- 1Department of Medical Laboratory Sciences, Masinde Muliro University of Science and Technology, Kakamega, Kenya
- 2Department of Zoological Sciences, Kenyatta University, Nairobi, Kenya
- 3School of Medicine, Catholic University of Health and Allied Sciences, Mwanza, Tanzania
- 4Tropical Pesticides Research Institute, Arusha, Tanzania
Background: Malaria vector control efforts have taken malaria related cases down to appreciable number per annum after large scale of intervention tools. Insecticides-based tools remain the major control option for malaria vectors in Kenya and, therefore, the potential of such programs to be compromised by the reported insecticide resistance is of major concern. The objective of this study was to evaluate the status of insecticide resistance in malaria vectors in different agro ecosystems from western Kenya.
Methods: The study was carried out in the lowlands and highlands of western Kenya namely; Ahero, Kisian, Chulaimbo, Emutete, Emakakha, Iguhu, and Kabula. World Health Organization tube bioassays was conducted using standard diagnostic dosages of Lambdacyhalothrin, Deltamethrin, Permethrin, DDT, Bendiocarb, and Malathion tested on Anopheles mosquitoes collected from seven sites; Ahero, Kisian, Chulaimbo, Emutete, Emakakha, Iguhu, and Kabula. Biochemical assays, where the enzymatic activity of three enzymes (monooxygenases, esterases, and glutathione S-transferases) were performed on susceptible and resistant mosquito populations. Wild mosquito populations were identified to species level using polymerase chain reaction (PCR). The species of the wild mosquito populations were identified to species level using PCR. Real-time PCR was performed on the susceptible and resistant mosquitoes after the WHO tube bioassays to determine the presence of knockdown resistance (kdr) allele.
Results: WHO susceptibility tests indicated that Anopheles gambiae showed resistance to Pyrethroids and DDT in all the study sites, to Bendiocarb in Iguhu and Kabula and susceptible to Malathion (100% mortality) in all the study sites. There was an elevation of monooxygenases and esterases enzymatic activities in resistant An. gambiae mosquito populations exposed to Lambdacyhalothrin, Permethrin, Deltamethrin and DDT but no elevation in glutathione S-transferases. A high frequency of L1014S allele was detected in An. gambiae s.s. population, but there was no kdr allele found in Anopheles arabiensis mosquitoes.
Conclusion: An. gambiae mosquitoes from western Kenya have developed phenotypic resistance to pyrethroids and DDT. Therefore, there is a need for further research covering different climatic zones with different agroeconomic activities for detailed report on current status of insecticide resistance in malaria vectors.
Introduction
Malaria control programs in Sub Saharan Africa involve the use of Pyrethroid insecticides both in long-lasting insecticide nets (LLINs) and for indoor residual spraying (IRS) (1). Currently, malaria control mainly depends on pyrethroids, the only class of insecticide approved to be impregnated on mosquito nets, and it is also being widely used in IRS programs in Africa (2). Proper use of these malaria control tools can result in a remarkable reduction of morbidity and mortality associated with malaria (3, 4). There is hope of elimination of malaria in Africa as the usage of LLINs and IRS have proven to be effective in control of malaria (5). However, the recent emergence of mosquitoes to pyrethroids has become a major problem and, therefore, a threat to malaria vector control interventions.
Other classes of insecticides are available and have been used in some regions for malaria control. For instance, organophosphates have been used for IRS in form of Fenithrothion, Malathion, and Priphos-methyl in some countries (6). They have shown to be highly potent to mosquitoes, although they have a relatively short residual activity of 2–3 months when used for IRS (6). The mode of action of organophosphates involves inhibition of cholinesterase, stopping the breakdown of acetylcholine, which results in the overstimulation of the neuromuscular leading to the death of the vector (7). Carbamates, have been used in form of Bendiocarb and Propoxur, for IRS. They act the same way as organophosphates and, like organophosphates, they are very effective, but have a short residual activity (7).
There are two main mechanisms of insecticide resistance, which include: target site alteration, which reduces the capability of the insecticide to bind to the insect, thus lowering the amount of the insecticide reaching the insect (8, 9). This type of resistance is exhibited by knockdown resistance (kdr) to pyrethroids and DDT, which is caused by mutation in the voltage-gated sodium channel (VGSC) (10). L1014F-kdr mutation is mainly found in Anopheles gambiae from West Africa where as L1014S-kdr mutation mainly seen in An. gambiae from East Africa regions (11). Resistance to Organophosphates and Carbamates is caused by Alteration of target site in An. gambiae single amino acid substitution of glycine to serine at position 119 in the catalytic domain of the acetylcholinesterase gene (12). The second mechanism of resistance involves metabolic resistance, which occurs when there is elevation in metabolic enzyme activities, which detoxifies insecticides before binding to the target site (13). Monooxygenases are the major group of enzymes responsible for pyrethroid metabolism in insects (14).
Previous studies have reported an increase in pyrethroid resistance in East Africa; Ethiopia, Tanzania, Sudan, Uganda, and Kenya (9, 15–21). Pyrethroid resistance in malaria vectors has been reported in Kenya (22–29). With wide spread resistance to pyrethroids and DDT, carbamate and organophosphate classes of insecticides are the possible alternatives that can be considered for malaria control. Few studies have addressed the patterns of insecticide resistance in different agro ecosystems and insecticide susceptibility profile to the available alternative insecticides such as organophosphates and carbamates for malaria control. The objective of this study was to assess the status of An. gambiae resistance to pyrethroids, carbamates, and organophosphates in the highlands and low lands of western Kenya.
Materials and Methods
Study Sites
The study was conducted in lowlands and highlands of Western Kenya namely Ahero, Kisian, Chulaimbo, Emutete, Emakakha, Iguhu, and Kabula. Ahero (00.17259°S, 034.91983°E, altitude 1,162–1,360 m above the sea level), Chulaimbo (00.03572°S, 034.62196°E, altitude 1,328–1,458 m above the sea level) and Kisian (00.02464°S, 033.60187°E, altitude 1,280–1,330 m above the sea level) are located in Kisumu County (Low lands); Emutete (34°64 E, 00°22°N, elevation 1,463–1,603 m above the sea level), and Emakakha (34°64′E, 0°22′N, 1,463–1,604 m above sea level), are found in Vihiga County (highlands); Iguhu (0°17′N, 34°74′E, and elevation 1,450–1,580 m above sea level) is located in Kakamega County (highland) and Kabula (00.54057°N, 034.56410°E, altitude 1,545 m above the sea level) is located in Bungoma County, in highland area (Figure 1).
