- 1HPBioTECH, Gradignan, France
- 2Laboratoire de Bacteriologie, Centre Hospitalier Universitaire de Bordeaux, Bordeaux, France
- 3UMR1313 GABI, INRA, AgroParisTech, Université Paris-Saclay, Jouy-en-Josas, France
- 4Department Nutrition-Health & Lipid biochemistry of ITERG, Bordeaux, France
- 5Neonatology Nutrition, Lactarium Bordeaux-Marmande, CIC Pédiatrique 1401 Children's Hospital, Bordeaux, France
Background: The main process used to pasteurize human milk is the low-temperature, long-time Holder method. More recently, the high-temperature, short-time method has been investigated. Both processes lead to the appropriate inactivation of vegetative bacterial forms but are ineffective against bacterial spores.
Research Aims/Questions: We aimed to accomplish two main objectives: inactivation of all pathogens, including spores; and preservation of the activity of milk components.
Design/Methods: Recently, a novel high-hydrostatic pressure process has been developed by HPBioTECH. Using the same raw human milk samples, we compared the effects of this method with those of the Holder method on vegetative and spore forms of pathogens and on bioactive components (lipase activity, immunoproteins).
Results: Two main microbial strains were selected: Staphylococcus aureus (as a reference for vegetative forms) and Bacillus cereus (as a reference for spores). Use of the high-hydrostatic pressure process led to microbial decontamination of 6 log for both S. aureus and B. cereus. Additionally, the bioactivity of the main components of human milk was preserved, with activities of lipase, α-lactalbumin, casein, lysozyme, lactoferrin, and sIgA of ~80, 96–99, 98–100, 95–100, 93–97, and 63–64%, respectively.
Conclusions: Use of this novel high-hydrostatic pressure process to generate microbiologically safe human milk may provide important benefits for preterm infants, including improved assimilation of human milk (leading increased weight gain) and improved resistance to infections. Because 10% of all human milk collected is contaminated by B. cereus, use of this method will also prevent waste.
Introduction
Human milk is the appropriate standard nutrient for infant development (1) and is also given to preterm and very low birth weight infants (2, 3).
Two types of pathogens can contaminate such a medium: (i) endogenous pathogens from the mother and (ii) exogenous pathogens that mainly result from human milk collection by milk banks (4). Consequently, ensuring the safety and quality of donor human milk appears to be a crucial issue (5–8).
The main processes used for human milk pasteurization are based on thermal pathogen inactivation: (i) the low-temperature, long-time (LTLT) method (62.5°C; 30 min) (5, 6), which is also called the Holder method (traditionally developed in milk banks); and (ii) the high-temperature, short-time (HTST) method or flash heat pasteurization, which has been more recently investigated (9–13).
Regarding microbial safety, both processes (LTLT and HTST) lead to appropriate inactivation of the vegetative forms of pathogens; however, these methods are completely ineffective against bacterial spores from exogenous contamination. During the last few years, two other human milk treatments have been developed: UV (14–16) and ultrasound (17, 18).
In terms of preserving the activity of human milk after these pasteurization treatments, the LTLT process leads to many components (with nutritional enzymatic and immune properties) with reduced activity.
Over the last 25 years, high hydrostatic pressure (HHP) processes have been developed in food processing to mainly induce microbial safety (19–22), which also consequently increases shelf life. Because HHP processes apply weaker energy than thermal ones, their main advantage is the preservation of the intrinsic properties of the treated medium. More recently, HHP processes have been extended to biological applications (23–30).
The first industrial developments of HHP processes were established in Japan (1985–1990). In this first approach (called a “conventional approach”), HHP processes were defined by only three main parameters: pressure (P), temperature (T), and duration of treatment (t). When HHP processes are managed by only these three parameters, high pressure values (450–600 MPa) must be applied to ensure high microbial safety (31), which has a negative consequence of inducing the modification of biological components or organoleptic properties of the handled product (32). If high temperatures (80–120°C) are not used to induce detrimental modifications to biological activity, these HPP processes are ineffective at spore inactivation (33).
Definition of an HHP Process Applied to Human Milk
Different applications of this “conventional approach” to HHP treatment of human milk were tested with an emphasis on their ability to improve microbial safety. Viazis et al. (34) applied constant pressure (400 MPa) to human milk inoculated with different microorganisms [Staphylococcus aureus (ATCC 6538 and ATCC 25923), Streptococcus agalactiae (ATCC 12927), Listeria monocytogenes (ATCC 19115), and Escherichia coli (ATCC 25922)] to compare LTLT thermal pasteurization (Holder process) to high pressure treatment. The starting temperature was close to 21°C to reach a temperature of ~31°C due to adiabatic compression heating. Six- to eight-log reductions were observed in microbial populations during treatment. Unfortunately, this HHP treatment used a conventional approach and was ineffective against bacterial spores, particularly Bacillus cereus spores, which represent a microbial strain observed in the contamination of fresh milk, heat-treated milk and human milk (35, 36).
Research Aim
To establish an HHP process to inactivate both vegetative forms and bacterial spores contaminating human milk while preserving a substantial portion of the activity of milk components.
Methods
Considering that high temperatures are rejected and that the pressure–temperature range required for spore inactivation would also lead to strong alterations of the biological activity of human milk components, an HHP process that could induce the germination of bacterial spores at lower pressure conditions (a moderate pressure value: P ≈ 350 MPa) was needed to preserve the biological activity of human milk as required for infant feeding.
