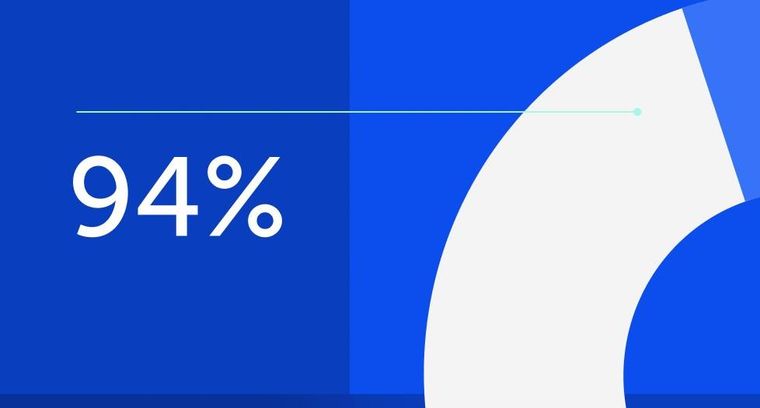
94% of researchers rate our articles as excellent or good
Learn more about the work of our research integrity team to safeguard the quality of each article we publish.
Find out more
ORIGINAL RESEARCH article
Front. Psychiatry, 17 February 2025
Sec. Mood Disorders
Volume 16 - 2025 | https://doi.org/10.3389/fpsyt.2025.1511397
Introduction: The aim of this study is to investigate whether arginine and its metabolites can be an endophenotype for bipolar disorder (BD) and to evaluate the role of arginine metabolites and neurocognitive function levels in unaffected healthy children of parents diagnosed with BD in cognitive impairment.
Methods: The study included 37 healthy children of parents diagnosed with BD Type I as the high-risk group and 36 healthy children of parents without any psychiatric disorders as the control group. The arginine, n-monomethyl-l-arginine acetate (L-NMMA), asymmetric dimethylarginine (ADMA), symmetric dimethylarginine (SDMA), citrulline, homoarginine, ornithine serum levels, and nitric oxide synthase (NOS) activity level of both groups were compared.
Results: The study found that in the high-risk group, ADMA, SDMA, and ornithine levels were significantly higher compared to the controls, while citrulline and NOS activity level were significantly lower in the high-risk group compared to the controls. All neurocognitive performances of the high-risk group were considered statistically significantly worse compared to controls. The impairment in neurocognitive functions in the high-risk group was found to be correlated with ADMA, L-NMMA, citrulline, homoarginine, ornithine levels, and NOS activity level.
Discussion: These findings highlight a potential link between arginine metabolism and executive dysfunction in individuals at high risk for BD. Further longitudinal studies are essential to fully understand the complex interactions between these factors.
Bipolar disorder (BD) is a chronic and severe psychiatric condition characterized by recurrent episodes of mania, hypomania, and depression, which significantly impact daily functioning. BD is known to have a strong genetic component, with heritability estimates suggesting that up to 85% of the variability in risk is attributable to genetic factors (1, 2). Beyond mood disturbances, individuals with BD often exhibit various neurocognitive deficits, including impairments in executive function, working memory, and attention. Notably, such cognitive deficits are also observed in the unaffected first-degree relatives of BD patients, which suggests these impairments may serve as potential endophenotypes that reflect underlying genetic vulnerability to the disorder (3).
The etiology of BD is multifactorial, involving a complex interaction of genetic, biochemical, environmental, and psychosocial factors. Recent research has highlighted the potential role of arginine and its metabolites in the pathophysiology of BD, particularly in relation to neurocognitive impairments (4). Elevated levels of methylarginines, especially asymmetric dimethylarginine (ADMA), have been observed in individuals with BD, and higher concentrations of ADMA have been linked to poorer performance on cognitive tasks involving executive function and attention (5). These findings suggest that disruptions in arginine metabolism may contribute to the cognitive deficits associated with BD. Available clinical evidence has demonstrated that the nitric oxide (NO) signaling pathway is associated with schizophrenia, anxiety disorders, and affective disorders (6). NO a key signaling molecule synthesized from arginine, has garnered significant interest in the context of BD. NO is an important messenger in brain signaling and influences the balance ofmonoaminergic and glutamatergic neurotransmission. NO is involved in various neural processes, including synaptic plasticity, neuroinflammation, neurotransmission, and the regulation of learning and memory. Disruptions in NO production and associated pathways have been implicated in the pathophysiology of BD (7). A meta-analysis has demonstrated that NO levels change in individuals with BD (8). Arginine is a semi-essential amino acid and a substrate of important metabolic pathways in the physiological processes of the central nervous system and immune defense (9, 10). Arginine is transformed into NO and citrulline by endothelial nitric oxide synthase (eNOS), inducible nitric oxide synthase (iNOS) and neuronal nitric oxide synthase (nNOS) (11). Arginine, the precursor molecule for NO synthesis, plays a crucial role in maintaining NO levels in the brain. In BD, abnormalities in arginine metabolism may lead to altered NO signaling, contributing to both mood dysregulation and cognitive deficits. Additionally, interactions between the arginine-vasopressin pathway and NO synthesis are thought to influence mood regulation and symptom expression in BD. Arginine plays a crucial role in neuroinflammatory processes, particularly through its involvement in microglial activation. During this process, arginine serves as a substrate for the production of NO and reactive nitrogen species (RNS). The dysregulation of NO production significantly influences the progression of neuroinflammation. While excessive NO levels induce neurotoxicity by triggering the release of pro-inflammatory cytokines such as interleukin-6, tumor necrosis factor-alpha, and interleukin-1 beta, insufficient NO production can also have profound consequences. These cytokines disrupt synaptic function, exacerbate glutamate-mediated excitotoxicity, and increase blood-brain barrier permeability, thereby facilitating the infiltration of peripheral immune cells into the central nervous system and amplifying inflammation (12, 13). Conversely, low NO levels also play a pivotal role in neuroinflammation. Under physiological conditions, NO is essential for neuronal protection and the regulation of immune responses. However, reduced NO production can lead to an imbalance in microglial activity and impair resolution mechanisms necessary for controlling inflammation. The deficiency