Malaria transmission is perennial in the lowland sites of Ahero, Kisian, and Chulaimbo. An. gambiae sensu strict (s.s. ) and Anopheles arabiensis are both found in lowland sites (27) except Ahero, where only An. arabiensis has been collected since the late 1990s (21). The highland sites of Emutete, Emakakha, Iguhu, and Kabula have low seasonal malaria transmission, with peaks at the end of the long (early April to early June) and short (October–November) rainy seasons and high year-to-year variation. The prevalent malaria vector in the highlands is An. gambiae s.s. (27) and others in minority are Anopheles funestus (30, 31) and An. arabiensis (32).
Ethical Clearance
Ethical clearance was obtained from the Ethical Review Board at the Kenya Medical Research Institute and University of California, Irvine (SSC no 1382). Our study activities were authorized by the area chiefs, subchiefs and village elders. The permission to collect mosquito from the fields was obtained from the field owners. Our study did not pose any danger to the communities involved.
Mosquito Larvae Sampling
Anopheles mosquito larvae were collected from various aquatic habitats using standard dipper (350 ml) and pipettes. The samples were then transported to an insectary within KEMRI, Kisumu. They were then transferred to rearing pans and fed on a mixture of fish food and brewer’s yeast that was changed daily. After pupation, the pupa was transferred to cages where they were allowed to emerge to adults. The adult mosquitoes were fed on 10% sucrose in cotton swabs.
WHO Tube Bioassays
Two to five days old unfed female mosquitoes collected from seven study sites (Kabula, Iguhu, Emutete, Emakakha, Ahero, Chulaimbo, and Kisian) were used to test for the susceptibility to the following insecticides; Lambdacyhalothrin (0.05%), deltamethrin (4%), permethrin (0.75%), DDT (0.05%), malathion (5%); and bendiocarb (0.1%). Kisumu strain, a reference mosquito insectary susceptible colony reared at KEMRI was used as control. Mosquitoes were released and kept in the exposure tubes for 60 min and then transferred back to the paper cups. They were then maintained in the paper cups for 24 h (to allow them to recover) where they were supplied with a pad of a cotton-wool soaked in 10% sugar solution. The mortality of the mosquitoes was scored immediately after 24 h, and the susceptibility status of the mosquito populations graded according to the WHO standard (33). A total of 200 mosquitoes were tested per site per insecticide. Tests were carried out at 24–26°C and 80–90% relative humidity during the 1-h exposure period and the subsequent 24-h holding period, with a 12D:12N photophase. Susceptible and resistant mosquitoes from this bioassay were separately kept in RNA later solution at −20°C for species identification and molecular characterization.
Biochemical Assays
Expression of metabolic enzymes and total protein was measured using the microplate enzyme system protocol developed by Brogdon and others (34). Freshly collected mosquitoes were used for these assays from all seven study sites after exposure to WHO insecticide impregnated filter papers (35).
Species Identification Using Polymerase Chain Reaction (PCR)
The methodology developed by Scott and others was used for Anopheles species identification of mosquitoes exposed to WHO tube bioassays (36). DNA was extracted using ethanol precipitation from legs and wings (37). The two sibling species (An. gambiae s.s. and An. arabiensis) of the An. gambiae s.l. species complex were distinguished using conventional PCR (36).
Genotyping Using Real-time PCR
The presence of kdr gene in the mosquitoes preserved after the WHO bioassays was detected using real-time PCR. The DNA extraction was conducted using the protocol established by Collins and others (37). The genotyping of amino acid position 1014 of the VGSC was done using, following the methods of Bass et al (38) and its modification done by Mathias and others (39).
Data Analysis
The mortality of mosquitoes after WHO tube resistance bioassay was calculated using the formula below (40):
Susceptibility status of a mosquitoes was classified using the WHO criteria (98–100% mortality indicates susceptibility, 90–97% mortality suggests possibility of resistance that needs to be confirmed, and <90% mortality suggests resistance) (35). The data were labeled by the site of origin. The mortality of mosquitoes was compared between different sites and between different insecticides using ANOVA. The fold increase in the enzymatic activity was calculated by dividing the optical density of the resistant mosquitoes by that of susceptible mosquitoes from the same site and was done separately for all sites. of the frequency of kdr allele in different mosquito populations was calculated. To determine if these genotypes were under selection, Hardy–Weinberg equilibrium test for kdr genotypes was performed, and χ2 test was used to determine the significance of the departure from Hardy–Weinberg equilibrium.
Results
The Status of Phenotypic Resistance of the Mosquito Population Studied
Kisumu Strain, Ahero, Emakakha, and Emutete were highly susceptible to Bendiocarb with 100% mortality but did not differ significantly from Kisian and Chulaimbo mosquitoes, which had 99 and 98% mortality, respectively. Iguhu (87%) and Kabula (84%) mosquito populations had a significantly lower mortality compared to the other sites and also differed significantly (F = 92.89, P = 0.0001).
All mosquito populations showed remarkable resistance to DDT except Kisumu strain (Insectary colony) that showed 100% mortality. Ahero mosquito populations had significantly higher mortality (73%) compared to the other sites (F = 16.70, P < 0.0001). There were no significant differences between the mortality of the mosquitoes from Emakakha, Emutete, Iguhu, Kisian, and Chulaimbo. The lowest mortality was recorded in Kabula mosquito population, which was significantly different from Kisian mosquitoes, but did not differ from Emakakha, Emutete, Iguhu, and Chulaimbo mosquitoes (Figure 2).
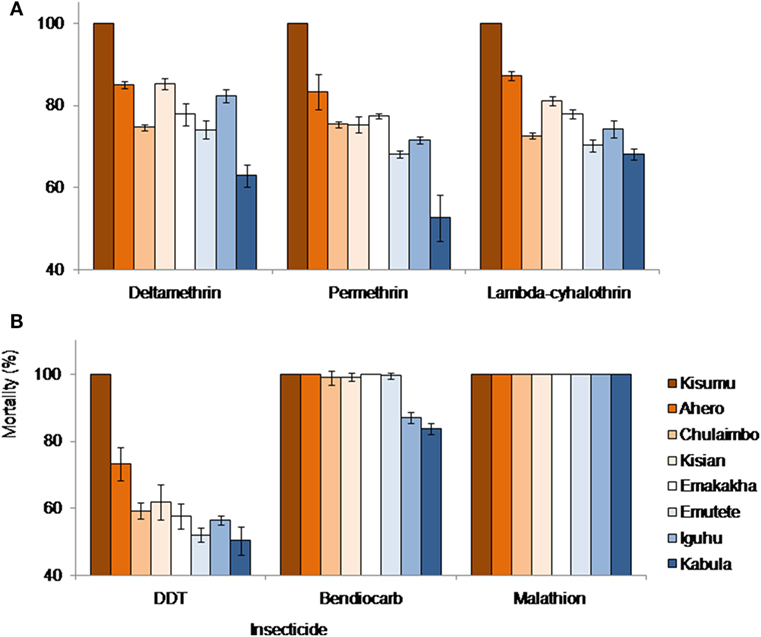
Figure 2. Anopheles gambiae s.l. mortalities against different insecticides and study sites. (A) Mortalities against different formulation of pyrethroid insecticides: deltamethrin, permethrin, and lambdacyhalothrin; (B) mortalities against DDT (Organochlorine), bendiocarb (Carbamate), and Malathion (Organophosphate). Kisumu strain was used as control.