Recently, a new approach to HHP processes was established by Demazeau et al. (37), and this approach accounts for parameters that characterize pressure delivery. Specifically, the compression rate (VA) or decompression rate (VD), application mode (MA) (continuous or cyclic) and latency time (tl) between each cycle were defined.
Design
(i) To prove that this novel HHP was efficient for all pathogens with vegetative and spore forms, we performed a “challenge test.” To validate this novel approach to HPP processes for the decontamination of human milk, we inoculated sterilized human milk at a level of 6 log with two main strains of microorganisms: S. aureus (ATCC 6538), which is a gram-positive vegetative bacterium resistant to pressure inactivation (38), and bacterial spores of B. cereus (ATCC 14579), a sporulated bacterial form that can induce severe intestinal infections (39).
After applying various optimization tests, we defined the HHP experimental conditions based on 6 parameters that can inactivate all vegetative forms and bacterial spores (such as B. cereus spores).
The set of optimized process parameters was as follows:
Pressure = 350 MPa, temperature = 38°C, VA (application rate) = 1 MPa.s−1, MA (application mode) with na (number of cycles) = 4 cycles and ta (duration of each cycle) = 5 min, and tl (latency time with normal pressure between each cycle) = 5 min.
(ii) To demonstrate the conservation of bioactive components of human milk, we compared raw human milk with pasteurized and HHP treatments of the same sample.
We measured the main biologic components of human milk, including lipase activity, lactoferrin, lysozyme, and IgA under either Holder pasteurization (62.5°C, 30 min) or novel high hydrostatic pressure.
Setting
The high-hydrostatic pressure machine is located at HPbioTech, which is situated 10 km from the Human Milk Bank (HMB) of Bordeaux-Marmande.
We used human milk after consent from the mother. Raw human milk is pasteurized and stored at −18°C at HMB; when transferred to HPbioTech, it is stored at −80°C until analysis.
Sample
The pasteurized human milk was used to set of optimized process parameters of HHP. Sterile human milk was inoculated with 5–6 log S. aureus or B. cereus and treated with the optimized set of HHP. This was termed the “Challenge test.”
The raw human milk was treated with the optimized set of HHP to measure the biologic products of human milk, such as lipase activity and immune proteins lactoferrin, lysozyme, IgAs.
After HHP treatment, the challenge was employed to identify conditions that allow for destroying vegetative and spore forms of bacteria and preserving lipase activity and immune bioactive proteins.
Compared to Holder pasteurization, which destroyed 0 spores (see Table 1), the HHP sample size was determined to be 6 log of B. cereus spores and 6 log of S. aureus. The value obtained after Holder pasteurization showed no destruction of B. cereus, but no B. cereus was found after HHP treatment. Thus, very few samples are needed (see Table 1). We measured the reproducibility of destroying 6 log B. cereus in 3 repeated HHP treatments.
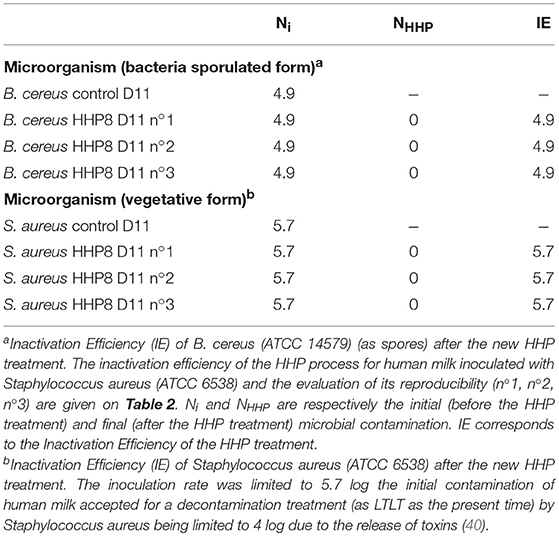
Table 1. Inactivation Efficiency (IE) of B. cereus (ATCC 14579) (as spores) and Staphylococcus aureus (ATCC 6538) after the new High Hydrostatic Pressure (HHP) treatment.
The Holder treatment destroyed all lipase activity (activity = 0), whereas between 70 and 100% of residual activity was found with the HHP treatment (see Table 3).
Data Analysis
We first verified the normality of the population and the homoscedasticity of variances. If verification was achieved, we used the Student t test to compare the two treatments (Holder vs. HHP); if not, we used the non-parametric test.
Two tailed p < 0.05 indicated significance.
Ethical Consideration
The milk used in this study was derived from the Human Milk Bank of Bordeaux-Marmande. Prior to donating milk, each mother signed a consent form indicating that any discarded milk could be used for research purposes. We therefore did not require approval for this study from the local Ethics Committee.
Moreover we can utilize human milk samples that cannot be used because the mother smokes or there are other contraindications to its donation.
Measurement
Protein and Lipid Analyses
Proteins
Caseins and the main soluble proteins were analyzed qualitatively and quantitatively using RP-HPLC coupled with Electro Spray Ionization-Mass Spectrometry (LC-MS); 50 samples of the same batch of raw HM (50), LTLTHM (50) and HPPHM (50) (INRA Jouy en Josas, Dr. P. Martin) were used (Figure 1).
