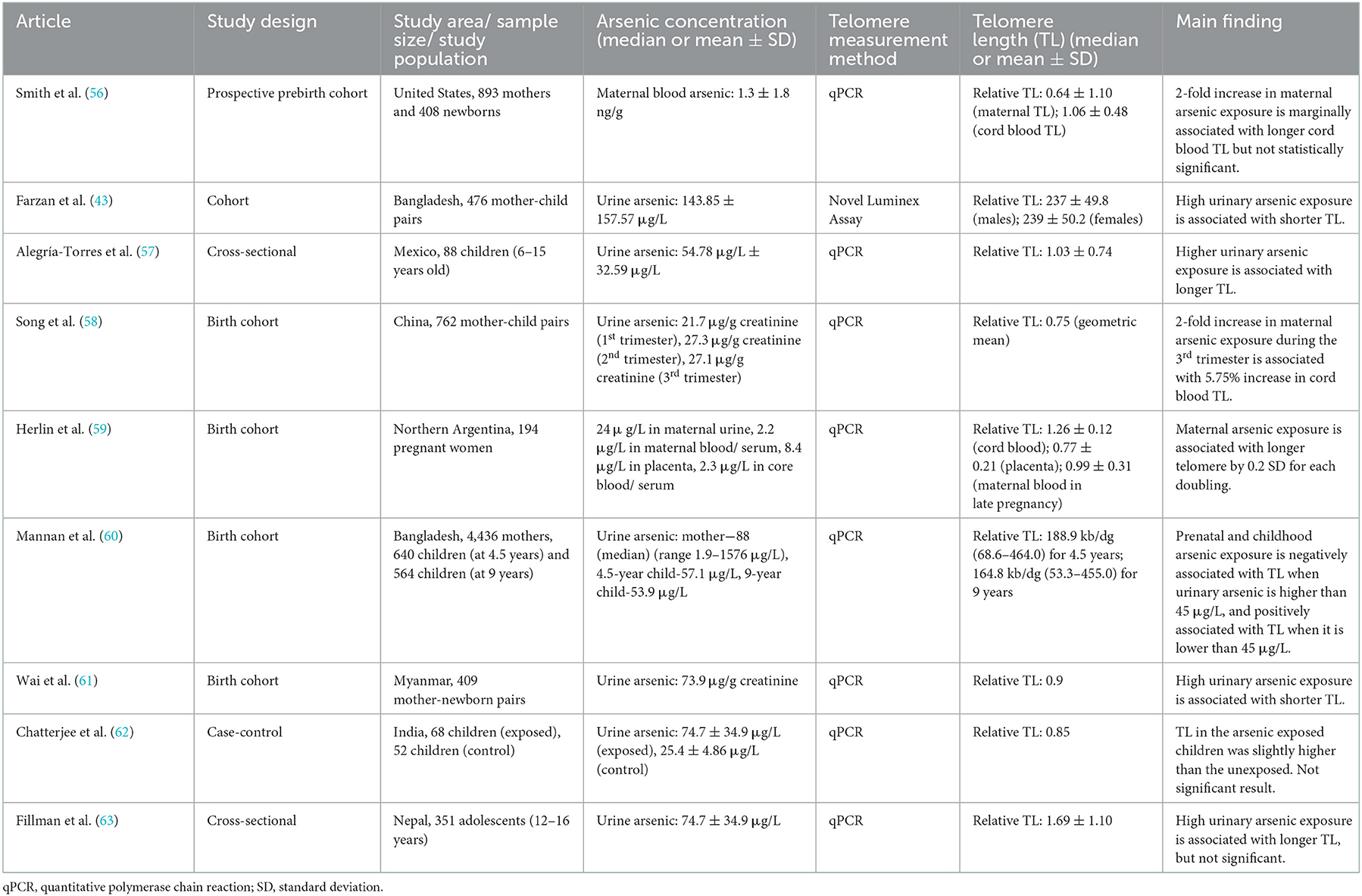
Table 2. Summarized studies regarding the associations between arsenic exposure and telomere length in children or adolescents.