Mosquitoes from all sites were resistant to lambdacyhalothrin (mortality <90%) except Kisumu strain that showed 100% mortality. Ahero mosquitoes had a significantly higher mortality compared to other sites, followed by Kisian mosquitoes, which were significantly different from Emakakha mosquitoes (F = 62.05, P = 0.0001). The mortality of Chulaimbo and Iguhu mosquitoes did not differ significantly. Kabula mosquitoes had the lowest mortality but did not differ significantly from Emutete and Chulaimbo mosquitoes.
When exposed to diagnostic dosage of Deltamethrin, Kisumu strain had a significantly high mortality compared to other sites, followed by the mortality of mosquitoes from Kisian mosquitoes, which did not differ significantly from the mortality of Ahero and Iguhu mosquitoes. There was no significant difference in the mortality of Emutete, Emakakha, and Chulaimbo mosquitoes. The mortality of Kabula mosquitoes was significantly lower than all the other sites (F = 55.65, P = 0.0001). Mosquitoes from all sites were resistant to Deltamethrin except Kisumu strain that was used as a control (Figure 2).
Finally, when exposed to Permethrin, the mortality of mosquitoes from Kisumu strain had significantly higher mortality compared to the other sites. The mortality of mosquitoes from Ahero significantly different from the mortality of Kisian, Chulaimbo and Emakakha mosquitoes. The mortality of Iguhu mosquitoes was not significantly different from that of Emutete mosquitoes. The mortality of Kabula mosquitoes was significantly lower compared to the other sites (F = 30.10, P = 0.0001). Mosquitoes from all the study sites except Kisumu strain were resistant to Permethrin (Figure 2).
Biochemical Assays
Metabolic Activity of Monooxygenases (P450)
There was an increase in the monooxygenases enzymatic activity in resistant mosquitoes exposed to Pyrethroids (Permethrin, lambdacyhalothrin, Deltamethrin) and DDT. There was a sevenfold increase in the monooxygenases enzymatic activity in Kabula mosquito populations exposed to lambdacyhalothrin, 2.5 in Emakakha, 1.8 Emutete populations, and 2 in Iguhu populations, but there was no increase in the monooxygenases activity in Kisian and Chulaimbo-resistant mosquitoes exposed to Lambdacyhalothrin (Figure 3).
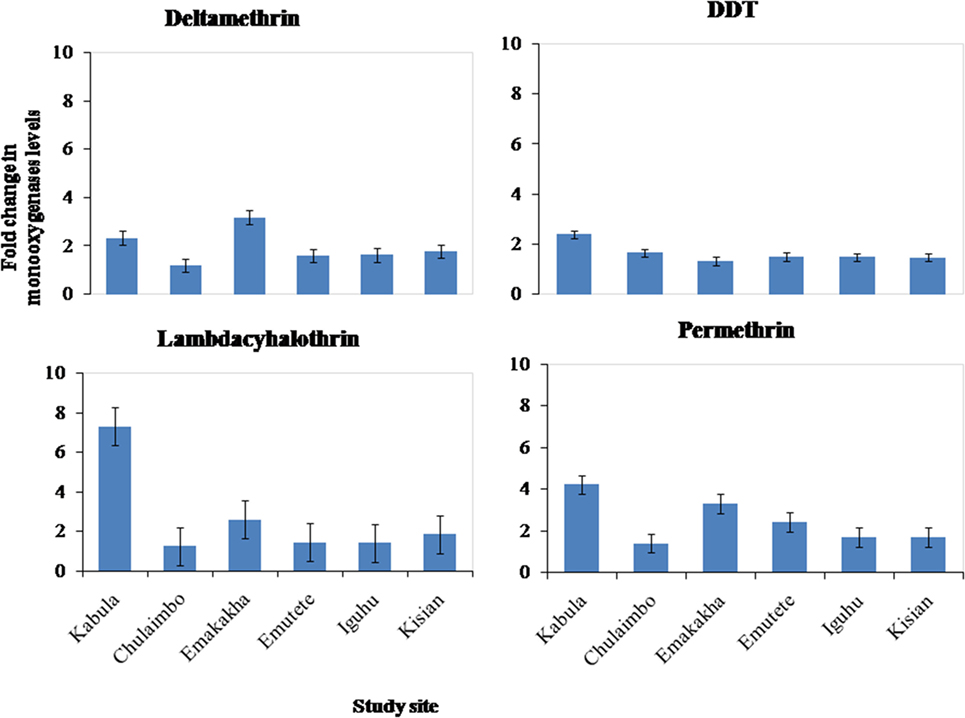
Figure 3. Fold change in the monooxygenases (P450) levels (±SE) in resistant mosquitoes against susceptible mosquitoes from the same study site.
There was a 4- and 2.5-fold in Kabula mosquito populations exposed to permethrin and deltamethrin, respectively, 3.8- and 3.6-fold in Emakakha populations, 3- and 2-fold in Emutete populations, 2- and 2-fold in Iguhu populations, and finally 2- and 2-fold increase in Kisian populations. The monooxygenase enzyme activity increased in resistant mosquito populations exposed to DDT in Kabula mosquito populations and there was a 2.5-fold increase, in Chulaimbo 1.8-fold increase, 1.7-fold increase in Emakakha, Emutete, Iguhu, and Kisian mosquito populations (Figure 3).
Metabolic Activity of Esterase Activity
There was also an increase in esterases activity in resistant mosquitoes exposed to pyrethroids (permethrin, lambdacyhalothrin, and deltamethrin) and DDT. There was a 1.1-, 1.2-, and 1.2-fold increase in Kabula mosquito populations exposed to Lambdacyhalothrin, permethrin, and deltamethrin, respectively, 1.2-, 1.8-, and 2-fold increase in Chulaimbo mosquito populations, 1.5-, 1.3-, and 1.5-fold increase in Emakakha populations, 1.5-, 1-, and 1.6-fold increase in Emutete populations, 1.4-, 1.7-, and 1.6-fold in Iguhu populations and finally 1.5-, 1.6-, and 1.5 in Kisian mosquito populations. There was also an increase in esterases activity in resistant mosquitoes exposed to DDT, 1.2-fold increase Kabula, Emutete, Iguhu, and Emutete mosquito populations, 1.5-fold increase in Chulaimbo populations, 1-fold increase in Kisian, and 0.7-fold increase in Emakakha mosquito populations (Figure 4).