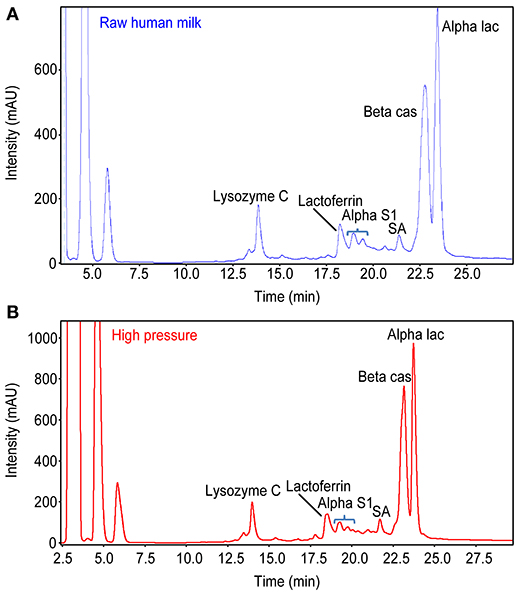
Figure 1. Comparison of proteins profile between (A) Raw Human Milk and (B) HHP Human Milk. There is strictly the same proteins profile of Raw HM and HHP HM.
Bioactive, antimicrobial and immune proteins: lactoferrin, lysozyme and IgA, showing more or less broad ranges of functions, were analyzed using Enzyme Linked ImmunoSorbent Assays (ELISAs) based on the sandwich technique; the antibody directed against the protein to analyze is pre-coated on the surface of microtiter wells. A biotinylated detection antibody is then added to the wells to bind to the captured protein. Streptavidin-conjugated horseradish peroxidase (SA-HRP) is then added to catalyze a colorimetric reaction with the chromogenic substrate 3,3′,5,5′-tetramethylbenzidine. The colorimetric reaction produces a blue product, which turns yellow when the reaction is terminated by addition of dilute sulfuric acid. The absorbance of the yellow product at 450 nm is proportional to the amount of protein present in the sample. The protein concentrations in the test samples can then be quantified by interpolating their absorbance from the standard curve generated in parallel with the samples (41).
Lipids
Lipase activity (Institut Biochimie Nutrition ITERG, Dr. C. Vaysse–Dr. L. Couedelo). The lipase activity compared to the substrate was monitored by quantitative release of fatty acids and glycerol generated during the hydrolysis of TAG lipase. The hydrolysis reaction is automatically followed by pH measurements via neutralization of fatty acids liberated by the enzyme over time by a standard solution of sodium hydroxide (NaOH 25 mmol/L) at pH 8 ± 0.2.
The results are expressed as the in International Units as micromoles of fatty acids liberated per minute and per milliliter of breast milk.
We used the granulometry of lipid droplets of human milk: raw, pasteurized, and novel HHP of the same batch (Figure 2).
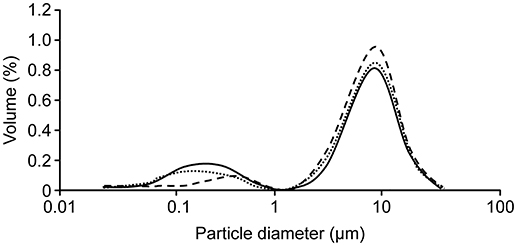
Figure 2. Distribution of the volume size of milk fat globules (MFGs): raw (____) pasteurized (- - - -) or high pressure (………). Evaluation of the size of MFGs showed that the population was bimodal he proportion of “small MFGs” was greater in raw milk and HHP-treated milk (d3.2 = 0.6 vs. 0.8 μm, respectively) compared to that in LTLT-pasteurized human milk (d3.2 = 3.1 μm). This result suggests that the size structure of raw breast milk is preserved by HHP treatment, whereas LTLT promotes coalescence and therefore increases the number of “large” MFGs.
Results
Microbial Spore and Vegetative Destruction
In these experimental conditions, total inactivation of the microbial contamination of human milk was possible in challenge tests with S. aureus and B. cereus spores.
The IE of the new HHP process for human milk inoculated with spores of B. cereus (ATCC 14579) and an evaluation of its reproducibility (n°1, n°2, and n°3) are provided in Table 1. Ni and NHHP are, respectively, the initial (prior to HHP treatment) and final (after HHP treatment) microbial concentrations. IE is provided for HHP treatment
Tables 1, 2 provide the corresponding inactivation efficiency (IE) of each microorganism. The HHP experiment and microbial analysis were repeated 3 times (n°1, n°2, and n°3) for the same human milk samples, with parameters of New HHP-P = 350 MPa, T = 38°C, MA = 4 × 5 min, Tl = 5 min, VA = 1 MPa/s
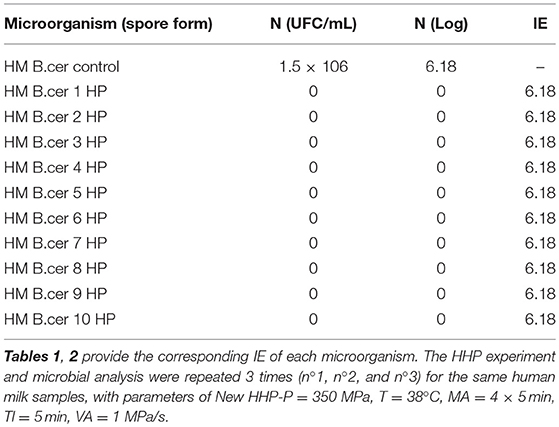
Table 2. The effect of High Hydrostatic Pressure (HHP) on 10 samples of (1.5 × 106) colonies of Bacillus cereus; HHP destroyed all the colonies, i.e., 1.5 × 106 colonies with Inactivation Efficiency (IE) = 6.18 Log.