In concern of the trans-placental transfer of arsenic, a couple of birth-cohort studies have been performed to examine the effect of prenatal arsenic exposure on the offspring's telomere length. A previous study in Wuhan (China) with 762 mother-newborn pairs reported a strong association between maternal arsenic exposure during the third trimester and the telomere length of the newborns (58). If arsenic exposure levels are raised by 2-fold among mature pregnant women, telomere length in their offspring is predicted to be longer by 5.75% (58). However, with blood arsenic levels being in the range of 1.3 ± 1.8 ng/g, there was only a marginal association in a similar prebirth cohort in the United States (56). Furthermore, a research team led by Herlin M, et al. (59) from Northern Argentina conducted a mother-child cohort study to pinpoint the consequences of multiple toxic metals including arsenic in the body, showing that the arsenic content in maternal blood and cord blood has no association with the offspring′s telomere length; arsenic concentration in the placenta blood was somehow found to have an association with telomere elongation in the offspring.
In contrast, a previous study in Myanmar among 409 mother-child pairs indicated a negative correlation between the telomere length in newborns and prenatal arsenic exposure (median urinary arsenic = 73.9 μg/g creatinine) (61). In another cohort study in Bangladesh, children from mothers with a higher arsenic concentration in urine or a higher arsenic exposed children had shorter telomeres when their urinary arsenic values exceeded 45 μg/L, demonstrating a negative relationship. Numerically, if the arsenic exposure was doubled, 34 kb/dg of telomere length was shortened. Interestingly, at below 45 μg/L, arsenic was found to be related to telomere elongation among the 9-year-olds, demonstrating a positive relationship (60). These contrary results suggest a call to explore more for an understanding dose-response effect of arsenic on telomere maintenance.
4. Considerations for the effects of arsenic on telomere length
4.1. A possible dose-response effect of arsenic on telomere length
Telomere susceptibility to arsenic exposure could be dose-dependent, and it would be worth focusing on the understanding of the dose-response effect of arsenic on telomere and telomerase. In the previous in-vitro study on human cord blood cells, it was stated that telomere length was lengthened at an inorganic arsenic exposure level of both 0.0001 μM and 1 μM after 24 h of treatment, while telomere length was shortened at a high concentration of 1 μM after 7 days in culture (28). Consistently, another study on human cell lines in-vitro also supported that telomerase activity induced by arsenite (AsIII) concentration at 0.1–1 μM resulted in telomere elongation, whereas a high concentration of more than 1–40 μM shortened telomere length (25). The above-mentioned findings highlight that it is necessary to investigate at which dose arsenic may activate the ALT pathway that leads to telomere lengthening or may induce oxidative pathway that leads to telomere shortening in the human population.
4.2. Differences in the responses by age groups
Most studies agreed upon the shortening effect of arsenic on telomere length among childhood and adolescents. Contrarily, a previous study among Mexican elementary children reported a telomere lengthening where the study population had a mean urinary arsenic concentration of 54.78 μg/L (57). In fact, the study among elementary Bangladesh children stated the opposite findings; their mean urinary arsenic level (143.8 μg/L) was twice that of the Mexicans′ study (42). The relationship among adolescents was non-significant according to Indians, whose concentration was at 74.7 μg/L (62). Nonetheless, telomere length was significantly shorter among high arsenic-exposed Nepali adolescents (114.5 μg/L) (63). According to these controversial relationships among childhood communities, it could be deduced that the larger exposure to arsenic could lead to telomere shortening while the smaller exposure might respond differently among children or adolescents.
The findings of some cohort studies that studied mother-newborn or mother-children's pairs are also controversial; two studies reckoned the association between arsenic and telomere length to be positive (58, 59); however, the two other papers revealed a negative association (60, 61). A study in Chinese mother-newborn pairs claimed a direct correlation between prenatal arsenic exposure, especially during the third trimester of pregnancy, and telomere length at a mean urinary arsenic concentration of 27.1 μg/g creatinine (58). In contrast, another Argentinian study claimed that telomere attrition was found only with higher urine arsenic content (mean = 124 μg/L) (59). Similarly, the Myanmar population with a slightly lower concentration on average at 73.9 μg/g creatinine showed a negative association between prenatal arsenic exposure and newborn telomere length (61). All the above three studies were further strengthened by the fourth birth cohort study in Bangladesh, which agreed on the dose-related interrelation, demonstrating a positive association at a level below the mean urine arsenic concentration of 45 μg/L and a negative association if above 45 μg/L (60).
