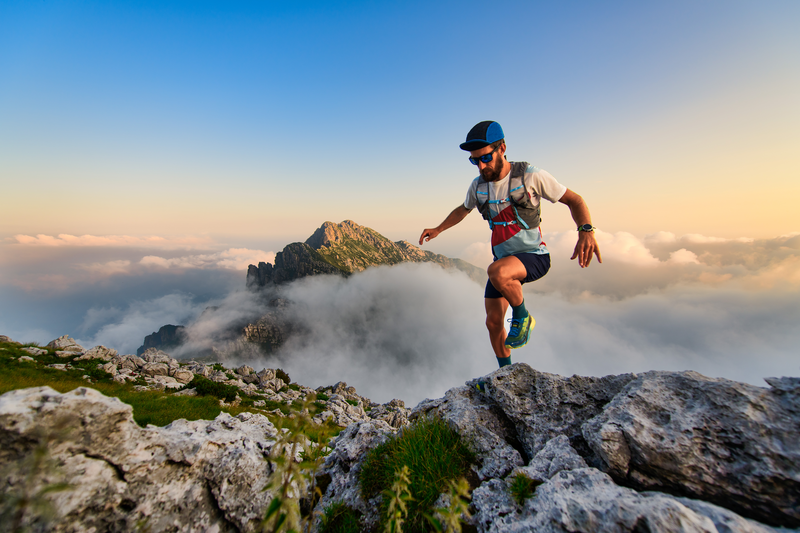
94% of researchers rate our articles as excellent or good
Learn more about the work of our research integrity team to safeguard the quality of each article we publish.
Find out more
ORIGINAL RESEARCH article
Front. Public Health , 12 August 2021
Sec. Infectious Diseases: Epidemiology and Prevention
Volume 9 - 2021 | https://doi.org/10.3389/fpubh.2021.707435
Acinetobacter baumannii is an important nosocomial pathogen, which is multidrug resistant (MDR). Acinetobacter baumannii has become a major threat to public health worldwide due to its ability to easily acquire resistant genes. In order to analyze its epidemiology characteristics and the genetic evolution, A. baumannii isolates obtained from a Chinese tertiary hospital in the past 12 years (2008–2019), 295 isolates of non-repetitive A. baumannii, were recovered from patients and wards environments. The resistance genes were analyzed using antimicrobial susceptibility testing. The genetic relatedness of 295 isolates was identified by multilocus sequence typing (MLST) and eBURST analysis. It was found that the antibiotic-resistant and carbapenemase-resistant genes of all the 295 MDR A. baumannii in the hospital have not changed significantly over the past 12 years; all of them were resistant to multiple antibiotics except the polymyxin E and tigecycline. The results of drug-resistant genes showed that the detection rates of carbapenemase-resistant genes blaOXA−23, blaTEM−1, and blaOXA−66 were 97.6, 75.3, and 71.9%, respectively, which were detected almost every year from 2008 to 2019. Additionally, 16s rRNA methylation enzyme gene armA, aminoglycoside-resistant gene ant(3")-I, and class I integrase gene could also have a high positive rate. By MLST, these isolates were assigned to 12 sequence types (STs), including ST369, ST208, ST195, ST191, ST368, ST530, ST469, ST451, ST229, ST381, ST543, and ST1176. eBURST analysis showed that 9 STs with ST208 as the founder genotype belonged to Group 1 except for ST229, ST530, and ST1176. Therefore, most MDR A. baumannii isolates had a relatively close genetic relationship. Notably, the predominant ST208 and ST369 at the early stage changed to ST451 in 2019, indicating that the complex and diverse genetic background of the prevalence of A. baumannii isolates in the hospital. Overall, further epidemiological surveillance and genetic evolution analysis of A. baumannii are required, which can provide new strategies for the prevention and control of A. baumannii infections.
Acinetobacter baumannii, a Gram-negative and non-fermentative bacterium, is an important opportunist pathogen in hospitals. It can cause a wide range of severe nosocomial infections, including ventilator-associated pneumonia, bloodstream infections, skin and soft tissue infections, wound infections, urinary tract infections, and meningitis (1).
