- 1Office of Population Health Genomics, Public and Aboriginal Health Division, Department of Health, Government of Western Australia, East Perth, WA, Australia
- 2Office of the Chief Health Officer, Public and Aboriginal Health Division, Department of Health, Government of Western Australia, East Perth, WA, Australia
- 3PathWest Laboratory Medicine, Sir Charles Gairdner Hospital, Nedlands, WA, Australia
- 4Faculty of Health and Medical Sciences, School of Biomedical Sciences, The University of Western Australia, Crawley, WA, Australia
- 5Genetic Services of Western Australia, King Edward Memorial Hospital, Department of Health, Government of Western Australia, Subiaco, WA, Australia
- 6Faculty of Health and Medical Sciences, School of Medicine, The University of Western Australia, Crawley, WA, Australia
- 7Western Australian Register of Developmental Anomalies, King Edward Memorial Hospital, Department of Health, Government of Western Australia, Subiaco, WA, Australia
- 8Centre for Child Health Research, The University of Western Australia and Telethon Kids Institute, Perth, WA, Australia
- 9Sir Walter Murdoch School of Policy and International Affairs, Murdoch University, Murdoch, WA, Australia
- 10School of Public Health, Curtin University of Technology, Bentley, WA, Australia
- 11Harry Perkins Institute of Medical Research, QEII Medical Centre, Nedlands, WA, Australia
- 12Faculty of Health and Medical Sciences, School of Population and Global Health, The University of Western Australia, Crawley, WA, Australia
The expanding use of genomic technologies encompasses all phases of life, from the embryo to the elderly, and even the posthumous phase. In this paper, we present the spectrum of genomic healthcare applications, and describe their scope and challenges at different stages of the life cycle. The integration of genomic technology into healthcare presents unique ethical issues that challenge traditional aspects of healthcare delivery. These challenges include the different definitions of utility as applied to genomic information; the particular characteristics of genetic data that influence how it might be protected, used and shared; and the difficulties applying existing models of informed consent, and how new consent models might be needed.
Introduction
Genomic testing is used to diagnose, monitor, treat, predict and prevent disease, as well as promote good health in individuals, across communities and whole populations. Technological advances have allowed for greater integration of genomics into healthcare delivery, from screening and molecular diagnostics, to the accurate detection of microbes, and the ability to prescribe and monitor the efficacy of more precise therapeutics (1). The potential for increased use of genomic testing in the health setting is available throughout the life cycle, including in preimplantation, prenatal, neonatal, pediatric, adult, preconception, and posthumous settings (2). The person (who is often, but not always, also the “patient”) should be firmly at the center of the genomics revolution in healthcare. We begin this review by discussing a variety of current and emerging situations in which genomic testing is being utilized in health settings, focusing on the ethical, legal and social issues that apply at each point in the cycle-of-life and at particular decision points relevant to specific healthcare situations.
Subsequently, we focus on three main areas in which genomic technology, which is considered to be both disruptive and transformative to healthcare delivery (3), creates unique ethical issues that can challenge traditional aspects of healthcare. We summarize some of the key challenges and considerations surrounding the increasing application of this technology, highlighting issues that may arise when genomic tests are used at different life cycle stages. Within this section, we firstly outline the juxtaposition between clinical utility of a genomic test with personal and other utility, particularly where genomic testing is utilized in non-clinical settings (4). Related to questions around the utility of testing are issues surrounding the limited ability to interpret incidental findings and variants of unknown significance, which presents ethical challenges for the responsibility to return such results to patients (5).
Secondly, we consider how the personal nature of genomic data is such that it can never be truly de-identified. This creates potential issues around data storage and sharing; however, integral to this is the necessity to share genomic information to allow for advancement of knowledge of the etiology of disease (6). Furthermore, appropriate reference genomes are critically important for capturing the genomic diversity of the population being tested (7) so as to deliver equitable healthcare.
Finally, we discuss how genomic testing can challenge traditional models of informed consent in an environment where online DNA tests are available, where genomic testing is being increasingly utilized for individuals who are unable to consent, and where re-interrogation of stored genomic data is possible (8). For the purposes of this review, the term “genomics” is used to encompass both genetics (individual genes) and genomics (all genes in a genome).
Current and Emerging Applications of Genomic Testing Across the Life Cycle
Genomic testing in the healthcare context can be applied in a multitude of ways throughout the human lifespan (Table 1). The application of massively parallel sequencing is expanding across different healthcare domains. This technique allows for the concurrent sequencing of numerous DNA fragments, enabling multiple loci to be investigated at one time and consequently, more efficient and cost-effective genomic analysis. Most of the current and emerging uses of genomic healthcare technology involve analyses for screening, diagnostic or prognostic purposes; testing to guide and evaluate treatment options; and identification and tracking of human disease-causing pathogens. Furthermore, genomic technology is increasingly available outside of healthcare settings through personal genomics tests that may be accessed directly by consumers, also known as direct-to-consumer or personal DNA tests (13, 22). Genomic sequencing technology has provided numerous benefits, particularly the significant improvement in the provision rate of molecular diagnoses (22). Continued developments with massively parallel sequencing include greater sensitivity of detecting previously difficult disease-causing deletions (23) and growing ability to detect copy number variants (24).
Genomic technology has increasing potential to contribute transformative new treatments in the form of genetic therapy. Recently this was demonstrated in a research setting with the treatment in utero of 3 fetuses affected with X-linked hypohydrotic ectodermal dysplasia. In this study, prenatal intervention occurred in the successful delivery of a recombinant version of the previously absent protein (25). Also on the horizon is the potential to use circulating tumor DNA in the blood or urine to assist in the clinical diagnosis of cancer, potentially alleviating the current reliance on invasive tissue sampling of solid tumors (26, 27).
The potential for genomic technology to continually improve the health and wellbeing of the population across the life cycle is anticipated. Further genomic and associated phenotypic data are integral for the advancement of knowledge and interpretation of genomic variants, and in turn the understanding of disease risk and disease etiology to inform better healthcare (6, 28, 29). The benefits of genomic technology in healthcare go beyond the immediate improvements in diagnosis and treatment, where a diagnosis is the portal to best care, to contributions to general understanding about disease and health and informing appropriately targeted public health initiatives.
The future ability to utilize “big data,” not only for the incorporation of genomic information but also other “-omic” information (e.g., metabolomics, proteomics), epigenetic, phenotypic, environmental and personal data, will depend on data collection and sharing. Big data will allow more precise healthcare, specifically healthcare that is tailored to individuals (i.e., precision medicine), and will facilitate precision public health interventions tailored to genetically identified population subgroups (30, 31). Additionally, large, shared datasets are likely to become even more important to further knowledge about disease mechanisms for common and complex polygenic diseases (29).
Challenges and Considerations for Genomic Applications Across the Life Cycle
As described above, application of genomic technology and new genomic knowledge is being applied across the life cycle in both clinical and wider healthcare settings. Importantly, although the genomic technology itself may remain fairly consistent across different applications, the scope of the test can differ in terms of who is tested, for whom healthcare decisions are made about, the types of tests available, the potential conditions that can be identified, the clinical information available and the potential consequences of false positives or false negatives (see Table 2). Moreover, the issues surrounding utility, incidental findings and informed consent may differ depending on the life cycle stage and purpose of the test (Table 2).