On the other hand, most studies among the adult population somehow agreed with the results of telomere elongation by arsenic exposure. Although the mean or median value of exposure concentration varies widely across the populations, the positive association between arsenic exposure and telomere length was concluded in most of the studies. For example, higher arsenic exposure was associated with longer telomere length in the Indian population at a mean urinary arsenic level of 290 μg/L (31) and the South African population at a similar exposure dose (median = 230 μg/L) (55). A longer telomere was associated with very high exposure to arsenic (mean = 497.5 μg/L) in Mexican males (52). Meanwhile, the results are consistent in Bangladesh adults, where the mean arsenic concentration was about half of the above studies at 188 μg/L (32). In Bolivian women, the exposure dose was as low as 88 μg/L (50). Even though most exposure values are higher than those in younger populations, it is noteworthy that arsenic-induced telomere lengthening is prevalent in adulthood, thereby calling for further research on other possible contributing factors such as genetic polymorphism, occupation, living environment, and other stresses.
4.3. Differences in the responses by arsenic metabolites
Another point to be considered is the toxicity of the metabolites of arsenic. Even among inorganic forms of arsenic, arsenite (AsIII) is considered more toxic than arsenate (AsV), which is methylated by a specific enzyme, arsenite methyltransferase (AS3MT), to form end products monomethylarsinic acid (MMA), and dimethylarsinic acid (DMA) (50, 64). The relative fractions of MMA and DMA, which are produced as a result of a series of reduction and methylation reactions of inorganic arsenic, represent the effectiveness of arsenic metabolism (65). Since MMA and DMA are less toxic to tissues than AsIII or AsV, and are more readily excreted in the urine, the methylation process is thought to be a detoxification process (66). Meanwhile, higher urinary MMA levels imply more harmful to the body, promoting more arsenic-induced health conditions (66), whilst higher urinary DMA levels mean healthier, with a lower inner arsenic pool (64). Henceforth, while inorganic urinary arsenic contributes to telomere elongation, DMA has the opposite effect, according to Li′s study (55). Apart from these two end products, other metabolites, MMAIII and DMAIII are also released from the methylation process (64). Some in-vitro studies have reported that MMAIII is more reactive than AsIII and AsV and could result in DNA damage (23, 64).
Since the toxicity of arsenic varies by its metabolites, the efficiency of arsenic metabolism may alter the effect of arsenic on telomere length. In accordance, a previous study in Bolivia shows that the individuals with less efficient arsenic metabolism capacity showed a longer telomere length (50). Moreover, a study in Argentina argues that the positive association between arsenic and telomere length is more robust in individuals with less efficient arsenic metabolism (55). In addition, AS3MT, the most important metabolizing enzyme, could be of variants that could influence metabolic efficiency and vulnerability to arsenic toxicity (67–70). A previous study supports the fact by suggesting that people with the AS3MT haplotype 1 may have been linked to a slower and more hazardous metabolism of arsenic, resulting in a longer telomere than those without this haplotype (55). The study also revealed a positive trend between telomere length and arsenic exposure with increasing copy number of AS3MT haplotype 1 (55). Accordingly, previous studies also reported that the efficacy of arsenic metabolism is strongly and consistently predicted by genetic differences in AS3MT (68–71). Thus, the toxic effects of arsenic metabolites may have influenced the extent of cellular damage.
5. Conclusion
In conclusion, available information on the effects of environmental arsenic exposure on telomere length among human populations are controversial and limited. As summarized in Figure 1, this review suggested that different age groups may respond differently to arsenic exposure, and the dose-response effect of arsenic could be a critical factor in its effect on telomere length. Moreover, speciation analysis of arsenic could be more informative in identifying the effect of arsenic on telomere length. Targeting telomere and telomerase could be a possible rationale for alternative therapeutic and preventative strategies for age-related and malignant diseases. Consequently, assessment of telomere length in the early stage could be helpful in biomonitoring of health risks in later life. Therefore, further studies should focus on the dose-response effect of arsenic exposure on telomeres in human populations. Additionally, compelling comparative studies should be performed to determine the predictive impact of the toxicity of arsenic metabolites on telomere length to constitute a promising knowledge for early clinical and biological effects.