Acinetobacter baumannii is found almost exclusively in the hospital environment; it can easily colonize the skin surface, respiratory tract, and digestive tract of patients (2). Moreover, another concern for A. baumannii is the drug resistance. A large number of studies showed that A. baumannii presents resistance to multiple antimicrobial agents, including carbapenems, and multidrug resistance (MDR) is very common (3). Recently, extensively drug-resistant (XDR) and pandrug-resistant (PDR) A. baumannii isolates have rapidly increased (4). Therefore, the World Health Organization (WHO) has assigned A. baumannii as a critical priority pathogen posing a great threat to public health, and toward which new antibiotics are urgently needed.
Although there are a good deal of studies on the outbreaks caused by A. baumannii worldwide, medical environments and drug and disinfection strategies for A. baumannii infection are different in different regions and hospitals, leading to the different selective pressures on this bacteria; as a result, there are certain differences in bacterial dominance types and resistance. In the present study, A. baumannii isolates over the past 12 years (2008–2019) have been analyzed for the molecular epidemiology and evolution characteristics in a tertiary hospital in Shandong province, China. The epidemiology analysis of A. baumannii is helpful for understanding its genetic variation and providing insights into the treatment and control of this bacterial infection.
The study was carried out in accordance with the approved guidelines of the Ethics Committee of Taian City Central Hospital with written informed consent from all subjects. All the subjects gave a written informed consent in accordance with the Declaration of Helsinki.
Two hundred and ninety-five isolates of non-repetitive A. baumannii were isolated from clinical samples collected during a routine checkup by medical professionals and the wards environments from October 2008 to October 2019. During the 12 years period, 195 isolates were recovered from the sputum, 86 isolates were from the wards environments, and 9, 3, and 2 isolates were from cerebrospinal fluid, wound, and urine, respectively. These samples were collected during a period when A. baumannii was relatively prevalent clinically. As for wards distribution, most isolates (64.7%) were collected from intensive care unit (ICU), and 29.2% isolates were from ICU environments. A small number of isolates was from other wards, including nephrology ward (NW), health care ward (HCW), and cardiology ward (Table 1). On the whole, 295 isolates were from patients (sputum, cerebrospinal fluid, and urine) and wards environments, including pillow slips, quilts, stethoscopes, infusion pumps, ventilators, monitors, nurse/doctor cuffs, wristbands, blood pressure monitors, bedside tables, and others used by patients. The detailed isolation information of MDR A. baumannii isolates from 2018 and 2019 are shown in Supplementary Table 1.
Bacterial identification was performed by WalkAway 96 PLUS-NC50 combo panel (Beckman, United States) following the instructions of the manufacturer. Antimicrobial susceptibility testing was performed by three different methods: the sensitivity of meropenem and cefotaxime was determined by the disk diffusion method, the sensitivity of tigecycline and polymyxin E was determined by the Etest method (AB Biodisk, Solna, Sweden), and the sensitivity of other antimicrobial agents was detected using the WalkAway 96 PLUS-NC50 combo panel. The criteria of the susceptibility of the PLUS-NC50 combo panel, polymyxin E, meropenem, and cefotaxime were adapted from the Clinical and Laboratory Standards Institute (CLSI; http://clsi.org/standards/). The criteria of the susceptibility of tigecycline were adapted from the U. S. Food and Drug Administration (http://www.fda.org.uk/sitemap.aspx).
Bacterial DNAs were extracted and the primers of the related resistance genes were designed as described previously (5–8), including carbapenemase-resistant genes blaOXA−23, blaOXA−24, blaOXA−48, blaOXA−50, blaOXA−58, blaOXA−60, blaOXA−66, blaOXA−197, blaKPC, blaTEM−1, blaNDM−1, and blaIMP−4; the 16s rRNA methylase-resistant genes armA; and the aminoglycoside-resistant genes ant(3")-I, aac(3)-I, aac(3)-II, aac(6′)-I, aac(6′)-II, and aph(3′)-VI. In addition, the detection primers of integrases and integron genes were designed and synthesized, the specific sequences are shown in Table 2.