of NO has been associated with an increased accumulation of reactive oxygen species, thereby exacerbating oxidative stress and contributing to the persistence of neuroinflammatory processes (14, 15). Arginine metabolism generates key biomarkers that provide insights into these mechanisms. NO synthesis via iNOS has been shown to exert both protective and detrimental effects in the context of neuroinflammation. Notably, insufficient NO levels may impair anti-inflammatory responses and weaken cellular defense mechanisms. Arginase competes with NOS for arginine, converting it into ornithine and polyamines. Elevated arginase activity reduces NO production while promoting polyamine accumulation, a process linked to oxidative stress and inflammatory responses (16). Methylarginines, including ADMA and symmetric dimethylarginine (SDMA), are formed through the degradation of proteins containing arginine residues (see Figure 1). These molecules play important roles in regulating NO synthesis and cellular signaling pathways. ADMA, in particular, inhibits nitric oxide synthase, thereby reducing NO production. Elevated levels of ADMA have been associated with increased oxidative stress and neuroinflammation, which are believed to contribute to cognitive impairment in BD. Although the precise mechanisms through which methylarginines influence neurocognitive function remain unclear, their role in modulating NO levels, oxidative stress, and inflammation suggests they may be key contributors to the cognitive deficits seen in BD (17).
Figure 1. Metabolism of arginine and metabolites (18). PRMT’s, protein-arginine methyl transferase L-NMMA, n-monomethyl-l-argınıne acetate; ADMA, Asymmetric dimethylarginine; SDMA, Symmetric Dimethylarginine; NO, Nitric Oxide.
Patients with bipolar disorder frequently show cognitive dysfunction even during euthymia (3, 19). Areas of cognitive dysfunction in bipolar disorder include attention, language learning, memory and executive function (20, 21). Neurocognitive impairments have also been observed in the offspring of individuals with BD. Studies have demonstrated that children of BD patients tend to have lower intelligence quotients (IQ) compared to controls (22). Additionally, these children exhibit poorer cognitive performance on tasks involving processing speed and visual memory (23). It has been proposed that these cognitive deficits in the healthy offspring of BD patients may be related to disruptions in arginine metabolism, though this hypothesis has yet to be fully explored.
To date, no studies have specifically examined the relationship between arginine metabolites and executive function in the healthy offspring of BD patients. Given the role of arginine and its metabolites in NO regulation, oxidative stress, and neuroinflammation, it is plausible that abnormal arginine metabolism could contribute to the cognitive impairments observed in this at-risk population. Therefore, the present study aims to investigate the association between arginine metabolite levels and executive function in the healthy offspring of individuals with BD. Furthermore, we seek to determine whether elevated levels of methylarginines, such as ADMA and SDMA, are linked to cognitive impairments, and whether these molecules may serve as potential endophenotypes for BD. By elucidating the biochemical underpinnings of cognitive deficits in this population, this study may provide valuable insights into the biological factors that predispose individuals to BD and its associated cognitive challenges.
This study was conducted in collaboration with the Child and Adolescent Psychiatry Department, the Psychiatry Department, and the Medical Biochemistry Department at Selçuk University Faculty of Medicine Hospital. Consent was obtained from both the participating children/adolescents and their parents to voluntarily partake in the study. The sample for the study included 37 children and adolescents, aged 8-17, who had a parent diagnosed with bipolar I disorder according to DSM-5 (The Diagnostic and Statistical Manual of Mental Disorders) criteria following psychiatric interviews at the Psychiatry Clinic between June 2022 and December 2022, and who met the inclusion and exclusion criteria.
The control group consisted of 36 healthy volunteers, aged 8-17, who attended the Child and Adolescent Psychiatry Clinic at Selçuk University Faculty of Medicine Hospital for psychiatric consultation, and who did not have any psychiatric or other medical disorders. These controls were matched with the high-risk group based on age and sex and participated on a voluntary basis.
For all participants, medical history was taken to assess whether they met the inclusion and exclusion criteria. The Sociodemographic Data Questionnaire and Schedule for Affective Disorders and Schizophrenia for School Age Children-Present and Lifetime Version-Turkish Version (K-SADS-PL-DSM-5-T), stroop test, serial digit learning test (SDLT), and cancellation test (CT) were applied to the high-risk and control groups. Serum levels of arginine, N-monomethyl-L-arginine acetate (L-NMMA), ADMA, SDMA, citrulline, homoarginine, and ornithine, as well as the citrulline/arginine ratio reflecting NOS activity, were compared between the high-risk and control groups.
The high-risk group consisted of children aged 8-17 who had at least one parent diagnosed with bipolar I disorder, who did not have any psychiatric disorder according to the K-SADS-PL-DSM-5-T administered by a clinician, and who consented to participate voluntarily along with their parents.
Exclusion Criteria for High-Risk Group:
1. Inadequate cognitive functions or intellectual levels to comprehend the tests.
2. Presence of other medical inflammatory disorders or psychiatric disorders.
3. History of alcohol or substance use.
4. Use of any medications that could affect cognitive functions and TRP metabolite levels.
The control group consisted of children aged 8-17 who did not have any psychiatric disorder according to the K-SADS-PL-DSM-5-T administered by a clinician and who consented to participate voluntarily along with their parents.