The effect of HHP on 10 samples (1.5E+06), whereby colonies of B. Cereus were all destroyed
i.e., 1.5E+06 Colonies Inactivation Efficiency (IE) = 6.18 Log
In addition, this HHP process was evaluated for the inactivation of cytomegalovirus (CMV). This virus was selected due to its risk of human milk contamination and risk of postnatal infection (40, 42–44).
Different attempts were made using a suspension of cytomegalovirus with an initial viral particle concentration of up to 7 log. After application of the HHP treatment, which was characterized by the optimized process parameters used for the inactivation study of either S. aureus or bacterial spores of B. cereus, total CMV inactivation was observed.
Activity Retention of The Main Constituents of Human Milk
As a first evaluation, three main types of human milk components were selected:
- A component with enzymatic properties (lipase),
- Components with antimicrobial properties (lysozyme and lactoferrin) or with immunological properties (IgA), and
- Components with nutritional properties.
Impact of the HHP Process on Lipase Activity
An evaluation of lipase activity was important to compare the biological effects of this HPP process with those of thermal LTLT treatment (induction of total inactivation). The experimental conditions of HHP treatment were the same as those used for microbial decontamination (P = 350 MPa, T = 38°C, VA = 1 MPa.s−1, MA = 4 cycles of 5 min and tl ± 5 min).
Residual activities of the lipase enzyme in three samples (raw milk, LTLT-pasteurized milk and HHP-treated milk) and in three replicates (n°1, n°2, and n°3) for HHP treatment are provided in Table 3. Due to the variability of lipase activity in human milk, three different human milk samples (A, B, and C) were used. The lipase activity was completely destroyed (0% lipase activity) by LTLT, whereas lipase activity after the new HHP remained similar to its original value (Raw Human Milk) between 78.6 and 100% with a mean of 87.8% (Wilcoxon test p = 0.25) (Table 3).
The residual activity of the lipase enzyme after HHP processing of human milk was between 80 and 85% of the initial value of human milk treated at 38°C.
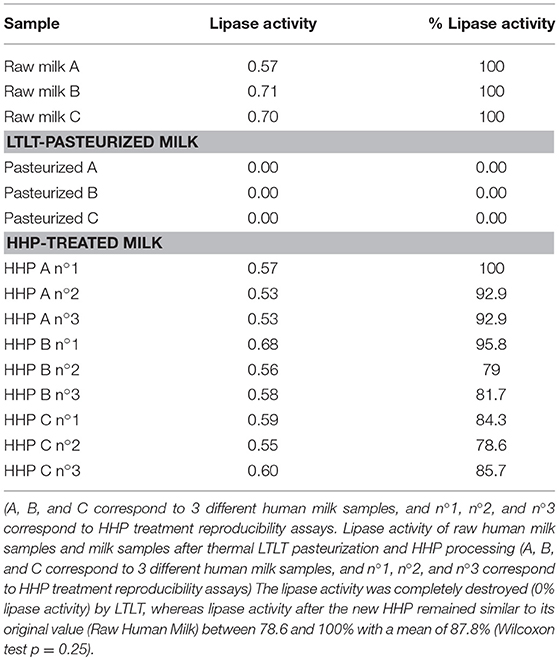
Table 3. Lipase activity of raw human milk samples and milk samples after thermal “Low-Temperature, Long-Time” (LTLT) pasteurization and High Hydrostatic Pressure (HHP) processing.
A comparison with the residual activity from the conventional HHP process [reproducing Viazis's et al. (34) HHP treatment] resulted in a residual lipase activity of close to 75%.
Impact of the HHP Process on the Activity of Different Components With Antibacterial or Immune Properties
The activity of biological components (lysozyme, lactoferrin, α-lactalbumin, and IgA) was also evaluated before and after HHP processing of human milk using the same set of experimental parameters (Table 5; Figure 1).
Considering the differences between our HHP process and those described in the literature for human milk, the following remarks can be made.
Impact of the HHP Process on Human Milk Components With Nutritional Properties Milk Fat Globule (MFG) Granulometry in Raw, LTLT-Pasteurized, and HHP-Treated Human Milk Using This Novel HHP Process
Evaluation of the size of MFGs showed that the population was bimodal with an approximately equivalent average diameter (d4.3) for all types of milk (raw milk: 5.5 μm; LTLT: 5.6 μm; and HHP: 5.4 μm). In addition, the proportion of “small MFGs” was greater in raw milk and HHP-treated milk (d3.2 = 0.6 vs. 0.8 μm, respectively) compared to that in LTLT-pasteurized human milk (d3.2 = 3.1 μm). This result suggests that the size structure of raw breast milk is preserved by HHP treatment, whereas LTLT promotes coalescence and therefore increases the number of “large” MFGs. In addition, the total fat content was similar regardless of the performed treatment (raw, LTLT and HHP: 34.0, 34.1, and 32.3 mg/mL milk, respectively) and of the fatty acid profile of the milk.