Author contributions
KW designed and conceptualized the study. KW, TS, MM, and TH drafted the original manuscript. All authors critically reviewed, edited, and approved the manuscript.
Funding
This work was supported by the Hirose Foundation Research Grant. The funder has no role in designing or decision to publish the results.
Acknowledgments
The authors would like to thank members of the Department of Social Medicine, Hirosaki University for their kind support.
Conflict of interest
The authors declare that the research was conducted in the absence of any commercial or financial relationships that could be construed as a potential conflict of interest.
Publisher's note
All claims expressed in this article are solely those of the authors and do not necessarily represent those of their affiliated organizations, or those of the publisher, the editors and the reviewers. Any product that may be evaluated in this article, or claim that may be made by its manufacturer, is not guaranteed or endorsed by the publisher.
References
1. Lu W, Zhang Y, Liu D, Songyang Z, Wan M. Telomeres-structure, function, and regulation. Exp Cell Res. (2013) 319:133–41. doi: 10.1016/j.yexcr.2012.09.005
2. Blackburn EH. Structure and function of telomeres. Nature. (1991) 350:569–73. doi: 10.1038/350569a0
3. de Lange T. Shelterin: the protein complex that shapes and safeguards human telomeres. Genes Dev. (2005) 19:2100–10. doi: 10.1101/gad.1346005
4. Palm W, de Lange T. How shelterin protects mammalian telomeres. Annu Rev Genet. (2008) 42:301–34. doi: 10.1146/annurev.genet.41.110306.130350
5. Axelrad MD, Budagov T, Atzmon G. Telomere length and telomerase activity; a Yin and Yang of cell senescence. J Vis Exp. (2013) 2013:e50246. doi: 10.3791/50246
6. Murnane JP. Telomere dysfunction and chromosome instability. Mutat Res. (2012) 730:28–36. doi: 10.1016/j.mrfmmm.2011.04.008
7. Murnane JP. Telomeres and chromosome instability. DNA Repair (Amst). (2006) 5:1082–92. doi: 10.1016/j.dnarep.2006.05.030
8. Stewart SA, Ben-Porth I, Carey VJ, O'Connor B, Hahn WC, Weinberg R, et al. Erosion of the telomeric single-strand overhang at replicative senescence. Nat Genet. (2003) 33:492–6. doi: 10.1038/ng1127
9. Shay JW, Zhou Y, Hiyama E, Wright WE. Telomerase and cancer. Hum Mol Genet. (2001) 10:677–85. doi: 10.1093/hmg/10.7.677
10. Cawthon RM, Smith KR, O'Brien E, Sivatchenko A, Kerber RA. Association between telomere length in blood and mortality in people aged 60 years or older. Lancet. (2003) 361:393–5. doi: 10.1016/S0140-6736(03)12384-7
11. Ma H, Zhou Z, Wei S, Liu Z, Pooley KA, Dunning AM, et al. Shortened telomere length is associated with increased risk of cancer: a meta-analysis. PLoS ONE. (2011) 6:e20466. doi: 10.1371/journal.pone.0020466
12. Barrett JH, Iles MM, Dunning AM, Pooley KA. Telomere length and common disease: study design and analytical challenges. Hum Genet. (2015) 134:679–89. doi: 10.1007/s00439-015-1563-4
13. Epel ES, Blackburn EH, Lin J, Dhabhar FS, Adler N, Morrow JD, et al. Accelerated telomere shortening in response to life stress. Proc Natl Acad Sci U S A. (2004) 101:17312–5. doi: 10.1073/pnas.0407162101
14. Haycock PC, Heydon EE, Kaptoge S, Butterworth AS, Thompson S, Willeit P. Leucocyte telomere length and risk of cardiovascular disease: systematic review and meta-analysis. BMJ. (2014) 349:g4227. doi: 10.1136/bmj.g4227