The study reached out to 242 patients diagnosed with bipolar disorder according to DSM-5 criteria through psychiatric interviews and follow-ups at the Psychiatry Department of Selçuk University Faculty of Medicine. Fifty patients were excluded because they did not have bipolar I disorder, and 108 were excluded because they did not have children aged 8-17. The remaining 84 parents, with a total of 94 children, were evaluated for participation. However, 17 children were excluded due to missing contact information, 16 parents declined participation, 13 children had other medical conditions, and 11 children were diagnosed with a psychiatric disorder according to the K-SADS-PL-DSM-5-T. Ultimately, the study group consisted of 37 children.
The Sociodemographic Data Form is used to collect information about the age, educational status, ages of parents and family socioeconomic status (SES) of the children and adolescents participating in the study.
This semi-structured interview schedule is designed to assess past and current psychopathologies based on DSM-5 diagnostic criteria. The Turkish adaptation, which incorporates both dimensional and categorical diagnostic assessments aligned with DSM-5, was validated and deemed reliable in 2018. The interview schedule is systematically structured into three primary sections. The first section involves an unstructured interview conducted with the child and their family, aimed at gathering sociodemographic information, presenting complaints, developmental history, and an assessment of the child’s overall functioning at school and home. The second section comprises screening questions designed to evaluate over 200 specific symptoms, covering both past experiences and recent occurrences (within the last two months). Finally, the third section integrates assessment results with clinician observations to confirm diagnoses based on DSM-5 criteria. The K-SADS-PL-DSM-5-T facilitates the systematic screening of a wide range of primary psychiatric disorders. These encompass mood disorders, psychotic disorders, anxiety disorders, obsessive-compulsive disorder, neurodevelopmental and behavioral disorders, elimination disorders, eating disorders, tic disorders, substance-related disorders, as well as trauma- and stressor-related conditions (24).
The Turkish validity and reliability study for the Stroop Test TBAG form was conducted in 1999 (25). This test, used in the study, is a combination of the original Stroop Test and the Victoria Form. The Stroop Test TBAG Form is considered the gold standard for attention measurement. The Stroop Test is a neuropsychological assessment tool primarily designed to evaluate concentration and selective attention, while also providing insights into frontal region activity. Among neuropsychological tests, the Stroop Test is considered one of the most effective for assessing the ability to resist interfering stimuli, suppress inappropriate responses, shift targets in accordance with changing demands, and process information rapidly.The Stroop Test TBAG Form comprises five sections, each evaluated based on three parameters: duration, number of corrections, and number of errors. Duration reflects reaction time and the speed of information processing, corrections indicate impulsivity, and errors signify inattention. Prolonged durations and an increased number of errors and corrections are indicative of cognitive impairments, particularly in selective attention and information processing speed (26).
Developed by Zangwill, this test is used to evaluate short-term memory and learning ability. The Turkish validity and reliability studies for the SDLT were conducted in 1996 (27). The brain regions implicated in this test correspond to the mesial temporal lobe, frontal lobe, and hippocampus. SDLT has two variants: the 8-item and 9-item forms. During the test, the practitioner presents a sequence of numbers, and the subject is required to repeat the sequence in the same order. The test is concluded either when the subject successfully repeats the sequence twice consecutively or when all 12 attempts are exhausted. Performing this task, which involves learning a specific number and its spatial location, necessitates the engagement of various cognitive processes, including attention, information processing, memory, organization, and association (28). In this study, the 8-item form of the SDLT was utilized, emphasizing its application in evaluating these cognitive domains.
The CT consists of four subtests, which include both regular and irregular distributions of letters and shapes. These subtests are referred to as Regular Letters, Irregular Letters, Regular Shapes, and Irregular Shapes. Each subtest contains 60 target stimuli embedded within a total of 300 stimuli. In this test, participants are tasked with identifying the target stimuli and marking them by circling. Separate scores are calculated for each subtest: number of correctly marked targets (CT1), number of missed targets (CT2), number of incorrectly marked letters/shapes (CT3), total number of errors (CT4), and scanning time (CT5). The total error score is derived from the sum of missed target scores and incorrectly marked letter/shape scores. Developed by Weintraub and Mesulam in 1985, the Turkish version of the CT is one of seven separate neuropsychological tests used in the Cognitive Potentials’ Neuropsychological Test (BILNOT) Battery in Turkey (29). Although the test primarily evaluates selective attention and attention maintenance skills, it also enables the assessment of executive functions, including visual scanning, reaction speed, visual-motor coordination, retention of targets in memory, learning, strategy use, sequencing of planned responses, and maintaining behavioral set-up (30).
Blood samples were collected in a laboratory environment into gel vacutainer biochemistry tubes after at least 8 hours of fasting, in the morning. Blood samples from both the high-risk and control groups were centrifuged at 3000 rpm for 10 minutes. Plasma samples were collected into Eppendorf tubes and stored at -80°C until further processing. For deproteinization, 100 microliters of serum sample was mixed with 100 microliters of trichloroacetic acid containing an internal standard. The mixture was vortexed at 14,000 rpm for 5 minutes. Subsequently, 50 microliters of the supernatant was taken and used for analysis. The analyses were performed using the liquid chromatography-mass spectrometry (LC-MS/MS) equipment available within our hospital. Chromatographic separation was performed on a reverse-phase C18 column under gradient elution. The mobile phases consisted of 0.1% formic acid in water and 0.1% formic acid in acetonitrile. Mass spectrometric detection was conducted in positive ion mode using Multiple Reaction Monitoring. Analyte concentrations were calculated based on calibration curves. The method was validated for linearity, precision, accuracy, and sensitivity according to international standards.