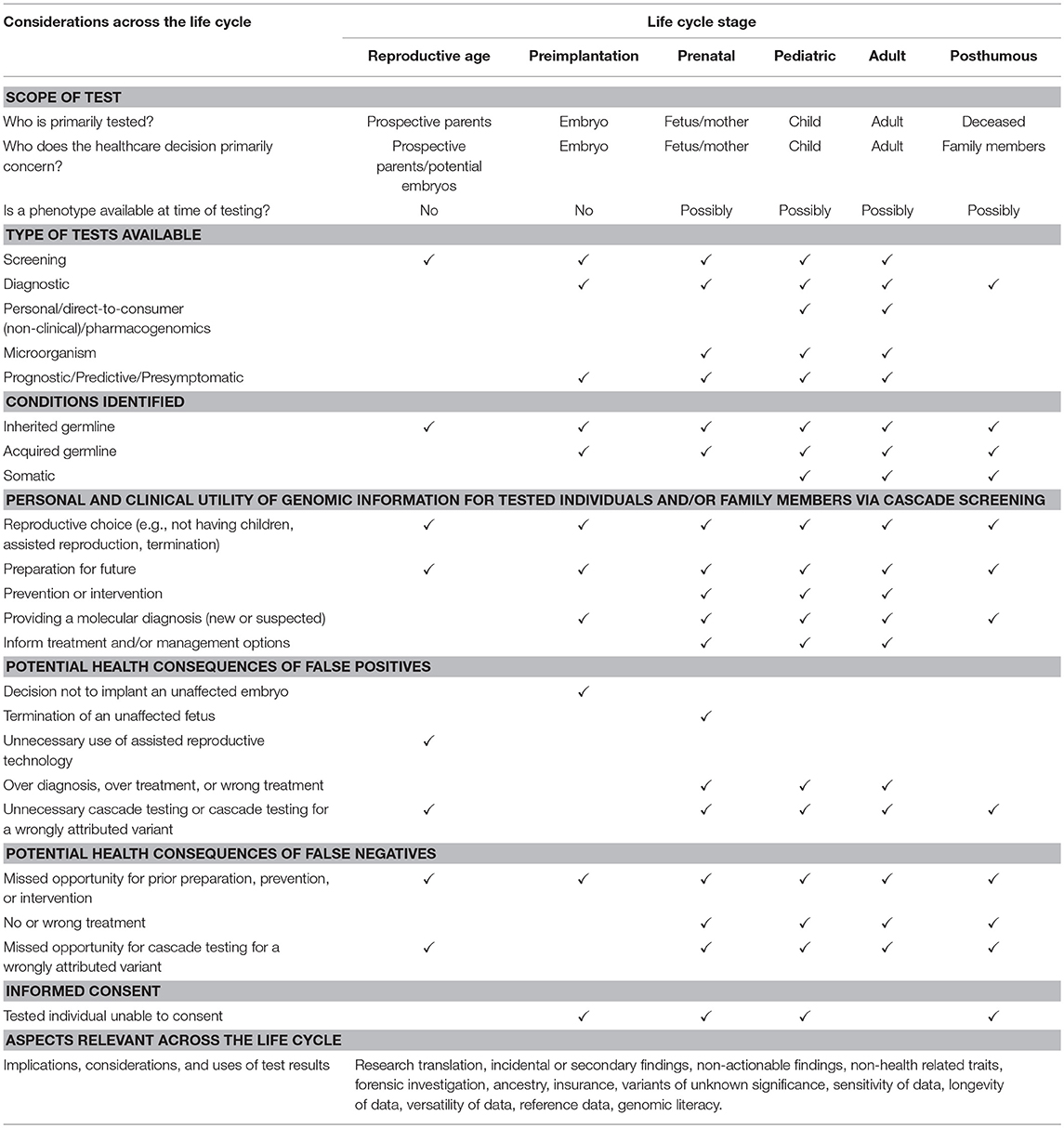
Table 2. The scope and challenges associated with genomic testing across different life cycle stages.
For example, the ethical issues surrounding diagnostic testing are undoubtedly less contentious than those involved with predictive testing of a living individual. In the first scenario a patient has usually already presented to a healthcare professional with symptomology, therefore the choice of the genomic diagnostic test and subsequent data interpretation is simplified and clinical utility usually aligns well with an individual's expectation of personal utility. This is true even if a treatment or intervention is not available, as a molecular diagnosis can end the diagnostic odyssey and potentially facilitate access to applicable health and social services (32). Comparatively, it can prove more complicated to predict the likelihood of a person potentially developing a disease at some point in the future. This is especially true for a person who has not yet been born, such as when predicting risk of a disease for a current (preimplantation or prenatal diagnosis) or future (preconception carrier screening) embryo or fetus. The absence of familial information such as in the case of adoption or gamete donation can add more complexity. The potential implications of the action/s taken based on genomic information in these situations are also greater, due to the possibility of an embryo not being selected for implantation, or the choice to terminate an affected fetus (Table 2).
Despite the potential benefits associated with increasing use of genomic testing, our knowledge of the relationship between genomic variants and health is still evolving and is limited by the complex interactions between the genome and other biological and environmental influences. Three key considerations relating to this potential include the utility of genomic testing for clinicians and the person, or patient; issues around genomic data such as the sensitivity and potential longevity of the data, data sharing and appropriateness of the reference genome; and issues around informed consent in the context of the complexity and expanding usage of genomic testing. The issues that are explored below, while not exhaustive, provide a broad overview of some of the challenges and considerations of genomic testing across the life cycle. Additional ethical, legal and social issues associated with genomic testing, including challenges associated with direct-to-consumer testing, are beyond the scope of this paper and are discussed elsewhere (33, 34).
Utility
Personal and Clinical Uses for Genomic Information
Clinical utility for genomic testing is generally limited to situations where genomic information directs and improves patient care. Therefore, genomic testing may not be recommended clinically if the results are unlikely to impact care. However, genomic information has multiple applications beyond the healthcare setting. For example, individuals may wish to have their genome sequenced to learn about their ancestry or non-health related traits; a genomic diagnosis can inform eligibility for special education and employment services; and genomic information is of interest to insurance companies and can be used in forensic settings. The manifold nature of genomic information could allow for more efficient healthcare due to the potential ability to reinterrogate data for multiple healthcare purposes. However, the value of such data to individuals and organizations beyond healthcare raises issues around consumer expectations when clinical utility and other kinds of utility diverge.
The utility of testing varies across the lifespan, potentially in relation to when the testing is performed and for which conditions the testing may relate (Table 2). In some settings genomic information is used to make healthcare decisions about an individual who may not have been able to provide consent (e.g., prenatal screening or diagnosis; patients with cognitive impairment). Furthermore, in particular settings the test not only informs treatment and management, but influences reproductive decisions which can be inherently more personal (as opposed to clinically led), compared to other healthcare decisions.