15. Sanders JL, Fitzpatrick AL, Boudreau RM, Arnold AM, Aviv A, Kimura M, et al. Leukocyte telomere length is associated with noninvasively measured age-related disease: the cardiovascular health study. J Gerontol A Biol Sci Med Sci. (2012) 67:409–16. doi: 10.1093/gerona/glr173
16. Gebel TW. Genotoxicity of arsenical compounds. Int J Hyg Environ Health. (2001) 203:249–62. doi: 10.1078/S1438-4639(04)70036-X
17. Jomova K, Jenisova Z, Festerova M, Baros S, Liska J, Hudecova D, et al. Arsenic: toxicity, oxidative stress and human disease. J Appl Toxicol. (2011) 31:95–107. doi: 10.1002/jat.1649
18. Weerasundara L, Ok YS, Bundschuh J. Selective removal of arsenic in water: a critical review. Environ Pollut. (2021) 268:115668. doi: 10.1016/j.envpol.2020.115668
19. Ng JC, Wang J, Shraim A. A global health problem caused by arsenic from natural sources. Chemosphere. (2003) 52:1353–9. doi: 10.1016/S0045-6535(03)00470-3
20. Zhang X, Lin S, Funk WE, Hou L. Environmental and occupational exposure to chemicals and telomere length in human studies. Occup Environ Med. (2013) 70:743–9. doi: 10.1136/oemed-2012-101350
21. Liu L, Trimarchi JR, Navarro P, Blasco MA, Keefe DL. Oxidative stress contributes to arsenic-induced telomere attrition, chromosome instability, and apoptosis. J Biol Chem. (2003) 278:31998–2004. doi: 10.1074/jbc.M303553200
22. Barnes RP, Fouquerel E, Opresko PL. The impact of oxidative DNA damage and stress on telomere homeostasis. Mech Ageing Dev. (2019) 177:37–45. doi: 10.1016/j.mad.2018.03.013
23. Nesnow S, Roop BC, Lambert G, Kadiiska M, Mason RP, Cullen WR, et al. DNA damage induced by methylated trivalent arsenicals is mediated by reactive oxygen species. Chem Res Toxicol. (2002) 15:1627–34. doi: 10.1021/tx025598y
24. Mar Wai K, Umezaki M, Mar O, Umemura M, Watanabe C. Arsenic exposure through drinking Water and oxidative stress Status: a cross-sectional study in the Ayeyarwady region, Myanmar. J Trace Elem Med Biol. (2019) 54:103–9. doi: 10.1016/j.jtemb.2019.04.009
25. Zhang TC, Schmitt MT, Mumford JL. Effects of arsenic on telomerase and telomeres in relation to cell proliferation and apoptosis in human keratinocytes and leukemia cells in vitro. Carcinogenesis. (2003) 24:1811–7. doi: 10.1093/carcin/bgg141
26. Jiao Y, Zhang W, Liu J, Ni W, Xu W, Jin J, et al. Telomere attrition and chromosome instability via downregulation of TRF2 contributes to arsenic trioxide-induced apoptosis of human T-Cell leukemia cell line molt-4 cells. Cancer Biol Ther. (2007) 6:1186–92. doi: 10.4161/cbt.6.8.4381
27. Mo J, Xia Y, Ning Z, Wade TJ, Mumford JL. Elevated human telomerase reverse transcriptase gene expression in blood cells associated with chronic arsenic exposure in Inner Mongolia, China. Environ Health Perspect. (2009) 117:354–60. doi: 10.1289/ehp.11532
28. Ferrario D, Collota A, Carfi M, Bowe G, Vahter M, Hartung T, et al. Arsenic induces telomerase expression and maintains telomere length in human cord blood cells. Toxicology. (2009) 260:132–41. doi: 10.1016/j.tox.2009.03.019
29. Zhang Y, Sun M, Shi W, Yang Q, Chen C, Wang Z, et al. Arsenic trioxide suppresses transcription of hTERT through down-regulation of multiple transcription factors in HL-60 leukemia cells. Toxicol Lett. (2015) 232:481–9. doi: 10.1016/j.toxlet.2014.11.028
30. Ghaffari SH, Momeny M, Bashash D, Mirzaei R, Ghavamzadeh A, Alimoghaddam K. Cytotoxic effect of arsenic trioxide on acute promyelocytic leukemia cells through suppression of NFkβ-dependent induction of hTERT due to down-regulation of Pin1 transcription. Hematology. (2012) 17:198–206. doi: 10.1179/1607845412Y.0000000008