Seven housekeeping genes (gltA, gyrB, gdhB, recA, cpn60, gpi, rpoD) were amplified and sequenced to determine the genotypes of all isolates. DNA sequence variations and sequence types (STs) were analyzed using the multilocus sequence typing (MLST) database for A. baumannii (http://pubmlst.org/abaumannii). MLST was performed using the Oxford scheme as previously described (9), and eBURST method was used for the analysis of the novel alleles and genetic evolution (http://www.phyloviz.net/goeburst/).
The sensitivity of all A. baumannii isolates recovered from 2008 to 2019 to 15 antimicrobial agents was shown in Table 3, all of them exhibited an MDR phenotype, being resistant to three or more classes of antibiotics, such as amikacin, gentamicin, ceftazidime, ceftriaxone, piperacillin/tazobactam, imipenem, meropenem, levofloxacin, and ciprofloxacin, and the resistance of isolates to these drugs has not changed much over the past 12 years. However, all 295 MDR A. baumannii isolates were sensitive to polymyxin E, indicating that this antibiotic had a good therapeutic effect on A. baumannii in the hospital. In addition, these isolates were highly sensitive to tigecycline, although the sensitivity declined during the years 2010–2013 (59.5–60.9%) and 2015–2016 (74.5%).
Table 3. The antimicrobial susceptibility testing of A. baumannii isolates to 15 antibiotics over the past 12 years.
As many as 288 isolates (97.6%) carried blaOXA−23 gene, followed by blaTEM−1 (75.3%) and blaOXA−66 (71.9%) genes, which were detected almost every year from 2008 to 2019. The drug-resistant gene blaOXA−197 was detected in 28 isolates in 2014, blaNDM−1and blaIMP−4 genes were only detected in 2010–2011, the corresponding numbers of isolates were 1 and 6, respectively (Table 4). Other carbapenemase genes were not detected in any of these isolates. The 16s rRNA methylation enzymes gene armA can be detected every year, and the total 250 isolates carried this resistant gene over the past 12 years (Table 5). The aminoglycoside-resistant gene ant(3")-I can also be detected every year, and the number of A. baumannii isolates harboring this gene was the most, accounting for 88.1%, followed by aac(6")-I (41.7%) and aac(3)-I (32.2%). However, all A. baumannii isolates carrying aac(3)-I gene appeared before 2016, and aac(6")-I was predominant in A. baumannii isolates from 2017 to 2019 (77.2%) (Table 6).
Furthermore, Classes I, II, and III integrase genes of several MDR A. baumannii isolates from 2014 to 2019 (197 isolates) were detected. The results showed that 170 isolates carried Class I integrase gene (Intl1), with a positive rate of 86.3%, but none of them contained Classes II and III integrase genes. The PCR-positive products of eight isolates of Class I integrase gene were sequenced and confirmed its accuracy. Additionally, we further detected the integron variable regions of some MDR A. baumannii isolates that were positive for class I integrase genes. It was found that there were no drug-resistant genes in the 500, 750, and 1,000 bp segments, while the 1,500–2,200 bp segments contained aacC1, aadA1, aacA4, catB8, and arr3 genes. Among them, aacA4 and aadA1 were aminoglycoside-resistant genes, and catB8 was a chloramphenicol-resistant gene. These results indicated that the Class I integrase genes of MDR A. baumannii isolates from this hospital may mainly mediate aminoglycoside and chloramphenicol resistance.