There are also limitations to the clinical utility of testing. For example in the preconception setting, the genomic test can only inform parents about risk of inherited conditions, and cannot provide information about de novo or acquired genetic conditions (Table 2). A particular consideration for the utility of testing across the life cycle is the presence (or absence) of a phenotype to aid clinical, as well as personal, decision-making. Genomic data has the potential to identify disease risk earlier than is possible through the use of clinical or physiological symptoms alone, meaning that interventions can be enacted more promptly. However, there is a conundrum presented whereby presence of a genetic variant may not necessarily mean that the disease will manifest (i.e., there is incomplete penetrance) (35), or where a variant is variably expressed among individuals and it is not possible to predict the severity based on genomic information alone.
In such situations, clinicians would ideally draw upon the presence of phenotype to aid in decision-making, and this is absent in the preconception and preimplantation stages, and often absent, or markedly limited, in the in utero setting (Table 2). Likewise, the use of genomic testing for screening includes the testing of asymptomatic individuals by definition, and this can occur at any life stage (Table 2). Assurance of the natural progression of genetic variants and the likelihood of resulting disease is required for individuals to make reproductive and healthcare choices. Similarly this information is needed for clinicians to make clinical decisions, yet currently this knowledge is limited. As a consequence, the clinical utility of genomic testing for many conditions currently remains relatively low.
In some circumstances, genomic information may have greater personal utility to patients compared to other medical test results. Therefore, there may be times where personal utility and clinical or healthcare utility are non-congruent. Available evidence indicates that many consumers would only seek genomic testing for actionable health information, particularly in situations where there is a perceived need for that information (4). However, certain subsets of the population may be more receptive to receiving non-actionable genomic results, in which case personal utility may override a lack of overt clinical utility. Common situations where these benefits arise include for parents of children with rare undiagnosed genetic diseases (36), testing for the risk of late-onset conditions at a point in the lifecycle where there is no clinical utility for such information (37), and testing for risk of conditions with no current treatments, such as Alzheimer's disease predisposition (38). Additional benefits cited for wanting to access testing in these situations include ending the diagnostic odyssey, a clearer understanding of the cause of a condition, greater understanding of future needs, the ability to connect with others in the same situation, and helping to gain access to social and healthcare services (32, 38, 39). Personal utility of a genomic test may also vary depending on a person's cultural background (40, 41).
In the era of personalized medicine and given that the degree of personal utility is likely to vary between persons, the utility of a genomic test is ultimately best estimated on an individual basis (42). Empowering patients and families to be involved in decisions will help to facilitate this (32). However, individuals and healthcare providers should recognize the inherent differences in value placed on genomic testing by different stakeholders. Variation in perceptions of the utility of genomic testing highlights the importance of accessible and appropriate education and genetic counseling for anyone considering a genomic test (43) (see Box 1). To enable this, sufficient genomic literacy is required across the health workforce to ensure health professionals are able to competently counsel individuals and families (46, 47). This could be enabled through increased education and training of health professionals (48).
Box 1. Genetic counseling.
Genetic counseling has traditionally focused on the education and support for patients and family members who have, or are at risk of, Mendelian disorders (44). With the increased integration of genomic testing in the healthcare system across the life cycle, the ability to provide adequate counseling to patients with the current workforce and services model will be tested. The current workforce will be challenged to provide optimal counseling with increased demand, and new models for delivering genetic counseling services may be required to fill this gap. New models could include bolstering the skills of the primary healthcare workforce (45), increasing the use of evidence-based online delivery, and utilization of technology such as telehealth to mitigate hurdles such as geography for the rural and remote population. Issues around genetic counseling for genomic tests are outlined in Burns et al. 2019 (46).
Research and Translational Uses of Genomic Testing
Genomic testing may be used in situations where the primary utility is to inform basic research or to identify eligibility for treatments or services. For genetic conditions without well-defined natural histories, for which there are few or no treatments available (e.g., rare diseases, including rare cancers), or where disease progression is rapid (e.g., advanced cancers), research and translational uses of genomic testing may be crucial. There could be a lack of knowledge or clinical consensus of the clinical utility of genomic testing in such contexts (49), but there might still be real or perceived utility for patients, clinicians, and researchers. Due to the rarity of genetic conditions and the increasing utility for genomic testing in treating cancer, management of these particular patient cohorts relies heavily on basic research.
Consideration of these additional uses for genomic testing in healthcare is important, particularly through the lens of providing equitable healthcare to those who need it. Only 14% of new scientific discoveries enter daily clinical practice, after an average lag of 17 years (50). This lag is especially problematic for (i) cancer patients, where disease prognosis can often be poor, but for whom tailored treatment is showing promise, and (ii) for patients with rare diseases for whom no, or limited pre-existing treatment is available. In this context, translating the benefits of genomic technology into the healthcare setting in a timely manner is challenging (51). Multiple agencies at both the national and international levels may be working on similar, smaller-scale research projects. This could lead to inconsistent findings due to sample size limitations, thereby becoming one of the rate-limiting steps in the integration of genomic advances into daily practice, and then into population-level programs. National and international collaboration between researchers has the potential to streamline this approach so that collective goals are expedited, and translation into practice is accelerated (51–53).
An additional consideration around the lag in translation of testing into the public healthcare setting is the potential for individuals to have their genome sequenced in a non-clinical setting (e.g., personal genomics) or a private healthcare setting, which may create expectations for increased access in public health systems. Genomic tests may be introduced first into private (user pays) settings due to the initially high cost and the lag in translation into public health systems, which must balance investment into multiple different aspects of healthcare (54), and as a result, often have a high evidence threshold for integrating new tests into practice. This can increase demand for, and produce inequity of access to, testing that may be beneficial for improving healthcare, but which health systems are not yet prepared to offer at a population-wide level (55).
Incidental or Secondary Findings
Genomic data can be interrogated in different ways, including broad approaches designed to capture as much information as possible and targeted approaches focused on a few variants of interest. Incidental or secondary findings are classified as gene variants that are not the primary focus of a specific genomic test and are not necessarily related to the condition/s being investigated [e.g., (56)]. These variants can be obtained when performing whole genome and exome sequencing, as well as with certain gene panels, and if scrutinized, may reveal the need to consider medical action. There have been efforts to identify a minimum list of variants that are considered medically actionable. The American College of Medical Genetics and Genomics has developed a widely used list of 59 genes to be reported in clinical settings as incidental or secondary findings (57). Incidental findings relating to germline variants and familial relationships are relevant across the life cycle (Table 2). A particular point of difference is the use of non-invasive prenatal testing, in which fetal DNA and maternal DNA can be identified in the same sample, which may reveal incidental findings relating to somatic changes present in the mother.
There is variation among consumers, pathologists, specialists, researchers and professional societies regarding the need to return incidental findings to patients, with the definition of incidental often being dependent on the medical specialty or setting (58, 59). Consumers may consider that raw data or medically significant incidental findings should be accessible to them even when their genome is sequenced for a non-clinical purpose (60, 61). This raises important legal issues surrounding the right to access personal information (43, 60), particularly when incidental findings relate to potentially actionable variants (62). However, there may be issues in the clinical utility of such information given the different requirements for sequencing quality in non-clinical settings (60, 63). Managing consumer/patient expectations is therefore of critical importance.
