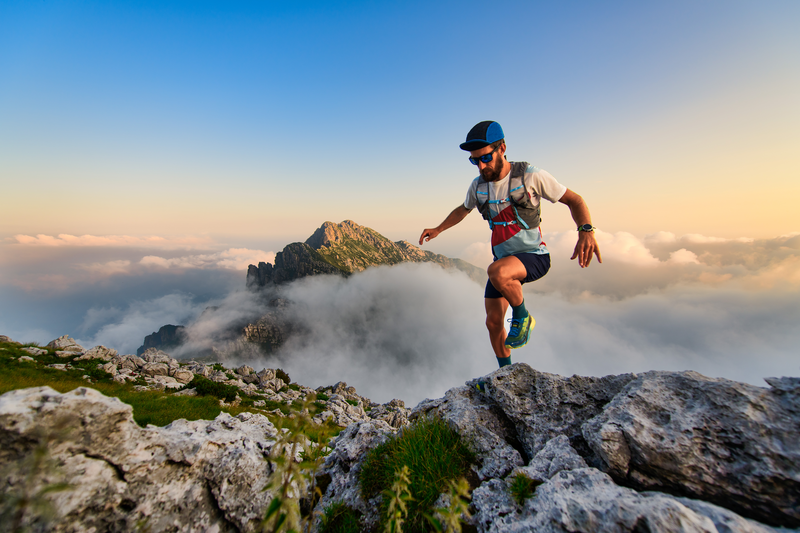
95% of researchers rate our articles as excellent or good
Learn more about the work of our research integrity team to safeguard the quality of each article we publish.
Find out more
REVIEW article
Front. Psychiatry , 27 January 2025
Sec. Psychopathology
Volume 16 - 2025 | https://doi.org/10.3389/fpsyt.2025.1518435
This article is part of the Research Topic Translational research advancements utilizing the Iowa Gambling Task in preclinical and clinical studies: 30 years of the IGT View all 7 articles
The Iowa gambling task is widely employed to assess the evaluation of risk versus reward contingencies, and how the evaluations are implemented to gain advantageous returns. The cognitive processes involved can be compromised in psychiatric conditions, leading to the development of analogous tasks with translational value for use in rodents. The rodent touchscreen apparatus maximises the degree of similarity with the human task, and in this review we provide an outline of the use of rodent touchscreen gambling tasks in preclinical studies of psychiatric conditions. In particular, we describe how the basic task has been adapted to probe the relative contributions of different neurotransmitter systems, and specific aspects of cognition. We then offer a perspective on how the task might be employed most beneficially in future studies.
The ability to make sound decisions is critical in everyday life and is influenced by cognitive control and emotional systems, underpinned by shifting influences of cortico-striato-thalamo-cortical networks. During decision-making, individuals evaluate the probabilities and risks associated with different options, and this includes during recreational pastimes such as gambling. Whilst gambling is often a harmless pursuit, for some decision making can become maladaptive, leading to a diagnosis of Gambling disorder, which is identified as a behavioural addiction under DSM-5 (1).
The Iowa gambling task (IGT) developed ~30 years ago by Bechara and co-workers (2), captures ‘real world’ decision making performance under ambiguous and risky conditions in a clinical laboratory setting. The IGT involves probabilistic learning via monetary reward and punishment. Participants choose from 4 decks of cards. Unbeknown to them, some decks are advantageous in the short term (large wins) but disadvantageous in the long term (large frequent losses) whereas others are less attractive in the short term (small wins) but advantageous over time (small, less frequent losses). This induces a conflict between immediate high rewards and long-term gains. Hence, the optimal strategy to maximise earnings on the task is to select cards that yield smaller gains over time but lower penalties and avoid the ‘high risk, high reward’ decks.
The dynamics of decision making in the IGT are complex and involve a range of neurophysiological processes, each underpinned by distinct neural networks. Participants must learn and remember the different contingencies as the task progresses, integrate affective and cognitive information over time into future strategy, along with inhibiting attractive but risky choices. Early ‘exploratory’ stages of the task that involve decision-making under uncertainty likely recruit the ‘emotional system’ and as the task progresses the ‘cognitive control’ system orchestrates instrumental behaviour to enable the best long-term option (3). As elegantly summarised throughout this Special Edition, the IGT has proved an important tool for probing aspects of cognitive function related to the perception and experience of risk and reward.
Rodent models of decision-making processes akin to the IGT are important in translational research, as these have the potential to increase understanding of the neurobiological mechanisms involved, which in turn can inform new treatments. The validity of animal models is frequently assessed against criteria of face, predictive and construct validity (4). Face validity is the similarity of what is observed in the animal model compared to human symptoms, predictive validity relates to the model’s potential to predict human processes, often with respect to identifying drug treatments, and construct validity signifies the extent to which the model has similar neurobiological processes to those in humans.
Several rodent versions of the IGT (rGT) have evolved, with an initial focus on maze-based non operant tasks and automated operant tasks in adapted 5-hole operant chambers (for reviews see (3, 5, 6). More recently, automated touchscreen operant tasks of the rGT have been adopted (7–11). In terms of satisfying criteria for animal models, these models exhibit good face validity despite differences in task features, training, single and multiple session learning processes and end point assessments (Table 1). Hence animals can make decisions in the face of uncertainty (similar to the human IGT) in the sense that they are able to evaluate which options are advantageous in the long-term and adapt their behaviour by avoiding risky options typically associated with larger rewards. All studies report that rats and mice successfully learn the task and show clear preference for the most optimal option. However, assessment of construct and predictive validity is more difficult to assess in these models, given the incomplete knowledge of the neurobiological processes in decision making and the lack of pharmacological treatments available for gambling. Features of construct validity will be discussed when relevant in evaluation of the tasks below. Nonetheless, the RDoC and CNTRICS initiatives have confirmed that the rGT has an important role to play in cross species translation (from rodent to human) of decision-making processes (12).
Table 1. Comparison of the characteristics of maze-based and operant rodent gambling tasks (5-hole chamber and Touchscreen) with the human IGT.
The first rGT protocol to be established was a non-automated 4 arm maze-based task for rats and mice (13, 14). The task measures choices between two goal arms for obtaining differing amounts of sugar pellet reward or punishment (bitter-tasting quinine pellet). For choices in the ‘advantageous’ arm there are low immediate rewards but with a net gain over time, whereas for choices in the ‘disadvantageous’ arm the chance of high immediate rewards is offset by high net loss in the long-term. Hence this task shows good face-validity as there is conflict between short-term and long-term pay off of choices as in the human IGT. Interestingly, there was a similar sex difference in reward-related decision making in this rat task as has been shown in humans (13). By comparison with operant based models and the human IGT, this paradigm does not differentiate between long-term outcome and frequency of options of reward/punishment. Variations of this non-operant maze-based task have been developed in mice (15, 16) with suggestions that the inter-individual differences in decision making offer good face-validity.
With the introduction of operant based automated tasks, there is the opportunity to investigate a wide range of neurocognitive parameters concurrently that are relevant to human gambling such as impulsivity, compulsivity and cognitive inflexibility The operant chamber rGT model introduced by Zeeb et al. (17) utilises the standard five-hole operant chamber typically used for the five-choice serial reaction time task adapted such that only four holes are used in the task. Since features of this task protocol have been adopted in recent touchscreen tasks for rats and mice, a brief resume of the face, predictive and construct validity of this task is described here. Animals have a choice between four distinct options which are similar to the four decks of cards in the IGT (face validity). The options differ in frequency and magnitude of reward/punishment possibilities. Nose poking in the two options which result in small numbers of sucrose pellets as rewards and short unpredictable penalties are ultimately advantageous compared to nose poking in the other two holes which result in higher numbers of rewards but higher unpredictable penalties (disadvantageous options). In this task, penalties are ‘time-out’ periods during which time no reward can be obtained and have been likened to ‘loss’ in the human task (18).