A total of 12 STs were detected for the 295 MDR A. baumannii isolates by MLST molecular typing, including ST369, ST208, ST195, ST191, ST368, ST530, ST469, ST451, ST229, ST381, ST540, and ST1176. As shown in Table 7, ST208 was predominant in all A. baumannii isolates (30.8%); then ST451 (27.1%), ST369 (17.6%), ST195 (12.9%), and ST368 (6.1%); and the remaining STs were few, with 1–6 isolates. Notably, the STs of all A. baumannii isolates from 2008 to 2019 showed a certain change. In 2010–2016, the number of ST208 was highest (44.8%, 87/194) and the isolation rate of ST208 in this period accounted for 95.6% (87/91) of the total ST208 in 12 years, especially it was predominant in 2010–2013. During 2014–2016, the prevalence of ST369 gradually increased. In 2017–2018, ST195 was the dominant ST in the hospital, but in 2019, ST451 was predominant. These results demonstrated that the predominant STs of A. baumannii isolates are different in different periods in the hospital, indicating the diverse and complicated genetic background of A. baumannii isolates in the hospital.
In order to analyze the genetic evolution of 295 MDR A. baumannii isolates, eBURST method was performed. As was shown in Figure 1, these 12 STs could be divided into three groups. ST369, ST208, ST195, ST191, ST368, ST469, ST451, ST381, and ST540 belonged to Group 1, with the founder genotype ST208. ST229 belonged to Group 2, and ST530 and ST1176 constituted Group 3. Therefore, most MDR A. baumannii isolates obtained in this study were of Group 1, and they had relatively close genetic relationship. Moreover, some representative isolates were selected for pulse field gel electrophoresis analysis (Supplementary Figure 1), and it was found that MDR A. baumannii isolates with the same ST and obtained from the same year were not exactly the same clone, indicating the relative complexity of prevalent MDR A. baumannii isolates in this hospital.
Figure 1. eBURST analysis of 295 MDR Acinetobacter baumannii isolates. It was found that these 12 STs could be divided into three groups. ST369, ST208, ST195, ST191, ST368, ST469, ST451, ST381, and ST540 belonged to Group 1, and ST208 was the central type. ST229 belonged to Group 2, and ST530 and ST1176 constituted Group 3.
Acinetobacter baumannii has recently been considered the most critical pathogen for posing a great threat to public health. In order to systematically summarize and investigate the prevalence and genetic evolution of A. baumannii and prevent the outbreak and patient-infections caused by MDR A. baumannii, we retrospectively analyzed the molecular epidemiology characteristics of 295 A. baumannii isolates in a tertiary teaching hospital for 12 years (2008–2019), including drug resistance, drug-resistant genes, and STs. In the present study, 295 A. baumannii isolates were mainly recovered from sputum, cerebrospinal fluid, and ICU wards environments, among which 195 isolates were from sputum and 86 were from ICU wards environments. As for the distribution of wards, 295 isolates were mainly from ICU (277), including ICU environments. Other wards, such as NW and HCW, had a few isolates. These results demonstrated that ICU was always the ward with the most serious nosocomial infection of A. baumannii (10–12), indicating that regular disinfection of the ICU and air environment is necessary.