A dialogue involving all stakeholders, including the patient or consumer, may be required to agree on a set of criteria that could indicate when incidental findings should or should not be returned to individuals (64). A relevant question is whether researchers and genomics laboratories should be returning raw data or incidental findings given that many patients access genomic testing through research projects or directly from laboratories. Recent changes to the National Health and Medical Research Council's National Statement for Ethical Conduct in Human Research, with which researchers must comply, require a system to be in place to return findings that have health significance to participants (65). Other relevant questions include, will guidelines and regulations around incidental findings influence clinicians in ordering gene-specific analyses rather than clinical genomes or exomes? What is the likely effect of this on the utility of the test?
Variants of Uncertain Significance
By definition, variants of uncertain significance (VUS) have unknown clinical utility (66). It is understood that as knowledge of genomic variants and the relationship between variants and disease pathology advances, variants that have previously been identified as uncertain may be recognized as pathogenic, or alternatively benign. Internationally, guidelines surrounding the responsibility to report VUS are a work in progress. Variant interpretation, even in the setting of a clear phenotype, can be challenging at both the individual and health system levels. The medico-legal implications of variants at one point in time being identified as uncertain, and then with advances in knowledge being later identified as pathogenic, are highlighted in the law case of Williams vs. Athena (see Box 2). Significant questions include where do we draw the line on requirements to report based on knowledge of pathogenicity? How frequently should reference databases be updated and consulted to inform contemporary interpretation of results? Do patients wish to know about VUS if they are found? Is there an obligation to follow up VUS after the test has been taken, in accordance with changes in classification as knowledge develops? If so, who is responsible for doing this?
Box 2. Case study-Legal issues in variant interpretation.
A case study which highlights potential legal issues surrounding variant interpretation is the current USA lawsuit, Williams vs. Athena, where a 2 year old boy, Christian Jacob Millare, died of a fatal seizure from Dravet syndrome in January 2008. In 2007, Christian was tested for Dravet syndrome, and the report concluded that he had a “variant of unknown significance,” and as such Christian continued to be treated as if he had a mitochondrial disorder rather than Dravet syndrome. In 2015, the laboratory responsible for the test, Athena, updated its report to reclassify the mutation as pathogenic for Dravet syndrome, as Christian was found to have a pathogenic variant in the SCN1A gene. Interestingly at the time of the initial report, there were two publications (published in 2006 and 2007) each based on another single case reporting the same variant that Christian had as being pathogenic. Inherent to this case were the issues of whether a laboratory classifies as a health service provider, and relatedly the communication and responsibilities of laboratories and physicians. The case also emphasized the need for stricter governance around how variant databases report and interpret their data (6).
Genomic Data
Genomic data are becoming increasingly useful not only for understanding the causes of ill health, but also the genomic determinants of good health. However, there are ethical, legal, and social issues that need to be considered to ensure appropriate use of genomic data in healthcare. These include that genomic information is not only personal, but also familial (i.e., it can reveal familial relationships and personal information about relatives); that the longevity, particularly of germline genomic data, exceeds that of typical health information; that the broad utility of genomic data increases the demand for data sharing; and that equitable genomic testing relies on appropriate reference data.
Sensitivity of Genomic Data
The collection and generation of genomic information comes with some unique ethical issues. The security and privacy of genomic information challenges traditional paradigms of confidentiality for sensitive information, given that a person's genomic information can be compared to a fingerprint, such that it can never be truly de-identified. Genomic information may therefore require additional regulation, such as the addition of noise to the data to ensure protection of privacy (67, 68).
Genomic data can impact biologically related family members even if they have not accessed genomic testing themselves. With the possibility for on-sharing of genomic information for future uses other than the intended purpose of the initial test, concerns over the privacy of genomic data and issues of informed consent for the disclosure and use of genomic information become paramount. Recently this was illustrated in California, where police used genomic information from an open source database from genealogy company GED match to facilitate the arrest of a serial rapist and murderer known as “The Golden State Killer” (69). The suspect, who is accused of committing crimes more than 3 decades ago, did not himself have genomic information in the database, but rather one of his relatives had participated.
Longevity of Genomic Data
Germline genomic data is somewhat unique in its unchanging nature, meaning that the same data could be reinterrogated over the life cycle for different health and non-health related purposes. The potential of genomic information is therefore longer lasting in contrast to many other types of health test results, which may provide more of a health snapshot. This makes genomic information more similar to stored biological samples collected in biobanks. Future innovations will allow for improved interrogation of historical genomic data (3, 70). In the inevitable advent of improved interrogation ability, is it ethical to analyse existing, historical datasets with improved technologies, and contrastingly, is it ethical to omit reinterrogation of existing datasets with new technologies (71)? Future tools for analysis and enhanced genomic knowledge may alter an individual's risk profile with reinterrogation of existing data (72). With increasing applications in precision medicine and precision public health, disease risk could become dynamically updated depending on current knowledge and data. The potential for rapid change in technology should be considered when determining how long to store genomic data. Careful management of consumer or patient expectations should also be considered.
The integration of genomic testing that is currently occurring separately across the life cycle may facilitate greater efficiencies in healthcare, but would require coordination and communication among previously isolated healthcare settings. With the increasing application of genomic testing across the life cycle, the potential to use one test for multiple purposes is increasing (73). For example, if genomic testing is introduced at a population level in more than one setting, the possible number of individual tests taken over the lifespan for a single individual is likely to increase. Assuming there is agreed utility for each type of testing at a population level, would broad sequencing such as whole genome or whole exome sequencing and long-term storage of genomic test results ultimately become more cost effective than repeat testing?
What if every baby's exome or genome was sequenced and reanalyzed as needed for different purposes throughout their life, would the cost savings from a reduced need to re-test outweigh the cost of storing the relevant data? A single genomic test could provide the answer to a patient's symptomatology that would have otherwise required multiple tests (74), and may also provide information relevant for different healthcare decisions in that person's life (Table 2). However, it may also lead to a short-term increase in healthcare demand from different healthcare professionals. For example, an actionable secondary finding may mobilize cascade screening in family members that would otherwise not have occurred (53), or may indicate consideration of alternative reproductive choices for individuals in the family. In addition, such long term storage and reinterrogation of genomic data would require processes for obtaining consent for each subsequent analysis over a long time period. The implications of broad sequencing methods and use of genomic data should be carefully examined prior to any large-scale implementation (75).
The potential for integration of genomic testing across the life cycle will not be without challenges. In such a context it is important to determine who is responsible for reporting results; which results should be reported and when; and whether to store and reanalyse results at a later time. The complexities of healthcare systems and governance arrangements across responsible organizations create the need for a harmonized approach at the local, national and international levels.
Data Sharing and Regulation
The benefits to be obtained from the use of genomic technology in healthcare are reliant on global cooperation and data sharing (2, 76). This is particularly the case for rare genetic conditions, where sharing of patient data can be critical to achieving a diagnosis or conducting research to improve healthcare. Adequate governance and regulation is required to ensure that genomic data are used responsibly by those who are permitted access. In 1997, the United Nations Educational, Scientific and Cultural Organization developed a Universal Declaration on the Human Genome and Human Rights, and, in 2003, adopted an International Declaration on Human Genetic Data to guide the use of such data at an international level (77).