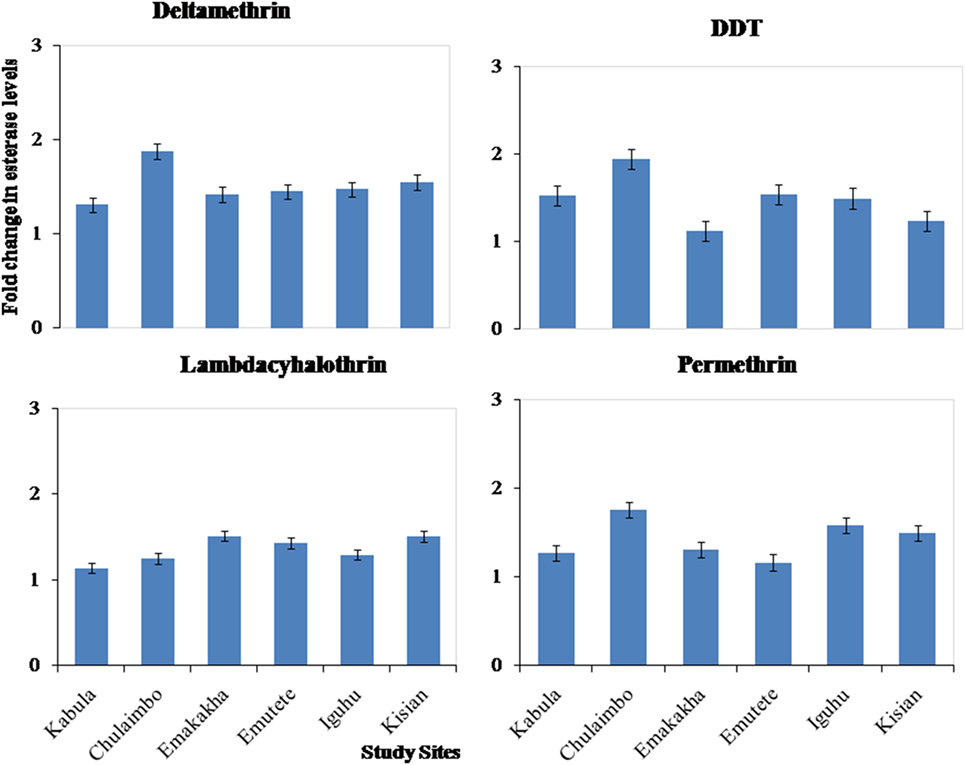
Figure 4. Fold changes in esterase levels (±SE) in resistant mosquitoes against susceptible mosquitoes from the same study site.
Glutathione-S-Transferase Enzymatic Activity
There was onefold change in the GST activity in the resistant mosquitoes that were exposed to pyrethroids and DDT in all the study sites (Figure 5).
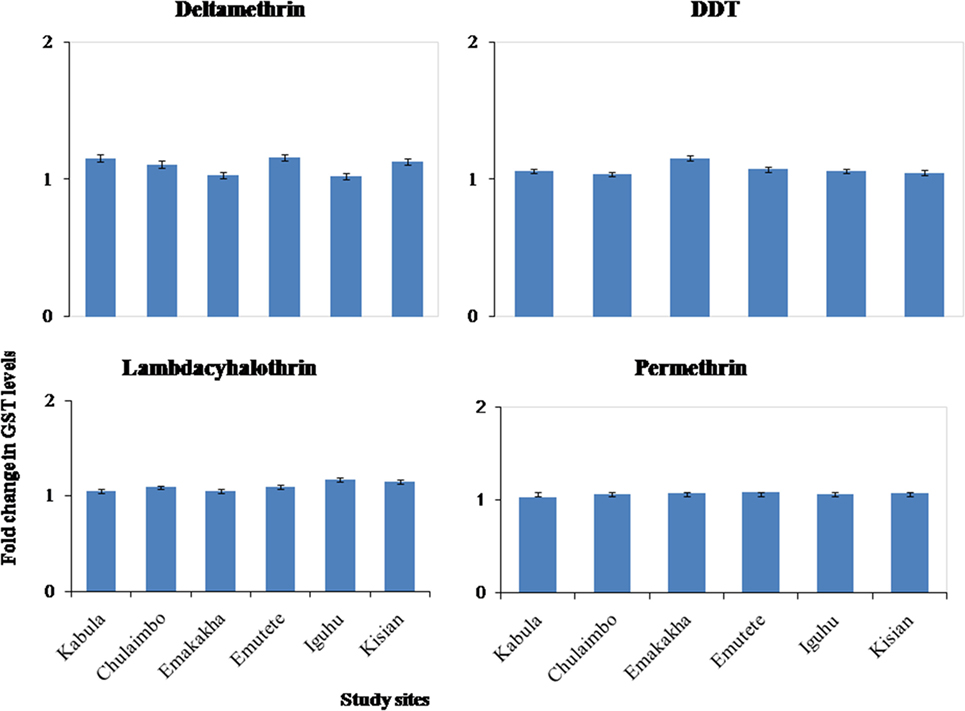
Figure 5. Fold changes in GST levels (±SE) in resistant mosquitoes against susceptible mosquitoes from the same study site.
Species Identification
Polymerase chain reaction results indicated that An. gambiae was the predominant species in Chulaimbo (72%), Emutete (94.0%), Emakakha (93.4%), Iguhu (88.0%), and Kabula (90.0%), where as An. arabiensis was predominant in Kisian (64.4%) and Ahero (89.3%) (Table 1).
Kdr Allele Genotyping
Homozyzygous kdr (L1014S) was high in An. gambiae s.s. populations, but relatively low in An. arabiensis dominant populations. The frequency of kdr allele was significantly high in Chulaimbo and Emakakha, followed by Emutete, Iguhu, and Kabula. The mosquitoes from Kisian had the lowest frequency of kdr allele in the An. gambiae mosquitoes. In An. arabiensis mosquitoes, the frequency of the kdr allele was high in Chulaimbo mosquitoes and Bungoma mosquitoes followed by Ahero, Iguhu, and finally Kisian. There was no mutation in the An. arabiensis mosquitoes from Emakakha. Kdr genotyping was not successful in 2.8% of the samples and mutations were observed in An. gambiae were homozygous. Only one population (Chulaimbo) out of the four An. arabiensis populations tested showed significant deviation that resulted from heterozygosity deficiency. On the other hand, five out of the six An. gambiae populations tested showed significant departure from Hardy–Weinberg equilibrium, all caused by heterozygosity deficiency (Table 2).
