- 1Point-of-Care Testing Center for Teaching and Research (POCT·CTR™), University of California, Davis, Davis, CA, United States
- 2Knowledge Optimization®, Davis, CA, United States
Objectives: (a) To understand how to integrate geospatial concepts when implementing point-of-care testing (POCT); (b) to facilitate emergency, outbreak, and disaster preparedness and emergency management in healthcare small-world networks; (c) to enhance community resilience by using POCT in tandem with geographic information systems (GISs) and other geospatial tools; and (d) to advance crisis standards of care at points of need, adaptable and scalable for public health practice in limited-resource countries and other global settings.
Content: Visual logistics help integrate and synthesize POCT and geospatial concepts. The resulting geospatial solutions presented here comprise: (1) small-world networks and regional topography; (2) space-time transformation, hubs, and asset mapping; (3) spatial and geospatial care paths™; (4) GIS-POCT; (5) isolation laboratories, diagnostics isolators, and mobile laboratories for highly infectious diseases; (6) alternate care facilities; (7) roaming POCT—airborne, ambulances, space, and wearables; (8) connected and wireless POCT outside hospitals; (9) unmanned aerial vehicles; (10) geospatial practice—demographic care unit resource scoring, geographic risk assessment, and national POCT policy and guidelines; (11) the hybrid laboratory; and (12) point-of-careology.
Value: Small-world networks and their connectivity facilitate efficient and effective placement of POCT for optimal response, rescue, diagnosis, and treatment. Spatial care paths™ speed transport from primary encounters to referral centers bypassing topographic bottlenecks, process gaps, and time-consuming interruptions. Regional GISs position POCT close to where patients live to facilitate rapid triage, decrease therapeutic turnaround time, and conserve economic resources. Geospatial care paths™ encompass demographic and population access features. Timeliness creates value during acute illness, complex crises, and unexpected disasters. Isolation laboratories equipped with POCT help stop outbreaks and safely support critically ill patients with highly infectious diseases. POCT-enabled spatial grids can map sentinel cases and establish geographic limits of epidemics for ring vaccination.
Impact: Geospatial solutions generate inherently optimal and logical placement of POCT conceptually, physically, and temporally as a means to improve crisis response and spatial resilience. If public health professionals, geospatial scientists, and POCT specialists join forces, new collaborative teamwork can create faster response and higher impact during disasters, complex crises, outbreaks, and epidemics, as well as more efficient primary, urgent, and emergency community care.
Goal and Mission
The goal of this review is to analyze the geospatial point-of-care testing (POCT) solutions in Table 1 (1–77), while addressing key issues and determining how the different solutions can help meet public health challenges. This article applies POC-geospatial concepts to disaster preparedness, emergency management, and public health challenges, such as outbreaks and their containment. The long-term mission is to prepare public health practitioners and laboratory medicine professionals to collaboratively employ geospatial tools and POCT in emergencies, epidemics, and disasters and to enable these specialists to make rapid diagnostic-therapeutic decisions at points of need.
Introduction
Rationale
Synthesis of geospatial and POC concepts can facilitate emergency care, crisis response, and control of highly infectious diseases, such as Ebola virus disease (“Ebola”). Integration of both concepts improves population access to healthcare. For efficiency and cost-effectiveness, POCT must fulfill healthcare needs on a daily basis, improve diagnostic skills, and enable public health, emergency medicine, and other personnel with fast decision making.
Definitions
Point-of-care testing is defined as diagnostic testing at or near the site of patient care (78, 79). It is inherently spatial, that is, performed at or near points of need, and also intrinsically temporal, because it produces fast actionable results. This definition does not depend on the size or format of the handheld, portable, or transportable instrument, test module, or assay design.
It includes disposable test strips and in situ, ex vivo, in vivo, and on vivo monitoring (e.g., pulse oximeters or wearables). The “Cape Cod” group (80) codified the definition, which appears in standard dictionaries of the English language. The POCT•CTR wrote the original Wikipedia article (see https://en.wikipedia.org/wiki/Point-of-care_testing).
Organization
Table 1 focuses on the geospatial tools most relevant for creating the right location and timing for implementing POCT, especially in resource-limited settings. For example, small-world networks (SWN) led to striking nation-wide improvements in the care of patients with acute coronary syndromes in Thailand (see Table 1, Solution 1). References cited in Table 1 provide practical details, so readers can custom tailor solutions to their own settings.
Background
Highly infectious diseases, regional wars exacerbating deadly outbreaks [e.g., Ebola in the Democratic Republic of the Congo (DRC)], populations growing exponentially at least until mid-century (81), dense coastal communities, global warming and rising oceans, weather catastrophes, and migrating refugees seeking refuge and asylum, among others, challenge our ability to rectify health disparities. The Global Preparedness Monitoring Board (GPMB) warns, “The world is at acute risk for devastating regional or global disease epidemics or pandemics that not only cause loss of life but upend economies and create social chaos (82).”
However, the GPMB report did not recognize the importance of geospatial science and POCT. Population clusters in highly vulnerable areas amplify the risk. Nonetheless, we must eliminate inequities, while also responding quickly and effectively to increasingly frequent disasters, complex crises, and epidemics. To understand how to mitigate risk, we can use geospatial science mappings and produce “visual logistics,” that is, easily understood graphics, flowcharts, topography maps, time contours, and other illustrations that show where, when, and how to position POCT optimally, create mobile rapid response, and implement the solutions in Table 1.
Methods and Scope
Methods
PubMed, the World-wide Web, web sites of educational institutions, and other relevant sources were searched for papers, articles, chapters, documents, flowcharts, maps, and schematics in the form of published works and field research in limited-resource and other settings. Searches using IEEE Xplore did not yield additional relevant articles. In the interests of brevity, the reader can find detailed discussions of geospatial theory, software apps, and analytical techniques in Ferguson et al. (28–30). Visual logistics illustrate several key concepts. EndNote X9.1 (Clarivate Analytics, https://clarivate.com/) was used to consolidate over 500 entries retrieved as abstracts, ULRs, and PDFs, then subsequently pruned to slightly more than 100 articles to focus this review.
Scope
Numerous sources identified through PubMed dealt with the general area of geographic information systems (GISs) in healthcare. The majority addressed GISs for tracking, monitoring, and managing infectious diseases, such as malaria and HIV. This article assesses the importance of geospatial science as it pertains specifically to POCT. Only those geospatially oriented publications explicitly discussing or integrating POCT and mobile technologies in relevant spatial settings, such as ring vaccination and space flight, are assessed here. Molecular diagnostics for Ebola and other highly infectious threats can be found in comprehensive reviews (36, 83) and a book chapter (44).
Geospatial Science
This section analyzes 10 geospatial science approaches (Table 1) where optimal interplay of space and time can enhance healthcare and improve positioning of POCT resources. It also identifies current research gaps and future horizons. Historically, SWNs facilitated placement of POC cardiac biomarker testing in limited-resource emergency rooms of Thailand, so we start with that, then move through space-time transformations, spatial care paths™ (SCPs), geographic information systems (GISs), and other high yield solutions.
Outbreaks are spatially dynamic. Starting with the recent epidemic in 2014, stopping Ebola outbreaks from spreading and caring for infected patients who are critically ill unequivocally proved the need for POCT (16, 34–36, 44, 83). The current situation in the DRC is no exception. Hence, Table 1 also covers physical spatial designs needed to address safe handling of highly infectious threats, care for infected patients, and render communities more resilient.
Small-World Networks and Regional Topography (Solution 1, Table 1)
Small-world networks evolve naturally from social, political, and economic interactions; population dynamics; and medical-cultural ecosystems. Figure 1 illustrates relationships in a typical healthcare in a SWN in a limited-resource setting. Analysis of SWNs discloses how healthcare is delivered, whether population access is adequate, and where gaps in emergency service occur. Unique topographic features, such as mountains, lakes, and rivers within a limited geographic region typically bound a specific SWN. During a crisis, transportation routes can become constrained by storms, floods, earthquakes, interrupted roadways, telecommunication disruptions, systems failures, unexpected mishaps, supply chain shortages, and failures of emergency vehicles and aircraft. Rescue and response become compromised, complicated, and prolonged, often at the cost of human life.
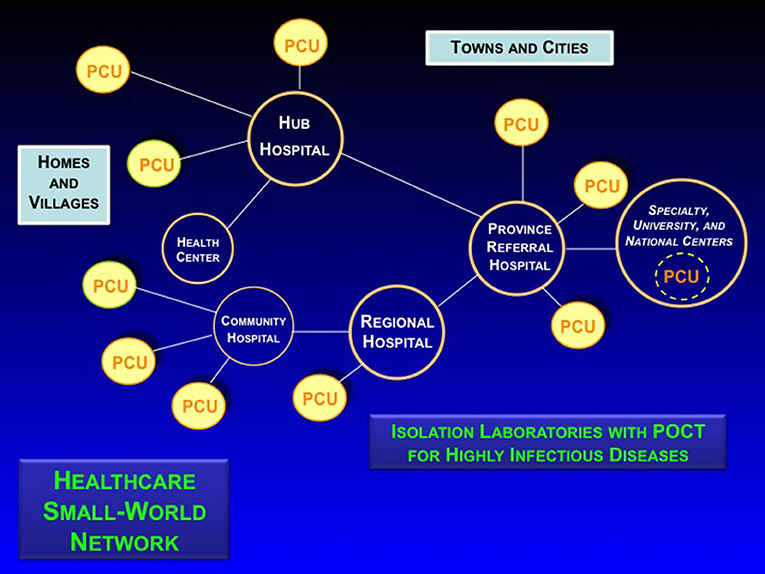
Figure 1. Small-world networks in limited-resource settings. Primary care units (“PCU”) serve patients close to home. Clusters, nodes, and relatively few connections characterize the typical healthcare SWN in a limited-resource country. Hub hospitals can expedite referrals to the province hospital. The SWN should include isolation laboratories for rapid and safe testing of specimens from patients with highly infectious diseases. Image reproduced with permission of Knowledge Optimization.
Practice principles for optimizing POCT in SWNs are designed to enhance disaster preparedness, emergency management, and public health response at local (district, county, province, or state), regional, and national levels, while simultaneously improving urgent and routine care in the community. This allows POCT operators to garner substantial experience with the use of POC instruments, reagents, and quality assurance through daily experience. POCT should not be used without proper training of those who will perform testing and quality control.
For details of SWN theory, please see “Using small-world networks to optimize preparedness, response, and resilience,” chapter 49 in Global Point of Care: Strategies for Disasters, Emergencies, and Public Health Resilience (12). Briefly, a SWN represents a loosely tied and well, but not necessarily evenly, connected set of nodes and clusters in a scale-free network with a topology that is neither completely regular nor entirely random. Most nodes in a SWN are not neighbors, but can be reached in a few steps. Scale-free networks have hubs of connectivity that shape the way the network operates. Hubs provide robustness to failure, a key point when improving community resilience. Hubs connect nodes locally while also connecting clusters globally, conferring SWN properties to the healthcare system.
Regional hubs represent an advantage when planning POCT sites. However, removal of a busy hub during a disaster, because of physical destruction, invasive flooding, or some other calamity can turn the SWN into an isolated graph. Hence, resilience depends in part on self-reliance at individual nodes and clusters, an attribute that POCT confers nicely by enabling evidence-based decision making and local treatment. Only a few edges (interactions) separate nodes, a property leading to the popular notion of only 6 degrees of separation between any two people in the world. Separation impacts SWNs, so separation, per se, is to be avoided. Transit and information delays among SWNs can be fixed with physical splines (e.g., transportation “short-cuts”) and virtual connectivity (e.g., using POC disposable test modules on smartphones with built-in wireless communication).
Small-world networks must be discovered, drawn, and metricized through grass roots field investigations and interviews of emergency personnel. Figure 2 illustrates the process of discovering the characteristics and infrastructure of a SWN in rural impoverished provinces in Isaan, Northeast Thailand. Interactions, especially emergency links, infectious vectors, and other systematic and random phenomena tend to transcend simple policy, roadmaps, phone lines, cellular towers, and radio transmissions. They encompass personal and professional interactions, resource limitations, adaptable social networks, and government requirements regulating tiered use of health maintenance sites, primary care units (PCUs), clinics, community and regional hospitals, and university or referral medical centers. Their interdependencies, both routine and emergent, often cross established boundaries and bridge international borders through fluxes of supplies, laborers, professional personnel, and also, communicable diseases.