Internationally, the regulatory space continues to evolve. The United States of America (USA) has developed regulations and legislation governing the protection and use of genetic information. The Genetic Information Nondiscrimination Act of 2008 protects the genetic privacy of individuals by preventing insurers or employers from requesting genetic information. However, a bill for the Preserving Employee Wellness Programs Act has been introduced to the USA Senate, and has the potential to jeopardize present legislation protecting individuals from their employers requesting disclosure of genetic information and imposing penalties for non-disclosure (35). In Canada, the Genetic Non-Discrimination Act prohibits a person from requiring an individual to disclose their genetic information as a condition of providing goods or services to that individual, with exceptions for healthcare practitioners and researchers.
The requirements for responsible use of genomic information are similar to those for other health-related and personal information. The European Union (EU) has developed a General Data Protection Regulation that applies to businesses that process or control data from within the EU. Discrimination against individuals on the basis of their genetic information is also banned in Europe in accordance with the Council of Europe's Oviedo Convention. These regulations are important to ensure that data can be shared responsibly and ethically, which will encourage individuals to continue to participate in health research.
Reference Data
The importance of reference genomes in utilizing genomic testing is reliant on large-scale data sharing and collaboration. Reference genomes are used for comparison purposes, as a representative example of a typical human's genome sequence. These genomes are developed using a mosaic of genetic information sourced from different individuals and combined to form a template sequence. The use of reference genomes in utilizing genomic technology raises concerns around offering genomic testing that is less effective in some ethnicities compared to others, since genetic disease risk varies among ethnicities (78, 79). However, the use of an appropriate reference genome is made more difficult by the increasing multiculturalism within countries.
For equitable understanding of genomic variants, reference databases must be capable of reflecting the ethnic diversity of the relevant population/s, such as minority groups and/or Indigenous populations (80). It has been highlighted that most genomic reference databases do not adequately reflect the diversity of human populations (7). As an example, the cultural makeup of Australia's population is highly diverse, including Aboriginal and Torres Strait Islander peoples, and having a higher proportion of people born overseas compared to the USA, New Zealand, and Canada (81). However, there is a paucity of genetic data available for Aboriginal and Torres Strait Islander peoples, such that interpretation of genetic variants currently needs to be addressed with caution (82). For multicultural societies, it is vital to ensure that minority ethnic populations are not disadvantaged in accessing the benefits of genomic technology.
Informed Consent
Informed consent for genomic testing in healthcare is complex when we try to consider all of the issues outlined above, including the variety of different testing methods and potential to test for multiple conditions at once; the testing of non-consenting individuals; potential for reinterrogation or sharing of data; the lack of diagnostic certainty in particular test settings; the complexities surrounding interpretation of tests, VUS and incidental findings; implications for insurance and other services; and the potential impact of testing on family members. Some of these issues are more pertinent in particular test settings across the life cycle (Table 2).
For example, the current guidelines on prenatal testing from the Royal Australian and New Zealand College of Obstetricians and Gynaeocologists state that information provided to women should include descriptions of conditions to be tested, as well as information about both the variance in phenotype and ability to predict the conditions tested (83). In the context of increasing lists of conditions to be screened for through the use of expanded genetic testing panels or whole exome/genome sequencing, how can healthcare providers ensure that the decision to undergo such testing is adequately informed?
The complexity of ethical, legal, and social issues surrounding consent for genomic testing indicate that substantial effort is required to ensure adequate understanding of the test by consumers. Depending on how many of these issues apply, professional genetic counseling may be critical for obtaining truly informed consent for genomic tests. Informed consent should be obtained based on the individual's circumstances to the extent that this is possible. Patient decision aids may be helpful for achieving this approach, particularly for applications where for some people the personal benefits outweigh the lack of clinical utility (84, 85). Such tools have been developed to help parents decide whether to undergo screening for Down syndrome (86), and to support reproductive decision-making for individuals with a genetic predisposition to heritable cancer (87). Decision aids may need to be tailored to the level of health literacy of users (88).
Consideration must also be given to a model where informed consent to personal genomic data analysis and storage can allow for the possibility that data may be reviewed, and thus for the possibility that an individual or their family might be contacted in relation to the updated outcomes in the future if this occurs (89). In the context of including late-onset conditions in tests performed on fetuses or children, is there a moral obligation to ensure this information can be communicated to individuals once they reach the age of informed consent? Dynamic consent models in this instance are one such example of a flexible, digitally-enabled consent model that may cater more broadly to the needs of healthcare consumers (8). Evidence so far shows that healthcare consumers want ownership and control over their health data (90, 91). Several companies are developing applications to allow greater access to, and control of, genomic information by consumers (e.g., Helix online genomics marketplace; Seqster platform). Much of this development is currently occurring in the personal genomics space, but similar efforts are being made in public health systems in association with moves to electronic health records. This patient-centered approach will be increasingly important with the rise in personalized medicine and precision public health, but will need to be implemented in a considered and ethical manner.
Conclusions
Genomic technologies challenge aspects of traditional healthcare delivery, with new ethical issues arising from these unchartered waters. The increasing utilization of genomic testing across different healthcare settings over the life cycle necessitates increased clarity of purpose and raises important ethical, legal, and social issues. Healthcare providers will be required to adopt an approach to genomic technology that will allow for the advancement of genomic knowledge and the responsible application of technology to benefit the population across the life cycle. In the context of the complexity and versatility of genomic information and its inherently personal and familial nature, adequate governance and informed consent are critical considerations for implementing genomic testing for healthcare.
Author Contributions
TW, HD, and GAB conceived the paper concept. BB, GAB, EC, and KN drafted the manuscript. All authors provided critical input and approved submission of the final manuscript.
Funding
In-kind support was provided through the Western Australian Department of Health.
Conflict of Interest Statement
The authors declare that the research was conducted in the absence of any commercial or financial relationships that could be construed as a potential conflict of interest.
References
1. Williamson R, Anderson W, Duckett S, Frazer I, Hillyard C, Kowal E, et al. The Future of Precision Medicine in Australia. Melbourne, VIC: Australian Council of Learned Academies (2018).