In this operant task, animals are first trained to make a basic operant response (nose-poke response) into an illuminated hole within a short timeframe, in order to receive a reward. Animals are then trained to experience four reinforcement contingencies in forced choice sessions before undertaking the decision-making tests. The advantage of this approach is that it ensures that all animals have equal exposure to the four different reinforcement contingencies and minimises any biases due to inadequate sampling. Thereafter the impact of manipulations (e.g. drugs or lesions) can be evaluated robustly using a “within subjects” design. Whilst it could be argued that task performance may relate more to the later phase of the IGT when contingencies are known (exploitation), the finding that choice options vary between sessions earlier in training suggests that exploration learning does occur. A further advantage of this rGT task is that, in addition to decision-making processes related to ‘risk/reward’ evaluation, output measures, including gambling-related premature responses (inhibitory/impulse control), perseverative (compulsive) behaviours, and also executive function (e.g. attention) can also be measured, thereby increasing face validity.
Construct validity relates in part to similar neurobiological systems underpinning the behaviour in animal and human tasks. As with human studies, components of the cortico-striato-thalamo-cortical circuits have been identified in the rGT (5). For example, lesion studies in rats (19, 20) have shown the amygdala and orbitofrontal cortex (OFC) to differentially affect exploration and exploitation phases of the operant based rat gambling task.
The development of mouse paradigms is important for improving construct validity since they allow the use of genetic manipulations (GM), optogenetic and imaging tools to probe neural systems, genetic and environmental risk factors relevant to gambling disorders. Young et al. (21) utilised a mouse version of the validated operant task (17), to demonstrate that knockdown of the dopamine transporter in GM mice resulted in increased risk-taking behaviour. By comparison alpha-synuclein deletion mice showed decreased impulsive action (premature responding) without an effect on risky decision making (22). However, there has been limited use of this mouse operant paradigm to dissect the neural circuits and systems that underpin behaviours relevant to gambling.
There is a huge unmet need in psychiatry to develop improvements in therapies for mental health conditions. The majority of clinical trials for new treatments fail in part due to limitations in forward and reverse translational approaches (23). Discrepancies between how behavioural constructs - in particular within neurocognitive domains - are assessed in animals and humans are a key factor in this problem.
The advent of touchscreen tasks for assessing cognitive domains in rodents (24, 25) is a significant advance for translational research. Commercially available touchscreen systems provide an opportunity to evaluate a range of cognitive constructs (e.g. learning and memory, executive function, reward learning, impulsivity and working memory) using tasks very similar/identical to those used to assess cognition in humans (e.g. CANTAB) (25–28), thereby providing superior face validity and translational potential compared to previous methods. As in human studies, animals are presented with visual stimuli (that can be easily manipulated) on a computer monitor before selecting a response. In the case of rodents this is achieved through a nose poke approach to an infra-red ‘touchscreen’ assembly.
By comparison with automated cognitive tasks in a five-hole operant chamber, touchscreen tasks offer similar advantages in that the automation enables high throughput of experiments under rigorously controlled conditions, measurement of several behaviours concurrently, the ability to test numerous subjects simultaneously, use of within-subject designs, minimisation of confounds related to animal handling, and less experimenter data analysis bias through computerised data collection. One limitation common to many operant tasks is that confounds such as motoric impairment, basic learning mechanisms and motivational factors can impact on task performance. These however can often be addressed by scrutinising variables such as trial omissions, or reaction times to collect rewards or produce a response.
Touchscreen tasks require animals to evaluate a visual stimulus on the touchscreen and approach the infrared screen to register a response without necessarily touching it (25, 27). This feature is considered to facilitate training and allows assessment of animals that may have motor impairments. However, a potential disadvantage, as compared to operant tasks that require nose-pokes to elicit a response, is that touchscreen tasks may not sustain behaviours relevant to motivation. These include paradigms such as progressive ratio (PR) for assessing motivation, and effort-related choice (ERC) related to decision-making. Importantly, Heath et al. (29) demonstrated that mice were indeed able to sustain vigorous repetitive responding in PR and ERC paradigms using the touchscreen, confirming that motivation and reward-related decision making are quantifiable in a touchscreen task. Moreover, responses to amphetamine and dopamine-receptor antagonists showed similar profiles to those found in lever and nose-poke operant versions of these tasks, suggesting that touchscreen tasks are suitable for assessing motivational factors and decision making.
Touchscreen tasks offer an advantage in that appetitive (reward) learning approaches are typically adopted (using reinforcers such as strawberry milkshake/food pellets) rather than aversive learning stimuli such as bitter-tasting food or mild electric shock. Stress is known to modulate cognitive performance, including that in IGT/rGT (30–32), so minimising any potential stressful confound is important. By utilising positive (appetitive) reinforcement and minimally stressful negative reinforcement (time-out), combined with minimal experimenter exposure through automation, touchscreen-based tasks represent an attractive strategy (33). Nevertheless, mice could be trained to obtain a large milkshake reward associated with varying probability of footstock, or a small amount of reward with no punishment in a risky decision-making task (34). However, the incorporation of aversive stimuli does have the potential to induce unwanted stress that could impact on endpoints of rGT paradigms.
The most obvious translational advantage of touchscreen-based paradigms, as compared to other operant paradigms, is that they maximise the similarity with the procedures and equipment used clinically. While the neural circuitry recruited between presentation of the visual stimulus and the ultimate selection of response and pressure on the screen in humans may not be completely characterised, it clearly makes sense to minimise any differences in procedure between species. The touchscreen approach further benefits from efficient standardisation and automation, flexibility in terms of applicability to other cognitive tasks, and minimal motor demands compared to other forms of apparatus (26, 27).
The application of touchscreen tasks in mice and rats is powerful for dissecting the impact of genetic variants upon neurocognitive behaviours, and also permits electrophysiological recordings and optogenetic manipulations to investigate the neurobiological mechanisms underpinning specific behaviours (35–38). Importantly, the ability to use similar types of visual stimuli, responses and reinforcers, using identical apparatus for different cognitive tasks, enables insight into the specific cognitive constructs important in an experimental model or manipulation. Whilst touchscreen tasks have been widely used to explore neurochemical mechanisms and neural systems important in a range of cognitive behaviours (25–27, 39–43), few studies have utilised rGT protocols.
The ability of the rGT to probe multiple aspects of cognitive function (see next section) allows the role of specific genes in these aspects of cognitive function to be assessed with great sensitivity. A transcriptomic study has explored the possibility that genomic differences may underlie cautious versus risk-seeking behaviours in the touchscreen version of the task (44). A direct link with glutamatergic synapse function has been proposed, since a peptide mimetic of the actin-regulatory protein radixin, injected into the rat nucleus accumbens, altered dendritic spine morphology and reduced rGT performance in risk-averse rats (38). This gene-specific approach has proved especially informative in relation to genes associated with schizophrenia risk, and we return to this in more detail below.
Decision making in the Iowa gambling task integrates a wide range of neurobehavioural and neurocognitive processes. These include reward processing (to evaluate risk-reward ratio), attention (constantly to monitor information), cognitive flexibility (to account for various outcomes), inhibitory control (to refrain from choosing immediate high reward option - impulsivity), and compulsivity (persistence/perseverative responses that do not relate to achieving the overall goal).
It has long been argued that substance-use-disorder (SUD) involves a shift from being more novelty-driven and impulsive to being more habit-driven and compulsive (45, 46). Given that DSM-5 has categorised gambling disorder in the same section as ‘Substance related and Addictive disorders’ it is important to assess these behaviours in experimental models using the same task, in order to understand the neurobiological mechanisms underpinning risk and protective factors for gambling and to inform new treatments.
The touchscreen platform provides an ideal opportunity to measure a range of cognitive behaviours concurrently and to dissect the precise neural mechanisms involved in decision making in the rGT. Importantly, studies are beginning to unravel the neural mechanisms of how genetic, neurodevelopmental and environmental factors impact on different behavioural and cognitive processes in the rGT, together with the impact of drugs on performance. Here we summarise some of the approaches used to investigate these factors, along with modifications of the basic rGT that allow additional behaviours relevant to gambling to be quantified.
