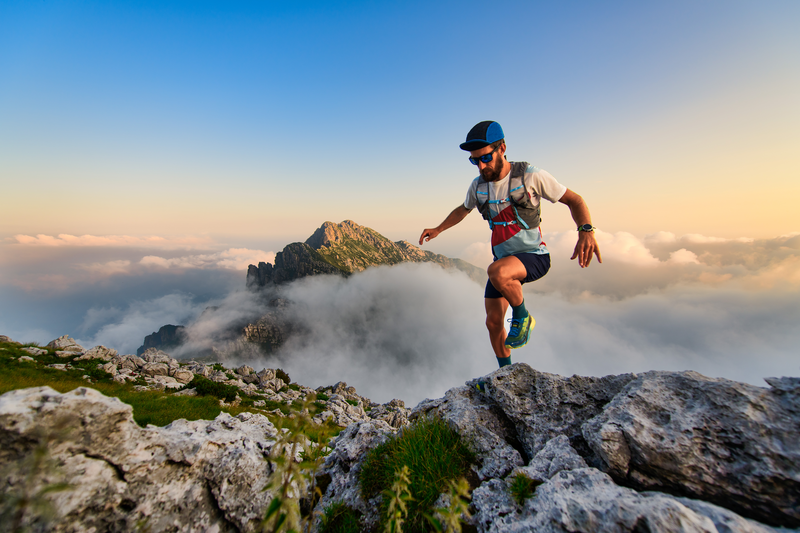
94% of researchers rate our articles as excellent or good
Learn more about the work of our research integrity team to safeguard the quality of each article we publish.
Find out more
ORIGINAL RESEARCH article
Front. Psychiatry
Sec. ADHD
Volume 16 - 2025 | doi: 10.3389/fpsyt.2025.1549318
This article is part of the Research Topic ADHD and Anxiety: Causality Sequences Through a Biopsychosocial Model View all 9 articles
The final, formatted version of the article will be published soon.
You have multiple emails registered with Frontiers:
Please enter your email address:
If you already have an account, please login
You don't have a Frontiers account ? You can register here
There is a high prevalence of fetal alcohol spectrum disorders (FASD) in the US and the world, which is caused by prenatal ethanol exposure (PE). Most individuals with FASD show attention deficit hyperactivity disorder (ADHD) -like symptoms. Using a rat model of FASD, we have successfully demonstrated that moderate and heavy PE leads to persistent attention deficits, including augmented impulsivity and impaired sustained attention. Anxiety is another primary symptom of FASD. Anxiety and ADHD are closely associated in clinical studies. However, the causal relationship between anxiety and ADHD is not clear. In the present study, we used the strategy of environmental enrichment to reduce anxiety after PE in rats and investigated if attention deficits could be ameliorated. A 2nd-trimester binge-drinking pattern of heavy PE was used. Environmental enrichment consisted of neonatal handling and postweaning complex housing. Action impulsivity and sustained attention were tested in adult males and females using the 2-choice reaction time task. The results show environmental enrichment effectively ameliorated action impulsivity and improved sustained attention in male and female PE rats. Action impulsivity was also improved in control rats with environmental enrichment. In addition, environmental enrichment improved the efficiency of obtaining rewards in male and female control but not PE rats. Environmental enrichment altered the pattern of reaction time components, favoring slower movement initiation but faster movement. These observations support that environmental enrichment could be an effective strategy in ameliorating ADHD-like symptoms in FASD. The reduced anxiety could contribute to such an effect.
Keywords: attention deficit-hyperactivity disorder, Anxiety, Fetal Alcohol Spectrum Disorders, impulsivity, sustained attention
Received: 20 Dec 2024; Accepted: 06 Mar 2025.
Copyright: © 2025 Wang, martin, Lei, Hausknecht, Richards, Haj-Dahmane and Shen. This is an open-access article distributed under the terms of the Creative Commons Attribution License (CC BY). The use, distribution or reproduction in other forums is permitted, provided the original author(s) or licensor are credited and that the original publication in this journal is cited, in accordance with accepted academic practice. No use, distribution or reproduction is permitted which does not comply with these terms.
* Correspondence:
Roh-Yu Shen, University at Buffalo, Buffalo, United States
Disclaimer: All claims expressed in this article are solely those of the authors and do not necessarily represent those of their affiliated organizations, or those of the publisher, the editors and the reviewers. Any product that may be evaluated in this article or claim that may be made by its manufacturer is not guaranteed or endorsed by the publisher.
Research integrity at Frontiers
Learn more about the work of our research integrity team to safeguard the quality of each article we publish.