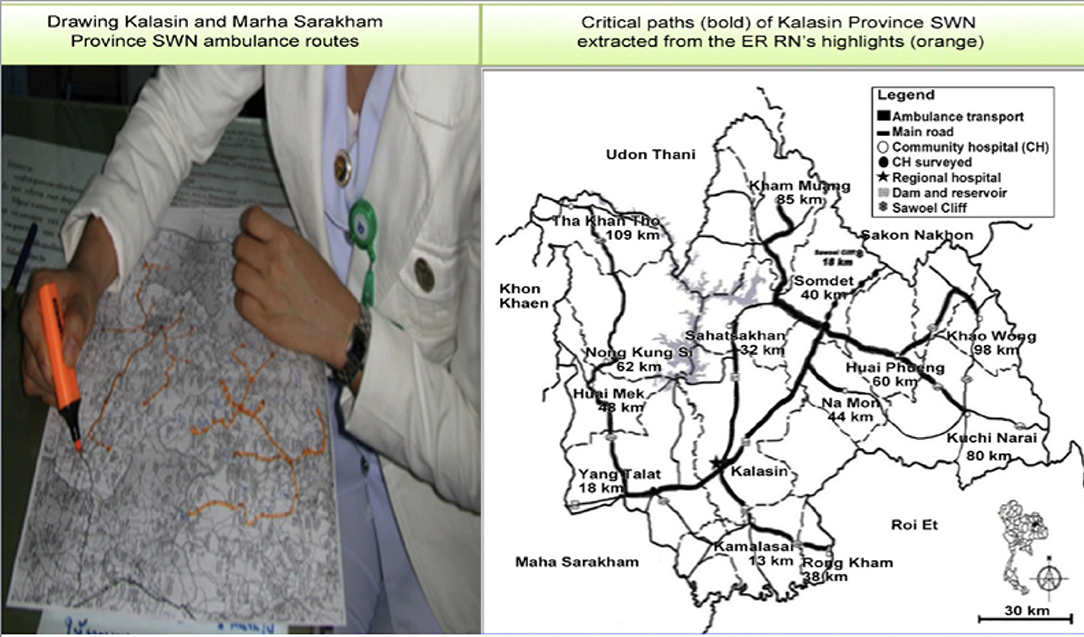
Figure 2. Configuring the healthcare small-world network, Kalasin Province, Thailand. Image reproduced with permission of Knowledge Optimization.
Solution 1 in Table 1 provides analysis (right column) of SWN field applications of POCT in limited-resource settings. Typically, these settings do not have helicopter or fixed-wing aircraft rescue and often lack adequate ambulance service. After successful pilot studies and educational seminars held for emergency staff, critical care nurses, and cardiologists, an executive decision was made at the national level by an academic (GK, while a Fulbright Scholar), professional, and industry team to implement approximately 700 POC cardiac troponin T (cTnT) handheld devices (cobas h 232, Roche Diagnostics) in over 500 community hospitals, revolutionizing the care of acute coronary syndrome patients in Thailand [5]. The other applications under Solution 1 demonstrate similar utility and value of POCT in SWNs. The keys are flexibility and adaptability. Well-placed POCT can make SWN response “organic,” that is, rapidly adaptable in the face of evolving needs and crises.
Space-Time Transformation, Hubs, and Asset Mapping (Solution 2)
Figure 3 illustrates space-time transformation of the healthcare network with analysis of mobility, geographic routes, and ambulance travel. For example, an emergency room physician in a community hospital distant from the provincial regional hospital could rule in the diagnosis of acute myocardial infarction using POC cTn testing, and then, save time by transporting the patient immediately and directly to a referral site where an interventional cardiologist is available (upper left, Figure 3), rather than routing the patient through the regional hospital in the center of the province when there is no cardiologist available.
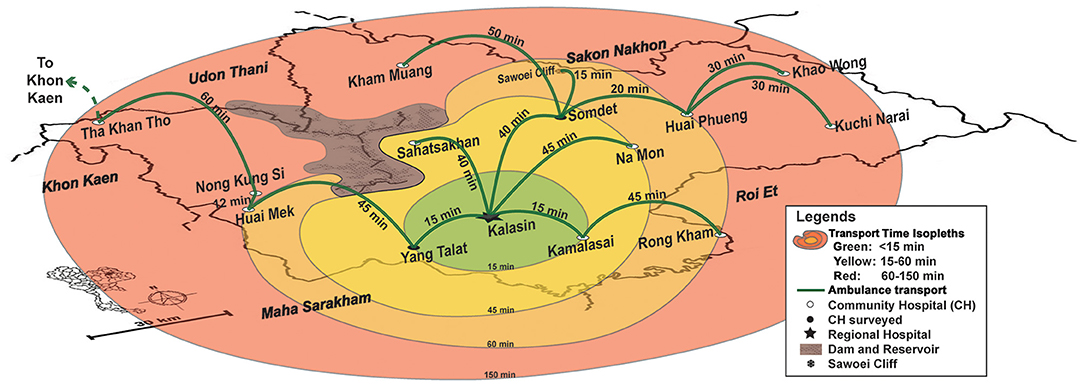
Figure 3. Transforming the small-world network from the physical to the temporal domain. Emergency room physicians in the Tha Khan Tho Community Hospital near the border of Kalasin Province (upper left) could refer acute coronary syndrome patients directly to an interventional cardiologist at the Khon Kaen Heart Center if elevated cTnT rules in acute myocardial infarction. Otherwise, patients are referred to the Kalasin Regional Hospital, but transportation is prolonged. At the time of the field survey, no cardiologists were available in the province, so patients would only receive enzyme or palliative treatment, unless referred to the Heart Center. Hence, POC cTn, rapid response, and efficient routing increase chances of survival. Image reproduced with permission of Knowledge Optimization.
Figure 4 illustrates temporal contour map analysis in northern Nan Province of Thailand, where a team from the POCT•CTR, Siriraj Hospital (Mahidol University), and the College of Population Studies (Chulalongkorn University) conducted extensive field surveys of healthcare needs, resources, transportation routes, emergency medical systems/services (EMS), radio dispatching, and delivery gaps that could be filled with POC, near-patient, or satellite laboratory testing. The right frame shows the benefit of reduced response time when there is a regional hub. Hubs arise because combining resources overcomes constraints. Contour analysis clarified trade-offs of time and locale in the province SWN. The hub hospital substantially improved the efficiency of providing care to those living near the Thailand-Laos PDR border.
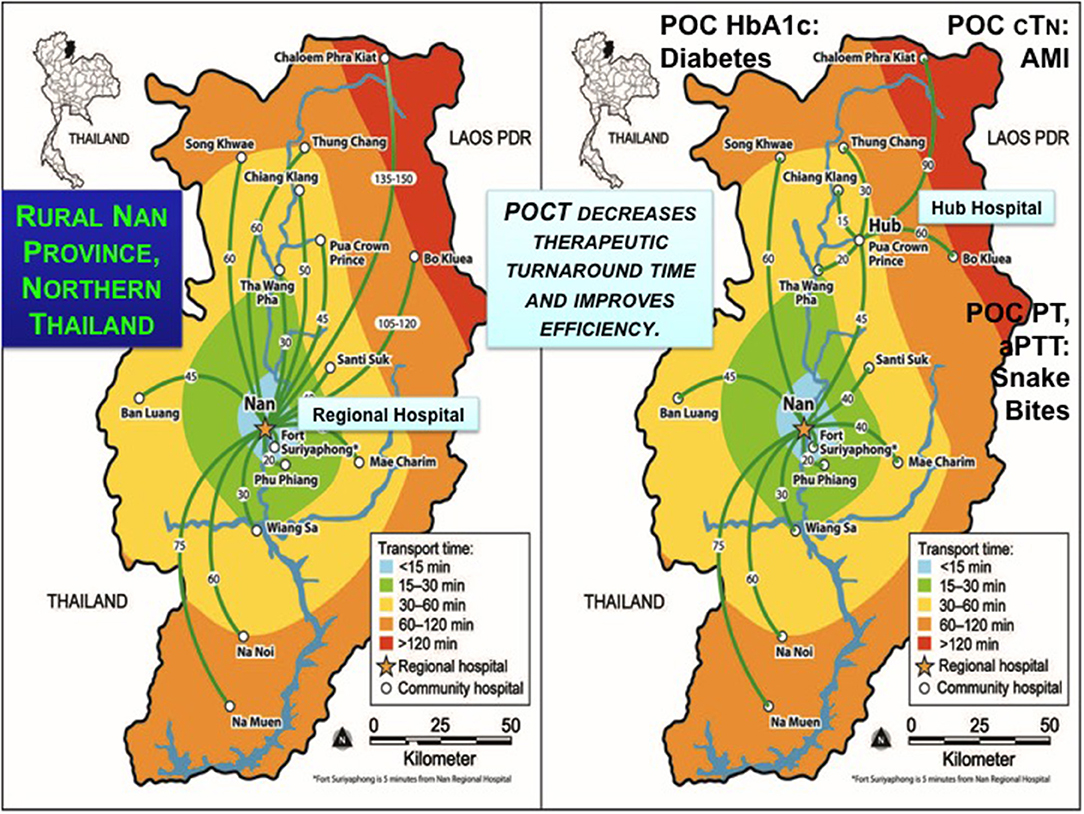
Figure 4. Contour maps and hub concept for efficient regional care in Nan Province, Northern Thailand. The left frame shows the SWN of rural Nan Province, and the right, the utility of a hub hospital to improve efficiency by decreasing transit times indicated by temporal contours. Pont-of-care testing can accelerate evidence-based decision making for the conditions shown, which community hospitals identified as high priority. Image reproduced with permission of Knowledge Optimization.
A surprise outcome in Nan Province was that nursing staff in the most northern regions adjacent to Laos PDR in Chaloem Phra Kiat Community Hospital insisted POCT be implemented to enable young physicians to make evidence-based decisions based on diagnostic bedside test results. Education became a primary motivator for implementing POCT. Junior physicians are conscripted to work in rural hospitals for 3 years to pay back government funding of their education. The number of people per doctor ranged from a low of 954 in Bangkok to a high of 8,510 in neglected resource-limited northern and northeastern provinces (64, 65). Often, the young doctors are on duty by themselves throughout the night without backup and no access to definitive diagnostic tests, such as the biomarkers of cardiac injury.
During interviews the physicians said they did not like watching their patients, perhaps with equivocal EKGs, die while under observation, but instead wanted to use POC cTn testing to rule in acute myocardial infarction and refer quickly. Similar themes (see Figure 4) of rapid response cropped up for urgent POC coagulopathy tests (PT and aPTT) when children presented to the emergency room at high risk from potentially fatal snakebites and the only analysis available was timing blood clotting in a tube. For diabetes, medical staff wanted to use immediate onsite HbA1c results to avoid long specimen transport to referral sites, week or longer delays waiting for results, time and money consuming return trips by patients, and their forfeiture of employment income. HbA1c also can help identify patients with diabetes in field facilities, quarantine, and isolation for highly infectious diseases, such as Ebola when specimens cannot be sent to the clinical laboratory for fear of contamination. In settings where population migration complicates public health screening, such as influx of workers with HIV and possibly also TB into the SWN from across a natural geographic border (Figure 5), rapid response testing can facilitate patient workup and case reporting for epidemiological databases in primary care sites.
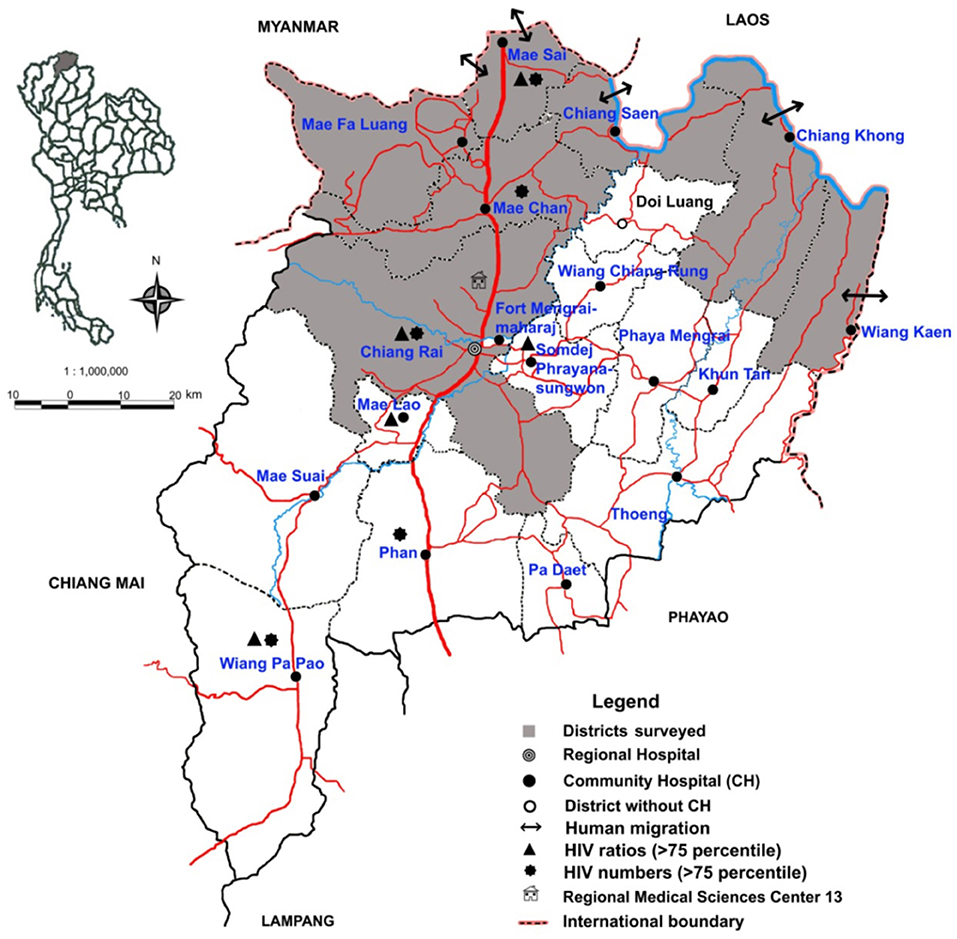
Figure 5. Chiang Rai Province small-world network and human migration across the Laotian border to Thailand and back. Clandestine and night crossings by boat on the Mekong River (blue line, northeast) allow prolific international human traffic into and out of the province. That brings HIV and other problems associated with migration to bear on the healthcare SWN. Clinic point-of-careologists could help abate spread of unreported disease. Image reproduced with permission of Knowledge Optimization.