Kim et al. (11) were the first to investigate the interaction of environmental factors with trait on decision making in a rat rGT touchscreen task using the method previously used for operant ‘nose poking’ chambers (17). Animals were trained (housed in pairs or isolation) to detect 4 choices differing in the probability and magnitude of reward (food pellet) and punishment (time-out). Once trained, they were assessed for risk-averse or risk preference behaviours under free choice conditions and their response to cocaine evaluated. Interestingly, rats could be divided into risk-averse and risk-seeking groups according to their preference for advantageous or disadvantageous choices in the free choice stage, indicating trait differences. Furthermore, pre-existing trait towards risk and the environment (housing conditions) interacted to affect decision making, and cocaine appeared to heighten this process.
Cho et al. (47) focussed on the impact of age on impulsivity measures in the rGT. Impulsivity can be broadly divided into ‘impulsive action’ and ‘impulsive choice’ (48). Impulsive action relates to premature responding through failure to inhibit an inappropriate response, whereas impulsive choice relates to impulsive decision making (choosing immediate rewards over more beneficial long-term rewards). Importantly, they found that rats exposed to the task early in life (late adolescents/young adults) showed increased impulsive action compared to those exposed as mature adults. By contrast, rats exposed to the task as mature adults showed an increase in impulsive choice after cocaine administration, which was only apparent in a sub-group of rats pre-categorised as ‘high impulsive action and risk averse’. These data highlight that the neurodevelopment period in which rats are exposed to the task differentially impacts on these two aspects of impulsivity, and that cocaine administration and/or stressors may be necessary to reveal differences. The neurobiological mechanisms underpinning these impulsive processes in neurodevelopment remain to be established, as well as whether they translate to human adolescence and vulnerability to gambling disorders.
One of the features of human pathological gambling is loss of control and the emergence of loss-chasing behaviour. Loss chasing is the drive to continue gambling despite successive and accumulating losses. It has been argued that different mechanisms mediate the commencement of gambling choices and their persistence, and that the neural systems recruited in the habitual nature of gambling differ from those involved in the goal-directed actions of loss chasing. To explore this, Humby et al. (7) developed a novel touchscreen task to assess gambling and loss-chasing performance under different win/loss probabilities in mice, and then assessed the impact of a 5-HT2C receptor antagonist and a 5-HT1A receptor agonist. The translational validity of the task was demonstrated as mice showed the expected patterns of behaviour when the odds for winning were altered, resembling behaviours seen in humans. Notably, antagonism of 5-HT2C receptors with SB242084 decreased the likelihood to initially gamble but did not affect loss chasing behaviour. By contrast the 5-HT1A receptor agonist 8-OH-DPAT did not affect initiation of gambling, but increased gambling choices once started. These findings support the involvement of distinct 5-HT receptors in mediating discrete components of gambling behaviours and provide the basis for further studies to explore the neurobiological mechanisms involved.
There is good cross-species correspondence in the brain circuitry involved in IGT performance. The core circuitry recruited to perform the task in rodents includes prefrontal cortex (PFC), OFC, amygdala and striatum/accumbens (5, 19, 49). Lateral OFC is believed to be involved in the integration of historical and recent choice outcomes (50).
Serotonergic pathways contribute to various aspects of cognitive function, including attentional process, executive function and impulse control, in a distributed neural network prominently including PFC and OFC (51). They are hence likely to contribute to risk/reward processing. As noted previously, antagonism of 5-HT2C receptors in mice seemed to reduce inclination to gamble, without affecting loss-chasing, but stimulation of 5-HT1A receptors (0.03mg/kg 8-OH-DPAT) tended to increase loss-chasing (7). This may be related to results in the 5-hole operant box version of the task where 8-OH-DPAT (0.3 mg/kg) in rats increased selection of the two least advantageous options (17). The 5HT2A/C agonist LSD also failed to modify option selection in mice (8). There is also little effect of LSD in a different gambling task, without any learning component, in humans (52).
Dopaminergic pathways, both mesocortical (to PFC/OFC) and mesolimbic (to accumbens), are important for various aspects of cognitive function, including executive processes (51). Clinical studies support a role for dopaminergic pathways in IGT performance. IGT performance is impaired in early-stage Parkinson’s disease (53–55), and with cocaine exposure (56, 57), yet no overt impairment is observed after amphetamine administration (58). In rodents, the results for indirectly-acting dopamine agonists with the touchscreen rGT largely parallel those obtained using the 5 hole operant box. Acute administration of cocaine (15mg/kg) had little overall effect on choice selection in rats (11, 47). Rats receiving a cocaine challenge (15mg/kg), having been withdrawn for 2 weeks after a week of repeated cocaine exposure, showed decreased selection of the optimal choice, and increased selection of the second-worst but not the worst option (11). However, a low dose of amphetamine (1.5mg/kg) in mice slightly increased selection of the 2nd best option, without affecting premature responses (10). A marginally higher dose (2mg/kg), also in mice, reportedly had no significant effect on choice selection, but with a tendency towards increased selection of the 2nd best option, while decreasing premature responses and increasing omission rates (8). In rats, amphetamine (0.3-1.0 mg/kg) also increased selection of the 2nd best option at the expense of the best option, while additionally increasing premature responding (17, 59–61). The same effect is seen with 2mg/kg amphetamine in mice (62), although here it was omissions that increased rather than premature responses.
The reason why amphetamine leads to slightly less advantageous responding, and in particular elevated responses at the 2nd best option, are unclear. Amphetamine does tend to increase impulsivity and slightly compromise attentional task performance in rodents (63). It may be that there is a reduction in the acuity of risk/reward assessment, but combined with an increased awareness of harm avoidance, leading to increased selection of the 2nd best choice, but not the least advantageous choices associated with greater levels of punishment.
Localised overexpression of D1 dopamine receptors in rat PFC increased selection of disadvantageous choices in the touchscreen rGT, and was interpreted as increased risk-taking (64). An agonist at D3 dopamine receptors increased selection of risky options in the 5 hole box rGT (65).
Pathological gambling behaviour is a particular problem associated with the use of directly acting dopaminergic agonists to treat Parkinson’s disease (66, 67). Pramipexole, an example of drugs of this class, increases risk-taking behaviour in the IGT in control subjects and in people with early Parkinson’s disease (68). Similar effects are observed in people with bipolar disorder (69). Equally, pramipexole is recently reported to increase selection of the 2nd worst option in control mice, and to increase selection of the least advantageous choice in mice with lesions of the dopamine system, using a touchscreen rGT (70). Hence there seems to be strong translational relevance of the rGT with dopaminergic agonists.
Modafinil, which facilitates dopaminergic and noradrenergic transmission via uptake transporter inhibition, along with other actions as well, reduced loss-chasing behaviour in a simplified touchscreen gambling task (7). No effect on choice selection was detected in a 5-hole operant box version of the task (62). The selective noradrenaline uptake inhibitor atomoxetine, administered acutely, did not affect gambling behaviour, including loss-chasing, but reduced premature responding (60, 61). Noradrenaline is known to play an important role in attention and executive processes (51, 71, 72). Interestingly, the alpha2 adrenoceptor agonist clonidine, while not affecting IGT performance in control subjects, produced a fairly clear improvement in heroin-users (73). A long-standing literature suggests functional links between alpha2 receptors and opioid receptors (74).
The widespread role of glutamatergic signalling in cognitive processes, in particular by NMDA receptors, would suggest that interfering with NMDA receptor signalling might impair performance on the IGT/rGT. Interestingly, just as with amphetamine, an NMDA receptor antagonist increases selection of the 2nd best option at the expense of the most favourable option in the 5-hole operant chamber rGT, while also dramatically increasing premature responses (75).
These psychiatric conditions arise due to a combination of genetic and non-genetic factors. In the case of schizophrenia, genetic advances have to some extent confirmed pre-existing ideas, based on drug effects, that there might be some fundamental alterations in the activity of dopaminergic and glutamatergic pathways (76, 77). These alterations are thought particularly to affect the neural circuitry centred on thalamic connections to PFC, OFC and hippocampus (78–80). Neurotransmitter disturbances in depression and bipolar disorder are less thoroughly investigated, but PFC/OFC dysfunction is also a core component of these conditions (81–84).