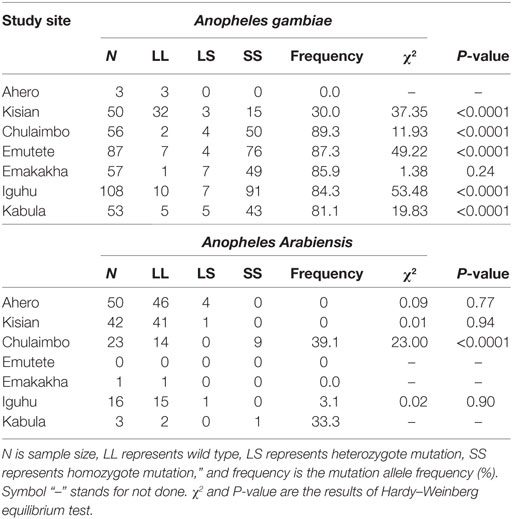
Table 2. Genotype and allele frequencies of knockdown resistance (kdr) at the seven study sites in western Kenya.
Discussion
Effective insecticide-based malaria vector control depends on the geographical location of the vectors and type of the insecticide used. Results from this study showed a marked variation of mortality of mosquitoes from different sites exposed to different classes of insecticide. The resistance of mosquitoes to insecticides that was observed in the study was brought about partly by elevation of metabolic enzymes and the presence of the resistance allele kdr in resistant mosquitoes.
Results from this study indicate that Malathion was highly potent to mosquitoes recording a mortality of 100% in all the study sites (Table 1). The mortality of mosquitoes from five study sites (Ahero, Kisian, Chulaimbo, Emutete, and Emakaha) was high when exposed to Bendiocarb, but resistance was observed in two sites, Iguhu and Kabula, when they were exposed to the same insecticide (Table 2). This suggests that mosquitoes in western Kenya have not yet developed resistance to Malathion and Bendiocarb. Mosquitoes from all the study sites showed marked resistance to DDT recording the highest mortality of 78% in Ahero mosquitoes. When exposed to pyrethroids (lambdacyhalothrin, permethrin, and deltamethrin), all mosquito populations showed resistance with the highest mortality of 87% observed in Ahero populations exposed to Lambdacyhalothrin. The difference in the mortality rates within different insecticide can be explained by the difference in the mode of actions, Malathion and Bendiocarb inhibit cholinesterase whereas pyrethroids and DDT are modulators of voltage gated sodium channels (7). The observed resistance of mosquitoes to pyrethroids can be caused by over use of this insecticide of malaria control in Kenya for control of Malaria, which has led to cross resistance in DDT, because the these classes of insecticides have the same similar mode of action (8).
The results from this study coincides with previous studies by Chouaibou et al. (41) who reported that An. gambiae populations in northern Cameroon were susceptible to carbamates and organophosphates but highly resistant to organochlorines and pyrethroids. However, they do not contradict the findings of Matowo et al. (42) who reported that An. arabiensis mosquito populations in Tanzania were highly susceptible to organochlorine (DDT), organophosphate, and carbamate, but highly resistant to pyrethroids. The observed pyrethroid resistance in western Kenya could be associated with massive scale up of insecticide-based vector control in Kenya and extensive use of this group of pesticide to control agricultural pests (28, 39).
Previous studies in Kenya reported that the localized use of insecticide-treated nets in Kisumu increased the permethrin tolerance of An. gambiae populations (43, 44). Stump et al. (23) also observed an increase in the level of mosquitoes to pyrethroids in western Kenya as a result of massive scale up of ITN distribution and usage. In Moshi, Tanzania, a field trial by Mosha et al. (45) reported that while commonly used ITNs killed relatively few host-seeking An. arabiensis, the nets continued to provide personal protection through the strong excito-repellent activity of permethrin (45). Kawada et al. (46) reported that resistant An. gambiae populations from Kenya were tolerant to excito-repellent activity of LLINs. However, LLINs have been reported to be still effective in malaria control, and the effect of insecticide resistance to malaria control has not been documented (47).
Results from this study indicated a significant difference in mortality of mosquitoes from different sites when exposed to Bendiocarb, Pyrethroids, and DDT. Ahero Mosquitoes recorded the highest mortality followed by Kisian, where as the mean mortality of mosquitoes from Emutete, Emakakha, and Iguhu did not differ significantly when exposed to the insecticides. Kabula Mosquito populations showed the lowest mortality when exposed to all the insecticide except Malathion. This suggests that topographical features can have an effect of the mosquito survival and longevity, thus affecting their response to insecticides (48–50). The marked resistance observed in Kabula mosquito populations can be linked to the spread of the resistance alleles from Uganda, since this region borders Uganda where insecticide resistance to pyrethroids is widespread (16, 19). The same observation was made by Ochomo et al. (27) who reported resistance in Bungoma mosquitoes and high susceptibility in Ahero Mosquitoes.
This study also reported the elevation of monooxygenases and esterases enzyme activity in resistant An. gambiae mosquito populations from Kabula, Iguhu, Emutete, Emakakha and Chulaimbo and Kisian exposed to Permethrin DDT, but no elevation of monooxygenases and esterases in An. arabiensis mosquitoes from Ahero. There was no elevation of GSTs in all mosquito populations. This suggests that the observed resistance to permethrin and DDT these areas could be caused by elevation of the enzyme activity of monooxygenases and esterases, but not the GSTs. For instance, mosquitoes from Kabula had the lowest mortality when exposed to pyrethroids and DDT, there was the highest fold change (eightfold) in the monooxygenase enzyme activity in the same mosquito population, when they were exposed to DDT. The lack of elevation in monooxygenases and esterase enzyme activity in Ahero mosquitoes could explain why the mosquitoes had the highest mortality when exposed to the same insecticides. In a similar study, Matowo et al. (42) eluded the observed pyrethroids and DDT resistance to the elevation of metabolic enzyme activities in the mosquito populations. The results from this study coincides with the previous studies by Ochomo et al. (27) that reported an elevation in esterases and monooxygenases enzyme activities in An. gambiae permethrin-resistant populations from Bungoma and Budalangi. Vulule et al. (43, 44) reported an elevation in both β-esterase and oxidase enzyme expression in An. gambiae permethrin-resistant populations, but there was no elevation in GST enzymes levels in the An. gambiae s.s. Furthermore, no elevation of monooxygenases enzyme activities was observed in An. arabiensis populations from Ahero even though this area had previously been covered by a pyrethroid-based IRS and LLINs. This contradicts the previous reports that extensive use of insecticide-based vector control methods could be selected for resistance in malaria vectors (23).