2. Rehm HL. Evolving health care through personal genomics. Nat Rev Genet. (2017) 18:259. doi: 10.1038/nrg.2016.162
3. Gaff CL, Winship IM, Forrest SM, Hansen DP, Clark J, Waring PM, et al. Preparing for genomic medicine: a real world demonstration of health system change. NPJ Genomic Med. (2017) 2:16. doi: 10.1038/s41525-017-0017-4
4. Metcalfe SA, Hickerton C, Savard J, Terrill B, Turbitt E, Gaff C, et al. Australians' views on personal genomic testing: focus group findings from the Genioz study. Eur J Human Genet. (2018) 26:1101–12. doi: 10.1038/s41431-018-0151-1
5. Turbitt E, Wiest MM, Halliday JL, Amor DJ, Metcalfe SA. Availability of treatment drives decisions of genetic health professionals about disclosure of incidental findings. Eur J Human Genet. (2014) 22:1225. doi: 10.1038/ejhg.2014.11
6. Thorogood A, Cook-Deegan R, Knoppers BM. Public variant databases: liability? Genet Med. (2017) 19:838–41. doi: 10.1038/gim.2016.189
7. Popejoy AB, Fullerton SM. Genomics is failing on diversity. Nature. (2016) 538:161. doi: 10.1038/538161a
8. Kaye J, Whitley EA, Lund D, Morrison M, Teare H, Melham K. Dynamic consent: a patient interface for twenty-first century research networks. Eur J Human Genet. (2015) 23:141. doi: 10.1038/ejhg.2014.71
9. Cookson W, Cox MJ, Moffatt MF. New opportunities for managing acute and chronic lung infections. Nat Rev Microbiol. (2017) 16:111–20. doi: 10.1038/nrmicro.2017.122
10. Routy B, Le Chatelier E, Derosa L, Duong CPM, Alou MT, Daillere R, et al. Gut microbiome influences efficacy of PD-1-based immunotherapy against epithelial tumors. Science. (2017) 359:91–7. doi: 10.1126/science.aan3706
11. Grice EA, Segre JA. The human microbiome: our second genome. Ann Rev Genomics Human Genet. (2012) 13:151–70. doi: 10.1146/annurev-genom-090711-163814
12. Jansen ME, Metternick-Jones SC, Lister KJ. International differences in the evaluation of conditions for newborn bloodspot screening: a review of scientific literature and policy documents. Eur J Human Genet. (2017) 25:10. doi: 10.1038/ejhg.2016.126
13. Allyse MA, Robinson DH, Ferber MJ, Sharp RR. Direct-to-consumer testing 2.0: emerging models of direct-to-consumer genetic testing. Mayo Clinic Proc. (2018) 93:113–20. doi: 10.1016/j.mayocp.2017.11.001
14. Chen M, Wei S, Hu J, Quan S. Can comprehensive chromosome screening technology improve IVF/ICSI outcomes? A meta-analysis. PloS One. (2015) 10:e0140779. doi: 10.1371/journal.pone.0140779
15. Taylor-Phillips S, Freeman K, Geppert J, Agbebiyi A, Uthman OA, Madan J, et al. Accuracy of non-invasive prenatal testing using cell-free DNA for detection of Down, Edwards and Patau syndromes: a systematic review and meta-analysis. BMJ Open. (2016) 6:e010002. doi: 10.1136/bmjopen-2015-010002
16. Dickinson JE. Noninvasive prenatal testing: known knowns and known unknowns. Aust N Z J Obst Gynaecol. (2014) 54:397–9. doi: 10.1111/ajo.12269
18. Lew RM, Burnett L, Proos AL, Delatycki MB. Tay-Sachs disease: current perspectives from Australia. Appl Clin Genet. (2015) 8:19–25. doi: 10.2147/TACG.S49628
19. Massie J, Delatycki MB. Cystic fibrosis carrier screening. Paediatr Respir Rev. (2013) 14:270–5. doi: 10.1016/j.prrv.2012.12.002
20. Edwards JG, Feldman G, Goldberg J, Gregg AR, Norton ME, Rose NC, et al. Expanded carrier screening in reproductive medicine—points to consider: a joint statement of the American College of Medical Genetics and Genomics, American College of Obstetricians and Gynecologists, National Society of Genetic Counselors, Perinatal Quality Foundation, and Society for Maternal-Fetal Medicine. Obst Gynecol. (2015) 125:653–62. doi: 10.1097/AOG.0000000000000666
21. Dewar LJ, Alcaide M, Fornika D, D'Amato L, Shafaatalab S, Stevens CM, et al. Investigating the genetic causes of sudden unexpected death in children through targeted next-generation sequencing analysis. Circ Cardiovasc Genet. (2017) 10:e001738. doi: 10.1161/CIRCGENETICS.116.001738
22. Lionel AC, Costain G, Monfared N, Walker S, Reuter MS, Hosseini SM, et al. Improved diagnostic yield compared with targeted gene sequencing panels suggests a role for whole-genome sequencing as a first-tier genetic test. Genet Med. (2017) 20:435–43. doi: 10.1038/gim.2017.119
23. Watson CM, Camm N, Crinnion LA, Clokie S, Robinson RL, Adlard J, et al. Increased sensitivity of diagnostic mutation detection by re-analysis incorporating local reassembly of sequence reads. Mol Diagn Ther. (2017) 21:685–92. doi: 10.1007/s40291-017-0304-x.
24. Gambin T, Akdemir ZC, Yuan B, Gu S, Chiang T, Carvalho CMB, et al. Homozygous and hemizygous CNV detection from exome sequencing data in a Mendelian disease cohort. Nucleic Acids Res. (2017) 45:1633–48. doi: 10.1093/nar/gkw1237
25. Schneider H, Faschingbauer F, Schuepbach-Mallepell S, Körber I, Wohlfart S, Dick A, et al. Prenatal correction of X-linked hypohidrotic ectodermal dysplasia. N Engl J Med. (2018) 378:1604–10. doi: 10.1056/NEJMoa1714322
26. Wan JC, Massie C, Garcia-Corbacho J, Mouliere F, Brenton JD, Caldas C, et al. Liquid biopsies come of age: towards implementation of circulating tumour DNA. Nat Rev Cancer. (2017) 17:223. doi: 10.1038/nrc.2017.7
27. Lu T, Li J. Clinical applications of urinary cell-free DNA in cancer: current insights and promising future. Am J Cancer Res. (2017) 7:2318–32.
28. Manolio TA, Chisholm RL, Ozenberger B, Roden DM, Williams MS, Wilson R, et al. Implementing genomic medicine in the clinic: the future is here. Genet Med. (2013) 15:258. doi: 10.1038/gim.2012.157
29. Green ED, Guyer MS. Charting a course for genomic medicine from base pairs to bedside. Nature. (2011) 470:204. doi: 10.1038/nature09764
30. Khoury MJ, Ioannidis JPA. Big data meets public health: human well-being could benefit from large-scale data if large-scale noise is minimized. Science. (2014) 346:1054–5. doi: 10.1126/science.aaa2709
31. PHG Foundation. Public Health in an Era of Genome-Based and Personalised Medicine. Cambridge: PHG Foundation (2010).
32. Brosco JP. Whose odyssey is it? Family-centered care in the genomic era. Hastings Cent Rep. (2018) 48:S20–2. doi: 10.1002/hast.879
33. Molster CM, Bowman FL, Bilkey GA, Cho AS, Burns BL, Nowak KJ, et al. The evolution of public health genomics: exploring its past, present, and future. Front Public Health. (2018) 6:247.doi: 10.3389/fpubh.2018.00247
34. Rafiq M, Ianuale C, Ricciardi W, Boccia S. Direct-to-consumer genetic testing: a systematic review of european guidelines, recommendations, and position statements. Genet Testing Mol Biomark. (2015) 19:535–47. doi: 10.1089/gtmb.2015.0051
35. Bilkey GA, Baynam G, Molster C. Changes to the employers' use of genetic information and non-discrimination for health insurance in the USA: implications for Australians. Front Public Health. (2018) 6:183. doi: 10.3389/fpubh.2018.00183
36. Sapp JC, Dong D, Stark C, Ivey LE, Hooker G, Biesecker LG, et al. Parental attitudes, values, and beliefs toward the return of results from exome sequencing in children. Clini Genet. (2014) 85:120–6. doi: 10.1111/cge.12254
37. Goldenberg AJ, Sharp RR. The ethical hazards and programmatic challenges of genomic newborn screening. JAMA. (2012) 307:461–2. doi: 10.1001/jama.2012.68
38. Lim Q, McGill BC, Quinn VF, Tucker KM, Mizrahi D, Farkas Patenaude A, et al. Parents' attitudes toward genetic testing of children for health conditions: a systematic review. Clini Genet. (2017) 92:569–78. doi: 10.1111/cge.12989
39. Gogarty B. Parents as Partners: A Report and Guidelines on the Investigation of Children with Developmental Delay; by Parents, for Professionals. Cambridge: Cambridge Genetics Knowledge Park (2006).