Spatial and Geospatial Care Paths™ (Solution 3)
A spatial care path (SCP) is defined as the most efficient route taken by the patient when receiving definitive care in a SWN (15). A geospatial care path™ (GCP) is a second generation SCP that integrates demography and other population factors to respond quickly to crisis stress (21). Solution 3 in Table 1 presents analysis of several applications of SCPs where the logic of their design is to identify the best routes for patient rescue and transport facilitated by shrewd placement of POCT for rapid diagnosis, triage, and treatment.
Figure 6 illustrates a SCP solution created after an in-depth field survey of Hue Province in Central Vietnam (19), where the rural mountainous regions present significant challenges for rescuing rural patients with acute chest pain. The same logic was applied to infectious diseases and diabetes care; for example, see the analysis for Ventura et al. (20) in Table 1. Spatial care paths also provide clever opportunities for implementing emergency room and satellite laboratory diagnostic testing in SWNs.
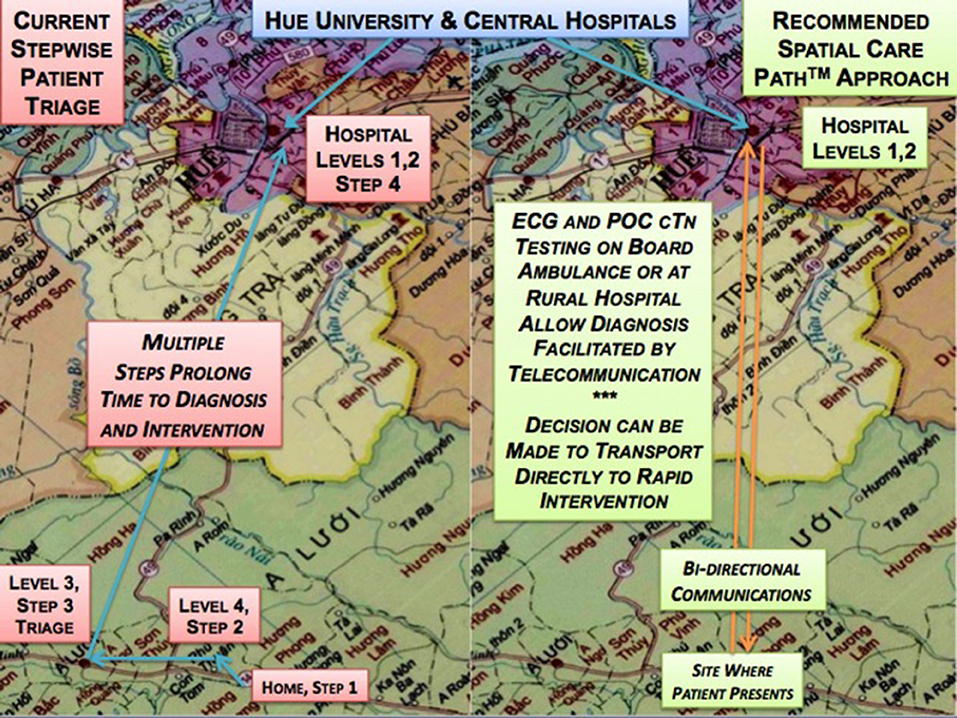
Figure 6. Spatial Care Path™ in Hue Province, Central Vietnam. Following an extensive needs assessment field survey of Hue Province, Central Vietnam, analysis of the traditional stepwise process (left) of patient evaluation in the rural mountainous areas and in multi-level government care sites downstream showed response time for patients with suspected acute myocardial infarction could be improved substantially by adopting POC cardiac biomarker testing upstream and more direct routes (right) to cardiologist intervention in Hue City. Image reproduced with permission of Knowledge Optimization.
It is likely that the SCP concept eventually will dominate healthcare delivery in limited-resource settings as populations expand, the number of elderly increase, and common sense, not to mention financial necessity, dictate that diagnosis and treatment must shift upstream nearer to the site of the patient's home in order to conserve resources, save time, and spare lives. As the availability of POC technologies expands and costs decrease through manufacturing efficiencies, countries with burgeoning population, such as China, India, Indonesia, and the Philippines, will find it easier to move initial diagnostic evaluation to primary sites.
In Indonesia and the Philippines, local independence of healthcare nodes, clusters, and hubs becomes essential. Time is of the essence. Transport to larger islands where referral hospitals are located is challenging. In fact, island nations are at risk of flooding displacing dense coastal populations. POCT can follow these migrations. Hospital directors stated that 80% of their community population should be diagnosed and ideally, treated, in primary care sites outside the hospital, in order to prevent saturation of emergency rooms that simply cannot handle huge numbers of patients and unexpected surges that show up for evaluation (e.g., during a seasonal Dengue hemorrhagic fever outbreak).
Earthquakes in densely populated areas (Figure 7) warrant serious advance design and planning of SCPs to back up traditional routes to and from health centers and hospitals. With its numerous fault lines, fire hazard areas, limited rural transportation routes, and poorly maintained roads, a state like California should have a geospatial-POCT master plan to fill gaps in emergency management and preparedness. In other countries, the dynamic GCP concept can augment GISs analysis, especially in times of dire need, such as during volcano eruptions, tsunamis, and outbreaks of highly infectious disease.
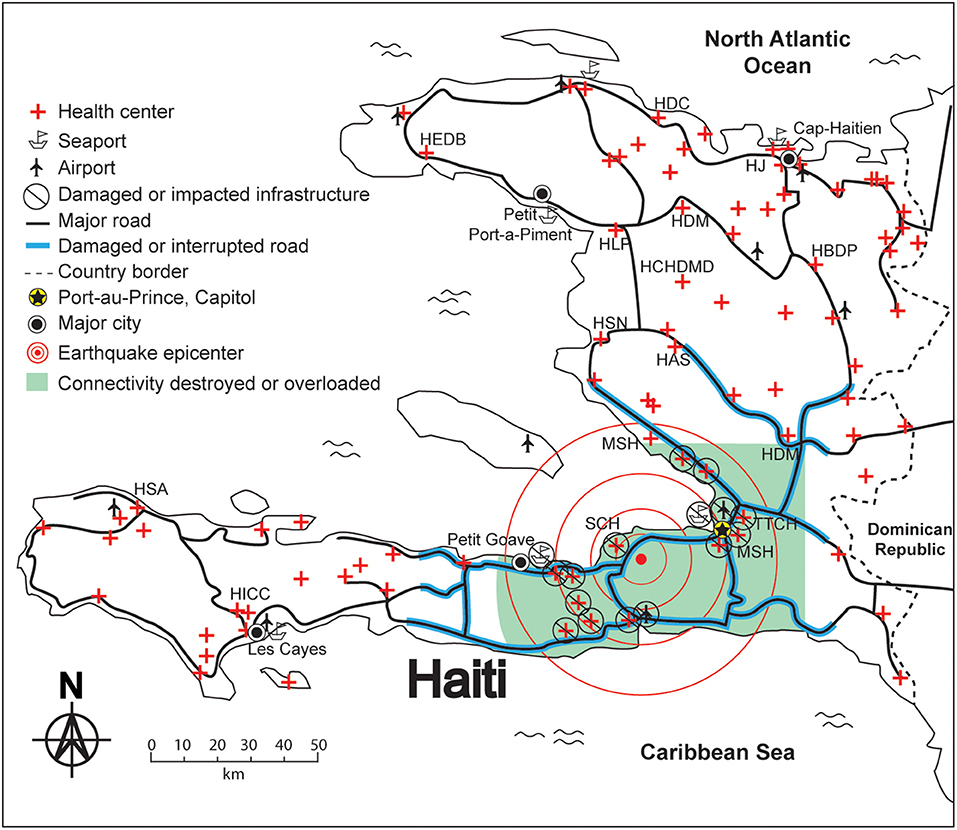
Figure 7. Geographic isolation by the Haiti Earthquake—need for spatial resilience. The circles show the earthquake epicenter. Bold lines show damaged or interrupted roads. United States Disaster Medical Assistance Teams carried suitcase size (<50 lbs) sets of POC diagnostics, but larger instruments transported to Haiti could not be used because there were no trained operators who could perform quality control. In settings like this one, communities should develop their own plans for resilience that include mobile POCT and properly trained, certified, and annually validated personnel. These resources should be placed at local nodes, clusters, or hubs in the SWN to assure optimal resilience. Image reproduced with permission of Knowledge Optimization.
Geographic Information Systems (GIS) + Point-of-care Testing (POCT)
A GIS is designed to capture, store, manipulate, analyze, manage, optimize, and present a broad spectrum of geographical data relevant to the task at hand. While the healthcare literature is replete with GIS papers, some proving efficacy for highly infectious diseases, such as Ebola (84–87), Solution 4 lists only those that combined both POC and GIS concepts, especially the analysis of population access in terms of travel time (Figure 8), an important criteria for deciding where to place POCT.
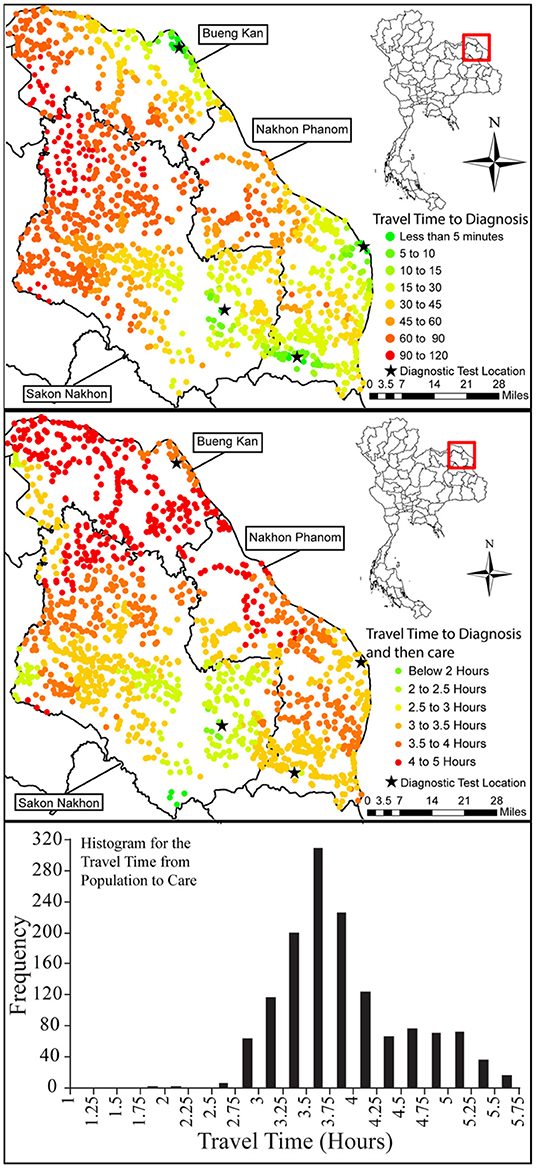
Figure 8. Travel times assessed using a geographic information system in the northern most region of Isaan, Thailand. Image reproduced with permission of Knowledge Optimization.
For example, Figure 9 illustrates GIS determination of how POCT could expedite patient access to care, triage, and intervention in high risk coastal Hualien County, Taiwan, and on remote Palawan Island, the Philippines. GISs are also useful for analyzing patterns of spread of infectious diseases and dynamically interactive treatment, such as ring vaccination (a geospatial strategy to inhibit spread by vaccinating those most likely to be infected).
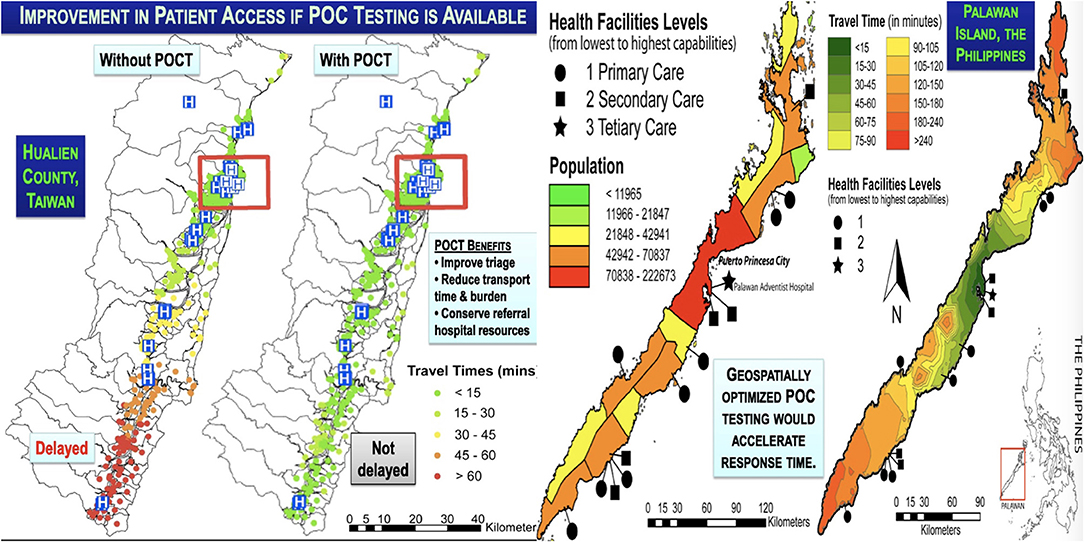
Figure 9. Geographic information system analysis of the impact of POCT in Hualien County, Taiwan, and Palawan Island, Philippines. Geographic information system analysis of population clusters illustrate how POCT could expedite patient access to care in Hualien County, Coastal East Taiwan (left), an area frequented by devastating typhoons. In the Philippines, extremely remote Palawan Island (right) is challenged by a similar long linear topography with poorly accessible rural northern and southern regions that lend themselves to geospatially placed POCT to improve patient access to healthcare. Image reproduced with permission of Knowledge Optimization.