There was a marked phenotypic resistance to deltamethrin and lambdacyhalothrin in this study, but no measurable elevated expression of monooxygenases and esterases in resistant mosquitoes that had been exposed to deltamethrin and lambdacyhalothrin. This suggests that mechanisms of resistance to Deltamethrin and Lambdacyhalothrin could be due to target site alteration as a result of the presence of the kdr alleles, whereas resistance against permethrin and DDT may involve target site resistance and metabolic resistance as a result of the elevation of β-esterase and monooxygenase enzymes activities (8).
Results of species identification indicated that An. gambiae was the predominant species in Kabula, Iguhu, Emutete, Emakakha, and Chulaimbo whereas An. arabiensis is predominant in Ahero and Kisian. Lack of An. gambiae s.s. in Ahero mosquito populations could be explained by An. gambiae s.s. species shift to zoophilic An. arabiensis following scaling-up of ITNs as reported earlier by Mathias et al. (39). Previous studies in these sites have reported the abundance of An. gambiae in Bungoma, Emutete, and Iguhu (51) and An. funestus in Kisian and Chulaimbo (52).
The findings from this study also showed that the frequency of kdr allele was high in An. gambiae from Kabula, Emutete, and Emutete mosquito populations. This could be due to extensive insecticide-based vector control activities in this regions especially IRS with deltamethrin and lambdacyhalothrin, and use of LLINs. kdr mutations in An. gambiae s.s. has been well documented (27, 46, 53, 54). Compared to An. gambiae s.s., kdr mutations in An. arabiensis was low in the study areas (mainly Ahero and Kisian), although WHO bioassay test did show that An. arabiensis were resistant to DDT and pyrethroid insecticides. This suggests that other insecticide-resistant mechanisms could be present in these mosquitoes. However, previous study by Kawada et al. (46) reported the presence of kdr alleles in An. Arabiensis, which is in contrast to the findings from this study (46). In Ahero, rice is the predominant crop and pesticides have been frequently used for pest control in Ahero even before the scaling-up of ITNs, but the kdr mutation at L1014S rate was found to be low. The agricultural use of pesticide types are different from the insecticides commonly used for IRS and ITNs in the area, this mixed use of multiple type of insecticides may have delayed the development of resistance in An. arabiensis. The increase in frequency of the 1014S kdr allele in western Kenya populations reported in a previous studies (39) and confirmed by this study indicate that the insecticide resistances is spreading and gradually increasing in the mosquito populations. Therefore, there is need for development and usage of non insecticide-based ecological tools such as house modification and improved larval control technologies, which may contribute to new intergrated vector management strategies, to mitigate against further spread of insecticide resistance.
Study Limitation
The end point was not prespecified during study design, and the control of overall type I error rate was not possible during data analysis using the post hoc tests used. Therefore, the study was not adjusted for multiple hypothesis testing.
Author Contributions
CW participated in the study design, performed laboratory work, data collection, data analysis, and drafted the manuscript. EK participated in study design and contributed to manuscript writing. Both authors agreed upon submission of this manuscript.
Conflict of Interest Statement
The authors declare that the research was conducted in the absence of any commercial or financial relationships that could be construed as a potential conflict of interest.
Acknowledgments
The authors wish to thank the staff in the Climate and Human Health Research Unit, Centre for Global Health Research, Kenya Medical Research Institute, Kisumu, Kenya, for assistance with field mosquito collection. This study was supported by grants from the National Institutes of Health (R01 AI050243, D43 TW001505, and RO1 AI094580). This paper is published with permission from the director of Kenya Medical Research Institute.
References
1. World Health Organization. Malaria Entomology and Vector Control. Geneva: World Health Organization (2013).
2. WHOPES. WHO Recommended Insecticides for Indoor Residual Spraying against Malaria Vectors. (2015). Available from: http://www.hoint/whopes/quality/en
3. Brooke B, Koekemoer L, Kruger P, Urbach J, Misiani E, Coetzee M. Malaria vector control in South Africa. S Afr Med J (2013) 29:784–8. doi:10.7196/SAMJ.7447
4. Zhou G, Li JS, Ototo EN, Atieli HE, Githeko AK, Yan G. Evaluation of universal coverage of insecticide-treated nets in Western Kenya: field surveys. Malar J (2014) 13(1):351. doi:10.1186/1475-2875-13-351
5. Kweka EJ, Mazigo HD, Munga S, Magesa SM, Mboera LE. Challenges to malaria control and success stories in Africa. Glob Health Perspect (2013) 1, 72–80. doi:10.5645/ghp2013.01.014
6. World Health Organization. Cyhalothrin and Lambda-Cyhalothrin Health and Safety Guide’ Health and Safety Guide No. 38, International Programme on Chemical Safety. Geneva: World Health Organization (1990).
8. Ranson H, N’Guessan R, Lines J, Moiroux N, Nkuni Z, Corbel V. Pyrethroid resistance in African anopheline mosquitoes: what are the implications for malaria control? Trends Parasitol (2011) 27:91–8. doi:10.1016/j.pt.2010.08.004
9. Yewhalaw D, Kweka EJ. Insecticide resistance in East Africa – history, distribution and drawbacks on malaria vectors and disease control. In: Trdan S, editor. Insecticides Resistance. InTech (2016). Available from: http://www.intechopen.com/books/insecticides-resistance/insecticide-resistance-in-east-africa-history-distribution-and-drawbacks-on-malaria-vectors-and-dise
10. Martinez Torres D, Chandre F, Williamson MS, Darriet F, Berge JB, Devonshire AL, et al. Molecular characterization of pyrethroid knockdown resistance (kdr) in the major malaria vector Anopheles gambiae ss. Insect Mol Biol (1998) 7:179–84. doi:10.1046/j.1365-2583.1998.72062.x
11. Ranson H, Jensen B, Vulule JM, Wang X, Hemingway J, Collins FH. Identification of a point mutation in the voltage gated sodium channel gene of Kenyan An. gambiae associated with resistance to DDT and pyrethroids. Insect Mol Biol (2000) 9:491–7. doi:10.1046/j.1365-2583.2000.00209.x
12. Weill M, Fort P, Berthomieu A, Dubois MP, Pasteur N, Raymond M. A novel acetylcholinesterase gene in mosquitoes codes for the insecticide target and is non homologous to the ace gene Drosophila. Proc Biol Sci (2002) 269:2007. doi:10.1098/rspb.2002.2122
13. Hemingway J, Hawkes NJ, McCarroll L, Ranson H. The molecular basis of insecticide resistance in mosquitoes. Insect Biochem Mol Biol (2004) 34:653–65. doi:10.1016/j.ibmb.2004.03.018
14. Stevenson BJ, Bibby J, Pignatelli P, Muangnoicharoen S, O’Neill PM, Lian LY, et al. Cytochrome P450 6M2 from the malaria vector Anopheles gambiae metabolizes pyrethroids: sequential metabolism of deltamethrin revealed. Insect Biochem Mol Biol (2011) 41(7):492–502. doi:10.1016/j.ibmb.2011.02.003
15. Mbogo CM, Mwangangi JM, Nzovu J, Gu W, Yan G, Gunter J, et al. Spatial and temporal heterogeneity of An. mosquitoes and Plasmodium falciparum transmission along the Kenyan coast. Am J Trop Med Hyg (2003) 68(6):734–42.