Compared to control subjects, people with schizophrenia, depression or bipolar disorder all show similarly altered levels of performance in the IGT (85–88), with reduced selection of the best choice and increased selection of the second worst choice (89, 90) or worst choice (91–93). The deficits observed in people with schizophrenia are particularly robust (88, 93–104). In depressed people, symptom severity correlates with harm avoidance, that is a tendency to avoid the least advantageous options (105).
A modification of the IGT that incorporates a contingency-shift element has proved especially informative for revealing altered cognitive risk/reward processes (103). In this modification, the reward/punishment contingencies associated with each choice option are modified during the course of the test session, this providing an indication of ability to adjust to differing risk/reward assessments and adapt behaviour accordingly. People with schizophrenia who were able to achieve levels of performance similar to controls in the baseline test then experienced difficulty in adjusting their responses when the contingences of 2 of the choices were switched. This could be interpreted as “harm avoidance”, manifest as a reluctance to select choices previously linked to unfavourable outcomes (103). There is some evidence that people with schizophrenia are particularly sensitive to the “worst” option, despite their impaired overall IGT performance (106). Anxiety, which is often present in schizophrenia (107, 108), is thought to impair IGT and rGT performance (5, 109), and can lead to avoidance of the worst IGT options (110). People prone to hallucinations/delusions were reported to perform at normal levels in the basic test, but to show impaired performance after the contingency shift (111), further suggesting that the manipulation increases sensitivity to detect impairment. People with depression are also impaired during this contingency-shift stage (90), showing an unwillingness to shift to the “previously bad, now good” choices. People with bipolar disorder taking the D2/D3 agonist pramipexole become more sensitive to gains than to losses (69).
We have adopted the touchscreen task to assess the impact of genetic manipulations relevant to schizophrenia upon mouse gambling performance, using four reward punishment contingencies based upon Zeeb et al. (17). The design of the task and the parameters used are summarised in Figure 1.
Figure 1. Task design and risk/reward contingencies as used by Thomson et al. (9).
GPR88 is an orphan G protein couple receptor enriched in striatal GABAergic medium spiny neurons, but also present in PFC (112). Based upon this location, GPR88 receptors are strategically placed to modulate the function of several cortico-striato-thalamo-cortical loops, suggesting potential utility as a target for treating schizophrenia and other neuropsychiatric conditions. Indeed, there is an interaction between Gpr88 and dopaminergic function (113–115), and we and others have reported impairments in mice lacking Gpr88 in cognitive domains involving PFC/OFC circuitry (9, 116).
Gpr88 KO mice show a perturbance of reward processing, selecting more risky choices (P4) at the expense of more advantageous, lower risk options (P1). At the same time, mice showed increased premature responding indicating motor impulsivity. These findings suggest a hyperdopaminergic phenotype, and together with performance in other tasks suggest that GPR88 KO mice are a useful model to evaluate novel targets for a range of cortico-striato-thalamo-cortical-mediated behaviours relevant to schizophrenia and other neuropsychiatric conditions (9). Hence these mice provide an opportunity to evaluate compounds and investigate neurobiological mechanisms from a transdiagnostic perspective.
We noted the particular difficulty that people with schizophrenia, or those prone to delusions and hallucinations, experience in the contingency-shifting modification of the IGT (103, 111). Common sequence variants in the MAP2K7 gene roughly double risk of schizophrenia (117). This is a large effect size for a common variant, making the gene of great interest for functional investigation in rodents. Mice heterozygous for deletion of the Map2k7 gene showed altered prepulse inhibition of the startle reflex, hyperlocomotion, and increased levels of omissions in a touchscreen attentional test, alongside reduced metabolic activity in the prefrontal cortex (39, 118). This is a pattern of phenotypes that closely relates to schizophrenia. There is also evidence for altered dopaminergic function in this strain of mice (118). However, when tested in the standard rGT, the mice performed at equivalent levels to control mice (10). We therefore incorporated a contingency-shifting component. When the two intermediate contingencies were switched, the Map2k7 hemizygous mice adapted as rapidly as the wild-type mice, showing no evidence for perseveration for the previous learned responses. However, when the best and worst options were switched, we found that the Map2k7 hemizygous mice were dramatically impaired, essentially being unable to complete the shift back to a more optimal choice selection, despite large numbers of retraining sessions (10). They continued to avoid the option that had previously been the worst choice. We hypothesise that the Map2k7 hemizygous mice are showing a form of harm avoidance behaviour, and that full function of the Map2k7 gene is necessary for overcoming this anxiety-related contribution to cognitive flexibility. Indeed, there is some evidence linking the MAP2K7 gene to panic disorder (119).
In summary touchscreen tasks are showing great promise as translational tools to dissect neurobiological mechanisms in the rGT. Since maladaptive decision making occurs in many psychiatric conditions, examining genetic, neurodevelopmental and environmental risk factors for these conditions in the rGT could provide greater insight into how these factors may cross current diagnostic boundaries and offer transdiagnostic opportunities to evaluate novel treatments. These studies also pave the way to dissect the specific neural circuits and neurotransmitter mechanisms underpinning distinct behaviours though the concurrent use of optogenetic approaches and in vivo electrophysiological recordings during evaluation of behaviours.
BM: Writing – original draft. JP: Writing – original draft.
The author(s) declare financial support was received for the research, authorship, and/or publication of this article. This research was supported by the Medical Research Council (UK), award no. MR/K501335/1.
The authors declare that the research was conducted in the absence of any commercial or financial relationships that could be construed as a potential conflict of interest.
The author(s) declare that no Generative AI was used in the creation of this manuscript.
All claims expressed in this article are solely those of the authors and do not necessarily represent those of their affiliated organizations, or those of the publisher, the editors and the reviewers. Any product that may be evaluated in this article, or claim that may be made by its manufacturer, is not guaranteed or endorsed by the publisher.
1. American Psychiatric Association. Diagnostic and statistical manual of mental disorders: DSM-5™. Washington, DC: American Psychiatric Publishing, Inc (2013). D.-T.F.