Solution 4 entries illustrate how to integrate POCT as an element of public health strategy. For example, spatial grids enabled with POCT can locate sentinel cases and establish geographic limits of epidemics. To encourage these types of creative strategies, curricula of public health educational institutions should include training in the principles and practice of POCT (88, 89). As noted earlier, education represents an adequate reason for implementing POCT.
Isolation Laboratories, Diagnostics Isolators, and Mobile Laboratories for Highly Infectious Diseases (Solution 5)
The 2014–16 Ebola epidemic proved unequivocally the need for POCT in isolation laboratories and diagnostics isolators to support critically ill patients in isolation (16, 34–36, 44, 83). These solutions are distinctively and discretely spatial. Part of public health repertoire, they should be incorporated into the scheme of the typical SWN, as recommended in Figure 1.
Solution 5 references (see Table 1) provide details of diagnostics isolators and isolation laboratories built and completed in response to the 2014–16 crisis in West Africa and the spread of Ebola to other continents. Figure 10 provides a schematic and workflow diagram of our design built in several hospitals in Bangkok. Chapter 24 (44) of A Practical Guide to Global Point-of-Care Testing presents isolation laboratories with POCT placed inside biosafety cabinets (16, 35, 36) and conceptual designs for safe self-testing (“FAST•POC”) and assisted testing (“POC•POD”). Types of instruments, diagnostic tests, and isolator designs can be found in Kost et al. (16, 34–37, 44, 83).
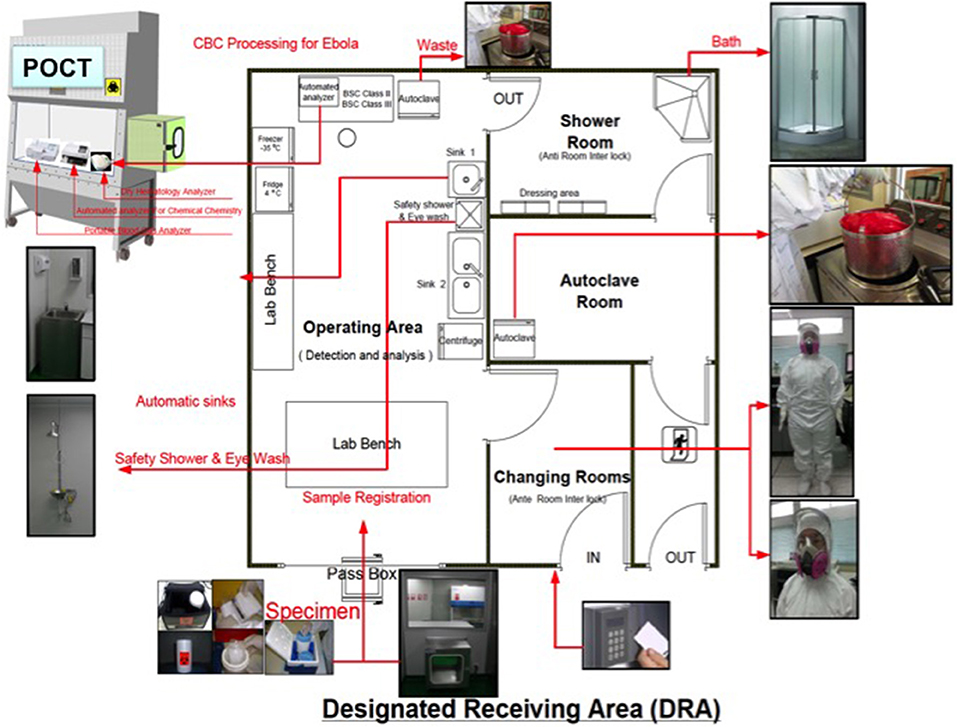
Figure 10. Design of an isolation laboratory with POCT inside a Biosafety Cabinet. This discrete spatial solution, an isolation laboratory design built in several Bangkok locations, offers vital engineering controls that reduce the risk of biohazards while also providing environmental temperature and humidity conditions for the proper operation of POC instruments. Personnel must practice donning personal protective equipment (PPE), then performing testing, followed by careful doffing, training that now is being implemented in US sites, such as the Center for Disaster Medicine at Massachusetts General Hospital (see https://www.massgeneral.org/News/newsarticle.aspx?id=7175). Image reproduced with permission of Knowledge Optimization.
Not all tests that have been used in isolation laboratories, such as prothrombin time (PT) (34), are US FDA-cleared for Ebola patients. A follow-up interview in 2017 describing the Emory Serious Communicable Disease Unit (SCDU) enumerated tests as: “Ebola virus PCR results were available in about 1.5 h after receipt of the specimen in the SCDU laboratory. Other tests available included CBC, CMP, magnesium, lactate dehydrogenase, gamma-glutamyl transferase, amylase, lactate, phosphorous, venous/arterial blood gases, urinalysis, FilmArray® gastrointestinal panel, FilmArray® respiratory panel, BinaxNOW® malaria assay, and Alere DetermineTM HIV-1/2 Ag/Ab Combo test (90).”
Kost et al. (36, 83) summarized molecular diagnostic technologies that emerged during and after the Ebola crisis. Food and Drug Administration Emergency Use Authorization (EUA) technologies can be found here: https://www.fda.gov/emergency-preparedness-and-response/mcm-legal-regulatory-and-policy-framework/emergency-use-authorization#current, and World Health Organization Emergency Use and Assessment Listings, here: https://www.who.int/diagnostics_laboratory/eual/emergency/en/.
Outbreaks continue in the DRC, now declared a global health emergency by the WHO. The number of cases (as of October 2019) totaled 3,274 with a death toll of 2,185 and mortality of 67% (see https://www.who.int/emergencies/diseases/ebola/drc-2019/). This Ebola outbreak is the second worst, in the face of a response marred by war zones, shootings of health workers, civil strife, abandonment by NGOs and healthcare personnel, strike threats by nurses, and resistance within local communities to preventative measures, care facilities, and safe burials. Several care centers (>132) and health care workers (300+) have been attacked. Nonetheless, novel POC technologies, when used with safe specimen processing, can enhance ring vaccination, which has benefited the DRC by mitigating spread.
On February 26, 2019, the CDC, FDA, and CMS announced a new “Tri-Agency Task Force for Emergency Diagnostics (TTFED),” (http://www.fda.gov/NewsEvents/Newsroom/PressAnnouncements/ucm632056.htm). The charter can be found here: http://www.fda.gov/downloads/EmergencyPreparedness/Counterterrorism/MedicalCountermeasures/MCMLegalRegulatoryandPolicyFramework/UCM631575.pdf. The consortium stated, “Through the TTFED, CDC, FDA, and CMS, where appropriate, intend to coordinate the implementation of EUA IVD assays in laboratories within the U.S. healthcare system, with the ultimate goal of improving responses to public health emergencies.” However, there is no task force plan to train public health students or POCT specialists in the use of EUA devices and associated quality control. Except for one medical technologist, laboratory medicine professionals, public health educational institutions, and industries developing new EUA technologies appear not to be represented.
The TTFED's focus on EUA IVD assays falls short of the need for strategically selected POC technologies that integrate and consolidate a broad range of tests intended to help the Ebola patient with a highly infectious disease survive. Samples cannot be sent to the clinical laboratory. If spilled or broken, the clinical laboratory must be shut down for clean up, causing unacceptable delays in hospital services.
Well-integrated and compact POC technologies with comprehensive test clusters are needed to fit within the confines of isolation laboratories and diagnostics isolators, which represent uniquely discrete spatial solutions. The devices must be user friendly for operators suited in personal protective equipment (PPE). Environmental conditions must be controlled, not only to assure accurate test results, but also for the comfort of operators wearing PPE.
Alternate Care Facilities; Roaming POCT—Airborne, Ambulances, Space, and Wearables; Connected and Wireless POCT Outside Hospitals; and Unmanned Aerial Vehicles (UAV, Drones) (Solutions 6–9)
Solutions 6–9 summarize spatially discrete solutions outside the hospital. Alternate care facilities (Solution 6) provide safe sites for quarantine and screening (Figure 11). Roaming POCT (Solution 7) will benefit from progressively smaller, smarter, and faster POC technologies adapted for remote applications, such as airborne (45–49), ambulances (50, 51, 91), space (52–55, 92), and wearables (52–55, 93, 94). Cummins et al. (95) reviewed POC technologies suitable or potentially usable in the micro-gravity international space station, during space flight, and possibly within confined space colonies subject to different gravitational fields (52).
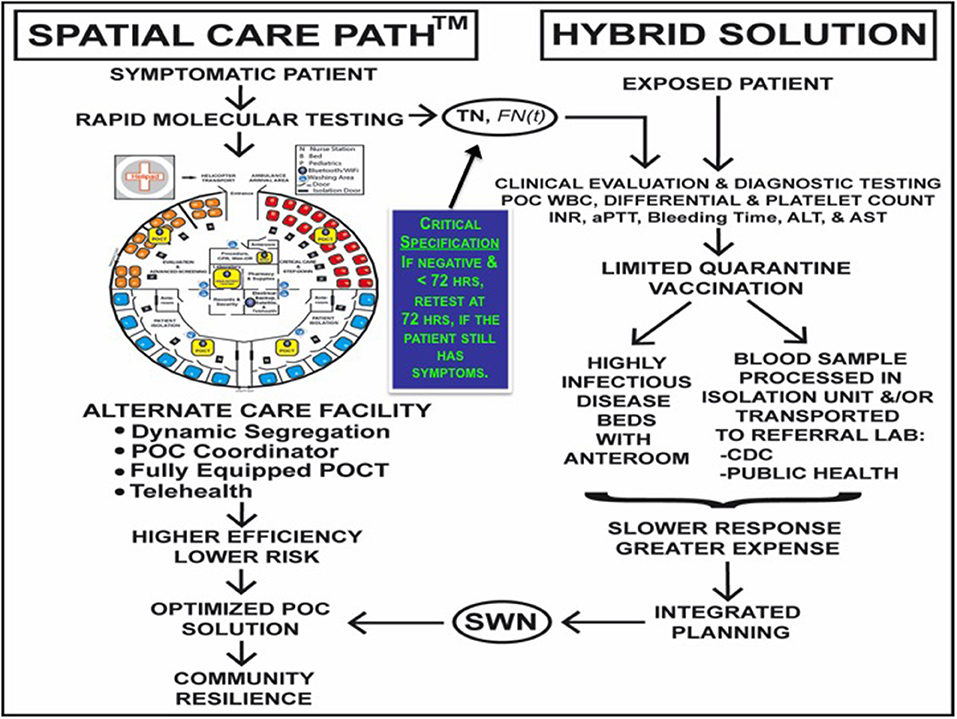
Figure 11. Spatiotemporal approach to highly infectious diseases with alternate care facility. This GCP design incorporates immediate high sensitivity POC molecular diagnostic testing to rule out the highly infectious disease, with the caveat that the false negative rate is a function of time and may be high initially when the viral load is evolving, requiring repeat testing. The alternate care facility (left), which is replete with POCT, provides a discrete spatial solution that is safe for patients, healthcare personnel, and the community. Screening, hospital quarantine, and specimen transport to distant referral laboratories for diagnosis (right) slow down patient throughput. Image reproduced with permission of Knowledge Optimization.
Regardless of where they are generated, test results must be connected (Solution 8) for seamless bidirectional electronic communications. Physical linkages using novel unmanned aerial vehicles (UAVs) (Solution 9) show promise for delivery of critical medical supplies, transport of laboratory samples, and spatial solutions for accessing challenging topographies, remote sites, and isolated islands. In limited-resource settings, drones can help optimize healthcare SWNs, document the status of disasters, and track outbreaks of highly infectious diseases.
Pilot studies show feasibility of drones for specimen transport (59–62) and instruments for rapid pathogen detection during flight (63). Zipline (https://flyzipline.com/) UAVs supply blood, vaccines, and drugs throughout Ghana and Rwanda in Africa. They claim 500 deliveries per day serving 11 million, with 30 min response time from app-initiated order. The drones have a 1.8 kg payload, fly 40,000 km per week (cumulatively over 1 million km), launch at 100 km/h, drop the payload at the target, and then return to base. This system could transport POC devices, reagents, and QC materials to remote sites within the 80 km service radii of base stations, which now cover most of Rwanda and central Ghana.
Geospatial Practice—Demographic Care Units, Geographic Risk Assessment, and National POCT Policy and Guidelines (Solution 10)
10.A. Demographic Care Units
The demographic care unit (DCU) concept gives policy makers a means of identifying the geographic locations most in need of POCT to improve standards of care (64, 65). DCU scoring can be applied to any country on a periodic basis to help identify inequities in regions of highest need. Thai provincial demographic features, health resources, and poverty measures were obtained through web research, published documents, and data from the MOPH and Office of the National Economic and Social Development Board. The number of people per individual health resource in each Thai province, that is, in each DCU, was, calculated utilizing the mid-year population.
