- 1Department of Plastic Surgery, Zhongnan Hospital of Wuhan University, Wuhan, China
- 2Department of Thyroid and Breast Surgery, Zhongnan Hospital of Wuhan University, Wuhan, China
- 3Department of Rheumatology and Immunology, Tongji Medical College, Tongji Hospital, Huazhong University of Science and Technology, Wuhan, China
- 4Department of Neurosurgery, Zhongnan Hospital of Wuhan University, Wuhan, China
Background: Six months since the outbreak of coronavirus disease (COVID-19), the pandemic continues to grow worldwide, although the outbreak in Wuhan, the worst-hit area, has been controlled. Thus, based on the clinical experience in Wuhan, we hypothesized that there is a relationship between the patient's CO2 levels and prognosis.
Methods: COVID-19 patients' information was retrospectively collected from medical records at the Leishenshan Hospital, Wuhan. Logistic and Cox regression analyses were conducted to determine the correlation between decreased CO2 levels and disease severity or mortality risk. The Kaplan-Meier curve analysis was coupled with the log-rank test to understand COVID-19 progression in patients with decreased CO2 levels. Curve fitting was used to confirm the correlation between computed tomography scores and CO2 levels.
Results: Cox regression analysis showed that the mortality risk of COVID-19 patients correlated with decreased CO2 levels. The adjusted hazard ratios for decreased CO2 levels in COVID-19 patients were 8.710 [95% confidence interval (CI): 2.773–27.365, P < 0.001], and 4.754 (95% CI: 1.380–16.370, P = 0.013). The adjusted odds ratio was 0.950 (95% CI: 0.431–2.094, P = 0.900). The Kaplan-Meier survival curves demonstrated that patients with decreased CO2 levels had a higher risk of mortality.
Conclusions: Decreased CO2 levels increased the mortality risk of COVID-19 patients, which might be caused by hyperventilation during mechanical ventilation. This finding provides important insights for clinical treatment recommendations.
Background
In December 2019, an outbreak of pneumonia of unknown etiology was reported in Wuhan, China, which then rapidly evolved into a pandemic (1). By January 7, 2020, Chinese scientists had rapidly isolated the novel coronavirus, the severe acute respiratory syndrome coronavirus 2 (SARS-CoV-2), with an incubation period of 2–14 days, and a potential asymptomatic human-to-human transmission; it is known to cause the coronavirus disease (COVID-19) (2–4). COVID-19 has been controlled in China, although the global number of infections continues to grow rapidly and has led to more than five million infections and 630,000 deaths (5).
In COVID-19 patients, fever and cough are the most common symptoms. There may also be uncommon symptoms, such as diarrhea (6). Thus, researchers have found that SARS-CoV-2 affects multiple organs in addition to the patients' lungs, based on the understanding garnered from COVID-19 studies. This explains the pathological changes identified from the minimal autopsies of three patients who died of COVID-19 in Chongqin, China (7–9). Studies have shown that the main targeted organs of SARS-CoV-2 are the lungs and airways. Furthermore, damage to other organs significantly increases the mortality rate of COVID-19 patients (10).
The measurement of carbon dioxide (CO2) level in blood is vital not only for the early detection of respiratory depression and airway disorders but also for airway management (11). Hypoxemia and hypercapnia predicted poor prognosis for COVID-19 patients in a previous study (4). Hence, this study aimed to investigate whether decreased CO2 levels would influence the prognosis of COVID-19 patients.
Methods
Study Design and Participants
In this retrospective study, we collected data from 1,880 patients, who were clinically diagnosed with COVID-19 between February 8, 2020, and March 19, 2020, at Wuhan Leishenshan Hospital. Exclusion criteria included missing data on mortality and CO2 level, pregnancy, death on admission, embolization, and transfer to any other hospital; thus, 1,776 patients were included finally. Data about demographics, medical history, treatment, laboratory findings, and imaging data were collected from the patients' original medical records. Two physicians independently reviewed these data.
This study was approved by the Research Ethics Commission of the Zhongnan Hospital of Wuhan University (approval number: 2020074). The need for patient consent was waived by the ethics committee because of the urgent need for insights into this rapidly evolving infectious disease.