The emergence of MDR A. baumannii has brought great challenges to clinical treatment. Fifteen antibiotics were used for susceptibility testing in this study, and the result showed that all A. baumannii isolates exhibit MDR phenotypes. In terms of time axis, there was no significant change in the resistance of MDR A. baumannii in the hospital over the 12 years from 2008 to 2019. Generally, except for tigecycline and polymyxin E, these isolates were almost resistant to all available antimicrobial agents, including imipenem and meropenem. The main mechanism of carbapenem resistance in MDR A. baumannii is the acquisition of carbapenem-hydrolyzing oxacillinase-encoding genes. Of these, blaOXA−23 carbapenemase-resistant gene was by far the most widespread in most countries (13), and it was found that blaOXA−23-producing A. baumannii isolates disseminated widely in China or Asian (14–16). In our study, the detection of resistant genes showed that the most common carbapenemase-resistant gene was blaOXA−23 (288 isolates) in all A. baumannii isolates, followed by blaOXA−66 (212), which nearly could be detected in all periods except 2015–2016, indicating that these two drug-resistant genes might be the main reason for bacterial carbapenem resistance. Additionally, blaTEM−1 is the most largely known and classic β-lactamase. It was reported that the expression of blaTEM−1 β-lactamase positively correlated with the minimum inhibitory concentration of sulbactam, and transfer of the blaTEM-1 gene into a susceptible A. baumannii strain resulted in resistance (17). Recently, Yang et al. collected 2,197 A. baumannii isolates from 27 provinces in China, found that the resistance rate for cefoperazone–sulbactam was 39.7%, and demonstrated that blaTEM−1 with four tandem copies structure played a key role in this resistance phenomenon (18). Meanwhile, Han et al. proved that blaOXA−23and blaTEM−1genes were more conducive to resistance to carbapenems in A. baumannii (19). In the current study, blaTEM−1 gene had been detected in all isolates since 2012, and the overall positive rate of this resistance gene was 75.3% (222/295), meanwhile, the cefoperazone–sulbactam resistance rate of the 295 MDR A. baumannii isolates was up to 94.5%, and both had a certain correlation relationship. Further genome sequence analysis of ST451 in 2019, there was only one copy of TEM-1 in the MDR A. baumannii isolates in the study, indicating the specific molecular mechanism of the resistance of A. baumannii to cefoperazone–sulbactam might be diversity. As an important resistance gene of A. baumannii, only one isolate carried blaNDM−1 in 2010–2011, however, given its distribution worldwide, enhanced monitoring is needed (20, 21). In addition, A. baumannii isolates carried several aminoglycoside-resistant genes and gene for 16s rRNA methylation enzymes, of which ant(3")-I and armA could be detected every year. Overall, these drug-resistant genes should be further monitored to understand their structure and analyze the characteristics of drug resistance.
It has been reported that ST208 and ST195 were the predominant epidemic types of MDR A. baumannii in China (22, 23). In this study, the STs of A. baumannii isolates have undergone a significant change over the past 12 years, the epidemic STs changed from ST208 and ST369 at the early stage (2008–2016) to the predominant ST451 in 2019 after the transition of ST195 (2017–2018). Lee et al. reported that the outbreak of XDR A. baumannii ST451 carrying MDR genes occurred in South Korea (24). Moreover, there have been outbreaks of A. baumannii ST451 in countries around China in recent years, such as Thailand and India (25–27), and ST451 was also isolated from patients with bloodstream infection, causing a certain mortality (28), so the prevalence of A. baumannii ST451 requires more attention. In order to understand the molecular characteristic and the transmission route of ST451 outbreak, one ST451 (Ab1) isolated from the environment and another ST451 (Ab2) from a patient in 2019 were selected for the genome sequence (Supplementary Figure 2). The results showed that the average nucleotide identity of both isolates was >99.9%, indicating that the ST451 MDR A. baumannii that caused an outbreak in 2019 were the same clone. Therefore, although there were 59 ST451 A. baumannii isolates, all isolates may be the same clone. Moreover, the STs of MDR A. baumannii isolates changed, but the antibiotic susceptibility of ST451 isolates did not change significantly, indicating that there was no significant relationship between the STs of the isolates and drug resistance. We thought that the drug-resistant phenotypes of bacteria were mainly related to the drug-resistant genes. According to eBURST analysis, ST451 and ST208 belonged to Group 1, and they had a close genetic relationship. The detection also showed that the drug-resistant genes carried by ST451 were similar to those carried by ST195 and ST208 earlier. The prevention and control strategy adopted by the hospital was to remove all patients from the wards for disinfection, and to disinfect or replace the medical supplies used by the patients. Moreover, we also found that MDR A. baumannii–infected patients with respiratory tract infection were easy to pollute the surrounding environments. When the bed sheets and pillowcases of patients were seriously polluted, MDR A. baumannii could be detected within 1.5 m when patients turned over. Therefore, the safe distance between patients should be increased to 3 m as far as possible to prevent cross-infection.