Health resource characteristics comprised the number of people per primary care unit, hospital bed, medical doctor, registered nurse, technical nurse, pharmacist, and medical technologist in a given province. The total number of resource categories above the cut-off was determined to obtain the score for each DCU. Summary statistical analyses included range, minimum, maximum, mean, median, percentile, and standard deviation. The seventy-fifth percentile of people per health resource in a province defined the cut-off for interpretation of whether a DCU qualified as having a health resource deficiency. Also reported are poverty lines, number of poor people, poor people ratio, poor people times health resource score, and death rates.
The higher the score, the more inadequate the health resource was relative to the number of people served. Figure 12 shows the striking geographic inequities in healthcare resources, the most deficient located mainly in The Northeast (Isaan), where most DCU scores were 5, 6, or 7. One advantage of this approach lies in its ability to use current data to update priorities for the geographic placement of POCT. Medical technologists who operate POC technologies should accompany POCT placements. Combined DCU and SWN analysis (see Solution 1), educational programs, a Thai language book with chapters on POCT (96, 97), and a collaborative strategy implemented by cardiologists, academicians, and industry significantly improved the standard of care for acute coronary syndrome patients, as described earlier.
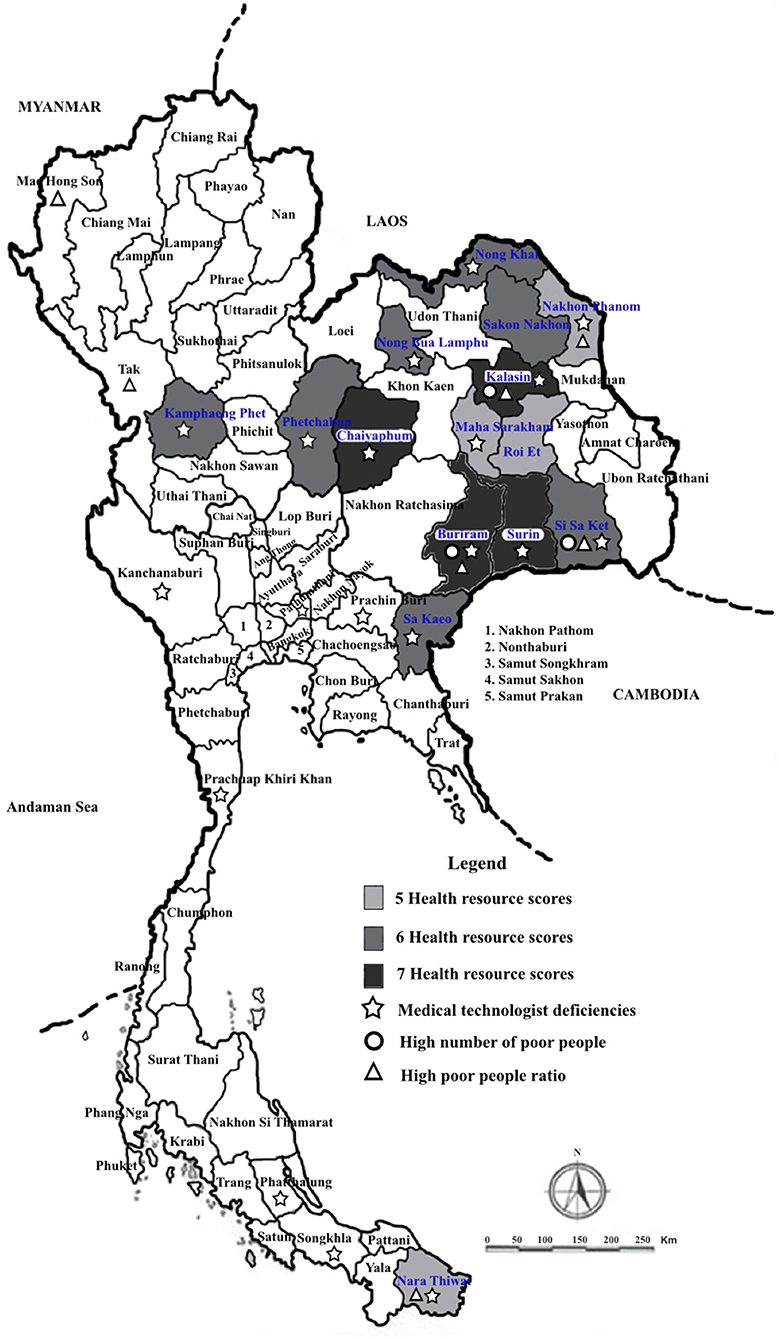
Figure 12. Demographic care unit scoring for Thailand. Image reproduced with permission of Knowledge Optimization.
10.B. Geographic Risk Assessment
The Southern Thailand case study in Figure 13 illustrates how to identify high risk in coastal SWNs. The map on the left shows the Phang Nga Province SWN, and on the right, Khao Lak and other areas hit hardest (in red) by the tsunami generated during the 2004 Andaman (Indian) Sea Earthquake. The center identifies hospitals and healthcare resources at risk. The earthquake was the third largest ever recorded. Waves as high as 30 meters smashed the coastal areas of Phang Nga Province and flooded the interior. The right panel shows blocked roads and routes to alternate hospitals.
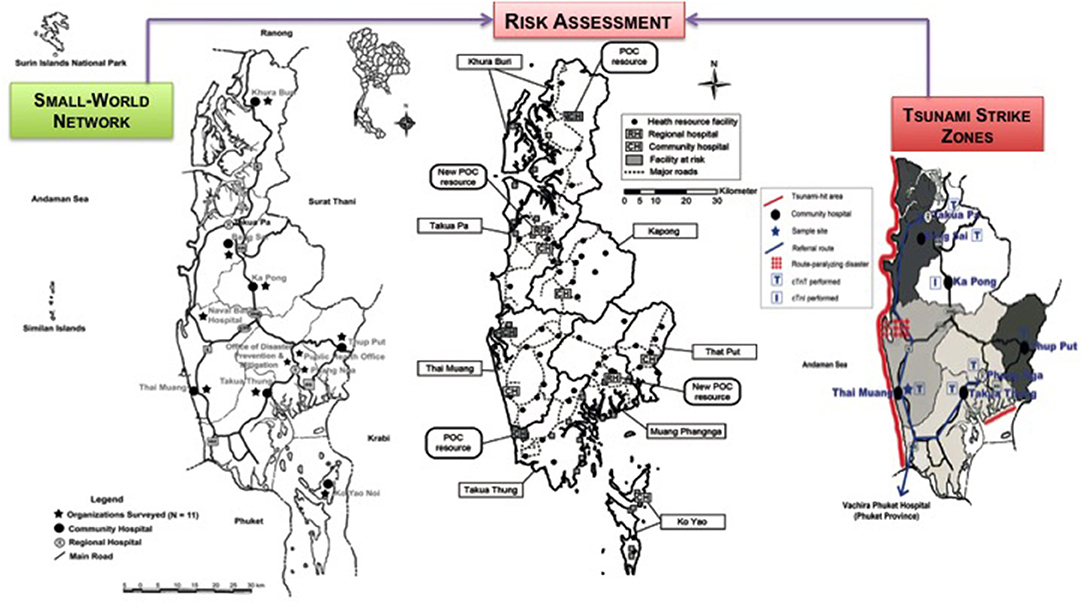
Figure 13. Risk assessment in Phang Nga Province hit by the massive Andaman Sea Tsunami. Image reproduced with permission of Knowledge Optimization.
While one account touted successful disaster response at the Takuapa District Hospital slightly north and inland in Phang Nga Province (98), several others more objectively documented broad failure and inadequate preparedness in community hospitals, especially coastal resorts and muubaans (villages) hit by the tsunami (67, 68, 99, 100). For example, the care of critical patients was compromised by lack of blood gas instruments throughout all community hospitals, and at the time, an analyzer that performs blood gases (pO2, pCO2), pH, and electrolyte (e.g., K+, Na+, and Ca+2) tests simultaneously on whole blood was inoperable in the Takuapa District Hospital, to which hundreds of critically injured tsunami victims were transferred (67, 68).
We (GK with three Chulalongkorn demography and economics graduate students on field survey) talked with the only doctor on call at one of the small coastal community hospitals in Phang Nga when the tsunami hit—roads were blocked by flood waters isolating the hospital, drugs were depleted rapidly as he triaged over 1,000 victims, diagnostic instruments were inaccessible, and staff personnel were totally inadequate, because they could not reach the hospital at the time of the crisis. The experience had a devastating psychological effect on the sole young physician in charge, who did not want to continue medical practice subsequently.
During follow-up surveys several years after the tsunami, still no blood gas testing (pO2, pCO2, pH) was found in any community hospital in Phang Nga Province (68). Default use of fingertip pulse oximeters (small battery-powered O2 saturation monitors, see https://www.youtube.com/watch?v=9ELSR7z0U4w) increased after the tsunami, but was complicated by the presence of several different brands that had been donated. In spite of dire need, the frequency of use of pulse oximeters in Phang Nga community hospitals did not match that in a survey control province, Chiang Rai, in northern Thailand (68). Detailed field studies are needed to fully assess which point-of-need diagnostic capabilities remain absent or at risk because of reagent supply chain issues, and whether, in fact, communities and ministries of health have acted responsibly to prepare for potential future disasters, such as a repeat tsunami in Southern Thailand or Indonesia.
In view of high probability of future occurrences of tsunamis from “ring of fire” earthquakes exacerbated by global warming and rising ocean levels encroaching on shore areas, geographic risk assessment should be performed in vulnerable coastal settings, particularly those with dense native population clusters and high tourist capacities. Point-of-care testing results are immediate and can be deployed rationally to support decision making based on diagnostic evidence obtained at points of need.
Similar delayed and inadequate response occurred during Hurricane Katrina in the United States (1), because of the complexities of the coastal topography, severe flooding, and submerged metropolitan areas (Figure 14). Knowing patient status and diagnosis rapidly on site can speed response and allay anxiety, so medical staff can endure the nearly impossible stress, not to mention save lives and mitigate economic and cultural losses. Following Superstorm Sandy, the largest Atlantic hurricane on record, which flooded several basement clinical laboratories in New York City, United Healthcare Workers recognized POCT as the first line of defense for emergency preparedness and risk mitigation (101), but public health agencies have not followed up adequately.
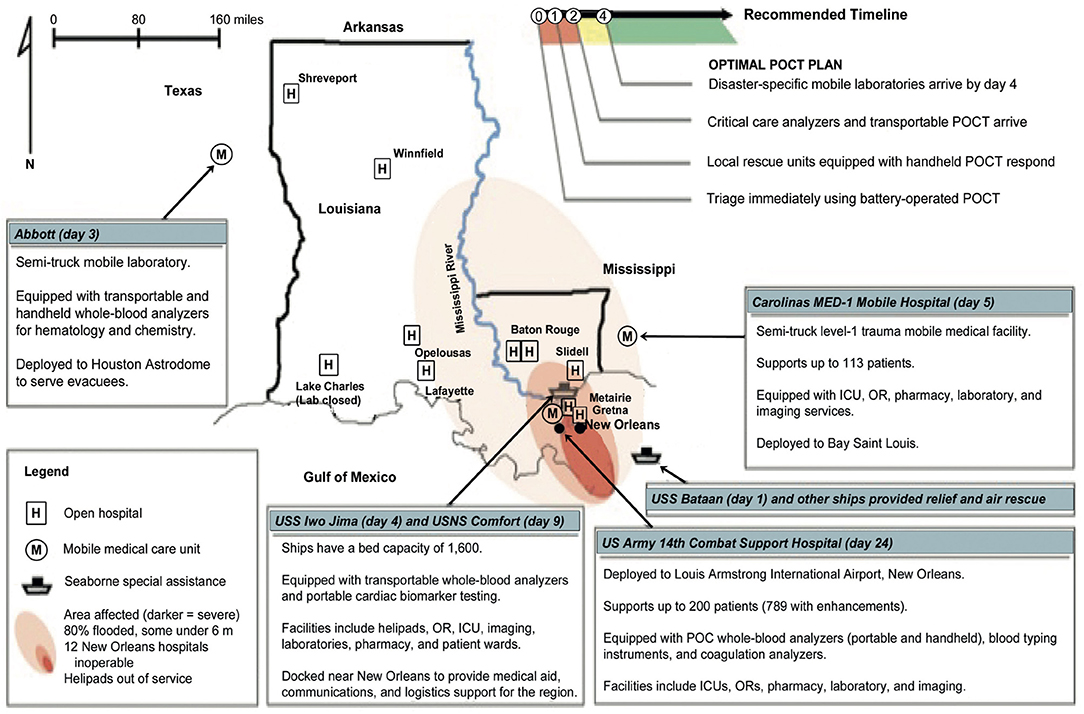
Figure 14. Geographic Risk—Delayed response during Hurricane Katrina in the US. The inset (upper right) suggests how the response timeline could have been improved using POCT resources. Image reproduced with permission of Knowledge Optimization.
10.C. National POCT Policy and Guidelines
A Malaysian national steering committee led by Dr. Baizurah produced the world's first national POCT policy and guidelines (71, 72), which was published in the English language. Please access the document directly (at http://www.moh.gov.my/moh/images/gallery/Garispanduan/National_Point_of_Care_Testing.pdf) to appreciate and understand the scope, depth, and breadth needed to harmonize POCT across the geopolitical expanse of the South China and Natuna Seas that separate mainland Malaysia and two of its states on the island of Borneo, a distance >1,000 miles (1,609 kilometers). The Malaysian initiative bases its guidelines on ISO (International Organization for Standardization) documents. Recognizing the importance of geopolicy in the use of new POC technologies can accelerate implementation, funding, and acceptance. Our book chapter (72) summarizes the status of other nation state-oriented policy and guidelines worldwide.