All data were evaluated using the SPSS-22 statistical program. Number, percentage, mean, and standard deviation were used in the evaluation of the data. The chi-square test was applied to two groups for categorical data. For numerical data, skewness and kurtosis values between -2 and +2 were used to comply with the normal distribution. When comparing the plasma tryptophan metabolites levels and neurocognitive properties of the high-risk and control groups, the student’s t-test was used for parameters with normal distribution, and the Mann–Whitney U test was applied for those with non-normal distribution. For all analyses, a significance value was accepted of p<0.05 at the 95% confidence interval. In addition, while examining the correlation and association between the cognitive characteristics of the high-risk group and plasma tryptophan metabolites, the data that fit the normal distribution were evaluated using Pearson’s correlation test, while the data that did not fit the normal distribution were evaluated with Spearman’s correlation analysis. Multivariate analyzes were planned to avoid type II errors due to multiple variables.
Socioeconomic status, BMI, age and sex were taken as covariates and blood levels of the groups were compared using MANCOVA. In addition, significant results from the cognitive tests were incorporated into the MANCOVA analysis. The Stroop 2nd Section Duration scores underwent square root transformation to meet the assumptions of parametric testing. However, CT2-FT scores were excluded from the MANCOVA analysis as they could not be normalized through any transformation. After observing a significant difference between the two groups with the MANCOVA test, one-way analysis of covariance (ANCOVA) was performed on the outcome variables separately. Effect sizes were estimated using Cohen’s d for parametric and non-parametric comparisons. Cohen’s d effect sizes were considered as ≥0.8 large, 0.5-0.7 intermediate, 0.2-0.4 small and <0.2 no effect (31).
The study included a total of 73 children and adolescents, comprising 37 unaffected high-risk group (17 boys, 20 girls) with a parent diagnosed with bipolar I disorder, and 36 healthy controls (23 boys, 13 girls). When the high-risk and control groups were compared in terms of age (t=-1.087, p=0.281), body mass index (t=-0.520, p=0.605), sex (x2 = 2,372, p=0.124) and SES (x2 = 3,154, p=0,207) no statistically significant differences were found between the two groups (see Table 1).
When the two groups were compared in terms of Stroop test performance, it was found that only the completion time for the second part (z=-2.146, p=0.032) was statistically significantly higher in the high-risk group. Comparing the two groups in terms of SDLT performance, it was found that SDLT scores (t=2.05, p=0.045) had a statistically significant difference. The high-risk group had significantly lower SDLT scores compared to the controls. When the results of the CT were compared between the two groups, it was found that only the number of incorrectly marked targets in the regular shape (z=-2.298, p=0.022) was statistically significantly higher in the high-risk group (see Table 2).
In the comparison of arginine and its metabolites between the high-risk group and the control group, levels of ADMA, SDMA, and ornithine were significantly higher (t=-2.118, p=0.038; t=-2.858, p=0.006; t=-4.636, p<0.001, respectively) in the high-risk group compared to the controls. The citrulline level and the citrulline/arginine ratio, which indirectly reflect NOS activity, were found to be significantly lower in the high-risk group compared to the control group (t=8.54, p<0.001; z=-6.83, p<0.001) (see Table 3).
In the correlation analysis between Stroop test performance and arginine metabolites, a significant association was identified between the number of errors in the fifth part of the Stroop test and ADMA levels (rho = 0.369, p = 0.025). Furthermore, significant correlations were found between the durations of the first and second parts of the Stroop test and homoarginine levels (rho = 0.344, p = 0.037; rho = 0.408, p = 0.012, respectively). However, no significant relationships were observed between SDLT performance and arginine metabolites.
In the correlation analysis between CT data and arginine metabolites, several significant relationships were detected. L-NMMA levels were significantly correlated with the number of marked targets in the irregular letter section (r = -0.359, p = 0.029), the number of missed targets (r = 0.370, p = 0.024), and the total number of errors (r = 0.343, p = 0.038). Citrulline levels were associated with the number of marked targets in the irregular letter section (r = 0.358, p = 0.029), missed targets (r = -0.357, p = 0.03), total number of errors (r = -0.341, p = 0.039), and the total time for the irregular shape section (rho = 0.359, p = 0.029). Ornithine levels were significantly correlated with the number of incorrectly marked targets in the irregular letter section (rho = -0.336, p = 0.042), and NOS activity levels were associated with the number of missed targets in the irregular letter section (rho = -0.325, p = 0.05).
To minimize Type II errors resulting from multiple testing and to control for confounding factors such as sex, BMI, SES, and age, a MANCOVA test was performed. The MANCOVA test indicated a significant difference between groups (Pillai’s trace V = 0.773, F (9,59) = 22.272, p < 0,001, ηp² = 0.773). After observing a significant difference between the two groups with the MANCOVA test, one-way analysis of covariance (ANCOVA) was performed on the outcome variables separately. The same variables were taken as covariates in the ANCOVA analysis to determine which variables caused the differences between the groups. After controlling for SES, sex, age, and BMI, the comparison of SDLT, SD2, and the levels of arginine and its metabolites is presented in Table 4.
Table 4. Comparison of SDLT, Stroop test, Arginine and Metabolite levels in both groups according to ANCOVA.