2. Bechara A, Damasio AR, Damasio H, Anderson SW. Insensitivity to future consequences following damage to human prefrontal cortex. Cognition. (1994) 50:7–15. doi: 10.1016/0010-0277(94)90018-3
3. van den Bos R, Koot S, de Visser L. A rodent version of the Iowa Gambling Task: 7 years of progress. Front Psychol. (2014) 5:203. doi: 10.3389/fpsyg.2014.00203
4. Pratt JA, Robinson ESJ, Fernandes C, Heal D, Stanford SC. BAP editorial: Improving the validity and translation of preclinical research. J Psychopharmacol. (2022) 36:779–80. doi: 10.1177/02698811221104064
5. de Visser L, Baars AM, Lavrijsen M, van der Weerd CM, van den Bos R. Decision-making performance is related to levels of anxiety and differential recruitment of frontostriatal areas in male rats. Neuroscience. (2011) 184:97–106. doi: 10.1016/j.neuroscience.2011.02.025
6. Winstanley CA, Clark L. Translational models of gambling-related decision-making. Curr Top Behav Neurosci. (2016) 28:93–120. doi: 10.1007/7854_2015_5014
7. Humby T, Smith GE, Small R, Davies W, Carter J, Bentley CA, et al. Effects of 5-HT(2C), 5-HT(1A) receptor challenges and modafinil on the initiation and persistence of gambling behaviours. Psychopharmacol (Berl). (2020) 237:1745–56. doi: 10.1007/s00213-020-05496-x
8. Elsilä LV, Korhonen N, Hyytiä P, Korpi ER. Acute lysergic acid diethylamide does not influence reward-driven decision making of C57BL/6 mice in the iowa gambling task. Front Pharmacol. (2020) 11:602770. doi: 10.3389/fphar.2020.602770
9. Thomson DM, Openshaw RL, Mitchell EJ, Kouskou M, Millan MJ, Mannoury la Cour C, et al. Impaired working memory, cognitive flexibility and reward processing in mice genetically lacking Gpr88: Evidence for a key role for Gpr88 in multiple cortico-striatal-thalamic circuits. Genes Brain Behav. (2021) 20:e12710. doi: 10.1111/gbb.12710
10. Openshaw RL, Pratt JA, Morris BJ. The schizophrenia risk gene Map2k7 regulates responding in a novel contingency-shifting rodent touchscreen gambling task. Dis Model Mech. (2022) 15. doi: 10.1242/dmm.049310
11. Kim WY, Cho BR, Kwak MJ, Kim JH. Interaction between trait and housing condition produces differential decision-making toward risk choice in a rat gambling task. Sci Rep. (2017) 7:5718. doi: 10.1038/s41598-017-06408-4
12. Cope ZA, Powell SB, Young JW. Modeling neurodevelopmental cognitive deficits in tasks with cross-species translational validity. Genes Brain Behav. (2016) 15:27–44. doi: 10.1111/gbb.2016.15.issue-1
13. van den Bos R, Jolles J, van der Knaap L, Baars A, de Visser L. Male and female Wistar rats differ in decision-making performance in a rodent version of the Iowa Gambling Task. Behav Brain Res. (2012) 234:375–9. doi: 10.1016/j.bbr.2012.07.015
14. van den Bos R, Lasthuis W, den Heijer E, van der Harst J, Spruijt B. Toward a rodent model of the Iowa gambling task. Behav Res Methods. (2006) 38:470–8. doi: 10.3758/BF03192801
15. Pittaras E, Rabat A, Granon S. The mouse gambling task: assessing individual decision-making strategies in mice. Bio Protoc. (2020) 10:e3479. doi: 10.21769/BioProtoc.3479
16. Cabeza L, Giustiniani J, Chabin T, Ramadan B, Joucla C, Nicolier M, et al. Modelling decision-making under uncertainty: A direct comparison study between human and mouse gambling data. Eur Neuropsychopharmacol. (2020) 31:58–68. doi: 10.1016/j.euroneuro.2019.11.005
17. Zeeb FD, Robbins TW, Winstanley CA. Serotonergic and dopaminergic modulation of gambling behavior as assessed using a novel rat gambling task. Neuropsychopharmacology. (2009) 34:2329–43. doi: 10.1038/npp.2009.62
18. Rivalan M, Ahmed SH, Dellu-Hagedorn F. Risk-prone individuals prefer the wrong options on a rat version of the Iowa Gambling Task. Biol Psychiatry. (2009) 66:743–9. doi: 10.1016/j.biopsych.2009.04.008
19. Zeeb FD, Winstanley CA. Lesions of the basolateral amygdala and orbitofrontal cortex differentially affect acquisition and performance of a rodent gambling task. J Neurosci. (2011) 31:2197–204. doi: 10.1523/JNEUROSCI.5597-10.2011
20. Pais-Vieira M, Lima D, Galhardo V. Orbitofrontal cortex lesions disrupt risk assessment in a novel serial decision-making task for rats. Neuroscience. (2007) 145:225–31. doi: 10.1016/j.neuroscience.2006.11.058
21. Young JW, van Enkhuizen J, Winstanley CA, Geyer MA. Increased risk-taking behavior in dopamine transporter knockdown mice: further support for a mouse model of mania. J Psychopharmacol. (2011) 25:934–43. doi: 10.1177/0269881111400646
22. Peña-Oliver Y, Sanchez-Roige S, Stephens DN, Ripley TL. Alpha-synuclein deletion decreases motor impulsivity but does not affect risky decision making in a mouse Gambling Task. Psychopharmacol (Berl). (2014) 231:2493–506. doi: 10.1007/s00213-013-3416-y
23. Pratt JA, Morris B, Dawson N. Deconstructing schizophrenia: advances in preclinical models for biomarker identification. Curr Top Behav Neurosci. (2018) 40:295–323. doi: 10.1007/978-3-319-99642-4
24. Bussey TJ, Muir JL, Robbins TW. A novel automated touchscreen procedure for assessing learning in the rat using computer graphic stimuli. Neurosci Res Commun. (1994) 15:103–10.
25. Bussey TJ, Holmes A, Lyon L, Mar AC, McAllister KAL, Nithianantharajah J, et al. New translational assays for preclinical modelling of cognition in schizophrenia: The touchscreen testing method for mice and rats. Neuropharmacology. (2012) 62:1191–203. doi: 10.1016/j.neuropharm.2011.04.011
26. Mar AC, Horner AE, Nilsson SR, Alsiö J, Kent BA, Kim CH, et al. The touchscreen operant platform for assessing executive function in rats and mice. Nat Protoc. (2013) 8:1985–2005. doi: 10.1038/nprot.2013.123
27. Horner AE, Heath CJ, Hvoslef-Eide M, Kent BA, Kim CH, Nilsson SRO, et al. The touchscreen operant platform for testing learning and memory in rats and mice. Nat Protoc. (2013) 8:1961–84. doi: 10.1038/nprot.2013.122
28. Lopez-Cruz L, Bussey TJ, Saksida LM, Heath CJ. Using touchscreen-delivered cognitive assessments to address the principles of the 3Rs in behavioral sciences. Lab Anim (NY). (2021) 50:174–84. doi: 10.1038/s41684-021-00791-2
29. Heath CJ, Bussey TJ, Saksida LM. Motivational assessment of mice using the touchscreen operant testing system: effects of dopaminergic drugs. Psychopharmacol (Berl). (2015) 232:4043–57. doi: 10.1007/s00213-015-4009-8
30. Joëls M, Baram TZ. The neuro-symphony of stress. Nat Rev Neurosci. (2009) 10:459–66. doi: 10.1038/nrn2632
31. Molins F, Serrano MÁ, Alacreu-Crespo A. Early stages of the acute physical stress response increase loss aversion and learning on decision making: A Bayesian approach. Physiol Behav. (2021) 237:113459. doi: 10.1016/j.physbeh.2021.113459
32. Georgiou P, Zanos P, Bhat S, Tracy JK, Merchenthaler IJ, McCarthy MM, et al. Dopamine and stress system modulation of sex differences in decision making. Neuropsychopharmacology. (2018) 43:313–24. doi: 10.1038/npp.2017.161
33. Lim J, Kim E, Noh HJ, Kang S, Phillips BU, Kim DG, et al. Assessment of mGluR5 KO mice under conditions of low stress using a rodent touchscreen apparatus reveals impaired behavioural flexibility driven by perseverative responses. Mol Brain. (2019) 12:37. doi: 10.1186/s13041-019-0441-8