40. Haidar H, Vanstone M, Laberge A-M, Bibeau G, Ghulmiyyah L, Ravitsky V. Cross-cultural perspectives on decision making regarding noninvasive prenatal testing: a comparative study of Lebanon and Quebec. AJOB Empirical Bioethics. (2018) 9:99–111. doi: 10.1080/23294515.2018.1469551
41. Amendola LM, Berg JS, Horowitz CR, Angelo F, Bensen JT, Biesecker BB, et al. The clinical sequencing evidence-generating research consortium: integrating genomic sequencing in diverse and medically underserved populations. Am J Human Genet. (2018) 103:319–27. doi: 10.1016/j.ajhg.2018.08.007
42. Foster MW, Mulvihill JJ, Sharp RR. Evaluating the utility of personal genomic information. Genet Med. (2009) 11:570. doi: 10.1097/GIM.0b013e3181a2743e
43. Middleton A, Mendes Á, Benjamin CM, Howard HC. Direct-to-consumer genetic testing: where and how does genetic counseling fit? Personal Med. (2017) 14:249–57. doi: 10.2217/pme-2017-0001
44. Kurti L, Tomiczek C, Brophy E, Fase D. The Changing Landscape of the Genetic Counselling Workforce: Final Report. NSW: Urbis (2017).
45. Robins R, Metcalfe S. Integrating genetics as practices of primary care. Soc Sci Med. (2004) 59:223–33. doi: 10.1016/j.socscimed.2003.10.025
46. Burns BL, Bilkey GA, Coles EP, Bowman FL, Beilby JP, Pachter NS, et al. Healthcare system priorities for successful integration of genomics: an Australian focus. Front Public Health. (2019) doi: 10.3389/fpubh.2019.00041
47. Marzuillo C, De Vito C, Boccia S, D'Addario M, D'Andrea E, Santini P, et al. Knowledge, attitudes and behavior of physicians regarding predictive genetic tests for breast and colorectal cancer. Prevent Med. (2013) 57:477–82. doi: 10.1016/j.ypmed.2013.06.022
48. Ricciardi W, Boccia S. New challenges of public health: bringing the future of personalised healthcare into focus. Eur J Public Health. (2017) 27:36–9. doi: 10.1093/eurpub/ckx164
49. Khoury M, Berg A, Coates R, Evans J, Teutsch S, Bradley L. The evidence dilemma in genomic medicine. Health Affairs. (2008) 27:1600–11. doi: 10.1377/hlthaff.27.6.1600
50. Morris ZS, Wooding S, Grant J. The answer is 17 years, what is the question: understanding time lags in translational research. J Royal Soc Med. (2011) 104:510–20. doi: 10.1258/jrsm.2011.110180
51. Khoury MJ, Gwinn M, Yoon PW, Dowling N, Moore CA, Bradley L. The continuum of translation research in genomic medicine: how can we accelerate the appropriate integration of human genome discoveries into health care and disease prevention? Genet Med. (2007) 9:665. doi: 10.1097/GIM.0b013e31815699d0
52. Manolio TA, Abramowicz M, Al-Mulla F, Anderson W, Balling R, Berger AC, et al. Global implementation of genomic medicine: we are not alone. Sci Transl Med. (2015) 7:290ps13-ps13. doi: 10.1126/scitranslmed.aab0194
53. Green RC, Goddard KA, Jarvik GP, Amendola LM, Appelbaum PS, Berg JS, et al. Clinical sequencing exploratory research consortium: accelerating evidence-based practice of genomic medicine. Am J Human Genet. (2016) 98:1051–66. doi: 10.1016/j.ajhg.2016.04.011
54. Tiller J, Lacaze P. Regulation of internet-based genetic testing: challenges for Australia and other jurisdictions. Front Public Health. (2018) 6:24. doi: 10.3389/fpubh.2018.00024
55. Khoury MJ, Feero WG, Reyes M, Citrin T, Freedman A, Leonard D, et al. The genomic applications in practice and prevention network. Genet Med. (2009) 11:488. doi: 10.1097/GIM.0b013e3181a551cc
56. Middleton A, Morley KI, Bragin E, Firth HV, Hurles ME, Wright CF, et al. Attitudes of nearly 7000 health professionals, genomic researchers and publics toward the return of incidental results from sequencing research. Eur J Human Genet. EJHG. (2016) 24:21–9. doi: 10.1038/ejhg.2015.58
57. Kalia SS, Adelman K, Bale SJ, Chung WK, Eng C, Evans JP, et al. Recommendations for reporting of secondary findings in clinical exome and genome sequencing, 2016 update (ACMG SF v2.0): a policy statement of the American College of Medical Genetics and Genomics. Genet Med. (2017) 19:249–55. doi: 10.1038/gim.2016.190
58. Turbitt E, Halliday JL, Metcalfe SA. Key informants' perspectives of implementing chromosomal microarrays into clinical practice in Australia. Twin Res Human Genet. (2013) 16:833–9. doi: 10.1017/thg.2013.43
59. Christensen KD, Vassy JL, Jamal L, Lehmann LS, Slashinski MJ, Perry DL, et al. Are physicians prepared for whole genome sequencing? A qualitative analysis. Clini Genet. (2016) 89:228–34. doi: 10.1111/cge.12626
60. Kaye J, Kanellopoulou N, Hawkins N, Gowans H, Curren L, Melham K. Can I access my personal genome? The current legal position in the UK. Med Law Rev. (2013) 22:64–86. doi: 10.1093/medlaw/fwt027
61. Middleton A, Wright CF, Morley KI, Bragin E, Firth HV, Hurles ME, et al. Potential research participants support the return of raw sequence data. J Med Genet. (2015) 52:571–4. doi: 10.1136/jmedgenet-2015-103119
62. Pike ER, Rothenberg KH, Berkman BE. Finding fault? Exploring legal duties to return incidental findings in genomic research. Georgetown Law J. (2014) 102:795–843.