Primary Outcomes in This Study
In this study, the survival and illness severity of COVID-19 patients during hospitalization and images obtained from computed tomography (CT) scan were used to evaluate the patients' primary outcomes. However, survival was the most significant indicator. According to the Seventh Interim Guidance of Diagnosis and Treatment of COVID-19 published by the Chinese National Health Commission, one patient was staged into mild COVID-19 in this study. Thus, the severity of COVID-19 was categorized into three degrees: mild/common, severe, and critical.
Furthermore, after fulfilling the common standard criteria, all chest CT images were inspected and independently categorized by two experienced radiologists using the following scoring system according to previous studies and the characteristics of COVID-19. Score 1 included ground-glass opacities (GGO) characteristics, reticulation or cord change, consolidation, and pleural effusion, in which each feature was assigned one point, and Score 1 was the sum of these features. Score 2 (from 0 to 4 points) was generated depending on the area of involvement of the lung lobes as follows: no involvement, 0; < 25% involvement, 1; 26–50% involvement, 2; 51–75% involvement, 3; 76–100% involvement, 4; the total score was the sum of scores 1 and 2.
Statistical Analyses
Continuous variables with normal distribution are presented as mean ± standard deviation (SD) or median and interquartile range (IQR). A CO2 level ≤23 mmol/L was considered a decreased level (normal CO2 range: 23–31 mmol/L). Furthermore, differences in continuous variables between the groups (decreased and non-decreased levels of CO2), were determined using independent group t-test or the Mann-Whitney U-test. Categorical variables are presented as frequencies and percentages. For the proportions of categorical variables, the chi-square test was used to compare participants with decreased and non-decreased CO2 levels. When parameters were expected to have a count ≤5, the Fisher exact test was used.
To determine whether the decreased CO2 levels would influence the prognosis of COVID-19 patients, we used Cox regression analysis, after adjusting for age, history of cardiovascular disease, erythrocyte count, hemoglobin, leucocyte count, platelet count, lymphocyte count, and oxygen support. Furthermore, Kaplan-Meier analyses with log-rank tests were used to analyze the survival trends of patients.
All statistical analyses were performed using SPSS (version 23.0 for Windows) and EmpowerStats (version 2.0). A two-sided P ≤ 0.05 was considered statistically significant.
Results
Demographics, Clinical Information, and Laboratory Findings
The demographic characteristics and symptoms of this study cohort of 1,776 patients are presented in Table 1. The ratio of female to male patients was approximately one. The IQR value of age in this study population was 59 (48–68) years, with no apparent differences in the groups with decreased and non-decreased CO2 levels.
In patients with cardiovascular comorbidity, a significant difference was observed between decreased and non-decreased CO2 levels. However, there were no significant differences in other comorbidities, including pulmonary disease, endocrine disease, malignancy, and neurological disorders. Furthermore, among COVID-19 patients with decreased or non-decreased CO2 levels, those with gastrointestinal disorders showed a significant difference. However, concerning fever, fatigue, or respiratory and neurological symptoms, there were no significant intergroup differences (Table 1).
We analyzed the laboratory results and the blood coagulation tests of patients in two groups (Table 2), and most of the laboratory indicators showed significant differences. The results of the blood coagulation test, except fibrinogen and thrombin time, showed significant intergroup differences among COVID-19 patients. The clinical treatment and outcomes are presented in Table 2. Anticoagulants and types of oxygen support significantly differed among patients in the two groups. However, the use of antiviral drugs, corticosteroids, and traditional Chinese medicine showed no significant differences between the groups. Concerning outcomes, disease progression showed a significant difference, with no significant difference in other outcome parameters.