On the whole, the resistance and resistant genes of the prevalent MDR A. baumannii isolates in the hospital have not changed significantly over the past 12 years. However, almost all MDR A. baumannii isolates carried multiple antibiotic-resistant genes, and the predominant MDR A. baumannii isolates have evolved from ST208 and ST369 to ST451. Therefore, it is of great significance to further strengthen the epidemiological surveillance of A. baumannii, analyze its genetic evolution, and provide new strategies for the prevention and control of nosocomial infections caused by MDR A. baumannii.
The original contributions presented in the study are included in the article/Supplementary Material, further inquiries can be directed to the corresponding authors.
The studies involving human participants were reviewed and approved by The Ethics Committee of Taian City Central Hospital. The patients/participants provided their written informed consent to participate in this study. All the subjects gave a written informed consent in accordance with the Declaration of Helsinki.
MJ and XC performed the main experiments, analyzed data, and wrote the manuscript. SL, ZZ, NL, CD, and LZ performed the experiment and analyzed data. HW and SZ reviewed the manuscript and approved it. All authors contributed to the article and approved the submitted version.
This research was supported by the Natural Science Foundation of Shandong Province (Nos. ZR2016HL44 and ZR2013HM009).
The authors declare that the research was conducted in the absence of any commercial or financial relationships that could be construed as a potential conflict of interest.
All claims expressed in this article are solely those of the authors and do not necessarily represent those of their affiliated organizations, or those of the publisher, the editors and the reviewers. Any product that may be evaluated in this article, or claim that may be made by its manufacturer, is not guaranteed or endorsed by the publisher.
The Supplementary Material for this article can be found online at: https://www.frontiersin.org/articles/10.3389/fpubh.2021.707435/full#supplementary-material
1. Moubareck CA, Halat DH. Insights into Acinetobacter baumannii: a review of microbiological, virulence, and resistance traits in a threatening nosocomial pathogen. Antibiotics (Basel). (2020) 9:119. doi: 10.3390/antibiotics9030119
2. Cerqueira GM, Peleg AY. Insights into Acinetobacter baumannii pathogenicity. IUBMB Life. (2011) 63:1055–60. doi: 10.1002/iub.533
3. Nasr P. Genetics, epidemiology, and clinical manifestations of multidrug-resistant Acinetobacter baumannii. J Hosp Infect. (2020) 104:4–11. doi: 10.1016/j.jhin.2019.09.021
4. Teerawattanapong N, Panich P, Kulpokin D, Ranong SN, Kongpakwattana K, Saksinanon A, et al. A systematic review of the burden of multidrug-resistant healthcare-associated infections among intensive care unit patients in Southeast Asia: the rise of multidrug-resistant Acinetobacter baumannii. Infect Control Hosp Epidemiol. (2018) 39:525–33. doi: 10.1017/ice.2018.58
5. Shen JL, Zhu DM, Wu WH, Xu XG, Wang MG The relationship between acquired carbapenemases resistance of gram-negative bacilli (in Chinese). Chin J Lab Med. (2008) 4:408–14. doi: 10.3321/j.issn:1009-9158
6. Yang XL, Chen MH, Liu Y, Deng Q, Guo F. Related drug resistance mechanism of New Delhi metallo-b-lactamase-producing pandrug-reistant Acinetobacter baumannii analysis of homology (in Chinese). Chin J Nosocomiol. (2015) 25:5049–52. doi: 10.11816/cn.ni2015-144658