The objective of this study was to explore the alterations in arginine and its metabolites in unaffected offspring of individuals diagnosed with BD compared to a control group. Additionally, we aimed to evaluate cognitive functions in these children and examine the potential relationships between these cognitive functions and the levels of arginine metabolites. Specifically, serum concentrations of arginine, L-NMMA, ADMA, SDMA, citrulline, homoarginine, ornithine, and NOS activity were measured in the high-risk offspring of BD patients and compared to those of the control group. Our findings indicated that the high-risk group exhibited significantly elevated levels of ADMA, SDMA, and ornithine, while citrulline levels and the citrulline/arginine ratio—an indirect measure of NOS activity—were significantly reduced in comparison to the control group. Moreover, the high-risk group demonstrated notable impairments in executive functions, which were correlated with elevated levels of ADMA, L-NMMA, citrulline, homoarginine, ornithine, and decreased NOS activity. These results suggest that arginine and its metabolites may play a contributory role in the underlying pathophysiology of BD.
MANCOVA analysis demonstrated that when SES, BMI, age, and sex were controlled as covariates, the significance of the cognitive data and arginine metabolites obtained in our study was largely preserved. Specifically, statistical significance was maintained for all metabolites and cognitive tests, except for ADMA. These results underscore the homogeneity of our study group and the reliability of the analyses, independent of these factors.
Moreover, previous studies have reported associations between SES, sex, BMI, and age with cognitive performance and metabolic processes (32–36). However, our findings highlight that the independent effects of arginine metabolism and cognitive functions remain evident even when these covariates are controlled. This suggests that arginine metabolism and cognitive processes may play distinct roles, and a homogeneous study group enables more robust and reliable analyses. However, which limits the ability to generalize across diverse populations. Future studies should aim to include larger and more diverse cohorts to facilitate subgroup analyses across SES, age, sex, and other relevant factors. Such analyses would provide a deeper understanding of how these variables interact with arginine metabolism and cognitive outcomes, thereby offering broader insights into their roles in bipolar disorder risk and progression.
Recent investigations into the role of arginine and related metabolic pathways in BD have focused on their influence on neurotransmitter regulation, neurovascular functioning, and cognitive processes (37). Nitrosative stress, resulting from an imbalance between RNS and antioxidant defenses, is a key factor in the pathophysiology of BD. Elevated RNS levels, such as peroxynitrite, cause oxidative damage to lipids, proteins, and DNA, leading to neuronal dysfunction, neuroinflammation, and synaptic impairment, which exacerbate mood and cognitive deficits in BD (5). ADMA contributes to nitrosative stress by reducing NO bioavailability. Increased ADMA, as observed in our study, disrupts endothelial function, neurovascular coupling, synaptic plasticity, and neurotransmission, further impairing mood regulation and cognition. Additionally, reduced NO levels, a critical regulator of neurovascular homeostasis and neurotransmitter systems, exacerbate neuroinflammation and neuronal dysfunction (8). The observed reductions in citrulline levels and NOS activity in the high-risk group support the role of impaired NO signaling in BD. The combined effects of elevated nitrosative stress and diminished NO levels create a pathological cycle that underlies BD’s clinical and cognitive symptoms. Targeting these pathways may offer promising therapeutic strategies for BD prevention and treatment. Elevated serum arginine levels have been reported in some studies, highlighting potential dysregulation in the urea cycle and arginine metabolism in BD patients (38). In addition elevated ADMA levels have been documented not only in BD patients but also in individuals with other psychiatric disorders (5). In parallel, increased levels of SDMA, ADMA, and arginine have been observed in individuals with BD and schizophrenia (39). L-NMMA, another inhibitor of NO synthesis, may modulate neurocognitive function through its effects on NO pathways, and thus could play a significant role in BD (17). Citrulline, which enhances arginine and NO levels, may contribute to neurological homeostasis; however, reduced citrulline levels have been documented in BD patients (40). NOS activity, which can be estimated by the citrulline/arginine ratio, is often diminished in BD, particularly nNOS activity in the central nervous system (41). Consistent with the findings of our study, emerging evidence supports the hypothesis that arginine pathway metabolites, such as ADMA, SDMA, and citrulline, may play a critical role in mood regulation and the pathogenesis of bipolar disorder (42).
Cognitive deficits in individuals with a familial predisposition to BD, particularly in verbal learning, memory, processing speed, and executive functions, have been well-documented, suggesting hereditary influences on cognitive functioning (43). In the present study, the Stroop test revealed that the high-risk group took significantly longer to complete the second section compared to controls, a result that aligns with previous literature. A recent study found that the offspring of bipolar parents performed below controls in areas such as processing speed, verbal fluency, visual memory, and working memory, with the greatest impairments observed in verbal memory and executive function (44). Performance on the SDLT indicated that the high-risk group showed poorer short-term memory and learning, lending further support to the notion that executive function deficits, particularly in verbal memory, may serve as an endophenotype for BD (45). The CT also indicated that the high-risk group made significantly more errors in target detection, further highlighting the executive dysfunction present in BD offspring.
A key hypothesis of this study was the relationship between arginine metabolites and neurocognitive functions. Prior research has demonstrated that NOS activity is associated with spatial memory (46). Furthermore, plasma ADMA levels have been found to be significantly elevated in patients with schizophrenia, with even higher concentrations in those experiencing multiple episodes. Notably, ADMA levels were shown to negatively correlate with attention, working memory, and executive function in schizophrenia patients (47). In individuals with major depressive disorder, a significant relationship has been observed between cognitive functions, particularly sustained attention, and the levels of ADMA and NO (48). Our findings revealed significant correlations between ADMA, L-NMMA, homoarginine, citrulline, ornithine levels, and NOS activity with executive and cognitive functions, which are in line with the literature. These results underscore the potential influence of arginine and its metabolites on cognitive performance, especially in high-risk individuals.
