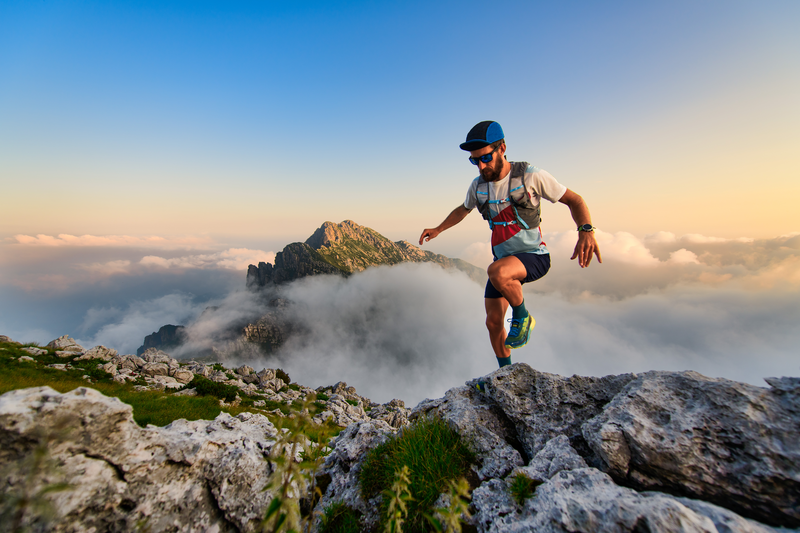
94% of researchers rate our articles as excellent or good
Learn more about the work of our research integrity team to safeguard the quality of each article we publish.
Find out more
CASE REPORT article
Front. Psychiatry
Sec. Psychopathology
Volume 16 - 2025 | doi: 10.3389/fpsyt.2025.1587875
This article is part of the Research Topic Diagnosis, Treatment or Prediction of Biomarkers in Depression View all articles
The final, formatted version of the article will be published soon.
You have multiple emails registered with Frontiers:
Please enter your email address:
If you already have an account, please login
You don't have a Frontiers account ? You can register here
Depression is a complex and heterogeneous mental health disorder affecting an estimated 280 million individuals worldwide. Although various antidepressant medications are available, a significant proportion of patients experience medication-resistant depression. This clinical case report highlights the critical importance of integrating pharmacogenomics into clinical practice, which is still not routinely done in many countries, through the detailed examination of a mid-20s male patient diagnosed with medication-resistant depression. Genetic analysis revealed specific variations in the cytochrome P450 genes, namely CYP2D6, CYP2C19, and CYP1A2, which are crucial for drug metabolism. By investigating the impact of these genetic variations on the patient's treatment response, we provide evidence-based recommendations for adjusting antidepressant medications based on the individual's unique pharmacogenomic profile. As demonstrated in the case report, this ultimately results in a positive clinical outcome and would have been advantageous to implement earlier as part of the patient's management.
Keywords: Antidepressant response, Medication-resistant depression, pharmacogenomics, CYP genes, PGx, Depression, clinical practice, case report
Received: 05 Mar 2025; Accepted: 21 Mar 2025.
Copyright: © 2025 Beer, Semmler and Voisey. This is an open-access article distributed under the terms of the Creative Commons Attribution License (CC BY). The use, distribution or reproduction in other forums is permitted, provided the original author(s) or licensor are credited and that the original publication in this journal is cited, in accordance with accepted academic practice. No use, distribution or reproduction is permitted which does not comply with these terms.
* Correspondence:
Joanne Voisey, School of Biomedical Sciences, Faculty of Health, Queensland University of Technology, Brisbane, Australia
Disclaimer: All claims expressed in this article are solely those of the authors and do not necessarily represent those of their affiliated organizations, or those of the publisher, the editors and the reviewers. Any product that may be evaluated in this article or claim that may be made by its manufacturer is not guaranteed or endorsed by the publisher.
Research integrity at Frontiers
Learn more about the work of our research integrity team to safeguard the quality of each article we publish.