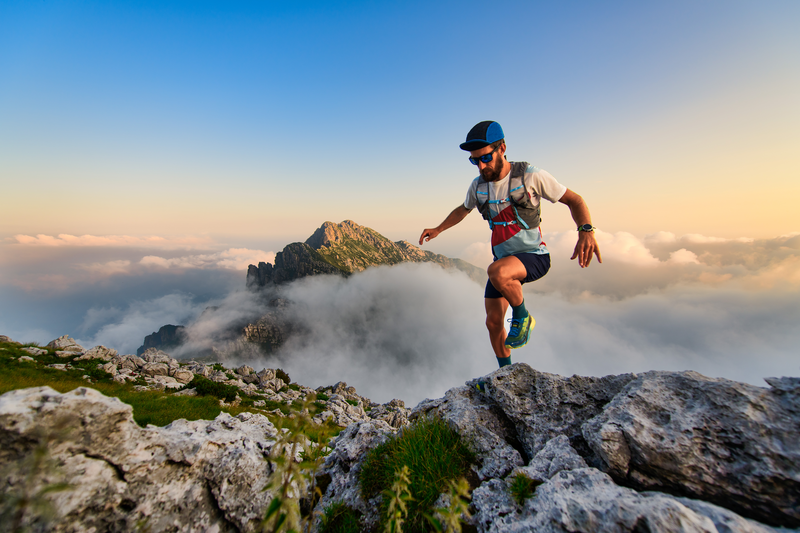
94% of researchers rate our articles as excellent or good
Learn more about the work of our research integrity team to safeguard the quality of each article we publish.
Find out more
ORIGINAL RESEARCH article
Front. Psychiatry
Sec. Psychopharmacology
Volume 16 - 2025 | doi: 10.3389/fpsyt.2025.1542000
The final, formatted version of the article will be published soon.
You have multiple emails registered with Frontiers:
Please enter your email address:
If you already have an account, please login
You don't have a Frontiers account ? You can register here
Background: Although several guidelines provide dosing recommendations for antidepressants based on patients’ genetic information, pharmacogenetic testing for antidepressant use is rarely routinely performed in Japan. To clarify the clinical impact of pharmacogenetic testing, this study estimated the potential drug-gene interactions for first-time antidepressant treatment in Japanese patients with major depressive disorder.Methods: This study retrospectively included Japanese patients who were registered for depressive episodes (F32, International Classification of Diseases, Tenth Revision) and initiated on antidepressants between July 2022 and March 2023. Antidepressant prescription rates were calculated using a nationwide hospital-based database (Medical Data Vision Co., Ltd). The incidence of actionable drug-gene interactions was estimated by multiplying the first-time prescription rate of each relevant antidepressant by the frequency of its corresponding actionable phenotype.Results: A total of 3,197 patients were included in the analysis. Escitalopram was the most frequently prescribed antidepressant (18.7%, n = 597), followed by mirtazapine (17.5%, n = 561), and sertraline (15.4%, n = 493). Of the patients receiving their first treatment of major depressive disorder, 56.5% (n = 1,807) were prescribed a drug with actionable pharmacogenetic implications, and 26.4% (n = 844) were estimated to have required actionable therapeutic recommendations. The highest incidence of actionable drug-gene interactions was observed in escitalopram and CYP2C19 pairs (12.4%, n = 398). For sertraline and CYP2C19 or CYP2B6 pairs, the incidence was 11.0% (n = 352). Among all antidepressants, paroxetine had the highest incidence of actionable drug-gene interactions related to CYP2D6 at 1.8% (n = 56); this interaction was rarely observed with other antidepressants (<1%).Conclusions: We estimated that one in four Japanese patients with major depressive disorder who were prescribed first-time antidepressants had actionable drug-gene interactions. These results suggest that pre-emptive pharmacogenetic testing in the treatment of major depressive disorder could have important clinical implications.
Keywords: Antidepressive Agents, CYP2B6, CYP2C19, CYP2D6, Depressive Disorder, Pharmacogenetics
Received: 09 Dec 2024; Accepted: 07 Mar 2025.
Copyright: © 2025 Hatano, Ikeda, Saito, Miyata, Iwata and Yamada. This is an open-access article distributed under the terms of the Creative Commons Attribution License (CC BY). The use, distribution or reproduction in other forums is permitted, provided the original author(s) or licensor are credited and that the original publication in this journal is cited, in accordance with accepted academic practice. No use, distribution or reproduction is permitted which does not comply with these terms.
* Correspondence:
Masakazu Hatano, Fujita Health University, Toyoake, Japan
Disclaimer: All claims expressed in this article are solely those of the authors and do not necessarily represent those of their affiliated organizations, or those of the publisher, the editors and the reviewers. Any product that may be evaluated in this article or claim that may be made by its manufacturer is not guaranteed or endorsed by the publisher.
Research integrity at Frontiers
Learn more about the work of our research integrity team to safeguard the quality of each article we publish.