Discussion
For the first time, this new HHP process for the microbial safety of human milk can irreversibly inactivate both the vegetative forms of microorganisms, such as gram-positive bacteria including S. aureus, and bacterial spores, such as those of the contaminant B. cereus, while preserving at least 80% of the biological activity of the main components. Previously reported works involving HHP treatments were based on so-called “conventional” approaches in which the applied pressure was not controlled (15). Table 4 provides average values of the resulting microbial safety using three processes (LTLT, HTST and this new HHP) on human milk with S. aureus (gram-positive bacterium) and B. cereus (sporulated bacterium) as contamination references. Consequently, inactivation of bacterial spores, such as those of B. cereus, was not possible with a technique other than the new HHP.
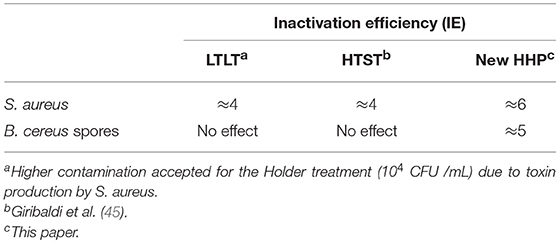
Table 4. Average values of the resulting microbial safety using Staphylococcus aureus (Gram-Positive Bacterium) and Bacillus cereus (Sporulated Bacterium) as contamination references.
The retention rates of the biological activity for different components with specific properties [BSSL lipase (with enzymatic properties), lysozyme and lactoferrin (characterized by antimicrobial properties), and IgA (with immunological properties)] are summarized in Table 5.
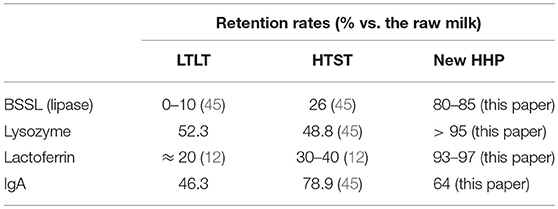
Table 5. Average retention rates of biological activity for different components with specific properties after “Low-temperature, long-time” (LTLT), “High-temperature, short-time” (HTST) and the new “High Hydrostatic Pressure” (HHP) treatments were applied to human milk.
Comparisons with the HTST process suggest that the retention rates of the biological activity of human milk components vary widely (particularly for BSSL) by author (9–13). In a recent paper by Giribaldi et al. (45), two aspects of the impact of the HTST process were evaluated: (i) microbial inactivation but not destruction of B. cereus and microbial spores and (ii) retention of the biological activity of human milk components using a specific HTST device for human milk pasteurization. Residual lysozyme activity was between 95% and 100% after application of our HHP process. Our value agrees with that reported by Viazis et al. (46) (96%) following HHP treatment of human milk at 400 MPa and 20°C. Viazis's et al. (34) HHP treatment resulted in a residual lipase activity of close to 75%.
Mayayo et al. (47) found that treatment at 300, 400, 500, and 600 MPa for 15 min and T = 20°C using the “conventional approach” to HHP processes denatured 9, 23, 34, and 48% of lactoferrin, respectively. In our approach, the retention rate of lactoferrin was over 93% (denaturation was below 7%) despite using a temperature of 38°C to limit the germination of B. cereus spores.
The residual activity of IgA was comparable to that obtained by Delgado et al. (48) (47.5% at 300 MPa and 50°C). In an early paper, Viazis et al. (46) found that high-pressure processing of human milk using the “conventional approach” at 400 MPa for 30, 60, 90, and 120 min and at a treatment temperature close to 31°C resulted in 85.6, 87.1, 80.6, and 75.4% retention, respectively. Permanyer et al. (49) claimed that after a treatment at 400 MPa for 5 min at 12°C, 100% of IgA activity was maintained, whereas IgA retention was 87.9 and 69.3% at higher pressure conditions (500 and 600 MPa, respectively). Contador et al. (50) evaluated the retention activity of IgA after high-pressure treatment at different pressures (400 and 600 MPa) and different treatment durations (3 and 6 min) with an initial temperature of 10°C at 400 MPa for 6 min; the retention of IgA activity was close to 90%.
Comparisons of these research studies suggest that IgA activity mainly depends on both the pressure and temperature of high-pressure treatment.
The retention rates of the biological activity for different components with specific properties [BSSL lipase (with enzymatic properties), lysozyme and lactoferrin (characterized by antimicrobial properties), and IgA (with immunological properties)] are summarized in Table 5.
Limitations
The new HHP process requires 90 min to treat human milk vs. 60 min for the Holder method; however, the cost of the HHP device is more than is a conventional pasteurizer. However, the new HHP saves up to 10% of material contaminated by B. cereus. For example, the Bordeaux Human Milk Bank collects 11,000 liters of human milk per year; 10% of this amount (or 1,100 liters) is contaminated with B. cereus and therefore must be discarded (51). This represents 165000€ /year, lost with the conventional pasteurizer per year, which would not be rejected with the new HHP.
Conclusion
This new HHP process is promising for implementation in human milk banks based on a comparison of three processes for the microbial safety and retention of the biological activity of different milk components. This approach is the first process that can inactivate bacterial spores, such as those of B. cereus; this point is important due to the risks of bacterial spores to preterm or young infants (52).
Author Contributions
GD and AP processed the human milk samples, and the results of bacteriological analyses were verified in double-blind experiments performed by PL of CHU. GD worked with AP to write the first version of the manuscript; unfortunately, GD is now deceased. PL performed a double-blind bacteriological study on the HHP samples from GD at the CHU. LC and PM performed the experiments, analyzed the data and wrote their part of manuscript and participated in revising the manuscript. Technis and results of Bacteriology was revised by PL and AP. The technics and results of Lipids by LC. The technics and results of proteins by PM. CB wrote the manuscript.
