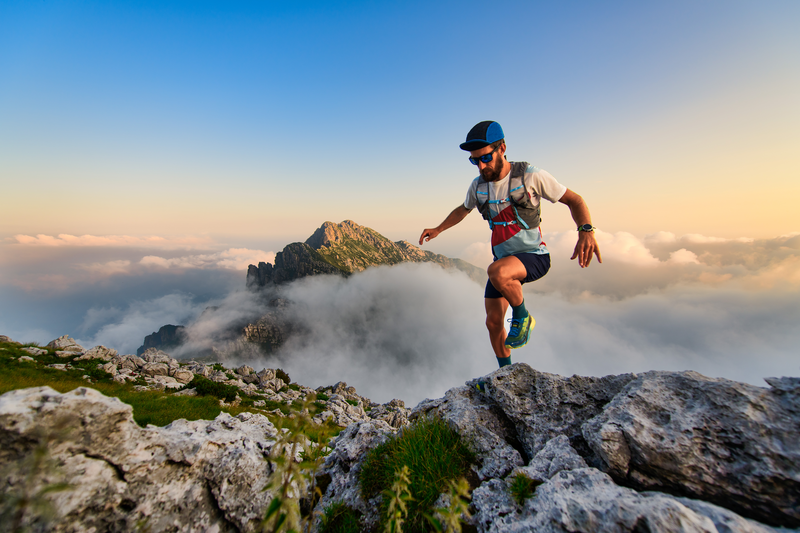
94% of researchers rate our articles as excellent or good
Learn more about the work of our research integrity team to safeguard the quality of each article we publish.
Find out more
ORIGINAL RESEARCH article
Front. Psychiatry
Sec. Adolescent and Young Adult Psychiatry
Volume 16 - 2025 | doi: 10.3389/fpsyt.2025.1555144
This article is part of the Research Topic Adolescent Emotional Disorders and Suicide Self-Harm Crisis Intervention View all 5 articles
The final, formatted version of the article will be published soon.
You have multiple emails registered with Frontiers:
Please enter your email address:
If you already have an account, please login
You don't have a Frontiers account ? You can register here
Adolescent depression is an increasingly serious public health issue, and traditional treatment methods often have side effects or limited efficacy. Electroconvulsive therapy (ECT), a widely used treatment for severe depression, has recently gained attention for its potential in treating adolescent depression. Previous studies suggest that mitochondrial dysfunction is closely related to the onset of depression. Therefore, investigating the mechanism by which ECT alleviates depressive symptoms through the improvement of mitochondrial energy metabolism is of great significance.This study employed the chronic unpredictable mild stress (CUMS) mouse model to assess the effects of ECT on depression-like behaviors through the sucrose preference test, open field test, and tail suspension test. Additionally, mitochondrial energy metabolism markers, including ATP levels, oxygen consumption rate (OCR), lactate, and pyruvate, were measured in both mouse and human plasma to evaluate the effects of ECT on mitochondrial function.The results showed that ECT significantly improved depression-like and anxiety-like behaviors in mice, as evidenced by the reversal of abnormal behaviors in the sucrose preference test, open field test, and tail suspension test. Analysis of plasma mitochondrial energy metabolism markers revealed that ECT significantly increased ATP levels, restored OCR, reduced lactate accumulation, and increased pyruvate levels. These findings suggest that ECT alleviates depressive symptoms by restoring mitochondrial energy metabolism and improving brain energy supply.This study systematically explored the potential mechanism by which ECT alleviates adolescent depression through the improvement of mitochondrial energy metabolism. The results indicate that ECT not only effectively alleviates depressive symptoms but also provides new insights and experimental evidence for the treatment of adolescent depression through mitochondrial function restoration. Future research could further investigate how to combine drug treatments to enhance mitochondrial function, improve ECT efficacy, and evaluate the effects of ECT in different depression subtypes, providing guidance for personalized clinical treatment.
Keywords: Electroconvulsive therapy (ECT), adolescent depression, Mitochondrial energy metabolism, Lactic Acid, Adenosine triphosphate (ATP)
Received: 03 Jan 2025; Accepted: 19 Mar 2025.
Copyright: © 2025 Du, Xie, Geng, Li, Xu, Wang, Gou, Tan, Xu, Shi, Chen, Chen, Zhou, Liu and Kuang. This is an open-access article distributed under the terms of the Creative Commons Attribution License (CC BY). The use, distribution or reproduction in other forums is permitted, provided the original author(s) or licensor are credited and that the original publication in this journal is cited, in accordance with accepted academic practice. No use, distribution or reproduction is permitted which does not comply with these terms.
* Correspondence:
Gang Liu, Department of Emergency and Critical Care Medicine, University-Town Hospital of Chongqing Medical University, Chongqing 401331, China, Chongqing, China
Li Kuang, Psychiatric Center,The First Affiliated Hospital of ChongQing Medical University, ChongQing,China, ChongQing, China
Disclaimer: All claims expressed in this article are solely those of the authors and do not necessarily represent those of their affiliated organizations, or those of the publisher, the editors and the reviewers. Any product that may be evaluated in this article or claim that may be made by its manufacturer is not guaranteed or endorsed by the publisher.
Research integrity at Frontiers
Learn more about the work of our research integrity team to safeguard the quality of each article we publish.