The Hybrid Laboratory and Point-of-Careology (Solutions 11 and 12)
Solutions 11 and 12 chronicle nearly three decades of spatial movement of whole-blood analysis to the bedside in the OR and ICU, known as the “hybrid laboratory” (73–76), progressing to the present day, when POC culture (11, 15, 18) has emerged as a new medical specialty, “point-of-careology”, in China (21, 77). The point-of-careologist performs testing and uses diagnostic results directly where he or she encounters patients in daily and emergency practice. Point-of-careologists also will fulfill multifunctional needs in confined spatial environments, such as the Mars colony and during extended extraterrestrial voyages. Physician astronauts (102) should be trained well in POCT and its quality assurance to anticipate this future.
Conclusions and Limitations
Critical Space and Time
POCT is inherently spatial, that is, performed at or near points of need, and intrinsically temporal, thereby accelerating critical evidence-based decisions. Point-of-care technologies have progressed well beyond mobile diagnostics to become a means for spatially and temporally optimizing preparedness, emergency management, and outbreak control. Advanced mobile technologies, such as modular assays attached to smartphones, will permeate healthcare.
However, without strategic planning, poorly organized SWNs will still suffer from disparities of access, service, rescue, response, and resilience. Geospatial care paths with integration of POC and other tests (103) selected to meet demographic priorities (64, 65) can fine-tune SWN logistics to accelerate point-of-need response and decision making. Additionally, parallel knowledge of geospatial distributions of diseases can aid the development, selection, and placement of POC tests specific for populations at risk.
Resource Constraints
Increasingly, resources appear inadequate to meet the needs of future world population growing by 83 million people each year and expected to hit 10 billion by 2050. Demographers debate whether this mid-century world population will expand or contract (81). Either pathway spells trouble for healthcare, including island nations facing the threat of inundation of coastal communities because of global warming. Economic reserves likely will shrink absolutely or relatively with respect to these overwhelming burdens, diminishing access and exacerbating healthcare disparities. Point-of-need testing and geospatial innovations have the potential to alleviate these imbalances.
Strategic Capacity
Surveys of public health curricula (88, 89) identified root cause deficiencies of POCT curriculum and geospatial education in schools and programs of public health. Inadequate professional training means that the workforce of POC specialists and geospatial scientists will not meet future needs. This deficiency must be corrected (88, 89, 104). Education of public health professionals in the principles and practice of POCT (78, 79) should start early, in order to generate trained, inspired, and enabled personnel who can make immediate evidence-based decisions in the field during crises and at community care sites.
Geospatial·POC
Disasters, outbreaks, complex crises, climate warming, terrorism, and rampant shootings have emerged as global norms. With over seven billion people in the world, highly clustered communities, dense collections of inhabitants, and numerous populations at risk, the overall goal is to integrate principles and practice of POCT and initiate a fresh approach to public health preparedness and community resilience (105, 106). This applies to limited-resource and advanced settings alike, such as urgent care on the islands of Indonesia and weather disasters like the Great Bangkok Flood (66) in large metropolitan areas.
Since 1980 in the US alone, 246 disasters have exceeded $1 billion in costs, totaling $1.6 trillion (107). Substantial human, economic, and social losses will continue to burden the global community. Responding rapidly will save lives, valuable resources, and irreplaceable culture. Therefore, geospatial·POC preparedness should become the new norm. In fact, two recent books point us toward preparedness (108, 109), but omit POC technologies, geospatial science, and integrated time-space solutions. The building blocks in Table 1 used in conjunction with complementary educational curricula (88, 89) will fill these gaps and enable rapid response solutions at points of need worldwide.
Public Health Recommendations
Public health professionals appreciate the importance of GISs and visualized health data in determining inequalities and inaccessibility issues and in addressing infectious disease epidemiology (110, 111). Geospatial solutions include isolation laboratories and diagnostics isolators for highly infectious diseases, but limited availability of this vital critical care support puts population clusters at risk (90, 112, 113). Therefore, key principles and value propositions for future implementation of geospatial science and POCT include—
• Educators in emergency management and preparedness should teach geospatial science and POCT principles, so that interventional public health practitioners will understand rapid response, point-of-need diagnostics, quality assurance, immediate decision making, isolation for critical treatment, and a fundamental need—spatial and temporal resilience.
• Spatially isolated laboratories and diagnostics isolators for the support of critically ill patients with highly infectious diseases, such as Ebola, can be positioned geographically to serve regional population clusters. These resources are distinctively and uniquely spatial, since they define biohazard “safe houses.”
• One can optimize these facilities by assessing travel, exposure (e.g., airline passengers), and treatment options in advance of outbreaks and epidemics, then equipping the facilities properly with POCT and personnel experienced in testing while wearing PPE, so they can operate instruments independently while in isolation.
• POCT-enabled spatial grids can locate sentinel cases and establish geographic limits of outbreaks. Integrating POCT plus GIS with ring vaccination will enable unique synergism for quickly detecting sentinel cases, geospatial tracking, and containment of epidemics.
• Analysis of population clusters, hubs, topographic features, and EMS routes in SWNs helps determine best sties for POC diagnostics, including devices on ambulance and other rescue vehicles or aircraft. These technologies also are needed for secure space flight.
• Small-world networks with multidimensional connectivity facilitate efficient and effective placement of POCT, which improves response time, rescue, diagnosis, treatment, and spatial resilience by accelerating evidence-based decision making at points of need.
• Visual logistics help design delivery systems capable of efficiently addressing individual crises, such as acute myocardial infarction, while analyzing healthcare strategies for difficult public health problems, such as Ebola, MERS-CoV, and H7N9; HIV, TB, and malaria; and differentiating concurrent Ebola infections from the others during outbreaks.
• Documenting transport time in contour maps and GISs facilitates EMS radio dispatching, regional coverage, and emergency transport, especially in the absence of helicopter transport or when ambulance service is inadequate.
• Placement of POC devices, ECG, and diagnostic tests on board ambulances with physician-guided prehospital diagnosis by telemetry accelerates pathways to tertiary care and intervention.
• Space-time transformations can identify POCT-facilitated (e.g., AMI ruled in by positive POC cTn testing) transport “short-cuts” for critically ill patients to referral centers for cardiologist intervention. Parallel analysis produces solutions for diabetes and other public health challenges.
• Use of GISs to position POCT close to where patients live and work facilitates triage and rapid therapeutic turnaround time during emergencies, disasters, and outbreaks. They can expedite urgent primary care and assist remote population clusters in need of access.
• Spatial care paths™ with POC devices positioned cleverly upstream speed transport from primary care to referral centers by bypassing geographic bottlenecks and time-consuming interruptions (e.g., roads flooded by seasonal downpours).
• Geospatial care paths™ encompass features of population clusters, demographic fluxes, and local POCT hubs to decrease therapeutic turnaround time by expediting intermediate stages of inefficient routines, government protocols, or perfunctory patient evaluations.
• Geographic information systems can provide backbones for these care paths and also for dispatching drones, routing flight paths, and delivering medical and POC supplies in remote or inaccessible areas.
• Analysis of demographic care units can guide ministries of health in providing adequate primary care access, hospital beds, physicians, nurses, pharmacists, medical technologists, POC coordinators, POC technologies, and other vital resources, especially when prioritizing rural regions most in need using fresh data renewed each year.
• Onsite, rapid, and highly sensitive/specific POCT will help identify highly infectious diseases, stop outbreaks, and establish topographic perimeters of contagion. False negatives as a function of time, FN(t), must be minimized early on by assuring high sensitivity for ruling out infection of exposed patients.
• Alternate care facilities equipped with POCT can enhance community resilience in the event of quarantine, disasters, or complex crises (e.g., the earthquake-tsunami-radiation leak on coastal Japan).
Closing Insights
High Impact
The POCT industry has grown to $31.4 billion worldwide, projected at $37.0 billion in 2021 (114). The POCT growth rate in the Asia-Pacific, the region of several field research reports in Table 1, is 14.2%. Numerous POC technologies in the pipeline soon will emerge. For example, NIH-BARDA will award a $20 million prize, the largest in United States history, for POC inventions addressing antimicrobial resistance (see https://dpcpsi.nih.gov/AMRChallenge). The idea is to administer targeted therapy immediately to individual patients, avoid indiscriminate use of antibiotics in the population at large, and save precious healthcare funds.
Future Direction
Best use of POCT occurs when shrewdly selecting, combining, and integrating solutions in Table 1 to create value, so that patient care is shifted toward the home, primary care, and community; critical care becomes more efficient; and deaths are avoided during public health crises. The education of young physicians in evidence-based medicine when delivering rapid emergency care in isolated remote settings constitutes adequate indication for implementing POCT. Inventions, innovations, and economies of scale will reduce POC manufacturing, supply chain, and utilization costs. In China the new medical field called “point-of-careology” (77) integrates diagnostics for rapid decision making at points of need by medical specialists enabled with POC technologies. This symbiosis will benefit professionals in other resource-, population-, and topography-challenged settings, such as countries with vast geographies and island nations, to care equitably for burgeoning populations.
Standards of Care
If public health professionals, geospatial scientists, and POCT specialists join efforts to use the solutions enumerated in Table 1, collaborative teamwork can create resilient and equitable healthcare in the community at points of critical need during emergencies, outbreaks, epidemics, and disasters, as well as more efficient primary, urgent, and emergency community care. Importantly, they will improve public health and crisis standards of care.
Author Contributions
The author confirms being the sole contributor of this work and has approved it for publication.
Conflict of Interest
The author declares that the research was conducted in the absence of any commercial or financial relationships that could be construed as a potential conflict of interest.
Acknowledgments
The author thanks the creative students who participate in the POCT·CTR and contribute to knowledge of point of care, especially William Ferguson, MS (Spatial Sciences Institute, USC Dornsife), for his contributions to POC geospatial sciences while a research assistant in the NIH NIBIB U54 UC Davis POC Pathogen Detection Technologies Center (GK, PI and Director) and the POCT·CTR, School of Medicine, UC Davis. Amanullah Zadran provided useful comments. The author is deeply grateful for a Fulbright Scholar Award and the many research and lecturing opportunities it provided in Thailand and SE Asia. Figures and the table are provided courtesy and permission of Knowledge Optimization™, Davis, California.
References
1. Kost GJ, Tran NK, Tuntideelert M, Kulrattanamaneeporn S, Peungposop N. Katrina, the tsunami, and point-of-care testing: optimizing rapid response diagnosis in disasters. Am J Clin Pathol. (2006) 126:513–20. doi: 10.1309/NWU5E6T0L4PFCBD9
2. Kost GJ. Newdemics, public health, small-world networks, and point-of-care testing. Point Care. (2006) 5:138–44.
3. Kost GJ, Suwanyangyuen A, Kulrattanamaneeporn S. The hill tribes of Thailand: synergistic health care through point-of-care testing, small-world networks, and nodally flexile telemedicine. Point Care. (2006) 5:199–204. doi: 10.1097/01.poc.0000232580.36762.68
4. Guandlapalli A, Ma X, Stat M, Benuzillo J, Pettty W, Greenberg R, et al. Social network analyses of patient-healthcare worker interactions: implications for disease transmission. AMIA Symp Proc. (2009) 213–17.
5. Kost GJ, Kost LE, Suwanyangyuen A, Cheema SK, Corbin C, Sumner S, et al. Emergency cardiac biomarkers and point-of-care testing: optimizing acute coronary syndrome care using small-world networks in rural settings. Point Care. (2010) 9:53–64. doi: 10.1097/POC.0b013e3181d9d45c
6. Yu JN, Brock TK, Mecozzi DM, Tran NK, Kost GJ. Future connectivity for disaster and emergency point of care. Point Care. (2010) 9:185–92. doi: 10.1097/POC.0b013e3181fc95ee
7. Kost GJ, Kanoksilp A, Mecozzi DM, Sonu R, Curtis C, Yu JN. Point-of-need hemoglobin A1c for evidence-based diabetes care in rural small-world networks: Khumuang Community Hospital, Buriram, Thailand. Point Care. (2011) 10:28–33. doi: 10.1097/POC.0b013e3182078402
8. Kleczkowski A, Oles K, Gudowska-Nowak E, Gilligan CA. Searching for the most cost-effective strategy for controlling epidemics spreading on regular and small-world networks. R Soc Interface. (2012) 9:158–69. doi: 10.1098/rsif.2011.0216
9. Kost GJ. Theory, principles, and practice of optimizing point-of-care small-world networks. Point Care. (2012) 11:96–101. doi: 10.1097/POC.0b013e31825a25b5
10. Kost GJ, Katip P, Tangchit T. Human immunodeficiency virus, population dynamics, and rapid strategies for medical diagnosis in the northern most province of Thailand—Chiang Rai. J Demography. (2012) 28:37–63.
11. Kost GJ, Katip P, Vanasith K, Negash H. The final frontier for point of care: performance, resilience, and culture. Point Care. (2013) 12:1–8. doi: 10.1097/POC.0b013e318266b7fe
12. Kost GJ. Chapter 49: using small-world networks to optimize preparedness, response, and resilience. In: Kost GJ, Curtis CM, editors. Global Point of Care: Strategies for Disasters, Emergencies, and Public Health Preparedness. Washington, DC: AACC Press-Elsevier (2015). p. 539–68.