The strengths of our study include the comprehensive analysis of arginine metabolite levels in unaffected offspring of BD patients, making this the first study to examine these metabolites in relation to cognitive function in this specific population. We ensured sample homogeneity by carefully screening both high-risk and control groups for comorbid illnesses. Additionally, fasting serum samples were collected after an 8-hour overnight fast, minimizing dietary effects on metabolite levels. Several limitations of the study should be acknowledged. These include the relatively small sample size, the cross-sectional study design, the absence of direct NO measurements, the indirect assessment of NOS activity, and the exclusive evaluation of arginine metabolites in peripheral blood without consideration of central levels. Future longitudinal studies with larger sample sizes, incorporating cerebrospinal fluid analysis and neuroimaging techniques, are warranted to validate and further expand upon these findings.
In conclusion, our findings indicate that alterations in SDMA, citrulline, ornithine, and NOS activity in the unaffected offspring of BD patients may contribute to BD pathophysiology and serve as peripheral biomarkers in this high-risk group. Further longitudinal studies are needed to better understand these metabolic changes and their potential role in biomarker-based prevention strategies. This study highlights the link between arginine metabolism and neurocognitive function, offering valuable insights into BD endophenotypes and the early identification of at-risk individuals.
The raw data supporting the conclusions of this article will be made available by the authors, without undue reservation.
The studies involving humans were approved by Local Ethics Committee of Selçuk University Faculty of Medicine. The studies were conducted in accordance with the local legislation and institutional requirements. Written informed consent for participation in this study was provided by the participants’ legal guardians/next of kin.
GA: Conceptualization, Data curation, Formal analysis, Funding acquisition, Investigation, Methodology, Resources, Validation, Visualization, Writing – original draft, Writing – review & editing. FÇ: Project administration, Supervision, Writing – review & editing. FA: Methodology, Resources, Writing – review & editing. OT: Methodology, Writing – review & editing. ÖÇ: Data curation, Resources, Writing – review & editing. MT: Formal analysis, Writing – review & editing. BS: Data curation, Writing – review & editing. ST: Writing – review & editing. HU: Writing – review & editing. BÖ: Methodology, Writing – review & editing. KA: Conceptualization, Supervision, Writing – review & editing.
The author(s) declare that no financial support was received for the research, authorship, and/or publication of this article.
We thank all participants for providing data.
The authors declare that the research was conducted in the absence of any commercial or financial relationships that could be construed as a potential conflict of interest.
The author(s) declare that no Generative AI was used in the creation of this manuscript.
All claims expressed in this article are solely those of the authors and do not necessarily represent those of their affiliated organizations, or those of the publisher, the editors and the reviewers. Any product that may be evaluated in this article, or claim that may be made by its manufacturer, is not guaranteed or endorsed by the publisher.
1. Kerner B. Genetics of bipolar disorder. Appl Clin Genet. (2014) 7:33–42. doi: 10.2147/TACG.S39293
2. Smoller JW, Craddock N, Kendler K, Lee PH, Neale BM, Nurnberger JI, et al. Cross-Disorder Group of the Psychiatric Genomics Consortium. Identification of risk loci with shared effects on five major psychiatric disorders: a genome-wide analysis. Lancet. (2013) 381:1371–9. doi: 10.1016/S0140-6736(12)62129-1
3. Arts B, Jabben N, Krabbendam L, van Os J. Meta-analyses of cognitive functioning in euthymic bipolar patients and their first-degree relatives. Psychol Med. (2009) 39:525–37. doi: 10.1017/S0033291707001675
4. Scaini G, Rezin GT, Carvalho AF, Streck EL, Berk M, Quevedo J. The role of nitric oxide and related pathways in the pathophysiology of bipolar disorder. Mol Neurobiol. (2016) 53:1–11. doi: 10.1007/s12035-015-9092-7
5. Braun D, Schlossmann J, Haen E. Asymmetric dimethylarginine in psychiatric disorders. Psychiatry Res. (2021) 300:113901. doi: 10.1016/j.psychres.2021.113901
6. Hallak JE, Crippa JA, Quevedo J, Roesler R, Schröder N, Nardin P, et al. Nitric oxide and pathophysiology of neuropsychiatric disorders. Front Neurosci. (2015) 9:73. doi: 10.3389/fnins.2015.00073
7. Bielau H, Brisch R, Bernard-Mittelstaedt J, Dobrowolny H, Gos T, Baumann B, et al. Immunohistochemical evidence for impaired nitric oxide signaling of the locus coeruleus in bipolar disorder. Brain Res. (2012) 1459:91–9. doi: 10.1016/j.brainres.2012.04.006