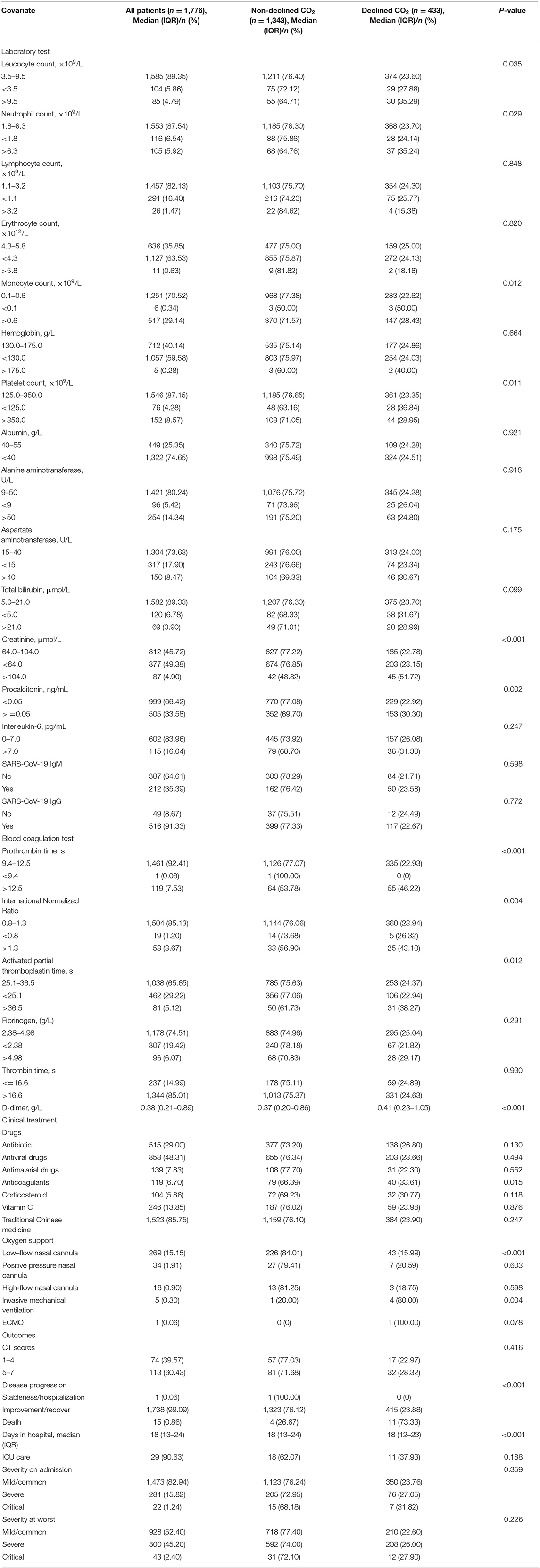
Table 2. Laboratory and blood coagulation test results, clinical treatment, and outcomes of 1,776 patients with COVID-19.
Analysis of Patient Prognosis
Table 3 shows the mortality risk of COVID-19 patients with decreased and non-decreased CO2 levels. Both unadjusted and adjusted Cox regression analyses showed that decreased CO2 levels were associated, with poor prognosis compared to non-decreased CO2 levels. After adjustment for age, history of cardiovascular disease, WBC, PLT, oxygen support, and lymphocyte count, the odds ratio for decreased CO2 levels in COVID-19 patients were 4.754 [95% confidence interval (CI): 1.380–16.370, P = 0.013]. The hazard ratio for decreased CO2 levels in COVID-19 patients was 8.710 (95% CI: 2.773–27.365, P < 0.001), and 4.754 (95% CI: 1.380–16.370, P = 0.013) after adjustment. Furthermore, the Kaplan-Meier curves illustrated that patients with decreased CO2 levels faced higher mortality risks (Figure 1). With the fitted curves, though, in Figure 2A, the curves of patients with non-decreased CO2 levels showed a slight downward trend, the CO2 levels of most patients were increased (Figures 2B–F).
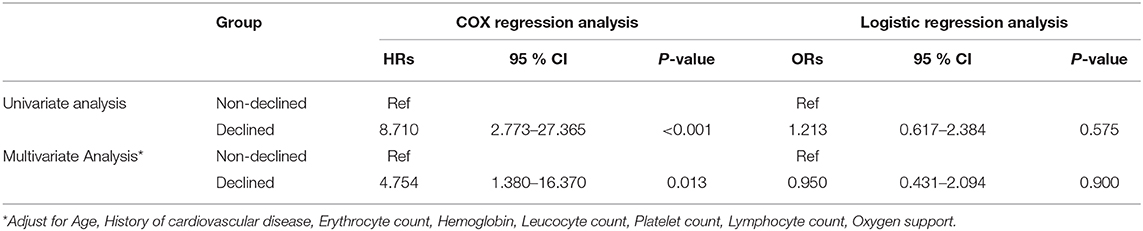
Table 3. The hazards ratio and odds ratio associated with decreased CO2 of patients with COVID-19 mortality/severity.