13. Girdwood SJ, Nichols BE, Moyo C, Crompton T, Chimhamhiwa D, Rosen S. Optimizing viral load testing access for the last mile: geospatial cost model for point of care instrument placement. PLoS ONE. (2019) 14:e0221586. doi: 10.1371/journal.pone.0221586
14. Mackenzie SLC, Hinchey DM, Cornfortrh KP. A public health service-learning capstone: ideal for students, academia and community. Front Public Health. (2019) 7:1–7. doi: 10.3389/fpubh.2019.00010
15. Kost GJ, Ferguson WJ, Kost LE. Principles of point of care culture, the spatial care path™, and enabling community and global resilience. e-Journal IFCC. (2014) 25:134–53.
16. Kost GJ, Ferguson WJ, Hoe J, Truong A-T, Banpavichit A, Kongpila S. The Ebola Spatial Care Path™: accelerating point-of-care diagnosis, decision making, and community resilience in outbreaks. Am J Disaster Med. (2015) 10:121–43. doi: 10.5055/ajdm.2015.0196
17. Kost GJ, Ferguson WJ. Spatial Care Paths™ strengthen links in the chain of global resilience: disaster caches, prediabetes, Ebola virus disease, and the future of point of care. Point Care. (2015) 15:43–58. doi: 10.1097/POC.0000000000000080
18. Kost GJ, Pratumvinit B. Diabetes Spatial Care Paths™, leading edge HbA1c testing, facilitation thresholds, proactive-preemptive strategic intelligence, and unmanned aerial vehicles in limited-resource countries. Point Care. (2017) 16:12–31. doi: 10.1097/POC.0000000000000122
19. Kost GJ, Zadran A, Duong TTB, Pham TT, Ho AVD, Nguyen NV, et al. Point-of-care diagnosis of acute myocardial infarction in Central Vietnam: international exchange, needs assessment, and Spatial Care Paths™. Point Care. (2018) 17:73–92. doi: 10.1097/POC.0000000000000167
20. Ventura IJ, Zadran A, Zadran L, Duong TB, Tang TP, Kost GJ. Rapid diagnosis and effective monitoring of diabetes mellitus in Central Vietnam: point-of-care needs, improved patient access, and spatial care path integration for enhanced public health. Point Care. (2019) 18:1–8. doi: 10.1097/POC.0000000000000178
21. Kost GJ. Point-of-care Cardiac biomarkers in Vietnam (the Philippines, Taiwan, and Thailand). In: Point of Care in Limited-Resource Settings Symposium, 26th International Molecular Med Tri•Con. San Francisco, CA: Cambridge Healthtech Institute (2019). p. 21.
22. Grusky O, Roberts KJ, Swanson AN, Rhoades H, Lam M. Staff strategies for improving HIV detection using mobile HIV testing. Behav Med. (2010) 35:101–11. doi: 10.1080/08964280903334501
23. Goswami ND, Hecker E, Holland DP, Naggle S, Cox GM, Mosher A, et al. Feasibility and willingness-to-pay for integrated community-based tuberculosis testing. BMC Infect Dis. (2011) 11:305–10. doi: 10.1186/1471-2334-11-305
24. Ferguson WJ, Louie RF, Tang CS, Vy JH, Wallace AP, Kost GJ, et al. Geographic information systems can enhance crisis standards of care during complex emergencies and disasters: a strategy for global positioning system-tracked, H2 fuel cell-powered, and knowledge-optimized point-of-care medical intelligence. Point Care. (2012) 11:184–90. doi: 10.1097/POC.0b013e3182666da9
25. Nagata T, Kimura Y, Ishii M. Use of a geographic information system (GIS) in the medical response to the Fukushima nuclear disaster in Japan. Prehosp Disaster Med. (2012) 27:213–5. doi: 10.1017/S1049023X1200060X
26. Alegana VA, Atkinson PM, Wright JA, Kamwi R, Uusiku P, Katokele S, et al. Estimation of malaria incidence in northern Namibia in 2009 using Bayesian conditional-autoregressive spatial-temporal models. Spatial Spatio-temporal Epidemiol. (2013) 7:25–36. doi: 10.1016/j.sste.2013.09.001
27. Yao J, Agadjanian V, Murray AT. Spatial and social inequities in HIV testing utilization in the context of rapid scale-up of HIV/AIDS services in rural Mozambique. Health Place. (2014) 28:133–41. doi: 10.1016/j.healthplace.2014.04.007
28. Ferguson WJ, Louie RF, Katip P, Kost GJ. Chapter 35: use of geographic information systems for placement and management of point-of-care technologies in small-world networks. In: Kost GJ, Curtis CM, editors. Global Point of Care: Strategies for Disasters, Emergencies, and Public Health Preparedness. Washington DC: AACC Press-Elsevier (2015). p. 393–404.
29. Ferguson W, Kost G. Streamlining Health Access Through Point of Care Technologies: A Spatial Model. Davis, CA: Point-of-Care Testing Center for Teaching and Research (2015).
30. Ferguson WJ, Kemp K, Kost G. Using a geographic information system to enhance patient access to point-of-care diagnostics in a limited-resource setting. Int J Health Geogr. (2016) 15:1–12. doi: 10.1186/s12942-016-0037-9
31. Larocca A, Visconti RM, Marconi M. Malaria diagnosis and mapping with m-Health and geographic information systems (GIS): evidence from Uganda. Malar J. (2016) 15:520:1–12. doi: 10.1186/s12936-016-1546-5
32. Lin JC, Kost GJ, Ferguson W. Bio-innovation in Taiwan, the first survey of point-of-care professional needs, and geospatially enhanced resilience in at-risk settings. Point Care. (2017) 16:78–88. doi: 10.1097/POC.0000000000000134
33. Kuupiel D, Adu KM, Apiribu F, Bawontuo V, Adogboba DA, Ali KT, et al. Geographic accessibility to public health facilities providing tuberculosis testing services at point-of-care in the upper east region, Ghana. BMC Public Health. (2019) 19:718:1–12. doi: 10.1186/s12889-019-7052-2
34. Hill CE, Burd EM, Kraft CS, Ryan EL, Duncan A, Winkler AM, et al. Laboratory test support for Ebola patients within a high-containment facility. Lab Med. (2014) 45:e109–11. doi: 10.1309/LMTMW3VVN20HIFS
35. Kost GJ, Ferguson WJ, Truong A-T, Prom D, Hoe J, Banpavichit A, et al. The Ebola Spatial Care Path™: point-of-care lessons learned for stopping outbreaks. Clin Lab Int. (2015) 39:6–14.
36. Kost GJ, Ferguson W, Truong A-T, Hoe J, Prom D, Banpavichit A, et al. Molecular detection and point-of-care testing in Ebola virus disease and other threats: a new global public health framework to stop outbreaks. Expert Rev Mol Diagnostics. (2015) 15:1245–59. doi: 10.1586/14737159.2015.1079776
37. Shorten RJ, Brown CS, Jacobs M, Rattenbury S, Simpson AJ, Mepham S. Diagnostics in Ebola virus disease in resource-rich and resource-limited settings. PLoS Negl Trop Dis. (2016) 10:1–16. doi: 10.1371/journal.pntd.0004948
38. Boonlert W, Kost GJ, Jiraviriyakul A, Tangvarasittichai S. Point-of-care testing on a mobile medical unit in northern Thailand: screening for hyperglycemia, hyperlipidemia, and thalassemia trait. Point Care. (2006) 5:164–7. doi: 10.1097/01.poc.0000232581.36762.21
39. Diers J, Kouriba B, Ladan Fofana L, Fleischmann E, Starke M, Diallo S, et al. Mobile laboratories for rapid deployment and their contribution to the containment of emerging diseases in Sub-Saharan Africa, illustrated by the example of Ebola virus disease. Med Sante Trop. (2015) 25:229–33. doi: 10.1684/mst.2015.0485
40. Mansuy JM. Mobile laboratories for Ebola and other pathogens. Lancet Infect Dis. (2015) 15:1135. doi: 10.1016/S1473-3099(15)00309-6
41. de La Vega MA, Bello A, Chaillet P, Kobinger GP. Diagnosis and management of Ebola samples in the laboratory. Expert Rev Anti Infect Ther. (2016) 14:557–67. doi: 10.1080/14787210.2016.1176912
42. Racine T, Kobinger GP. Challenges and perspectives on the use of mobile laboratories during outbreaks and their use for vaccine evaluation. Hum Vaccin Immunother. (2019) 20:1–5. doi: 10.1080/21645515.2019.1597595
43. Kost GJ, Sakaguchi A, Curtis C, Tran NK, Katip P, Louie RF. Enhancing standards of care using innovative point-of-care testing. Am J Disaster Med. (2011) 6:351–68. doi: 10.5055/ajdm.2011.0074
44. Kost GJ. Chapter 24: point-of-care testing for Ebola and other highly infectious threats: principles, practice, and strategies for stopping outbreaks. In: Shephard M, editor. A Practical Guide to Global Point-of-care Testing. Canberra: CSIRO (Commonwealth Scientific and Industrial Research Organization) (2016). p. 291–305.
45. Herr DM, Newton NC, Santrach PJ, Hankins DG, Burritt MF. Airborne and rescue point-of-care testing. Am J Clin Pathol. (1995) 104 (4 Suppl. 1):S54–8.
46. Davey AL, Macnab AJ, Green G. Changes in pCO2 during air medical transport of children with closed head injuries. Air Med J. (2001) 20:27–30. doi: 10.1016/S1067-991X(01)70043-0
47. Di Serio F, Petronelli MA, Sammartino E. Laboratory testing during critical care transport: point-of-care testing in air ambulances. Clin Chem Lab Med. (2010) 48:955–61. doi: 10.1515/CCLM.2010.190
48. Louie RF, Ferguson WJ, Curtis CM, Vy JH, Tang CS, Kost GJ. Effects of environmental conditions on point-of-care cardiac biomarker test performance during a simulated rescue: implications for emergency and disaster response. Am J Disaster Med. (2013) 8:205–12. doi: 10.5055/ajdm.2013.0126
49. Tideman PA, Tirimacco R, Senior DP, Setchell JJ, Huynh LT, Tavella R, et al. Impact of a regionalised clinical cardiac support network on mortality among rural patients with myocardial infarction. Med J Aust. (2014) 200:157–60. doi: 10.5694/mja13.10645
50. Sorensen JT, Stengaard C. Chapter 50: prehospital application of cardiac biomarkers for decision support of patients with suspected acute myocardial infarction. In: Kost GJ, Curtis CM, editors. Global Point of Care: Strategies for Disasters, Emergencies, and Public Health Preparedness. Washington, DC: AACC Press-Elsevier (2015). p. 569–76.
51. Rasmussen MB, Stengaard C, Sørensen JT, Riddervold IS, Hansen TM, Giebner M, et al. Predictive value of routine point-of-care cardiac troponin T measurement for prehospital diagnosis and risk-stratification in patients with suspected acute myocardial infarction. Eur Heart J Acute Cardiovasc Care. (2017) 8:299–308. doi: 10.1177/2048872617745893
52. Kost GJ. How POCT Improves Care and Educates Physicians: Exciting Contemporary Examples and Innovative Opportunities, including Point-of-Careology on Mars. In: 12th Annual Convention. Manila: Philippines Council for Quality Assurance in Clinical Laboratories (2015).
53. Canadian Consortium. Bio-Analyzer: Near Real-time Biomedical Results from Space to Earth. (2018). Available online at: http://asc-csa.gc.ca/eng/iss/bio-analyzer.asp
54. Roda A, Mirasoli M, Guardigli M, Zangheri M, Caliceti C, Calabria D, et al. Advanced biosensors for monitoring astronauts' health during long-duration space missions. Biosens Bioelectron. (2018) 111:18–26. doi: 10.1016/j.bios.2018.03.062
55. Zangheri M, Mirasoli M, Guardigli M, Di Nardo F, Anfossi L, Baggiani C, et al. Chemiluminescence-based biosensor for monitoring astronauts' health status during space missions: results from the International Space Station. Biosens Bioelectron. (2019) 129:260–68. doi: 10.1016/j.bios.2018.09.059
56. Kamanga A, Moono P, Stresman G, Mharakurwa S, Shiff C. Rural health centers, communities and malaria case detection in Zambia using mobile telephones: a means to detect potential reservoirs of infection in unstable transmission conditions. Malar J. (2010) 9:96. doi: 10.1186/1475-2875-9-96
57. Laksanasopin T, Gup TW, Sia SK. Chapter 36: integrating diagnostics tests and connectivity to enable disease diagnosis and tracking in remote settings. In: Kost GJ, Curtis CM, editors. Global Point of Care: Strategies for Disasters, Emergencies, and Public Health Preparedness. Washington, DC: AACC Press-Elsevier (2015). p. 405–10.