31. Chatterjee D, Bhattacharjee P, Sau TJ, Das JK, Sarma N, Bandyopadhyay AK, et al. Arsenic exposure through drinking water leads to senescence and alteration of telomere length in humans: a case-control study in West Bengal, India. Mol Carcinog. (2015) 54:800–9. doi: 10.1002/mc.22150
32. Gao J, Roy S, Tong L, Argos M, Jasmine F, Rahaman R, et al. Arsenic exposure, telomere length, and expression of telomererelated genes among Bangladeshi individuals. Environ Res. (2015) 136:462–9. doi: 10.1016/j.envres.2014.09.040
33. Buxton JL, Suderman M, Pappas JJ, Borghol N, McArdle W, Blakemore AIF, et al. Human leukocyte telomere length is associated with DNA methylation levels in multiple subtelomeric and imprinted loci. Sci Rep. (2014) 4:4954. doi: 10.1038/srep04954
34. Ng LJ, Cropley JE, Pickett HA, Reddel RR, Suter CM. Telomerase activity is associated with an increase in DNA methylation at the proximal subtelomere and a reduction in telomeric transcription. Nucleic Acids Res. (2009) 37:1152–9. doi: 10.1093/nar/gkn1030
35. Oh B-K, Um T-H, Choi GH, Park YN. Frequent changes in subtelomeric DNA methylation patterns and its relevance to telomere regulation during human hepatocarcinogenesis. Int J Cancer. (2011) 128:857–68. doi: 10.1002/ijc.25398
36. Shaw RJ, Akufo-Tetteh EK, Risk JM, Field JK, Liloglou T. Methylation enrichment pyrosequencing: combining the specificity of MSP with validation by pyrosequencing. Nucleic Acids Res. (2006) 34:e78. doi: 10.1093/nar/gkl424
37. Bhattacharjee P, Das A, Giri AK. Epigenetic regulations in alternative telomere lengthening: understanding the mechanistic insight in arsenic-induced skin cancer patients. Sci Total Environ. (2020) 704:135388. doi: 10.1016/j.scitotenv.2019.135388
38. Gonzalo S, Jaco I, Fraga MF, Chen T, Li E, Esteller M, et al. DNA methyltransferases control telomere length and telomere recombination in mammalian cells. Nat Cell Biol. (2006) 8:416–24. doi: 10.1038/ncb1386
39. Houben JM, Moonen HJJ, van Schooten FJ, Hageman GJ. Telomere length assessment: biomarker of chronic oxidative stress? Free Radic Biol Med. (2008) 44:235–46. doi: 10.1016/j.freeradbiomed.2007.10.001
40. Kawanishi S, Oikawa S. Mechanism of telomere shortening by oxidative stress. Ann N Y Acad Sci. (2004) 1019:278–84. doi: 10.1196/annals.1297.047
41. von Zglinicki T. Oxidative stress shortens telomeres. Trends Biochem Sci. (2002) 27:339–44. doi: 10.1016/S0968-0004(02)02110-2
42. Borghini A, Faita F, Mercuri A, Minichilli F, Bustaffa E, Bianchi F, et al. Arsenic exposure, genetic susceptibility and leukocyte telomere length in an Italian young adult population. Mutagenesis. (2016) 31:539–46. doi: 10.1093/mutage/gew017
43. Farzan SF, Shahriar M, Kibriya MG, Jasmine F, Sarwar G, Slavkovic V, et al. Urinary arsenic and relative telomere length in 5-7 year old children in Bangladesh. Environ Int. (2021) 156:106765. doi: 10.1016/j.envint.2021.106765
44. Wang L, Liu LZ, Jiang BH. Dysregulation of microRNAs in metal-induced angiogenesis and carcinogenesis. Semin Cancer Biol. (2021) 76:279–86. doi: 10.1016/j.semcancer.2021.08.009
45. Leonard SS, Harris GK, Shi X. Metal-induced oxidative stress and signal transduction. Free Radic Biol Med. (2004) 37:1921–42. doi: 10.1016/j.freeradbiomed.2004.09.010
46. Galaris D, Evangelou A. The role of oxidative stress in mechanisms of metal-induced carcinogenesis. Crit Rev Oncol Hematol. (2002) 42:93–103. doi: 10.1016/S1040-8428(01)00212-8