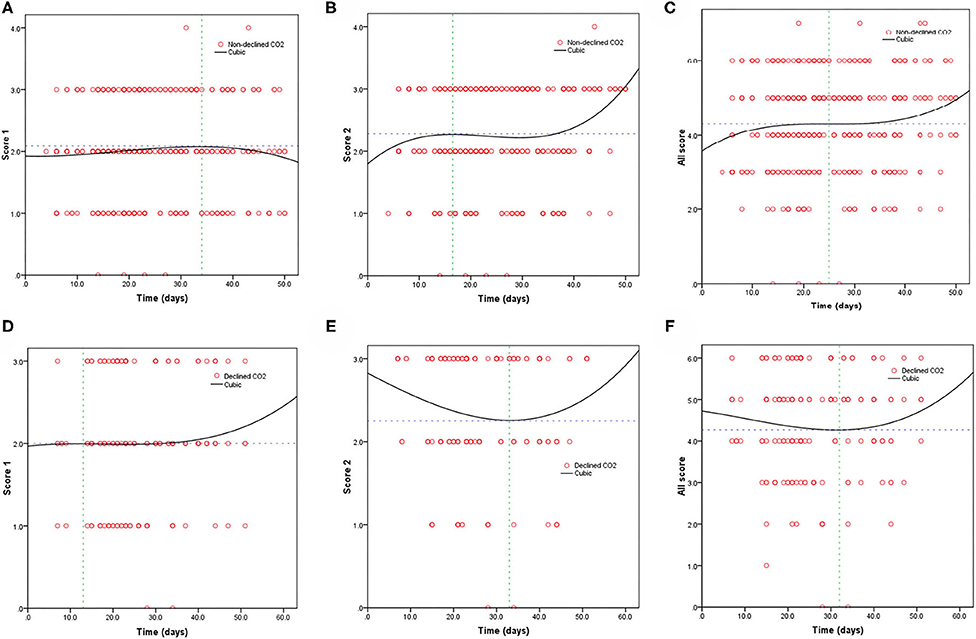
Figure 2. Fitting curves of patients with COVID-19 divided by declined/non-declined levels of CO2 based on CT score. Dynamic changes in patients with (A) CT score 1 and non-declined CO2; (B) CT score 2 and non-declined CO2; (C) total CT score and non-declined CO2; (D) CT score 1 and declined CO2; (E) CT score 2 and declined CO2; and (F) total CT score and declined CO2.
Discussion
In this latest outbreak of pneumonia due to COVID-19, patients initially presented with fever with or without respiratory symptoms, although various degrees of pulmonary abnormalities developed later in all patients (1, 12). Furthermore, Tian et al. reported the early phase of the lung pathology of COVID-19 pneumonia in a lung cancer excision, which exhibited edema, proteinaceous exudate, focal reactive hyperplasia of pneumocytes with patchy inflammatory cellular infiltration, and multinucleated giant cells. However, hyaline membranes were not prominent (13). A report demonstrated that the rate of critical illnesses among COVID-19 patients was ~26%, and critically ill patients had 61.5% mortality (12, 14). In another study from Wuhan, the 28-day mortality of COVID-19 patients who received mechanical ventilation (MV) was 81%, and patients with the acute respiratory distress syndrome (ARDS) had a mortality rate of nearly 50% (14, 15). Thus, it is undisputed that the lungs and airways are the target organs of this coronavirus infection.
In this study, we first proposed the correlation of patient prognosis with decreased CO2 levels. According to the adjusted logistic regression, Cox regression analyses, and Kaplan-Meier curves, decreased CO2 levels influenced the mortality of patients with COVID-19, but not disease severity. Furthermore, this influence on mortality did not differ by sex. However, decreased CO2 levels in patients with comorbidity of cardiovascular disease or older age indicated poorer prognosis. Moreover, blood coagulation parameters, such as prothrombin time, international normalized ratio, active partial thromboplastin time, and D-dimer level, showed significant differences between COVID-19 patients with decreased and non-decreased CO2 levels; however, decreased CO2 levels showed no significant differences in other laboratory parameters.