34. Glover LR, Postle AF, Holmes A. Touchscreen-based assessment of risky-choice in mice. Behav Brain Res. (2020) 393:112748. doi: 10.1016/j.bbr.2020.112748
35. Passecker J, Mikus N, Malagon-Vina H, Anner P, Dimidschstein J, Fishell G, et al. Activity of prefrontal neurons predict future choices during gambling. Neuron. (2019) 101:152–164.e7. doi: 10.1016/j.neuron.2018.10.050
36. Cao B, Wang J, Zhang X, Yang X, Poon DC, Jelfs B, et al. Impairment of decision making and disruption of synchrony between basolateral amygdala and anterior cingulate cortex in the maternally separated rat. Neurobiol Learn Mem. (2016) 136:74–85. doi: 10.1016/j.nlm.2016.09.015
37. Bousseyrol E, Didienne S, Takillah S, Solié C, Come M, Ahmed Yahia T, et al. Dopaminergic and prefrontal dynamics co-determine mouse decisions in a spatial gambling task. Cell Rep. (2023) 42:112523. doi: 10.1016/j.celrep.2023.112523
38. Kwak MJ, Choi SJ, Cai WT, Cho BR, Han J, Park JW, et al. Manipulation of radixin phosphorylation in the nucleus accumbens core modulates risky choice behavior. Prog Neurobiol. (2024) 242:102681. doi: 10.1016/j.pneurobio.2024.102681
39. Openshaw RL, Thomson DM, Penninger JM, Pratt JA, Morris BJ. Mice haploinsufficient for Map2k7, a gene involved in neurodevelopment and risk for schizophrenia, show impaired attention, a vigilance decrement deficit and unstable cognitive processing in an attentional task: impact of minocycline. Psychopharmacol (Berl). (2017) 234:293–305. doi: 10.1007/s00213-016-4463-y
40. Bristow GC, Thomson DM, Openshaw RL, Mitchell EJ, Pratt JA, Dawson N, et al. 16p11 duplication disrupts hippocampal-orbitofrontal-amygdala connectivity, revealing a neural circuit endophenotype for schizophrenia. Cell Rep. (2020) 31:107536. doi: 10.1016/j.celrep.2020.107536
41. Openshaw RL, Thomson DM, Bristow GC, Mitchell EJ, Pratt JA, Morris BJ, et al. 16p11.2 deletion mice exhibit compromised fronto-temporal connectivity, GABAergic dysfunction, and enhanced attentional ability. Commun Biol. (2023) 6:557. doi: 10.1038/s42003-023-04891-2
42. Nithianantharajah J, McKechanie AG, Stewart TJ, Johnstone M, Blackwood DH, St Clair D, et al. Bridging the translational divide: identical cognitive touchscreen testing in mice and humans carrying mutations in a disease-relevant homologous gene. Sci Rep. (2015) 5:14613. doi: 10.1038/srep14613
43. Coba MP, Komiyama NH, Nithianantharajah J, Kopanitsa MV, Indersmitten T, Skene NG, et al. TNiK is required for postsynaptic and nuclear signaling pathways and cognitive function. J Neurosci. (2012) 32:13987–99. doi: 10.1523/JNEUROSCI.2433-12.2012
44. Kwak MJ, Kim WY, Jung SH, Chung YJ, Kim JH. Differential transcriptome profile underlying risky choice in a rat gambling task. J Behav Addict. (2022) 11:845–57. doi: 10.1556/2006.2022.00068
45. Dalley JW, Everitt BJ, Robbins TW. Impulsivity, compulsivity, and top-down cognitive control. Neuron. (2011) 69:680–94. doi: 10.1016/j.neuron.2011.01.020
46. Everitt BJ, Robbins TW. Neural systems of reinforcement for drug addiction: from actions to habits to compulsion. Nat Neurosci. (2005) 8:1481–9. doi: 10.1038/nn1579
47. Cho BR, Kwak MJ, Kim WY, Kim JH. Impulsive action and impulsive choice are differentially expressed in rats depending on the age at exposure to a gambling task. Front Psychiatry. (2018) 9:503. doi: 10.3389/fpsyt.2018.00503
48. Dalley JW, Robbins TW. Fractionating impulsivity: neuropsychiatric implications. Nat Rev Neurosci. (2017) 18:158–71. doi: 10.1038/nrn.2017.8
49. Zeeb FD, Winstanley CA. Functional disconnection of the orbitofrontal cortex and basolateral amygdala impairs acquisition of a rat gambling task and disrupts animals’ ability to alter decision-making behavior after reinforcer devaluation. J Neurosci. (2013) 33:6434–43. doi: 10.1523/JNEUROSCI.3971-12.2013
50. Piantadosi PT, Lieberman AG, Pickens CL, Bergstrom HC, Holmes A. A novel multichoice touchscreen paradigm for assessing cognitive flexibility in mice. Learn Mem. (2019) 26:24–30. doi: 10.1101/lm.048264.118
51. Logue SF, Gould TJ. The neural and genetic basis of executive function: attention, cognitive flexibility, and response inhibition. Pharmacol Biochem Behav. (2014) 123:45–54. doi: 10.1016/j.pbb.2013.08.007
52. Pokorny T, Duerler P, Seifritz E, Vollenweider FX, Preller KH. LSD acutely impairs working memory, executive functions, and cognitive flexibility, but not risk-based decision-making. psychol Med. (2020) 50:2255–64. doi: 10.1017/S0033291719002393
53. Perretta JG, Pari G, Beninger RJ. Effects of Parkinson disease on two putative nondeclarative learning tasks: probabilistic classification and gambling. Cognit Behav Neurol. (2005) 18:185–92. doi: 10.1097/01.wnn.0000187939.81541.1d
54. Mapelli D, Di Rosa E, Cavalletti M, Schiff S, Tamburin S. Decision and dopaminergic system: an ERPs study of Iowa gambling task in Parkinson’s disease. Front Psychol. (2014) 5:684. doi: 10.3389/fpsyg.2014.00684
55. Evens R, Hoefler M, Biber K, Lueken U. The Iowa Gambling Task in Parkinson’s disease: A meta-analysis on effects of disease and medication. Neuropsychologia. (2016) 91:163–72. doi: 10.1016/j.neuropsychologia.2016.07.032
56. Nigro SE, Wu M, Juliano AC, Flynn B, Lu LH, Landay AL, et al. Effects of cocaine and HIV on decision-making abilities. J Neurovirol. (2021) 27:422–33. doi: 10.1007/s13365-021-00965-1
57. Kjome KL, Lane SD, Schmitz JM, Green C, Ma L, Prasla I, et al. Relationship between impulsivity and decision making in cocaine dependence. Psychiatry Res. (2010) 178:299–304. doi: 10.1016/j.psychres.2009.11.024
58. MacQueen DA, Minassian A, Henry BL, Geyer MA, Young JW, Perry W. Amphetamine modestly improves conners’ Continuous performance test performance in healthy adults. J Int Neuropsychol Soc. (2018) 24:283–93. doi: 10.1017/S135561771700090X
59. Baarendse PJ, Winstanley CA, Vanderschuren LJ. Simultaneous blockade of dopamine and noradrenaline reuptake promotes disadvantageous decision making in a rat gambling task. Psychopharmacol (Berl). (2013) 225:719–31. doi: 10.1007/s00213-012-2857-z
60. Higgins GA, Brown M, MacMillan C, Silenieks LB, Thevarkunnel S. Contrasting effects of d-amphetamine and atomoxetine on measures of impulsive action and choice. Pharmacol Biochem Behav. (2021) 207:173220. doi: 10.1016/j.pbb.2021.173220
61. Silveira MM, Murch WS, Clark L, Winstanley CA. Chronic atomoxetine treatment during adolescence does not influence decision-making on a rodent gambling task, but does modulate amphetamine’s effect on impulsive action in adulthood. Behav Pharmacol. (2016) 27:350–63. doi: 10.1097/FBP.0000000000000203
62. van Enkhuizen J, Geyer MA, Young JW. Differential effects of dopamine transporter inhibitors in the rodent Iowa gambling task: relevance to mania. Psychopharmacol (Berl). (2013) 225:661–74. doi: 10.1007/s00213-012-2854-2
63. Benn A, Robinson ESJ. Development of a novel rodent rapid serial visual presentation task reveals dissociable effects of stimulant versus nonstimulant treatments on attentional processes. Cognit Affect Behav Neurosci. (2024) 24:351–67. doi: 10.3758/s13415-023-01152-x