63. Wolf SM, Lawrenz FP, Nelson CA, Kahn JP, Cho MK, Clayton EW, et al. Managing incidental findings in human subjects research: analysis and recommendations. J Law Med Ethics. (2008) 36:219–48. doi: 10.1111/j.1748-720X.2008.00266.xt
64. Borry P, Bentzen HB, Budin-Ljosne I, Cornel MC, Howard HC, Feeney O, et al. The challenges of the expanded availability of genomic information: an agenda-setting paper. J Comm Genet. (2017) 9:103–16. doi: 10.1007/s12687-017-0331-7
65. Eckstein L, Chalmers D, Critchley C, Jeanneret R, McWhirter R, Nielsen J, et al. Australia: regulating genomic data sharing to promote public trust. Human Genet. (2018) 137:583–91. doi: 10.1007/s00439-018-1914-z
66. Cheon JY, Mozersky J, Cook-Deegan R. Variants of uncertain significance in BRCA: a harbinger of ethical and policy issues to come? Genome Med. (2014) 6:121. doi: 10.1186/s13073-014-0121-3
67. Lin Z, Owen AB, Altman RB. Genomic research and human subject privacy. Science. (2004) 305:183. doi: 10.1126/science.1095019
69. Stanton S, Lillis R. Relative's DNA from genealogy websites cracked East Area Rapist case, DA's office says. The Sacramento Bee (2018). Available online at: https://www.sacbee.com/latest-news/article209913514.html (Accessed September 7, 2018).
70. Bowdin S, Gilbert A, Bedoukian E, Carew C, Adam MP, Belmont J, et al. Recommendations for the integration of genomics into clinical practice. Genet Med. (2016) 18:1075. doi: 10.1038/gim.2016.17
71. Hall AE, Chowdhury S, Hallowell N, Pashayan N, Dent T, Pharoah P, et al. Implementing risk-stratified screening for common cancers: a review of potential ethical, legal and social issues. J Public Health. (2014) 36:285–91. doi: 10.1093/pubmed/fdt078
72. Wenger AM, Guturu H, Bernstein JA, Bejerano G. Systematic reanalysis of clinical exome data yields additional diagnoses: implications for providers. Genet Med. (2017) 19:209. doi: 10.1038/gim.2016.88
73. Khoury MJ, Feero WG, Chambers DA, Brody LE, Aziz N, Green RC, et al. A collaborative translational research framework for evaluating and implementing the appropriate use of human genome sequencing to improve health. PLoS Med. (2018) 15:e1002631. doi: 10.1371/journal.pmed.1002631
74. Science and Technology Committee. Genomics and Genome Editing in the NHS. House of Commons (2018).
75. Johnston J, Lantos JD, Goldenberg A, Chen F, Parens E, Koenig BA. Sequencing newborns: a call for nuanced use of genomic technologies. Hastings Cent Rep. (2018) 48:S2–6. doi: 10.1002/hast.874
76. Middleton A. Society and personal genome data. Human Mol Genet. (2018) 27:R8–13. doi: 10.1093/hmg/ddy084
77. The UNESCO Universal Declaration on Bioethics and Human Rights: Background, Principles and Application. UNESCO Publishing (2009).
78. Landry LG, Rehm HL. Association of racial/ethnic categories with the ability of genetic tests to detect a cause of cardiomyopathy. JAMA Cardiol. (2018) 3:341–5. doi: 10.1001/jamacardio.2017.5333
79. Mak ACY, White MJ, Eckalbar WL, Szpiech ZA, Oh SS, Pino-Yanes M, et al. Whole genome sequencing of pharmacogenetic drug response in racially diverse children with asthma. Am J Respir Crit Care Med. (2018) 197:1552–64. doi: 10.1164/rccm.201712-2529OC
80. Robertson S, Hindmarsh J, Berry S, Cameron V, Cox M, Dewes O, et al. Genomic medicine must reduce, not compound, health inequities: the case for hauora-enhancing genomic resources for New Zealand. N Zeal Med J. (2018) 131:81–9.
82. Nowak KJ, Bauskis A, Dawkins HJ, Baynam G. Incidental inequity. Eur J Human Genet. 26:616–7. doi: 10.1038/s41431-018-0101-y
83. The Royal Australian and New Zealand College of Obstetricians and Gynaecologists and Human Genetics Society of Australia. Prenatal Screening and Diagnosis of Chromosomal and Genetic Conditions in the Fetus in Pregnancy. Melbourne, VIC: Royal Australian and New Zealand College of Obstetricians and Gynaecologists and Human Genetics Society of Australia (2015).
84. Barry MJ, Edgman-Levitan S. Shared decision making — The pinnacle of patient-centered care. N Engl J Med. (2012) 366:780–1. doi: 10.1056/NEJMp1109283
85. O'Connor AM, Wennberg JE, Legare F, Llewellyn-Thomas HA, Moulton BW, Sepucha KR, et al. Toward the ‘tipping point': decision aids and informed patient choice. Health Affairs. (2007) 26:716–25. doi: 10.1377/hlthaff.26.3.716
86. Agbadjé TT, Menear M, Dugas M, Gagnon M-P, Rahimi SA, Robitaille H, et al. Pregnant women's views on how to promote the use of a decision aid for Down syndrome prenatal screening: a theory-informed qualitative study. BMC Health Services Res. (2018) 18:434. doi: 10.1186/s12913-018-3244-1
87. Reumkens K, Tummers MH, Gietel-Habets JJ, van Kuijk SM, Aalfs CM, van Asperen CJ, et al. The development of an online decision aid to support persons having a genetic predisposition to cancer and their partners during reproductive decision-making: a usability and pilot study. Familial Cancer. (2019) 18:137–46. doi: 10.1007/s10689-018-0092-4
88. McCaffery KJ, Holmes-Rovner M, Smith SK, Rovner D, Nutbeam D, Clayman ML, et al. Addressing health literacy in patient decision aids. BMC Med Informat Dec Making. (2013) 13:S10. doi: 10.1186/1472-6947-13-S2-S10
89. Cameron L, Burton H. Genetic Screening Programmes: An International Review of Assessment Criteria. Cambridge: PHG Foundation (2014).
Keywords: genomics, public health, healthcare, genomic testing, molecular diagnostics, genetic disease, clinical utility, genomic data
Citation: Bilkey GA, Burns BL, Coles EP, Bowman FL, Beilby JP, Pachter NS, Baynam G, Dawkins HJS, Nowak KJ and Weeramanthri TS (2019) Genomic Testing for Human Health and Disease Across the Life Cycle: Applications and Ethical, Legal, and Social Challenges. Front. Public Health 7:40. doi: 10.3389/fpubh.2019.00040
Received: 17 September 2018; Accepted: 14 February 2019;
Published: 11 March 2019.
Edited by:
Daniel F. Sarpong, Xavier University of Louisiana, United StatesCopyright © 2019 Bilkey, Burns, Coles, Bowman, Beilby, Pachter, Baynam, Dawkins, Nowak and Weeramanthri. This is an open-access article distributed under the terms of the Creative Commons Attribution License (CC BY). The use, distribution or reproduction in other forums is permitted, provided the original author(s) and the copyright owner(s) are credited and that the original publication in this journal is cited, in accordance with accepted academic practice. No use, distribution or reproduction is permitted which does not comply with these terms.
*Correspondence: Gemma A. Bilkey, Z2VtbWEuYmlsa2V5QGhlYWx0aC53YS5nb3YuYXU=
†These authors share first authorship
‡These authors share senior authorship