8. Brown NC, Andreazza AC, Young LT. An updated meta-analysis of oxidative stress markers in bipolar disorder. Psychiatry Res. (2014) 218:61–8. doi: 10.1016/j.psychres.2014.04.005
9. Clark TC, Tinsley J, Sigholt T, Macqueen DJ, Martin SA. Arginine, ornithine and citrulline supplementation in rainbow trout: Free amino acid dynamics and gene expression responses to bacterial infection. Fish Shellfish Immunol. (2020) 98:374–90. doi: 10.1016/j.fsi.2020.01.026
10. Mariotti F, Petzke K, Bonnet D, Szezepanski I, Bos C, Huneau J, et al. Kinetics of the utilization of dietary arginine for nitric oxide and urea synthesis: insight into the arginine-nitric oxide metabolic system in humans. Am J Clin Nutr. (2013) 97:972–9. doi: 10.3945/ajcn.112.048025
11. Narvik J, Vanaveski T, Innos J, Philips MA, Ottas A, Haring L, et al. Metabolic profile associated with distinct behavioral coping strategies of 129Sv and Bl6 mice in repeated motility test. Sci Rep. (2018) 8:1–11. doi: 10.1038/s41598-018-21752-9
12. Heneka MT, Kummer MP, Latz E. Innate immune activation in neurodegenerative disease. Nat Rev Immunol. (2014) 14:463–77. doi: 10.1038/nri3702
13. Block ML, Zecca L, Hong JS. Microglia-mediated neurotoxicity: uncovering the molecular mechanisms. Nat Rev Neurosci. (2007) 8:57–69. doi: 10.1038/nrn2038
14. Brown GC, Neher JJ. Inflammatory neurodegeneration and mechanisms of microglial killing of neurons. Mol Neurobiol. (2010) 41:242–7. doi: 10.1007/s12035-010-8105-9
15. Förstermann U, Sessa WC. Nitric oxide synthases: regulation and function. Eur Heart J. (2012) 33:829–37. doi: 10.1093/eurheartj/ehr304
16. Li J, Doyle KM, Tatlisumak T. Polyamines in the brain: distribution, biological interactions, and their potential therapeutic role in brain ischaemia. Curr Medicinal Chem. (2007) 14:1807–13. doi: 10.2174/092986707781368411
17. Zinellu A, Tommasi S, Sedda S, Mangoni AA. Arginine metabolomics in mood disorders. Heliyon. (2024) 10(6):e27292. doi: 10.1016/j.heliyon.2024.e27292
18. Ünlü A, Eryavuz Onmaz D, Abusoğlu S, Abusoğlu G. HPLC and LC-MS/MS measurement methods for the quantification of asymmetric dimethylarginine (ADMA) and related metabolites. Turk J Biochem. (2021) 46:327–47. doi: 10.1515/tjb-2021-0012
19. Bortolato B, Miskowiak KW, Köhler CA, Vieta E, Carvalho AF. Cognitive dysfunction in bipolar disorder and schizophrenia: a systematic review of meta-analyses. Neuropsychiatr Dis Treat. (2015) 11:3111–25. doi: 10.2147/NDT.S76700
20. Kurtz MM, Gerraty RT. A meta-analytic investigation of neurocognitive deficits in bipolar illness: profile and effects of clinical state. Neuropsychology. (2009) 23:551–62. doi: 10.1037/a0016277
21. Bourne C, Aydemir Ö, Balanzá-Martínez V, Bora E, Brissos S, Cavanagh JTO, et al. Neuropsychological testing of cognitive impairment in euthymic bipolar disorder: an individual patient data meta-analysis. Acta Psychiatr Scand. (2013) 128:149–62. doi: 10.1111/acps.12133
22. Klimes-Dougan B, Jeong J, Kennedy KP, Allen TA. Intellectual functioning in offspring of parents with bipolar disorder: A review of the literature. Brain Sci. (2017) 7:76. doi: 10.3390/brainsci7070076
23. De la Serna E, Sugranyes G, Sanchez-Gistau V, Rodriguez-Toscano E, Baeza I, Vila M, et al. Neuropsychological characteristics of child and adolescent offspring of patients with schizophrenia or bipolar disorder. Schizophr Res. (2017) 183:110–5. doi: 10.1016/j.schres.2016.11.011
24. Unal F, Oktem F, Cetin Cuhadaroglu F, Cengel Kultur SE, Akdemir D, Foto Ozdemir D, et al. Reliability and validity of the schedule for affective disorders and schizophrenia for school-age children-present and lifetime version, DSM-5 November 2016-Turkish adaptation (K-SADS-PL-DSM-5-T). Turk J Psychiatry. (2019) 30:42–50. doi: 10.5080/u23441
25. Karakaş S, Erdoğan E, Sak L, Soysal AŞ, Ulusoy T, Ulusoy İY, et al. Stroop test TBAG form: standardisation for turkish culture, reliability and validity. Clin Psychiatry. (1999) 2:75–88.
26. MacLeod CM. The Stroop task: The "gold standard" of attentional measures. J Exp Psychol Gen. (1992) 121:12–4. doi: 10.1037/0096-3445.121.1.12
27. Karakaş S. A collection of standardized neuropsychological tests for Turkish culture: BİLNOT Battery. In: Proceedings of the 32nd National Neurology Congress. İstanbul: Ufuk Matbaası Publishing (1996).
28. Karakaş HM, Karakaş S. Frontal cortical activations induced by the number sequence learning test: fMRI patterns. J Clin Psychiatry. (2001) 4:79–86.
29. Kiliç BG, Irak M, Koçkar Aİ, Şener Ş, Karakaş S. Standardization study of the turkish form of a cancellation test in 6-11 year old children. J Clin Psychiatry. (2002) 5:213–28.
30. Morris RD. Relationships and distinctions among the concepts of attention, memory, and executive function: A developmental perspective. Dev Neuropsychol. (1996) 12:469–92.