64. Beyer DKE, Horn L, Klinker N, Freund N. Risky decision-making following prefrontal D1 receptor manipulation. Transl Neurosci. (2021) 12:432–43. doi: 10.1515/tnsci-2020-0187
65. Barrus MM, Winstanley CA. Dopamine D3 receptors modulate the ability of win-paired cues to increase risky choice in a rat gambling task. J Neurosci. (2016) 36:785–94. doi: 10.1523/JNEUROSCI.2225-15.2016
66. Wolfschlag M, Håkansson A. Increased risk for developing gambling disorder under the treatment with pramipexole, ropinirole, and aripiprazole: A nationwide register study in Sweden. PloS One. (2021) 16:e0252516. doi: 10.1371/journal.pone.0252516
67. Etminan M, Sodhi M, Samii A, Procyshyn RM, Guo M, Carleton BC. Risk of gambling disorder and impulse control disorder with aripiprazole, pramipexole, and ropinirole: A pharmacoepidemiologic study. J Clin Psychopharmacol. (2017) 37:102–4. doi: 10.1097/JCP.0000000000000634
68. Kapogiannis D, Mooshagian E, Campion P, Grafman J, Zimmermann TJ, Ladt KC, et al. Reward processing abnormalities in Parkinson’s disease. Mov Disord. (2011) 26:1451–7. doi: 10.1002/mds.23701
69. Burdick KE, Braga RJ, Gopin CB, Malhotra AK. Dopaminergic influences on emotional decision making in euthymic bipolar patients. Neuropsychopharmacology. (2014) 39:274–82. doi: 10.1038/npp.2013.177
70. Kubota H, Zhou X, Zhang X, Watanabe H, Nagai T. Pramipexole hyperactivates the external globus pallidus and impairs decision-making in a mouse model of parkinson’s disease. Int J Mol Sci. (2024) 25:8849. doi: 10.3390/ijms25168849
71. Aston-Jones G, Cohen JD. An integrative theory of locus coeruleus-norepinephrine function: adaptive gain and optimal performance. Annu Rev Neurosci. (2005) 28:403–50. doi: 10.1146/annurev.neuro.28.061604.135709
72. Yu AJ, Dayan P. Uncertainty, neuromodulation, and attention. Neuron. (2005) 46:681–92. doi: 10.1016/j.neuron.2005.04.026
73. Zhang X-L, Wang G-B, Zhao L-Y, Sun L-L, Wang J, Wu P, et al. Clonidine improved laboratory-measured decision-making performance in abstinent heroin addicts. PloS One. (2012) 7:e29084. doi: 10.1371/journal.pone.0029084
74. Browning S, Lawrence D, Livingston A, Morris B. Interactions of drugs active at opiate receptors and drugs active at alpha 2-receptors on various test systems. Br J Pharmacol. (1982) 77:487–91. doi: 10.1111/j.1476-5381.1982.tb09322.x
75. Higgins GA, Silenieks LB, MacMillan C, Zeeb FD, Thevarkunnel S. Effects of the NMDA receptor antagonists dizocilpine and Ro 63-1908 on delay-discounting and risky decision-making in a gambling task. Behav Brain Res. (2018) 348:201–10. doi: 10.1016/j.bbr.2018.04.028
76. Keshavan MS, Tandon R, Boutros NN, Nasrallah HA. Schizophrenia, “just the facts”: What we know in 2008: Part 3: Neurobiology. Schizophr Res. (2008) 106:89–107. doi: 10.1016/j.schres.2008.07.020
77. Harrison PJ. Recent genetic findings in schizophrenia and their therapeutic relevance. J Psychopharmacol. (2015) 29:85–96. doi: 10.1177/0269881114553647
78. Swerdlow NR. Integrative circuit models and their implications for the pathophysiologies and treatments of the schizophrenias. Curr Top Behav Neurosci. (2010) 4:555–83. doi: 10.1007/978-3-642-13717-4
79. Pratt JA, Morris BJ. The thalamic reticular nucleus: a functional hub for thalamocortical network dysfunction in schizophrenia and a target for drug discovery. J Psychopharmacol. (2015) 29:127–37. doi: 10.1177/0269881114565805
80. Pratt JA, Winchester CW, Egerton A, Cochran SM, Morris B. J, Modelling prefrontal cortex deficits in schizophrenia: implications for treatment. Br.J.Pharmacol. (2008) 153:S465–70. doi: 10.1038/bjp.2008.24
81. Covington HE III, Lobo MK, Maze I, Vialou V, Hyman JM, Zaman S, et al. Antidepressant effect of optogenetic stimulation of the medial prefrontal cortex. J Neurosci. (2010) 30:16082–90. doi: 10.1523/JNEUROSCI.1731-10.2010
82. Fettes PW, Moayedi M, Dunlop K, Mansouri F, Vila-Rodriguez F, Giacobbe P, et al. Abnormal functional connectivity of frontopolar subregions in treatment-nonresponsive major depressive disorder. Biol Psychiatry Cognit Neurosci Neuroimaging. (2018) 3:337–47. doi: 10.1016/j.bpsc.2017.12.003
83. Anticevic A, Cole MW, Repovs G, Murray JD, Brumbaugh MS, Winkler AM, et al. Characterizing thalamo-cortical disturbances in schizophrenia and bipolar illness. Cereb Cortex. (2013) 24:3116–30. doi: 10.1093/cercor/bht165
84. Massalha Y, Maggioni E, Callari A, Brambilla P, Delvecchio G. A review of resting-state fMRI correlations with executive functions and social cognition in bipolar disorder. J Affect Disord. (2023) 334:337–51. doi: 10.1016/j.jad.2023.03.084
85. Lu J, Zhao X, Wei X, He G. Risky decision-making in major depressive disorder: A three-level meta-analysis. Int J Clin Health Psychol. (2024) 24:100417. doi: 10.1016/j.ijchp.2023.100417
86. van Enkhuizen J, Henry BL, Minassian A, Perry W, Milienne-Petiot M, Higa KK, et al. Reduced dopamine transporter functioning induces high-reward risk-preference consistent with bipolar disorder. Neuropsychopharmacology. (2014) 39:3112–22. doi: 10.1038/npp.2014.170
87. Powers RL, Russo M, Mahon K, Brand J, Braga RJ, Malhotra AK, et al. Impulsivity in bipolar disorder: relationships with neurocognitive dysfunction and substance use history. Bipolar Disord. (2013) 15:876–84. doi: 10.1111/bdi.2013.15.issue-8
88. Brambilla P, Perlini C, Bellani M, Tomelleri L, Ferro A, Cerruti S, et al. Increased salience of gains versus decreased associative learning differentiate bipolar disorder from schizophrenia during incentive decision making. psychol Med. (2013) 43:571–80. doi: 10.1017/S0033291712001304
89. Gu YT, Zhou C, Yang J, Zhang Q, Zhu GH, Sun L, et al. A transdiagnostic comparison of affective decision-making in patients with schizophrenia, major depressive disorder, or bipolar disorder. Psych J. (2020) 9:199–209. doi: 10.1002/pchj.v9.2
90. Cella M, Dymond S, Cooper A. Impaired flexible decision-making in Major Depressive Disorder. J Affect Disord. (2010) 124:207–10. doi: 10.1016/j.jad.2009.11.013
91. Rinaldi R, Lefebvre L, Joachim A, Rossignol M. Decision-making of patients with major depressive disorder in the framework of action control. Cognit Neuropsychiatry. (2020) 25:71–83. doi: 10.1080/13546805.2019.1685481
92. Adida M, Jollant F, Clark L, Besnier N, Guillaume S, Kaladjian A, et al. Trait-related decision-making impairment in the three phases of bipolar disorder. Biol Psychiatry. (2011) 70:357–65. doi: 10.1016/j.biopsych.2011.01.018
93. Kester HM, Sevy S, Yechiam E, Burdick KE, Cervellione KL, Kumra S. Decision-making impairments in adolescents with early-onset schizophrenia. Schizophr Res. (2006) 85:113–23. doi: 10.1016/j.schres.2006.02.028
94. Fond G, Bayard S, Capdevielle D, Del-Monte J, Mimoun N, Macgregor A, et al. A further evaluation of decision-making under risk and under ambiguity in schizophrenia. Eur Arch Psychiatry Clin Neurosci. (2013) 263:249–57. doi: 10.1007/s00406-012-0330-y
95. Cella M, Dymond S, Cooper A, Turnbull OH. Cognitive decision modelling of emotion-based learning impairment in schizophrenia: the role of awareness. Psychiatry Res. (2012) 196:15–9. doi: 10.1016/j.psychres.2011.08.015