47. Liu S, Sun Q, Wang F, Zhang L, Song Y, Xi S, et al. Arsenic induced overexpression of inflammatory cytokines based on the human urothelial cell model in vitro and urinary secretion of individuals chronically exposed to arsenic. Chem Res Toxicol. (2014) 27:1934–42. doi: 10.1021/tx5002783
48. Chou WC, Hawkins AL, Barrett JF, Griffin CA, Dang CV. Arsenic inhibition of telomerase transcription leads to genetic instability. J Clin Invest. (2001) 108:1541–7. doi: 10.1172/JCI14064
49. Bhattacharjee P, Banerjee M, Giri AK. Role of genomic instability in arsenic-induced carcinogenicity. A review. Environ Int. (2013) 53:29–40. doi: 10.1016/j.envint.2012.12.004
50. De Loma J, Krais AM, Lindh CH, Mamani J, Tirado N, Gardon J, et al. Arsenic exposure and biomarkers for oxidative stress and telomere length in indigenous populations in Bolivia. Ecotoxicol Environ Saf. (2022) 231:113194. doi: 10.1016/j.ecoenv.2022.113194
51. Srinivas N, Rachakonda S, Hielscher T, Calderazzo S, Rudnai P, Gurzau E, et al. Telomere length, arsenic exposure and risk of basal cell carcinoma of skin. Carcinogenesis. (2019) 40:715–23. doi: 10.1093/carcin/bgz059
52. Jimenez Villarreal J, Ortiz BM, Garza SM, Armendariz DIR, Villa VDB, Rosales PC, et al. Telomere length analysis in residents of a community exposed to arsenic. J Biochem Mol Toxicol. (2018) 2018:e22230. doi: 10.1002/jbt.22230
53. Zhang C, Kibriya MG, Jasmine F, Roy S, Gao J, Sabarinathan M, et al. A study of telomere length, arsenic exposure, and arsenic toxicity in a Bangladeshi cohort. Environ Res. (2018) 164:346–55. doi: 10.1016/j.envres.2018.03.005
54. Grau-Perez M, Zhao J, Pierce B, Francesconi KA, Goessler W, Zhu Y, et al. Urinary metals and leukocyte telomere length in American Indian communities: the strong heart and the strong heart family study. Environ Pollut. (2019) 246:311–8. doi: 10.1016/j.envpol.2018.12.010
55. Li H, Engstrom K, Vahter M, Broberg K. Arsenic exposure through drinking water is associated with longer telomeres in peripheral blood. Chem Res Toxicol. (2012) 25:2333–9. doi: 10.1021/tx300222t
56. Smith AR, Lin P-ID, Rifas-Shiman SL, Rahman ML, Gold DR, Baccarelli AA, et al. Prospective associations of early pregnancy metal mixtures with mitochondria DNA copy number and telomere length in maternal and cord blood. Environ Health Perspect. (2021) 129:117007. doi: 10.1289/EHP9294
57. Alegría-Torres JA, Perez-Rodriguez RY, Garcia-Torres L, Costilla-Salazar R, Rocha-Amador D. Exposure to arsenic and lead in children from Salamanca México, effects on telomeric lengthening and mitochondrial DNA. Environ Sci Pollut Res Int. (2020) 27:6420–8. doi: 10.1007/s11356-019-07108-4
58. Song L, Liu B, Zhang L, Wu M, Wang L, Cao Z, et al. Association of prenatal exposure to arsenic with newborn telomere length: results from a birth cohort study. Environ Res. (2019) 175:442–8. doi: 10.1016/j.envres.2019.05.042
59. Herlin M, Broberg K, Igra AM, Li H, Harari F, Vahter M, et al. Exploring telomere length in mother-newborn pairs in relation to exposure to multiple toxic metals and potential modifying effects by nutritional factors. BMC Med. (2019) 17:77. doi: 10.1186/s12916-019-1309-6
60. Mannan T, Ahmed S, Akhtar E, Ahsan KB, Haq A, Kippler M, et al. Associations of arsenic exposure with telomere length and naïve T cells in childhood-a birth cohort study. Toxicol Sci. (2018) 164:539–49. doi: 10.1093/toxsci/kfy105