Funding
We received a grant of 150.000€ from the Conseil Regional d'Aquitaine.
Conflict of Interest Statement
This new high-pressure hydrostatic process was developed by GD (Pr. Emeritus at the Science University Bordeaux) who created a start-up called HPbiotech. He cooperated with the Centre Hospital University of Bordeaux, and in particular with CB, to coordinate a study of a new high hydrostatic pressure (HHP) process capable of destroying all vegetative and spore forms of pathogens. His research was protected by a patent (HPbiotech-CHU Bordeaux) prior to any publication. This process was not marketed, and this study was financed by a grant of 150000€ from the Conseil Regional d'Aquitaine. AP is a paid employee of HPbiotech. CB performs industrial and public research for Nestle, but these industrial grants do not interfere with the research described herein concerning the safety of donated human milk. All analyses were funded by the previously mentioned grant from the Conseil Regional d'Aquitaine.
The remaining authors declare that the research was conducted in the absence of any commercial or financial relationships that could be construed as a potential conflict of interest.
Acknowledgments
The authors would like to acknowledge Dr. Delphine Lamireau and the technicians from the Bordeaux Human Milk Bank. We also thank LC, Carole Vaysse and Laurence Fonseca for performing the lipid assay and the technicians from ITERG, Bordeaux University, for performing lipid measurement. We thank PM, G. Miranda, Z. Krupova, and L. Bianchi for performing the protein assay UMR1313 GABI, INRA, AgroParisTech, Université Paris-Saclay, 78350 Jouy-en-Josas, France.
References
1. Menon G, Williams TC. Human milk for preterm infants: why, what, when and how? Arch Dis Child Fetal Neonatal Ed. (2013) 98:F559–62. doi: 10.1136/archdischild-2012-303582
2. Chu CH. Breastfeeding: best for babies. Pediatr Neonatol. (2013) 54:351–2. doi: 10.1016/j.pedneo.2013.06.004
3. Tudehope DI. Human milk and the nutritional needs of preterm infants. J Pediatr. (2013) 162:S17–25. doi: 10.1016/j.jpeds.2012.11.049
4. Dewitte C, Courdent P, Charlet C, Dumoulin D, Courcol R, Pierrat V. [Contamination of human milk with aerobic flora: Evaluation of losses for a human milk bank]. Arch Pediatr. (2015) 22:461–7. doi: 10.1016/j.arcped.2015.02.011
5. ESPGHAN Committee on Nutrition Arslanoglu S, Corpeleijn W, Moro G, Braegger C, Campoy C, et al. Donor human milk for preterm infants: current evidence and research directions. J Pediatr Gastroenterol Nutr. (2013) 57:535–42. doi: 10.1097/MPG.0b013e3182a3af0a
6. Corpeleijn WE, Vermeulen MJ, van Vliet I, Kruger C, van Goudoever JB. Human milk banking - facts and issues to resolve. Nutrients (2010) 2:762–9. doi: 10.3390/nu2070762
7. Peila C, Emmerik NE, Giribaldi M, Stahl B, Ruitenberg JE, van Elburg RM, et al. Human milk processing: a systematic review of innovative techniques to ensure the safety and quality of donor milk. J Pediatr Gastroenterol Nutr. (2017) 64:353–61. doi: 10.1097/MPG.0000000000001435
8. Picaud JC, Buffin R. Human milk - treatment and quality of banked human milk. Clin Perinatol. (2017) 44:95–119. doi: 10.1016/j.clp.2016.11.003
9. Baro C, Giribaldi M, Arslanoglu S, Giuffrida MG, Dellavalle G, Conti A, et al. Effect of two pasteurization methods on the protein content of human milk. Front Biosci. (2011) 3:818–29.