The measurement of the CO2 level is vital in airway management. Capnography is an effective method for the early detection of impaired airway function to identify early respiratory depression and airway disorders (16–18). For example, capnography presented results range 5–240 s earlier than dose pulse oximetry, and in many cases with sedation-induced apnea, doctors at the bedside did not recognize the apnea, whereas capnography could identify it (19, 20). Furthermore, capnography reduces serious complications by early diagnosis (16) and plays a critical role in detecting the CO2 level of COVID-19 patients, in whom the target organs are the lungs and airways.
Elevated CO2 levels and hypoxemia were associated with a poor prognosis in COVID-19 patients. For example, in the study conducted by Nuckton et al. (21), elevated CO2 level likely reflected ARDS severity and an increased dead space fraction. Similarly, Yang et al. reported that most COVID-19 patients usually develop severe pneumonia and are at a high risk factor of ARDS (22). Furthermore, Buchner et al. directly identified that patients with more severe CO2 retention might have a poor prognosis (23). Thus, most pneumonia patients with high CO2 levels had poor prognosis.
In our study cohort, we found that decreased CO2 levels increased mortality but had no significant effect on the disease severity. According to previous studies, the causes of decreased CO2 levels are as follows: shortness of breath, reduction of pulmonary perfusion and increased alveolar dead space, and MV hyperventilation (11, 24, 25). Because most COVID-19 patients require various forms of oxygen support, among other treatments, we thought that clinicians should focus their attention on MV hyperventilation (26), which is an effective and practical measure to improve patients' survival. Furthermore, according to the fitted curves, compared with pneumonia patients with non-decreased CO2 levels, the other study groups' trend showed an initial decrease and subsequent increase in CO2 levels. This indicates that the oxygen flow was adjusted to meet the patients' requirements to treat pneumonia and prevent a decrease in the CO2 levels due to hyperventilation.
This study has several limitations. Because the Leishenshan hospital was rapidly built as a designated hospital for COVID-19, it was difficult to share laboratory testing data with other hospitals. Thus, the data may be biased. For example, according to our study, there was no correlation between decreased CO2 levels and illness severity in COVID-19 patients. Furthermore, the mechanism of how oxygen support influences CO2 levels and thus affects patients' prognoses requires laboratory verification. However, this study makes a significant scientific contribution by providing evidence indicating that clinicians should pay attention to decreased CO2 levels in pneumonia patients with COVID-19, and so to prevent hypocapnia and maintain homeostasis.
In this study, we demonstrated that decreased CO2 levels increased the mortality risk of COVID-19 patients, but showed no significant impact on the severity of pneumonia. Furthermore, our study serves as evidence for clinicians to pay greater attention to the oxygen flow in COVID-19 patients who receive oxygen support to avoid treatment-related injuries. With these changes, the complications of COVID-19 can be further reduced, thereby improving the prognosis of COVID-19 patients with pneumonia.
Data Availability Statement
The original contributions presented in the study are included in the article/supplementary material, further inquiries can be directed to the corresponding authors.
Author Contributions
SC, JH, YH, ML, and WL undertook the research. SW and QL performed the analyses and interpretation of data. DH, JL, and RG wrote the main manuscript text and prepared figures. ZL, LG, and XW revised the article critically for important intellectual content and final approval of the version to be submitted. All authors contributed to the design of the study, writing of the manuscript, reviewed, and approved the manuscript.
Conflict of Interest
The authors declare that the research was conducted in the absence of any commercial or financial relationships that could be construed as a potential conflict of interest.
Acknowledgments
We appreciate all doctors, nurses, and other medical team members who provided assistance. We also would like to thank all patients and their families that made it through this difficult time together.
Abbreviations
COVID-19, coronavirus disease; CO2, carbon dioxide; CI, confidence interval; SARS-CoV-2, severe acute respiratory syndrome coronavirus 2; CT, computed tomography; GGO, ground-glass opacities; SD, standard deviation; IQR, median and interquartile range; WBC, white blood cell; PLT, platelet; MV, mechanical ventilation; ARDS, acute respiratory distress syndrome.