61. Wai KM, Umezaki M, Kosaka S, Mar O, Umemura M, Fillman T, et al. Impact of prenatal heavy metal exposure on newborn leucocyte telomere length: a birth-cohort study. Environ Pollut. (2018) 243:1414–21. doi: 10.1016/j.envpol.2018.09.090
62. Chatterjee D, Adak S, Banerjee N, Bhattacharjee P, Bandyopadhyay AK, Giri AK, et al. Evaluation of health effects, genetic damage and telomere length in children exposed to arsenic in West Bengal, India. Mutat Res Genet Toxicol Environ Mutagen. (2018) 836:82–8. doi: 10.1016/j.mrgentox.2018.06.012
63. Fillman T, Shimizu-Furusawa H, Sheng Ng CF, Parajuli RP, Watanabe C. Association of cadmium and arsenic exposure with salivary telomere length in adolescents in Terai, Nepal. Environ Res. (2016) 149:8–14. doi: 10.1016/j.envres.2016.04.037
64. Vahter M. Mechanisms of arsenic biotransformation. Toxicology. (2002) 181–2:211–7. doi: 10.1016/S0300-483X(02)00285-8
65. Ameer SS, Xu Y, Engstrom K, Li H, Tallving P, Nermell B, et al. Exposure to inorganic arsenic is associated with increased mitochondrial DNA copy number and longer telomere length in peripheral blood. Front Cell Dev Biol. (2016) 4:87. doi: 10.3389/fcell.2016.00087
66. Lindberg AL, Rahman M, Persson L-A, Vahter M. The risk of arsenic induced skin lesions in Bangladeshi men and women is affected by arsenic metabolism and the age at first exposure. Toxicol Appl Pharmacol. (2008) 230:9–16. doi: 10.1016/j.taap.2008.02.001
67. Pierce BL, Tong L, Argos M, Gao J, Farzana J, Roy S, et al. Arsenic metabolism efficiency has a causal role in arsenic toxicity: mendelian randomization and gene-environment interaction. Int J Epidemiol. (2013) 42:1862–71. doi: 10.1093/ije/dyt182
68. Engström K, Vahter M, Mlakar SJ, Concha G, Nermell B, Raqib R, et al. Polymorphisms in arsenic(+III oxidation state) methyltransferase (AS3MT) predict gene expression of AS3MT as well as arsenic metabolism. Environ Health Perspect. (2011) 119:182–8. doi: 10.1289/ehp.1002471
69. Drobná Z, Del Razo LM, Garcia-Vargas GG, Sanchez-Pena LC, Barrera-Hernandez A, Styblo M, et al. Environmental exposure to arsenic, AS3MT polymorphism and prevalence of diabetes in Mexico. J Expo Sci Environ Epidemiol. (2013) 23:151–5. doi: 10.1038/jes.2012.103
70. Schlebusch CM, Gattepaille LM, Engstrom K, Vahter M, Jakobsson M, Broberg K, et al. Human adaptation to arsenic-rich environments. Mol Biol Evol. (2015) 32:1544–55. doi: 10.1093/molbev/msv046
Keywords: telomere, telomerase, arsenic, metal, trace element (TE)
Citation: Wai KM, Swe T, Myar MT, Aisyah CR and Hninn TSS (2023) Telomeres susceptibility to environmental arsenic exposure: Shortening or lengthening? Front. Public Health 10:1059248. doi: 10.3389/fpubh.2022.1059248
Received: 01 October 2022; Accepted: 19 December 2022;
Published: 10 January 2023.
Edited by:
Andrea Maugeri, University of Catania, ItalyReviewed by:
Joel Henrique Ellwanger, Federal University of Rio Grande do Sul, BrazilCopyright © 2023 Wai, Swe, Myar, Aisyah and Hninn. This is an open-access article distributed under the terms of the Creative Commons Attribution License (CC BY). The use, distribution or reproduction in other forums is permitted, provided the original author(s) and the copyright owner(s) are credited and that the original publication in this journal is cited, in accordance with accepted academic practice. No use, distribution or reproduction is permitted which does not comply with these terms.
*Correspondence: Kyi Mar Wai, kyimar@hirosaki-u.ac.jp