7. Zhi ZQ, He ZH, Jiang P, Ma JG, Jiang GT, Xu HO, et al. The study on genotyping of β-lactamases aminoglycoside-modifying enzyme in multi-resistant Pseudomonas aeruginosa (in Chinese). Chin J Lab Med. (2005) 28:1211–4. doi: 10.3760/j:issn:1009-9158.2005.11.031
8. Jia ZY, He BH, Wang YT, Wang L, Chu HN, Li L, et al. Detection of blaNDM−1 gene drug resistance of three Enterobactercloacae strains isolated in Hebei province (in Chinese). Chin J Public Health. (2014) 30:524–7. doi: 10.11847/zgggws2014-30-04-47
9. Bartual SG, Seifert H, Hippler C, Luzon MAD, Wisplinghoff H, Rodríguez-Valera F. Development of a multilocus sequence typing scheme for characterization of clinical isolates of Acinetobacter baumannii. J Clin Microbiol. (2005) 43:4382–90. doi: 10.1128/JCM.43.9.4382-4390.2005
10. Gong YL, Shen XD, Huang GT, Zhang C, Luo XQ, Yin SP, et al. Epidemiology and resistance features of Acinetobacter baumannii isolates from the ward environment and patients in the burn ICU of a Chinese hospital. J Microbiol. (2016) 54:551–8. doi: 10.1007/s12275-016-6146-0
11. Zhao YX, Hu KW, Zhang JS, Guo YH, Fan XC, Wang Y, et al. Outbreak of carbapenem-resistant Acinetobacter baumannii carrying the carbapenemase OXA-23 in ICU of the eastern Heilongjiang Province, China. BMC Infect Dis. (2019) 19:452. doi: 10.1186/s12879-019-4073-5
12. Raro OHF, Gallo SW, Ferreira CAS, Oliveira SD. Carbapenem-resistant Acinetobacter baumannii contamination in an intensive care unit. Rev Soc Bras Med Trop. (2017) 50:167–72. doi: 10.1590/0037-8682-0329-2016
13. Hamidian M, Nigro SJ. Emergence, molecular mechanisms and global spread of carbapenem-resistant Acinetobacter baumannii. Microb Genom. (2019) 5:e000306. doi: 10.1099/mgen.0.000306
14. Jeon H, Kim S, Kim MH, Kim SY, Nam D, Park SC, et al. Molecular epidemiology of carbapenem-resistant Acinetobacter baumannii isolates from a Korean hospital that carry bla(OXA-23). Infect Genet Evol. (2018) 58:232–6. doi: 10.1016/j.meegid.2018.01.003
15. Wang X, Du ZY, Huang WC, Zhang XY, Zhou Y Outbreak of multidrug-resistant Acinetobacter baumannii ST208 producing OXA-23-like carbapenemase in a children's hospital in Shanghai China. Microb Drug Resist. (2021) 27:816–22. doi: 10.1089/mdr.2019.0232
16. Ning NZ, Liu X, Bao CM, Chen SM, Cui EB, Zhang JL, et al. Molecular epidemiology of bla (OXA-23) -producing carbapenem-resistant Acinetobacter baumannii in a single institution over a 65-month period in north China. BMC Infect Dis. (2017) 17:14. doi: 10.1186/s12879-016-2110-1
17. Krizova L, Poirel L, Nordmann P, Nemec A. TEM-1 β-lactamase as a source of resistance to sulbactam in clinical strains of Acinetobacter baumannii. J Antimicrob Chemother. (2013) 68:2786–91. doi: 10.1093/jac/dkt275
18. Yang YX, Fu Y, Lan P, Xu QY, Jiang Y, Chen Y, et al. Molecular epidemiology and mechanism of sulbactam resistance in Acinetobacter baumannii isolates with diverse genetic backgrounds in China. Antimicrob Agents Chemother. (2018) 62:e01947–17. doi: 10.1128/AAC.01947-17
19. Han L, Lei J, Xu JR, Han SS. blaOXA-23-like and blaTEM rather than blaOXA-51-like contributed to a high level of carbapenem resistance in Acinetobacter baumannii strains from a teaching hospital in Xi'an, China. Medicine (Baltimore). (2017) 96:e8965. doi: 10.1097/MD.0000000000008965
20. Maamar E, Alonso CA, Ferjani S, Jendoubi A, Hamzaoui Z, Jebri A, et al. NDM-1- and OXA-23-producing Acinetobacter baumannii isolated from intensive care unit patients in Tunisia. Int J Antimicrob Agents. (2018) 52:910–5. doi: 10.1016/j.ijantimicag.2018.04.008