References
1. Huang C, Wang Y, Li X, Ren L, Zhao J, Hu Y, et al. Clinical features of patients infected with 2019 novel coronavirus in Wuhan, China. Lancet. (2020) 395:497–506. doi: 10.1016/S0140-6736(20)30183-5
2. Phelan AL, Katz R, Gostin LO. The novel coronavirus originating in Wuhan, China: challenges for Global Health Governance. JAMA. (2020) 323:709–10. doi: 10.1001/jama.2020.1097
3. Gorbalenya AE, Baker SC, Baric RS, de Groot RJ, Drosten C, Gulyaeva AA, et al. Severe acute respiratory syndrome-related coronavirus: the species and its viruses – a statement of the Coronavirus Study Group. bioRxiv. (2020). doi: 10.1101/2020.02.07.937862
4. Zhou F, Yu T, Du R, Fan G, Liu Y, Liu Z, et al. Clinical course and risk factors for mortality of adult inpatients with COVID-19 in Wuhan, China: a retrospective cohort study. Lancet. (2020) 395:1054–62. doi: 10.1016/S0140-6736(20)30566-3
5. Worldometer COVID-19 Data. Available online at: https://www.worldometers.info/coronavirus/ (accessed date July 23, 2020).
6. Guan W-J, Ni Z-Y, Hu Y, Liang W-H, Ou C-Q, He J-X, et al. Clinical characteristics of coronavirus disease 2019 in China. N Engl J Med. (2020) 382:1708–20. doi: 10.1056/NEJMoa2002032
7. Li Q, Guan X, Wu P, Wang X, Zhou L, Tong Y, et al. Early transmission dynamics in Wuhan, China, of novel coronavirus-infected pneumonia. N Engl J Med. (2020) 382:1199–207. doi: 10.1056/NEJMoa2001316
8. Lu H, Stratton CW, Tang Y-W. Outbreak of pneumonia of unknown etiology in Wuhan, China: the mystery and the miracle. J Med Virol. (2020) 92:401–2. doi: 10.1002/jmv.25678
9. Yao XH, Li TY, He ZC, Pingnaan YF, Liu HW, Yu SC, et al. A pathological report of three COVID-19 cases by minimal invasive autopsies. Zhonghua Bing Li Xue Za Zhi. (2020) 49:411–7. doi: 10.3760/cma.j.cn112151-20200312-00193
10. Lei F, Liu Y-M, Zhou F, Qin J-J, Zhang P, Zhu L, et al. Longitudinal association between markers of liver injury and mortality in COVID-19 in China. Hepatology. (2020) 72:389–98. doi: 10.1002/hep.31301
11. Aminiahidashti H, Shafiee S, Zamani Kiasari A, Sazgar M. Applications of End-Tidal Carbon Dioxide (ETCO2) monitoring in emergency department; a narrative review. Emerg. (2018) 6:e5. doi: 10.22037/emergency.v6i1.19298
12. Wang D, Hu B, Hu C, Zhu F, Liu X, Zhang J, et al. Clinical characteristics of 138 hospitalized patients with 2019 novel coronavirus-infected pneumonia in Wuhan, China. JAMA. (2020) 323:1061–9. doi: 10.1001/jama.2020.1585
13. Tian S, Hu W, Niu L, Liu H, Xu H, Xiao S-Y. Pulmonary pathology of early-phase 2019 novel coronavirus (COVID-19) pneumonia in two patients with lung cancer. J Thorac Oncol. (2020) 15:700–4. doi: 10.1016/j.jtho.2020.02.010
14. Yang X, Yu Y, Xu J, Shu H, Xia J, Liu H, et al. Clinical course and outcomes of critically ill patients with SARS-CoV-2 pneumonia in Wuhan, China: a single-centered, retrospective, observational study. Lancet Respir Med. (2020) 8:475–81. doi: 10.1016/S2213-2600(20)30079-5
15. Bellani G, Laffey JG, Pham T, Fan E, Brochard L, Esteban A, et al. Epidemiology, patterns of care, and mortality for patients with acute respiratory distress syndrome in intensive care units in 50 countries. JAMA. (2016) 315:788–800. doi: 10.1001/jama.2016.0291
16. Krauss B. Capnography as a rapid assessment and triage tool for chemical terrorism. Pediatr Emerg Care. (2005) 21:493–7. doi: 10.1097/01.pec.0000173345.07530.c0