96. Sevy S, Burdick KE, Visweswaraiah H, Abdelmessih S, Lukin M, Yechiam E, et al. Iowa gambling task in schizophrenia: a review and new data in patients with schizophrenia and co-occurring cannabis use disorders. Schizophr Res. (2007) 92:74–84. doi: 10.1016/j.schres.2007.01.005
97. Shurman B, Horan WP, Nuechterlein KH. Schizophrenia patients demonstrate a distinctive pattern of decision-making impairment on the Iowa Gambling Task. Schizophr Res. (2005) 72:215–24. doi: 10.1016/j.schres.2004.03.020
98. Pedersen A, Göder R, Tomczyk S, Ohrmann P. Risky decision-making under risk in schizophrenia: A deliberate choice? J Behav Ther Exp Psychiatry. (2017) 56:57–64. doi: 10.1016/j.jbtep.2016.08.004
99. Woodrow A, Sparks S, Bobrovskaia V, Paterson C, Murphy P, Hutton P. Decision-making ability in psychosis: a systematic review and meta-analysis of the magnitude, specificity and correlates of impaired performance on the Iowa and Cambridge Gambling Tasks. Psychol Med. (2019) 49:32–48. doi: 10.1017/S0033291718002660
100. Betz LT, Brambilla P, Ilankovic A, Premkumar P, Kim MS, Raffard S, et al. Deciphering reward-based decision-making in schizophrenia: A meta-analysis and behavioral modeling of the Iowa Gambling Task. Schizophr Res. (2019) 204:7–15. doi: 10.1016/j.schres.2018.09.009
101. Kim YT, Lee KU, Lee SJ. Deficit in decision-making in chronic, stable schizophrenia: from a reward and punishment perspective. Psychiatry Investig. (2009) 6:26–33. doi: 10.4306/pi.2009.6.1.26
102. Kim MS, Kang BN, Lim JY. Decision-making deficits in patients with chronic schizophrenia: Iowa Gambling Task and Prospect Valence Learning model. Neuropsychiatr Dis Treat. (2016) 12:1019–27. doi: 10.2147/NDT.S103821
103. Turnbull OH, Evans CEY, Kemish K, Park S, Bowman CH. A novel set-shifting modification of the iowa gambling task: flexible emotion-based learning in schizophrenia. Neuropsychology. (2006) 20:290–8. doi: 10.1037/0894-4105.20.3.290
104. Saperia S, Da Silva S, Siddiqui I, Agid O, Daskalakis ZJ, Ravindran A, et al. Reward-driven decision-making impairments in schizophrenia. Schizophr Res. (2019) 206:277–83. doi: 10.1016/j.schres.2018.11.004
105. Must A, Horvath S, Nemeth VL, Janka Z. The Iowa Gambling Task in depression - what have we learned about sub-optimal decision-making strategies? Front Psychol. (2013) 4:732. doi: 10.3389/fpsyg.2013.00732
106. Brown EC, Hack SM, Gold JM, Carpenter WT Jr., Fischer BA, Prentice KP, et al. Integrating frequency and magnitude information in decision-making in schizophrenia: An account of patient performance on the Iowa Gambling Task. J Psychiatr Res. (2015) 66-67:16–23. doi: 10.1016/j.jpsychires.2015.04.007
107. Achim AM, Maziade M, Raymond E, Olivier D, Marette C, Roy M-A. How prevalent are anxiety disorders in schizophrenia? A meta-analysis and critical review on a significant association. Schizophr Bull. (2011) 37:811–21. doi: 10.1093/schbul/sbp148
108. Hall J. Schizophrenia — an anxiety disorder? Br J Psychiatry. (2017) 211:262–3. doi: 10.1192/bjp.bp.116.195370
109. Bagneux V, Font H, Bollon T. Incidental emotions associated with uncertainty appraisals impair decisions in the Iowa Gambling Task. Motivation Emotion. (2013) 37:818–27. doi: 10.1007/s11031-013-9346-5
110. Zhang L, Wang K, Zhu C, Yu F, Chen X. Trait Anxiety Has Effect on Decision Making under Ambiguity but Not Decision Making under Risk. PloS One. (2015) 10:e0127189. doi: 10.1371/journal.pone.0127189
111. Cella M, Dymond S, Cooper A. Impairment in flexible emotion-based learning in hallucination- and delusion-prone individuals. Psychiatry Res. (2009) 170:70–4. doi: 10.1016/j.psychres.2008.07.001
112. Ehrlich AT, Semache M, Bailly J, Wojcik S, Arefin TM, Colley C, et al. Mapping GPR88-Venus illuminates a novel role for GPR88 in sensory processing. Brain Struct Funct. (2018) 223:1275–96. doi: 10.1007/s00429-017-1547-3
113. Ingallinesi M, Galet B, Pegon J, Faucon Biguet N, Do Thi A, Millan MJ, et al. Knock-down of GPR88 in the dorsal striatum alters the response of medium spiny neurons to the loss of dopamine input and L-3-4-dyhydroxyphenylalanine. Front Pharmacol. (2019) 10:1233. doi: 10.3389/fphar.2019.01233
114. Mantas I, Yang Y, Mannoury-la-Cour C, Millan MJ, Zhang X, Svenningsson P. Genetic deletion of GPR88 enhances the locomotor response to L-DOPA in experimental parkinsonism while counteracting the induction of dyskinesia. Neuropharmacology. (2020) 162:107829. doi: 10.1016/j.neuropharm.2019.107829
115. Quintana A, Sanz E, Wang W, Storey GP, Guler AD, Wanat MJ, et al. Lack of GPR88 enhances medium spiny neuron activity and alters motor- and cue-dependent behaviors. Nat Neurosci. (2012) 15:1547–55. doi: 10.1038/nn.3239
116. Ben Hamida S, Sengupta SM, Clarke E, McNicholas M, Moroncini E, Darcq E, et al. The orphan receptor GPR88 controls impulsivity and is a risk factor for Attention-Deficit/Hyperactivity Disorder. Mol Psychiatry. (2022) 27:4662–72. doi: 10.1038/s41380-022-01738-w
117. Winchester CL, Ohzeki H, Vouyiouklis DA, Thompson R, Penninger JM, Yamagami K, et al. Converging evidence that sequence variations in the novel candidate gene MAP2K7 (MKK7) are functionally associated with schizophrenia. Hum Mol Genet. (2012) 21:4910–21. doi: 10.1093/hmg/dds331
118. Openshaw RL, Thomson DM, Thompson R, Penninger JM, Pratt JA, Morris BJ, et al. Map2k7 haploinsufficiency induces brain imaging endophenotypes and behavioral phenotypes relevant to schizophrenia. Schizophr Bull. (2020) 46:211–23. doi: 10.1093/schbul/sbz044
Keywords: gambling, touchscreen, dopamine, impulsivity, risk-reward
Citation: Pratt JA and Morris BJ (2025) Maximising translational value of the Iowa gambling task in preclinical studies through the use of the rodent touchscreen. Front. Psychiatry 16:1518435. doi: 10.3389/fpsyt.2025.1518435
Received: 28 October 2024; Accepted: 09 January 2025;
Published: 27 January 2025.
Edited by:
Vicki A. Nejtek, University of North Texas Health Science Center, United StatesReviewed by:
Michael F. Salvatore, University of North Texas Health Science Center, United StatesCopyright © 2025 Pratt and Morris. This is an open-access article distributed under the terms of the Creative Commons Attribution License (CC BY). The use, distribution or reproduction in other forums is permitted, provided the original author(s) and the copyright owner(s) are credited and that the original publication in this journal is cited, in accordance with accepted academic practice. No use, distribution or reproduction is permitted which does not comply with these terms.
*Correspondence: Brian J. Morris, YnJpYW4ubW9ycmlzQGdsYXNnb3cuYWMudWs=
Disclaimer: All claims expressed in this article are solely those of the authors and do not necessarily represent those of their affiliated organizations, or those of the publisher, the editors and the reviewers. Any product that may be evaluated in this article or claim that may be made by its manufacturer is not guaranteed or endorsed by the publisher.
Research integrity at Frontiers
Learn more about the work of our research integrity team to safeguard the quality of each article we publish.