21. Moubareck CA, Halat DH, Nabi A, AlSharhan MA, AlDeesi ZO, Han A, et al. Detection of OXA-23, GES-11 and NDM-1 among carbapenem-resistant Acinetobacter baumannii in Dubai: a preliminary study. J Glob Antimicrob Resist. (2021) 24:27–8. doi: 10.1016/j.jgar.2020.11.016
22. Qu JY, Du Y, Yu RJ, Lü XJ. The first outbreak caused by Acinetobacter baumannii ST208 and ST195 in China. Biomed Res Int. (2016) 2016:9254907. doi: 10.1155/2016/9254907
23. Jiang LL, Liang YC, Yao W, Ai JT, Wang X, Zhao ZG. Molecular epidemiology and genetic characterisation of carbapenem-resistant Acinetobacter baumannii isolates from Guangdong Province, South China. J Glob Antimicrob Resist. (2019) 17:84–9. doi: 10.1016/j.jgar.2018.11.002
24. Lee SY, Oh MH, Yun SH, Choi CW, Changkyun Park E, Song HS, et al. Genomic characterization of extensively drug-resistant Acinetobacter baumannii strain, KAB03 belonging to ST451 from Korea. Infect Genet Evol. (2018) 65:150–8. doi: 10.1016/j.meegid.2018.07.030
25. Kumar S, Patil PP, Midha S, Ray P, Patil PB, Gautam V. Genome sequence of Acinetobacter baumannii strain 10441_14 belonging to ST451, isolated from India. Genome Announc. (2015) 3:e01322–15. doi: 10.1128/genomeA.01322-15
26. Vijayakumar S, Anandan S, Prabaa MD, Kanthan K, Vijayabaskar S, Kapil A, et al. Insertion sequences sequence types profile of clinical isolates of carbapenem-resistant A. baumannii collected across India over four year period. J Infect Public Health. (2020) 13:1022–8. doi: 10.1016/j.jiph.2019.11.018
27. Kongthai P, Thummeepak R, Leungtongkam U, Pooarlai R, Kitti T, Thanwisai A, et al. Insight into molecular epidemiology, antimicrobial resistance, and virulence genes of extensively drug-resistant Acinetobacter baumannii in Thailand. Microb Drug Resist. (2021) 27:350–9. doi: 10.1089/mdr.2020.0064
Keywords: multidrug-resistant Acinetobacter baumannii, resistance genes, sequence types, molecular epidemiology, genetic evolution
Citation: Jiang M, Chen X, Liu S, Zhang Z, Li N, Dong C, Zhang L, Wu H and Zhao S (2021) Epidemiological Analysis of Multidrug-Resistant Acinetobacter baumannii Isolates in a Tertiary Hospital Over a 12-Year Period in China. Front. Public Health 9:707435. doi: 10.3389/fpubh.2021.707435
Received: 10 May 2021; Accepted: 08 July 2021;
Published: 12 August 2021.
Edited by:
Jessica L. Jones, United States Food and Drug Administration, United StatesReviewed by:
Miguel Angel Cevallos, National Autonomous University of Mexico, MexicoCopyright © 2021 Jiang, Chen, Liu, Zhang, Li, Dong, Zhang, Wu and Zhao. This is an open-access article distributed under the terms of the Creative Commons Attribution License (CC BY). The use, distribution or reproduction in other forums is permitted, provided the original author(s) and the copyright owner(s) are credited and that the original publication in this journal is cited, in accordance with accepted academic practice. No use, distribution or reproduction is permitted which does not comply with these terms.
*Correspondence: Haiyan Wu, cWlhbmd0b3VjYW8xOTc2QDEyNi5jb20=; Shuping Zhao, ZGN6aHNocEAxMjYuY29t
†These authors share first authorship
Disclaimer: All claims expressed in this article are solely those of the authors and do not necessarily represent those of their affiliated organizations, or those of the publisher, the editors and the reviewers. Any product that may be evaluated in this article or claim that may be made by its manufacturer is not guaranteed or endorsed by the publisher.
Research integrity at Frontiers
Learn more about the work of our research integrity team to safeguard the quality of each article we publish.