10. Dhar J, Fichtali J, Skura BJ, Nakai S, Davidson AGF. Pasteurization efficiency of a HTST system for human milk. J Food Sci. (1996) 61:569–73. doi: 10.1111/j.1365-2621.1996.tb13160.x
11. Goldblum RM, Dill CW, Albrecht TB, Alford ES, Garza C, Goldman AS. Rapid high-temperature treatment of human milk. J Pediatr. (1984) 104:380–5. doi: 10.1016/S0022-3476(84)81099-9
12. Klotz D, Joellenbeck M, Winkler K, Kunze M, Huzly D, Hentschel R. High-temperature short-time pasteurisation of human breastmilk is efficient in retaining protein and reducing the bacterial count. Acta Paediatr. (2017) 106:763–7. doi: 10.1111/apa.13768
13. Terpstra FG, Rechtman DJ, Lee ML, Hoeij KV, Berg H, Van Engelenberg FACV, et al. Antimicrobial and antiviral effect of high-temperature short-time (HTST) pasteurization applied to human milk. Breastfeed Med. (2007) 2:27–33. doi: 10.1089/bfm.2006.0015
14. Christen L, Lai CT, Hartmann B, Hartmann PE, Geddes DT. The effect of UV-C pasteurization on bacteriostatic properties and immunological proteins of donor human milk. PLoS ONE (2013) 8:e85867. doi: 10.1371/journal.pone.0085867
15. Gabriel AA, Marquez GGF. Inactivation behaviors of selected bacteria in ultraviolet-C-treated human breast milk. Innov Food Sci Emerg Technol. (2017) 41:216–23. doi: 10.1016/j.ifset.2017.03.010
16. Martysiak-Zurowska D, Puta M, Kotarska J, Cybula K, Malinowska-Panczyk E, Kołodziejska I. The effect of UV-C irradiation on lipids and selected biologically active compounds in human milk. Int Dairy J. (2017) 66:42–8. doi: 10.1016/j.idairyj.2016.10.009
17. Christen L, Lai CT, Hartmann PE. Ultrasonication and the quality of human milk: variation of power and time of exposure. J Dairy Res. (2012) 79:361–6. doi: 10.1017/S0022029912000246
18. Czank C, Simmer K, Hartmann PE. Simultaneous pasteurization and homogenization of human milk by combining heat and ultrasound: effect on milk quality. J Dairy Res. (2010) 77:183–9. doi: 10.1017/S0022029909990483
19. Huang H-W, Lung H-M, Yang BB, Wang C-Y. Responses of microorganisms to high hydrostatic pressure processing. Food Control (2014) 40:250–9. doi: 10.1016/j.foodcont.2013.12.007
20. Huang H-W, Wu S-J, Lu J-K, Shyu Y-T, Wang C-Y. Current status and future trends of high-pressure processing in food industry. Food Control (2017) 72:1–8. doi: 10.1016/j.foodcont.2016.07.019
21. Rastogi NK, Raghavarao KSMS, Balasubramaniam VM, Niranjan K, Knorr D. Opportunities and challenges in high pressure processing of foods. Crit Rev Food Sci Nutr. (2007) 47:69–112. doi: 10.1080/10408390600626420
22. Wang CY, Huang HW, Hsu CP, Yang BB. Recent advances in food processing using high hydrostatic pressure technology. Crit Rev Food Sci Nutr. (2016) 56:527–40. doi: 10.1080/10408398.2012.745479
23. Aertsen A, Meersman F, Hendrickx MEG, Vogel RF, Michiels CW. Biotechnology under high pressure: applications and implications. Trends Biotechnol. (2009) 27:434–41. doi: 10.1016/j.tibtech.2009.04.001
24. Demazeau G, Rivalain N. High hydrostatic pressure and biology: a brief history. Appl Microbiol Biotechnol. (2011) 89:1305–14. doi: 10.1007/s00253-010-3070-9
25. Demazeau G, Rivalain N. The development of high hydrostatic pressure processes as an alternative to other pathogen reduction methods. J Appl Microbiol. (2011) 110:1359–69. doi: 10.1111/j.1365-2672.2011.05000.x
26. Masson P, Tonello C, Balny C. High-pressure biotechnology in medicine and pharmaceutical science. J Biomed Biotechnol. (2001) 1:85–8. doi: 10.1155/S1110724301000158
27. Meyer-Pittroff R. High pressure applications in medecine. In: Winter R, editor. Advances in High Pressure Bioscience and Biotechnology II. New York,NY: Springer (2003). p. 295–305. doi: 10.1007/978-3-662-05613-4_53
28. Rigaldie Y, Demazeau G. [Contribution of high pressure to pharmaceutical and medical science]. Ann Pharm Fr. (2004) 62:116–27. doi: 10.1016/S0003-4509(04)94290-3
29. Rivalain N (2009). Sur un Procédé Hautes Pressions de Sécurisation du Plasma Sanguin Humain. Ph.D. thesis, University Bordeaux I, no. 3910.
30. Rivalain N, Roquain J, Demazeau G. Development of high hydrostatic pressure in biosciences: pressure effect on biological structures and potential applications in biotechnologies. Biotechnol Adv. (2010) 28:659–72. doi: 10.1016/j.biotechadv.2010.04.001
31. Huppertz T, Smiddy MA, Upadhyay VK, Kelly AL. High-pressure-induced changes in bovine milk: a review. Int J Dairy Technol. (2006) 59:58–66. doi: 10.1111/j.1471-0307.2006.00246.x
32. Lambert Y, Demazeau G, Largeteau A, Bouvier JM. Changes in aromatic volatile composition of strawberry after high pressure treatment. Food Chem. (1999) 67:7–16. doi: 10.1016/S0308-8146(99)00084-9
33. Sevenich R, Kleinstueck E, Crews C, Anderson W, Pye C, Riddellova K, et al. High-pressure thermal sterilization: food safety and food quality of baby food puree. J Food Sci. (2014) 79:M230–7. doi: 10.1111/1750-3841.12345
34. Viazis S, Farkas BE, Jaykus LA. Inactivation of bacterial pathogens in human milk by high-pressure processing. J Food Prot. (2008) 71:109–18. doi: 10.4315/0362-028X-71.1.109
35. Aires GSB, Walter EHM, Junqueira VCA, Roig SM, Faria JAF. Bacillus cereus in refrigerated milk submitted to different heat treatments. J Food Prot. (2009) 72:1301–5. doi: 10.4315/0362-028X-72.6.1301
36. Bartoszewicz M, Hansen BM, Swiecicka I. The members of the Bacillus cereus group are commonly present contaminants of fresh and heat-treated milk. Food Microbiol. (2008) 25:588–96. doi: 10.1016/j.fm.2008.02.001
37. Demazeau G, Rivalain N, Billeaud C. Procédé de Traitement Sous Hautes Pressions d'un Milieu Pour L'inactivation des Spores Bactériennes. Bordeaux: French Patent N° 12 60214. (p. 26/10/2012) (2012).