31. Cohen J. Statistical power analysis for the behavioral sciences. 2nd ed. Routledge: Routledge (2013). doi: 10.4324/9780203771587
32. Li ZA, Cai Y, Taylor RL, Eisenstein SA, Barch DM, Marek S, et al. Associations between socioeconomic status, obesity, cognition, and white matter microstructure in children. JAMA Network Open. (2023) 6:e2320276. doi: 10.1001/jamanetworkopen.2023.20276
33. Tomasi D, Volkow ND. Childhood obesity's effect on cognition and brain connectivity worsens with low family income. JCI Insight. (2024) 9:e181690. doi: 10.1172/jci.insight.181690
34. Schäfer I, Hansen H, Schön G, Höfels S, Altiner A, Dahlhaus A, et al. The influence of age, gender, and socio-economic status on multimorbidity patterns in primary care. First results from the multicare cohort study. BMC Health Serv Res. (2012) 12:89. doi: 10.1186/1472-6963-12-89
35. Eid L, Heim K, Doucette S, McCloskey S, Duffy A, Grof P. Bipolar disorder and socioeconomic status: what is the nature of this relationship? Int J Bipolar Disord. (2013) 1:9. doi: 10.1186/2194-7511-1-9
36. Sletved KSO, Coello K, Stanislaus S, Kjærstad HL, Melbye SA, Faurholt-Jepsen M, et al. Socio-economic status and functioning in patients newly diagnosed with bipolar disorder and their unaffected siblings: Results from a cross-sectional clinical study. J Affect Disord. (2022) 310:404–11. doi: 10.1016/j.jad.2022.05.023
37. Yanik M, Vural H, Tutkun H, Zoroğlu SS, Savaş HA, Herken H, et al. The role of the arginine-nitric oxide pathway in the pathogenesis of bipolar affective disorder. Eur Arch Psychiatry Clin Neurosci. (2004) 254:43–7. doi: 10.1007/s00406-004-0441-1
38. Yılmaz E, Şekeroğlu MR, Yılmaz E, Çokluk E. Evaluation of plasma agmatine level and its metabolic pathway in patients with bipolar disorder during manic episode and remission period. Int J Psychiatry Clin Pract. (2019) 23:128–33. doi: 10.1080/13651501.2019.1585897
39. Ustundag MF, Ozcan H, Gencer AG, Yilmaz ED, Uğur K, Oral E, et al. Nitric oxide, asymmetric dimethylarginine, symmetric dimethylarginine and L-arginine levels in psychotic exacerbation of schizophrenia and bipolar disorder manic episode. Saudi Med J. (2020) 41:38–45. doi: 10.15537/smj.2020.1.24869
40. Althoff MD, Peterson R, McGrath M, Jin Y, Grasemann H, Sharma S, et al. Phenotypic characteristics of asthma and morbidity are associated with distinct longitudinal changes in L-arginine metabolism. BMJ Open Respir Res. (2023) 10(1):e001683. doi: 10.1136/bmjresp-2023-001497
41. Baba H, Suzuki T, Arai H, Emson PC. Expression of nNOS and soluble guanylate cyclase in schizophrenic brain. Neuroreport. (2004) 15:677–80. doi: 10.1097/00001756-200403220-00026
42. Baranyi A, Amouzadeh-Ghadikolai O, Rothenhäusler HB, Theokas S, Robier C, Baranyi M, et al. Nitric oxide-related biological pathways in patients with major depression. PloS One. (2015) 10(11):e0143397. doi: 10.1371/journal.pone.0143397
43. Bora E. A comparative meta-analysis of neurocognition in first-degree relatives of patients with schizophrenia and bipolar disorder. Eur Psychiatry. (2017) 45:121–8. doi: 10.1016/j.eurpsy.2017.05.022
44. Bora E, Can G, Ildız A, Ulaş G, Öngün CH, İnal NE, et al. Neurocognitive heterogeneity in young offspring of patients with bipolar disorder: The effect of putative clinical stages. J Affect Disord. (2019) 257:130–5. doi: 10.1016/j.jad.2019.06.011
45. Cotrena C, Damiani Branco L, Ponsoni A, Samamé C, Milman Shansis F, Paz Fonseca R. Executive functions and memory in bipolar disorders I and II: new insights from meta-analytic results. Acta Psychiatr Scand. (2020) 141:110–30. doi: 10.1111/acps.13147
46. Majlessi N, Choopani S, Bozorgmehr T, Azizi Z. Involvement of hippocampal nitric oxide in spatial learning in the rat. Neurobiol Learn Mem. (2008) 90:413–9. doi: 10.1016/j.nlm.2008.05.006
47. Yang YJ, Xiong JW, Zhao Y, Zhan JQ, Chen HB, Yan K, et al. Increased plasma asymmetric dimethylarginine is associated with cognitive deficits in patients with schizophrenia. Psychiatry Res. (2016) 246:480–1. doi: 10.1016/j.psychres.2016.09.037
Keywords: arginine, asymmetric dimethylarginine (ADMA), bipolar disorder, children, neurocognitive function, high risk, offspring
Citation: Arıcı Sağlıyan G, Çetin FH, Akyürek F, Tok O, Çiçek Zekey Ö, Tezcan ME, Sağlıyan B, Türkoğlu S, Uçar HN, Öztürk B and Altınbaş K (2025) Arginine metabolism and neurocognitive impairment in offspring of bipolar parents: a high-risk case-control study. Front. Psychiatry 16:1511397. doi: 10.3389/fpsyt.2025.1511397
Received: 14 October 2024; Accepted: 30 January 2025;
Published: 17 February 2025.
Edited by:
Harry Pantazopoulos, University of Mississippi Medical Center, United StatesReviewed by:
Ravi Vumma, University of New England, United StatesCopyright © 2025 Arıcı Sağlıyan, Çetin, Akyürek, Tok, Çiçek Zekey, Tezcan, Sağlıyan, Türkoğlu, Uçar, Öztürk and Altınbaş. This is an open-access article distributed under the terms of the Creative Commons Attribution License (CC BY). The use, distribution or reproduction in other forums is permitted, provided the original author(s) and the copyright owner(s) are credited and that the original publication in this journal is cited, in accordance with accepted academic practice. No use, distribution or reproduction is permitted which does not comply with these terms.
*Correspondence: Gökçeçiçek Arıcı Sağlıyan, ZHIuZ29rY2VhcmljaUBob3RtYWlsLmNvbQ==
Disclaimer: All claims expressed in this article are solely those of the authors and do not necessarily represent those of their affiliated organizations, or those of the publisher, the editors and the reviewers. Any product that may be evaluated in this article or claim that may be made by its manufacturer is not guaranteed or endorsed by the publisher.
Research integrity at Frontiers
Learn more about the work of our research integrity team to safeguard the quality of each article we publish.