58. Smith S, Oberholzer A, Korvink JG, Mager D, Land K. Wireless colorimetric readout to enable resource-limited point-of-care. Lab Chip. (2019) 19:3344–53. doi: 10.1039/C9LC00552H
59. Amukele TK, Street J, Carroll K, Miller H, Zhang SX. Drone transport of microbes in blood and sputum laboratory specimens. J Clin Microbiol. (2016) 54:2622–5. doi: 10.1128/JCM.01204-16
60. Amukele TK, Sokoll LJ, Pepper D, Howard DP, Street J. Can unmanned aerial systems (drones) be used for the routine transport of chemistry, hematology, and coagulation laboratory specimens? PLoS ONE. (2015) 10:e0134020. doi: 10.1371/journal.pone.0134020
61. Amukele TK, Hernandez J, Snozek CLH, Wyatt RG, Douglas M, Amini R. Drone transport of chemistry and hematology samples over long distances. Am J Clin Pathol. (2017) 148:427–35. doi: 10.1093/ajcp/aqx090
62. Amukele T. Current state of drones in healthcare: challenges and Opportunities. J Appl Lab Med. (2019) 4:296–8. doi: 10.1373/jalm.2019.030106
63. Priye A, Wong S, Bi Y, Carpio M, Chang J, Coen M, et al. Lab on a drone: toward pinpoint deployment of smartphone-enabled nucleic acid-based diagnostic for mobile healthcare. Anal Chem. (2016) 88:4651–60. doi: 10.1021/acs.analchem.5b04153
64. Kost GJ, Peungposop N, Kulrattanamaneeporn C, Wongboonsin K, Charuruks N, Surasiengsunk S, et al. Chapter 3: minimizing health problems to optimize the demographic dividend: the role of point-of-care testing. In: Wongboonsin K, Guest P, editors. The Demographic Dividend: Policy and Options for Asia. Chulalongkorn: Chulalongkorn University Printing House (2005). p. 56–89.
65. Kost GJ, Katip P, Kanokslip A, Mecozzi DM. A new demographic strategy for point-of-need medical testing: linking health resource scores, poverty levels, and care paths. J Demography. (2011) 27:1–31.
66. Kost GJ, Katip P, Vinitwatanakhun C. Diagnostic testing strategies for health care delivery during the Great Bangkok Flood and other weather disasters. Point Care. (2012) 11:191–9. doi: 10.1097/POC.0b013e318265f255
67. Kost GJ, Katip P, Curtis C. Strategic point-of-care requirements of hospitals and public health for preparedness in regions at risk. Point Care. (2012) 11:114–8. doi: 10.1097/POC.0b013e31825a2442
68. Kost GJ, Katip P, Kulrattanamaneeporn S, Gentile N. Point-of-care testing value proposition for disaster preparedness in small-world networks: post-Tsunami Phang Nga Province, Coastal Thailand. Pont Care. (2013) 12:9–22. doi: 10.1097/POC.0b013e318265f3d4
69. Pigott DM, Golding N, Mylne A, Huang Z, Henry AJ, Weiss DJ, et al. Mapping the zoonotic niche of Ebola virus disease in Africa. eLife. (2014) 3:e04395. doi: 10.7554/eLife.04395
70. Pigott DM, Millear AI, Earl L, Morozoff C, Han BA, Shearer FM, et al. Updates to the zoonotic niche map of Ebola virus disease in Africa. eLife. (2016) 45:e16412. doi: 10.7554/eLife.16412
71. Baizurah MH, The Point of Care Testing Steering Committee. National Point of Care Testing Policy and Guidelines. Putrajaya: Ministry of Health Malaysia Medical Development Division (2012). Available online at: http://www.moh.gov.my/images/gallery/Garispanduan/National_Point_of_Care_Testing.pdf
72. Kost GJ, Baizurah MH. Chapter 53: national point of care testing policy and guidelines in Malaysia, standards of care, and impact worldwide. In: Kost GJ, Curtis CM, editors. Global Point of Care: Strategies for Disasters, Emergencies, and Public Health Preparedness. Washington, DC: AACC Press-Elsevier (2015). p. 595–610.
73. Kost GJ. The hybrid laboratory: shifting the focus to the point of care. Med Lab Obs. (1992) 24 (Suppl. 9):17–21.
74. Kost GJ. The hybrid laboratory. The clinical laboratory of the 1990s is a synthesis of the old and the new. Arch Pathol Lab Med. (1992) 116:1002–3.
75. Kost GJ. New whole blood analyzers and their impact on cardiac and critical care. Crit Rev Clin Lab Sci. (1993) 30:153–202. doi: 10.3109/10408369309084667
76. Kost GJeditors. chapter 2: The hybrid laboratory, therapeutic turnaround time, critical limits, performance maps, and Knowledge Optimization®. In: Principles and Practice of Point-of-Care Testing. Philadelphia, PA: Lippincott Williams and Wilkins (2002). p. 13–25.
77. Liu X, Zhu X, Kost GJ, Liu J, Huang J, Liu X. The creation of Point-of-Careology. Point Care. (2019) 18:77–84. doi: 10.1097/POC.0000000000000191
78. Kost GJ. Principles and Practice of Point-of-Care Testing. Philadelphia, PA: Lippincott Williams and Wilkins (2002).
79. Kost GJ, Curtis CM. Global Point of Care: Strategies for Disasters, Emergencies, and Public Health Preparedness. Washington, DC: AACC Press-Elsevier (2015).
80. Kost GJ, Ehrmeyer SS, Chernow B, Winkelman JW, Zaloga GP, Dellinger RP, et al. The laboratory-clinical interface: point-of-care testing. Chest. (1999) 115:1140–54. doi: 10.1378/chest.115.4.1140
81. Bricker D, Ibbitson J. Empty Planet: The Shock of Global Population Decline. Danvers, MA: Crown (2019).
82. Global Preparedness Monitoring Board. A World at Risk: Annual Report on Global Preparedness for Health Emergencies. Geneva: World Health Organization (2019).
83. Kost GJ. Molecular and point-of-care diagnostics for Ebola and new threats: national POCT policy and guidelines will stop epidemics. Expert Rev Mol Diagn. (2018) 18:657–73. doi: 10.1080/14737159.2018.1491793
84. Gleason BL, Foster S, Wilt GE, Miles B, Lewis B, Cauthen K, et al. Geospatial analysis of household spread of Ebola virus in a quarantined village - Sierra Leone, 2014. Epidemiol Infect. (2017) 145:2921–29. doi: 10.1017/S0950268817001856
85. Gomez-Barroso D, Velasco E, Varela C, Leon I, Cano R. Spread of Ebola virus disease based on the density of roads in West Africa. Geospat Health. (2017) 12:552. doi: 10.4081/gh.2017.552
86. Gonzalez JP, Souris M, Valdivia-Granda W. Global spread of hemorrhagic fever viruses: predicting pandemics. Methods Mol Biol. (2018) 1604:3–31. doi: 10.1007/978-1-4939-6981-4_1
87. Lau MSY, Gibson GJ, Adrakey H, McClelland A, Riley S, Zelner J, et al. A mechanistic spatio-temporal framework for modeling individual-to-individual transmission-With an application to the 2014–2015 West Africa Ebola outbreak. PLoS Comput Biol. (2017) 13:e1005798. doi: 10.1371/journal.pcbi.1005798
88. Kost GJ, Zadran A, Zadran L, Ventura I. Point-of-care testing curriculum and accreditation for Public Health—Enabling preparedness, response, and higher standards of care at points of need. Front Public Health. (2019) 6:1–15. doi: 10.3389/fpubh.2018.00385
89. Kost GJ, Zadran A. Schools or public health should be accredited for, and teach the principles and practice of point-of-care testing. J Appl Lab Med. (2019) 4:278–83. doi: 10.1373/jalm.2019.029249
90. Burnham CD, Kwon JH, Burd EM, Campbell S, Iwen PC, Miller MB. Are we there yet? Laboratory preparedness for emerging infectious diseases. Clin Chem. (2017) 63:807–11. doi: 10.1373/clinchem.2016.265850
91. Fuzery AK, Bobyak J, Chang E, Shaman R, Venner AA. Challenges of point-of-care testing in ambulances. J Appl Lab Med. (2019) 4:293–5. doi: 10.1373/jalm.2019.029439
92. Phipps WS, Yen Z, Bae C, Sharpe JZ, Bishara AM, Nelson ES, et al. Reduced-gravity environment hardware demonstrations of a prototype miniaturized flow cytometer and companion microfluidic mixing technology. J Visualized Experiments. (2014) 93:e51743. doi: 10.3791/51743
93. Wang P, Kricka LJ. Current and emerging trends in point-of-care technology and strategies for clinical validation and implementation. Clin Chem. (2018) 64:1439–52. doi: 10.1373/clinchem.2018.287052
94. Wu A. “On vivo” and wearable clinical laboratory (sic) testing devices for emergency and critical care testing. J Appl Lab Med. (2019) 4:254–63. doi: 10.1373/jalm.2018.028654
95. Cummins BM, Ligler FS, Walker GM. Point-of care diagnostics for niche applications. Biotechnol Adv. (2016) 34:161–76. doi: 10.1016/j.biotechadv.2016.01.005
96. Kost GJ. Chapter 1: overview of point-of-care testing: goals, guidelines, and principles. In: Charuruks N, Manarom W, editors. Point of Care Testing for Thailand. Bangkok: Thai Publishers (2006). p. 1–28.
97. Kost GJ. Chapter 10: point-of-care testing in province hospitals and primary care units (PCUs): optimizing critical care and disaster response. In: Charuruks N, Manarom W, editors. Point of Care Testing for Thailand. Bangkok: Thai Publishers (2006). p. 159–77.
98. Wattanawaitunechai C, Peacock SJ, Jitpratoom P. Tsunami in Thailand—Disaster management in a district hospital. NEJM. (2005) 352:362–4. doi: 10.1056/NEJMp058040
99. VanRooyen M, Leaning J. After the Tsunami—Facing the public health challenges. NEJM. (2005) 352:435–8. doi: 10.1056/NEJMp058013
100. Bronisch T, Maragkos M, Freyer C, Müller-Cyran A, Butollo W, Weimbs R, et al. Crisis intervention after the Tsunami in Phuket and Khao Lak. Crisis. (2006) 27:42–7. doi: 10.1027/0227-5910.27.1.42
101. Kost GJ. Future Role of the Laboratory in Emergency Medicine. New York, NY: The Laboratory's Role in Emergency Medicine (Natural Disasters), The Institute for Continuing Education of the 1199SEIU League Training and Upgrading Fund, 1199SEIU United Healthcare Workers East Professional and Technical Department, Gerald W. Lynch Theater at John Jay College (2013).
103. World Health Organization. Second WHO Model List of Essential In Vitro Diagnostics. Geneva: WHO (2019).
104. Zadran A, Kost GJ. Enabling rapid intervention and isolation for patients with highly infectious diseases at points of need. J Hosp Mgt Health Policy. (2019) 4:1–7. doi: 10.21037/jhmhp.2018.12.04
105. Kost GJ. Preparedness, Point-of-care Testing, and Global Resilience: Stopping Outbreaks and Assuring Equitable Care in Africa and Southeast Asia. Davis, CA: Knowledge Optimization® (2017).
106. Kost GJ. Preparedness, Point-of-care Testing, and Resilience—Stopping Outbreaks and Assuring Equitable Care for Island and High Risk Association of Southeast Asian Nations. Davis, CA: Knowledge Optimization® (2019).
108. Katz R, Banaski JA. Essentials of Public Health Preparedness and Management. Burlington, MA: Jones and Bartlett Learning (2019).
109. McKinney S, Papke ME. Public Health Emergency Preparedness: A Practical Approach for the Real World. Burlington, MA: Jones and Bartlett Learning (2019).
110. Carroll LN, Au AP, Detwiler LT, Fu T-C, Painter IS, Abernethy NF. Visualization and analytic tools for infectious disease epidemiology: a systematic review. J Biomed Info. (2014) 51:287–98. doi: 10.1016/j.jbi.2014.04.006
111. Ramadan AAB, Jackson-Thompson J, Boren SA. Geographic information systems: usability, perception, and preferences of public health professionals. Online J Public Health Informatics. (2017) 9:e191. doi: 10.5210/ojphi.v9i2.7437
112. Leligdowicz A, Fischer WA, Uyeki TM, Fletcher TE, Adhikari NK, Portella G, et al. Ebola virus disease and critical illness. Crit Care. (2016) 20:217. doi: 10.1186/s13054-016-1325-2
113. Dean CL, Hill CE. Caring for patients with Ebola virus disease: are U.S. biocontainment centers ready for the next outbreak? Semin Diagn Pathol. (2019) 36:160–3. doi: 10.1053/j.semdp.2019.04.007
Keywords: Demographic care unit, emergency management and preparedness, disasters, epidemics, geospatial care path™, point-of-care testing, small-world network, spatial care path™
Citation: Kost GJ (2019) Geospatial Science and Point-of-Care Testing: Creating Solutions for Population Access, Emergencies, Outbreaks, and Disasters. Front. Public Health 7:329. doi: 10.3389/fpubh.2019.00329
Received: 31 May 2019; Accepted: 24 October 2019;
Published: 26 November 2019.
Edited by:
Randy D. Kearns, University of New Orleans, United StatesReviewed by:
Dagan Schwartz, Ben-Gurion University of the Negev, IsraelKrzysztof Goniewicz, Polish Air Force Academy, Poland
Copyright © 2019 Kost. This is an open-access article distributed under the terms of the Creative Commons Attribution License (CC BY). The use, distribution or reproduction in other forums is permitted, provided the original author(s) and the copyright owner(s) are credited and that the original publication in this journal is cited, in accordance with accepted academic practice. No use, distribution or reproduction is permitted which does not comply with these terms.
*Correspondence: Gerald J. Kost, geraldkost@gmail.com