16. Verhaeghen K, Van Bortel W, Roelants P, Backeljau T, Coosemans M. Detection of the East and West African KDR mutation in An. gambiae and An. arabiensis from Uganda using a new assay based on FRET/melt curve analysis. Malar J (2006) 5:16–9. doi:10.1186/1475-2875-5-16
17. Ramphul U, Boase T, Bass C, Okedi LM, Donnelly MJ, Müller P. Insecticide resistance and its association with target-site mutations in natural populations of Anopheles gambiae from eastern Uganda. Trans R Soc Trop Med Hyg (2009) 103(11):1121–6. doi:10.1016/j.trstmh.2009.02.014
18. Verhaeghen K, Van Bortel W, Trung HD, Sochantha T, Keokenchanh K, Coosemans M. Knockdown resistance in Anopheles vagus, An. sinensis, An. paraliae and An. peditaeniatus populations of the Mekong region. Parasit Vectors (2010) 3(1):59–63. doi:10.1186/1756-3305-3-59
19. Morgan JC, Irving H, Okedi LM, Steven A, Wondji CS. Pyrethroid resistance in an Anopheles funestus population from Uganda. PLoS One (2010) 5(7):11872. doi:10.1371/journal.pone.0011872
20. Matowo J, Kulkarni MA, Mosha FW, Oxborough RM, Kitau JA, Tenu F, et al. Biochemical basis of permethrin resistance in An. arabiensis from Lower Moshi, North-Eastern Tanzania. Malar J (2010) 9:193. doi:10.1186/1475-2875-9-193
21. Mahande AM, Dusfour I, Matias JR, Kweka EJ. Knockdown resistance, rdl alleles, and the annual entomological inoculation rate of wild mosquito populations from lower Moshi, Northern Tanzania. J Glob Infect Dis (2012) 4:114. doi:10.4103/0974-777X.96776
22. Okia M, Ndyomugyenyi R, Kirunda R, Byaruhanga J, Adibaku A, Lwamala S, et al. Bioefficacy of long-lasting insecticidal nets against pyrethroid-resistant populations of An. gambiae ss from different malaria transmission zones in Uganda. Parasit Vectors (2013) 6:130–7. doi:10.1186/1756-3305-6-130
23. Stump AD, Atieli FK, Vulule JM, Besansky NJ. Dynamics of the pyrethroid knockdown resistance allele in western Kenyan populations of An. gambiae in response to insecticide-treated bed net trials. Am J Trop Med Hyg (2004) 70:591–6.
24. Kamau L, Vulule J. Status of insecticide susceptibility in An. arabiensis from Mwea rice irrigation scheme, Central Kenya. Malar J (2006) 5:46. doi:10.1186/1475-2875-5-46
25. Vulule JM, Beach RF, Atieli FK, Mount DL, Roberts JM, Mwangi RW. Longterm use of permethrin-impregnated nets does not increase An. gambiae Permethrin tolerance. Med Vet Entomol (1996) 10:71–9. doi:10.1111/j.1365-2915.1996.tb00084.x
26. Bonizzoni M, Afrane Y, Dunn WA, Atieli FK, Zhou G, Zhong D, et al. Comparative transcriptome analyses of deltamethrin-resistant and-susceptible Anopheles gambiae mosquitoes from Kenya by RNA-Seq. PLoS One (2012) 7(9):44607. doi:10.1371/journal.pone.0044607
27. Ochomo E, Bayoh MN, Brogdon WG, Gimnig JE, Ouma C, Vulule JM, et al. Pyrethroid resistance in An. gambiae s.s. and An. arabiensis in western Kenya: phenotypic, metabolic and target site characterizations of three populations. Med Vet Entomol (2012) 27(2):156–64. doi:10.1111/j.1365-2915.2012.01039.x
28. Wanjala CL, Mbugi JP, Ototo E, Gesuge M, Afrane YA, Githeko AK, et al. Pyrethroid and DDT-resistance and organophosphate susceptibility among Anopheles mosquito species Western Kenya. Emerg Infect Dis (2015) 21:12–5. doi:10.3201/eid2112.150514
29. Githeko AK, Adungo NI, Karanja DM, Hawley WA, Vulule JM, Seroney IK, et al. Some observations on the biting behavior of Anopheles gambiae s.s., Anopheles arabiensis, and Anopheles funestus and their implications for malaria control. Exp Parasitol (1996) 82:306–15. doi:10.1006/expr.1996.0038
30. Kweka EJ, Zhou G, Lee MC, Gilbreath TM III, Mosha F, Munga S, et al. Evaluation of two methods of estimating larval habitat productivity in western Kenya highlands. Parasit Vectors (2011) 4:110. doi:10.1186/1756-3305-4-110
31. Kweka EJ, Kamau L, Munga S, Lee MC, Githeko AK, Yan G. A first report of Anopheles funestus sibling species in western Kenya highlands. Acta Trop (2013) 128(1):158–61. doi:10.1016/j.actatropica.2013.06.006
32. Kweka EJ, Zhou G, Munga S, Lee MC, Atieli HE, Nyindo M, et al. Anopheline larval habitats seasonality and species distribution: a prerequisite for effective targeted larval habitats control programmes. PLoS One (2012) 7(12):e52084. doi:10.1371/journal.pone.0052084
33. Hemingway J, Ranson H. Insecticide resistance in insect vectors of human disease. Annu Rev Entomol (2000) 45:371–91. doi:10.1146/annurev.ento.45.1.371
34. Brogdon WG, McAllister JC. Insecticide resistance and vector control. Emerg Infect Dis (1998) 4:605–9. doi:10.3201/eid0404.980410
35. World Health Organization. Test Procedures for Insecticide Resistance Monitoring in Malaria Vector Mosquitoes. Geneva: World Health Organization (2013). p. 6–17.