38. Patterson MF, Quinn M, Simpson R, Gilmour A. Sensitivity of vegetative pathogens to high hydrostatic pressure treatment in phosphate-buffered saline and foods. J Food Prot. (1995) 58:524–9. doi: 10.4315/0362-028X-58.5.524
39. Decousser JW, Ramarao N, Duport C, Dorval M, Bourgeois-Nicolaos N, Guinebretière MH, et al. Bacillus cereus and severe intestinal infections in preterm neonates: putative role of pooled breast milk. Am J Infect Control (2013) 41:918–21. doi: 10.1016/j.ajic.2013.01.043
40. Kim JH, Chung E-J, Park HK, Moon SJ, Choi S-M, Oh SH. Postnatal cytomegalovirus infection in an extremely premature infant transmitted via breast milk: a case report. Korean J Pediatr. (2009) 52:1053–8. doi: 10.3345/kjp.2009.52.9.1053
41. Miranda G, Krupova Z, Bianchi L, Martin P. A novel LC-MS protein profiling method to characterize and quantify individual milk proteins and multiple isoforms. In: 10th Annual Symposium of the International Milk Genomics Consortium. Davis, CA: University of California, Davis Conference Center, (2013).
42. Kurath S, Halwachs-Baumann G, Müller W, Resch B. Transmission of cytomegalovirus via breast milk to the prematurely born infant: a systematic review. Clin Microbiol Infect. (2010) 16:1172–8. doi: 10.1111/j.1469-0691.2010.03140.x
43. Lawrence RM. Cytomegalovirus in human breast milk: risk to the premature infant. Breastfeed Med. (2006) 1:99–107. doi: 10.1089/bfm.2006.1.99
44. Numazaki K. Human cytomegalovirus infection of breast milk. FEMS Immunol Med Microbiol. (1997) 18:91–8. doi: 10.1111/j.1574-695X.1997.tb01032.x
45. Giribaldi M, Coscia A, Peila C, Antoniazzi S, Lamberti C, Ortoffi M, et al. Pasteurization of human milk by a benchtop high-temperature short-time device. Innov Food Sci Emerg Technol. (2016) 36:228–33. doi: 10.1016/j.ifset.2016.07.004
46. Viazis S, Farkas BE, Allen JC. Effects of high-pressure processing on immunoglobulin A and lysozyme activity in human milk. J Hum Lact. (2007) 23:253–61. doi: 10.1177/0890334407303945
47. Mayayo C, Montserrat M, Ramos SJ, Martínez-Lorenzo MJ, Calvo M, Sánchez L, et al. Kinetic parameters for high-pressure-induced denaturation of lactoferrin in human milk. Int Dairy J. (2014) 39:246–52. doi: 10.1016/j.idairyj.2014.07.001
48. Delgado FJ, Contador R, Álvarez-Barrientos A, Cava R, Delgado-Adámez J, Ramírez R. Effect of high pressure thermal processing on some essential nutrients and immunological components present in breast milk. Innov Food Sci Emerg Technol. (2013) 19:50–6. doi: 10.1016/j.ifset.2013.05.006
49. Permanyer M, Castellote C, Ramírez-Santana C, Audí C, Pérez-Cano FJ, Castell M, et al. Maintenance of breast milk immunoglobulin A after high-pressure processing. J Dairy Sci. (2010) 93:877–83. doi: 10.3168/jds.2009-2643
50. Contador R, Delgado-Adámez J, Delgado FJ, Cava R, Ramírez R. Effect of thermal pasteurisation or high pressure processing on immunoglobulin and leukocyte contents of human milk. Int Dairy J. (2013) 32:1–5. doi: 10.1016/j.idairyj.2013.03.006
51. Rigourd V, Barnier J, Ferroni A, Nicloux M, Hachem T, Magny J, et al. Recent actuality about Bacillus cereus and human milk bank: a new sensitive method for microbiological analysis of pasteurized milk. Eur J Clin Microbiol Infect Dis. (2018) 37:1297–303. doi: 10.1007/s10096-018-3249-z
Keywords: human milk, HHP, pasteurization, human milk bank, spores, lipase, immune proteins, CMV
Citation: Demazeau G, Plumecocq A, Lehours P, Martin P, Couëdelo L and Billeaud C (2018) A New High Hydrostatic Pressure Process to Assure the Microbial Safety of Human Milk While Preserving the Biological Activity of Its Main Components. Front. Public Health 6:306. doi: 10.3389/fpubh.2018.00306
Received: 07 July 2018; Accepted: 05 October 2018;
Published: 06 November 2018.
Edited by:
Guido Eugenio Moro, Associazione Italiana delle Banche del Latte Umano Donato (AIBLUD), ItalyReviewed by:
Shahanawaz Syed, Mahatma Gandhi Mission Institute of Health Sciences, IndiaCihad Dundar, Ondokuz Mayis University, Turkey
Copyright © 2018 Demazeau, Plumecocq, Lehours, Martin, Couëdelo and Billeaud. This is an open-access article distributed under the terms of the Creative Commons Attribution License (CC BY). The use, distribution or reproduction in other forums is permitted, provided the original author(s) and the copyright owner(s) are credited and that the original publication in this journal is cited, in accordance with accepted academic practice. No use, distribution or reproduction is permitted which does not comply with these terms.
*Correspondence: Claude Billeaud, claude.billeaud@chu-bordeaux.fr
†Deceased