17. Adams L, Butas S, Spurlock D. Capnography (ETCO2), respiratory depression, and nursing interventions in moderately sedated adults undergoing transesophageal echocardiography (TEE). J Perianesth Nurs. (2015) 30:14–22. doi: 10.1016/j.jopan.2013.07.009
18. Burton JH, Harrah JD, Germann CA, Dillon DC. Does end-tidal carbon dioxide monitoring detect respiratory events prior to current sedation monitoring practices? Acad Emerg Med. (2006) 13:500–4. doi: 10.1197/j.aem.2005.12.017
19. Deitch K, Miner J, Chudnofsky CR, Dominici P, Latta D. Does end tidal CO2 monitoring during emergency department procedural sedation and analgesia with propofol decrease the incidence of hypoxic events? A randomized, controlled trial. Ann Emerg Med. (2010) 55:258–64. doi: 10.1016/j.annemergmed.2009.07.030
20. Soto RG, Fu ES, Vila H, Miguel RV. Capnography accurately detects apnea during monitored anesthesia care. Anesth Analg. (2004) 99:379–82. doi: 10.1213/01.ANE.0000131964.67524.E7
21. Nuckton TJ, Alonso JA, Kallet RH, Daniel BM, Pittet J-F, Eisner MD, et al. Pulmonary dead-space fraction as a risk factor for death in the acute respiratory distress syndrome. N Engl J Med. (2002) 346:1281–6. doi: 10.1056/NEJMoa012835
22. Yang X, Cai S, Luo Y, Zhu F, Hu M, Zhao Y, et al. Extracorporeal membrane oxygenation for coronavirus disease 2019-induced acute respiratory distress syndrome: a multicenter descriptive study. Crit Care Med. (2020) 48:1289–95. doi: 10.1097/CCM.0000000000004447
23. Buchner J, Mazzeffi M, Kon Z, Menaker J, Rubinson L, Bittle G, et al. Single-center experience with venovenous ECMO for influenza-related ARDS. J Cardiothorac Vasc Anesth. (2018) 32:1154–9. doi: 10.1053/j.jvca.2017.09.031
24. Hawkins NM, Petrie MC, Jhund PS, Chalmers GW, Dunn FG, McMurray JJV. Heart failure and chronic obstructive pulmonary disease: diagnostic pitfalls and epidemiology. Eur J Heart Fail. (2009) 11:130–9. doi: 10.1093/eurjhf/hfn013
25. Anderson CT, Breen PH. Carbon dioxide kinetics and capnography during critical care. Crit Care. (2000) 4:207–15. doi: 10.1186/cc696
26. Chinese Clinical Guidance for COVID-19 Pneumonia Diagnosis and Treatment. 7th ed. Available online at: http://kjfy.meetingchina.org/msite/news/show/cn/3337.html
Keywords: infectious disease, pneumonia, COVID-19, CO2, mechanical ventilation
Citation: Hu D, Li J, Gao R, Wang S, Li Q, Chen S, Huang J, Huang Y, Li M, Long W, Liu Z, Guo L and Wu X (2021) Decreased CO2 Levels as Indicators of Possible Mechanical Ventilation-Induced Hyperventilation in COVID-19 Patients: A Retrospective Analysis. Front. Public Health 8:596168. doi: 10.3389/fpubh.2020.596168
Received: 18 August 2020; Accepted: 19 November 2020;
Published: 08 January 2021.
Edited by:
Fabrizio Ricci, University of Studies G. d'Annunzio Chieti and Pescara, ItalyReviewed by:
Xiaojiong Jia, Harvard Medical School, United StatesMengyuan Lyu, Sichuan University, China
Copyright © 2021 Hu, Li, Gao, Wang, Li, Chen, Huang, Huang, Li, Long, Liu, Guo and Wu. This is an open-access article distributed under the terms of the Creative Commons Attribution License (CC BY). The use, distribution or reproduction in other forums is permitted, provided the original author(s) and the copyright owner(s) are credited and that the original publication in this journal is cited, in accordance with accepted academic practice. No use, distribution or reproduction is permitted which does not comply with these terms.
*Correspondence: Zeming Liu, Nm15dEAxNjMuY29t; Liang Guo, Z3VvbGlhbmd3aHpuQDE2My5jb20=; Xiaohui Wu, d3V4aWFvaHVpMTk3MUBzaW5hLmNvbQ==
†These authors have contributed equally to this work