36. Scott JA, Brogdon WG, Collins FH. Identification of single specimens of the An. gambiae complex by the polymerase chain reaction. Am J Trop Med Hyg (1993) 49:520–6. doi:10.4269/ajtmh.1993.49.520
37. Collins FH, Mendez MA, Rasmussen MO, Mehaffey PC, Besansky NJ, Finnerty V. A ribosomal RNA gene probe differentiates member species of the An. gambiae complex. Am J Trop Med Hyg (1987) 37:37–45. doi:10.4269/ajtmh.1987.37.37
38. Bass C, Nikou D, Donely M, Williamson M, Ranson H, Ball A, et al. Detection of knockdown resistance (kdr) mutation in An. gambiae: a comparison of two new high-throughput assays with existing methods. Malar J (2007) 6:111–4. doi:10.1186/1475-2875-6-111
39. Mathias D, Ochomo E, Atieli F, Ombok M, Bayoh MN, Olang G, et al. Spatial and temporal variation in the kdr allele L1014S in An. gambiae ss and phenotypic variability in susceptibility to insecticides in Western Kenya. Malar J (2011) 10:10. doi:10.1186/1475-2875-10-10
40. WHO. Guidelines for Testing Mosquito Adulticides for Indoor Residual Spraying and Treatment of Mosquito Nets. WHO/CDS/NTD/WHOPES/GCDPP/2006.3 (2006). Available from: http://www.who.int/iris/handle/10665/69296
41. Chouaibou M, Etang J, Brevault T, Nwane P, Hinzoumbe CK, Mimpfoundi R, et al. Dynamics of insecticide resistance in the malaria vector An. gambiae s.l. from an area of extensive cotton cultivation in Northern Cameroon. Trop Med Int Health (2008) 13:476–86. doi:10.1111/j.1365-3156.2008.02025.x
42. Matowo J, Jones CM, Kabula B, Ranson H, Steen K, Mosha F, et al. Genetic basis of pyrethroid resistance in a population of Anopheles arabiensis, the primary malaria vector in Lower Moshi, North-Eastern Tanzania. Parasit Vectors (2014) 19(7):274–8. doi:10.1186/1756-3305-7-274
43. Vulule JM, Beach RF, Atieli FK, Roberts JM, Mount DL, Mwangi RW. Reduced susceptibility of An. gambiae to permethrin associated with the use of permethrine impregnated bednets and curtains in Kenya. Med Vet Entomol (1994) 8(1):71–5. doi:10.1111/j.1365-2915.1994.tb00389.x
44. Vulule JM, Beach RF, Atieli FK, McAllister JC, Brogdon WG, Roberts JM, et al. Elevated oxidase and esterase levels associated with permethrin tolerance in An. gambiae from Kenyan villages using permethrin impregnated nets. Med Vet Entomol (1999) 13(3):239–44. doi:10.1046/j.1365-2915.1999.00177.x
45. Mosha FW, Lyimo IN, Oxborough RM, Malima R, Tenu F, Matowo J, et al. Experimental hut evaluation of the pyrrole insecticide chlorfenapyr on bed nets for the control of An. arabiensis and Culex quinquefasciatus. Trop Med Int Health (2008) 5:644–52. doi:10.1111/j.1365-3156.2008.02058.x
46. Kawada H, Dida GO, Ohashi K, Komagata O, Kasai S, Tomita T, et al. Multimodal pyrethroid resistance in malaria vectors, An. gambiae ss, An. arabiensis, and An. funestus ss in western Kenya. PLoS One (2011) 6(8):e22574. doi:10.1371/journal.pone.0022574
47. Ochomo EO, Bayoh NM, Walker ED, Abongo BO, Ombok MO, Ouma C, et al. The efficacy of long-lasting nets with declining physical integrity may be compromised in areas with high levels of pyrethroid resistance. Malar J (2013) 24(12):368–71. doi:10.1186/1475-2875-12-368
48. Afrane YA, Zhou G, Lawson BW, Githeko AK, Yan G. Effects of microclimatic changes caused by deforestation on the survivorship and reproductive fitness of An. gambiae in western Kenya highlands. Am J Trop Med Hyg (2006) 74:772–8.
49. Githeko A, Ayisi J, Odada P, Atieli F, Ndenga B, Githure J, et al. Topography and malaria transmission heterogeneity in western Kenya highlands: prospects for focal vector control. Malar J (2006) 5:107–10. doi:10.1186/1475-2875-5-107
50. Wanjala CL, Waitumbi J, Zhou G, Githeko AK. Identification of malaria transmission and epidemic hotspots in the Western Kenya highlands: its application to malaria epidemic prediction. Parasit Vectors (2011) 4:81–2. doi:10.1186/1756-3305-4-81
51. Atieli FK, Munga SO, Ofulla AV, Vulule JM. The effect of repeated washing of long-lasting insecticide-treated nets (LLINs) on the feeding success and survival rates of An. gambiae. Malar J (2010) 9:29–32. doi:10.1186/1475-2875-9-304
52. Bayoh MN, Mathias DK, Odiere MR, Mutuku FM, Kamau L, Gimnig JE, et al. An. gambiae: historical population decline associated with regional distribution of insecticide-treated bednets in western Nyanza province, Kenya. Malar J (2010) 9:62–6. doi:10.1186/1475-2875-9-62
53. Okumu FO, Moore J, Mbeyela E, Sherlock M, Sangusangu R, Ligamba G, et al. A modified experimental hut design for studying responses of disease-transmitting mosquitoes to indoor interventions: the Ifakara experimental huts. PLoS One (2012) 7(2):e30967. doi:10.1371/journal.pone.0030967
54. Okumu FO, Mbeyela E, Lugamba E, Moore G, Janson N, Kenward D, et al. Comparative field evaluation of combinations of long-lasting insecticide treated nets and indoor residual spraying, relative to either method alone, for malaria prevention in an area where the main vector is An. arabiensis. Parasit Vectors (2013) 6:46–50. doi:10.1186/1756-3305-6-17
Keywords: malaria, control, resistance, Anopheles gambiae, Ahero, Kisian, Emutete, Kabula
Citation: Wanjala CL and Kweka EJ (2018) Malaria Vectors Insecticides Resistance in Different Agroecosystems in Western Kenya. Front. Public Health 6:55. doi: 10.3389/fpubh.2018.00055
Received: 15 September 2017; Accepted: 13 February 2018;
Published: 01 March 2018
Edited by:
Rubén Bueno-Marí, Lokimica Laboratorios, SpainReviewed by:
Terri Kang Johnson, Dexcom, Inc., United StatesMalathi Raghavan, Purdue University, United States
Copyright: © 2018 Wanjala and Kweka. This is an open-access article distributed under the terms of the Creative Commons Attribution License (CC BY). The use, distribution or reproduction in other forums is permitted, provided the original author(s) and the copyright owner are credited and that the original publication in this journal is cited, in accordance with accepted academic practice. No use, distribution or reproduction is permitted which does not comply with these terms.
*Correspondence: Christine Ludwin Wanjala, Y2x1ZHdpbjNAZ21haWwuY29t;
Eliningaya J. Kweka, a3dla2FlQHRwcmkub3IudHo=