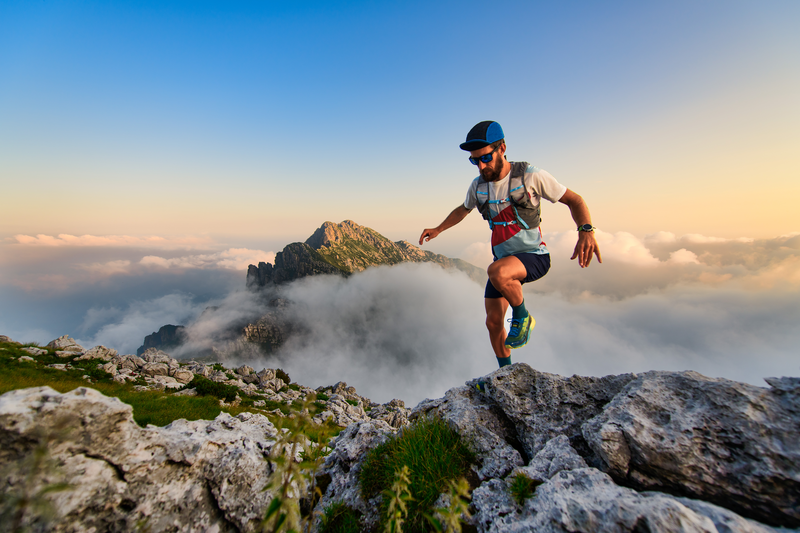
94% of researchers rate our articles as excellent or good
Learn more about the work of our research integrity team to safeguard the quality of each article we publish.
Find out more
REVIEW article
Front. Psychiatry , 30 January 2025
Sec. Neurostimulation
Volume 16 - 2025 | https://doi.org/10.3389/fpsyt.2025.1479240
Recent technological advances in intracranial brain stimulation have enhanced the potential of neuromodulation for addressing neuropsychiatric disorders. We present a review of the methodology and the preliminary outcomes of the pioneering studies exploring intracranial biomarker detection and closed-loop neuromodulation to modulate high-symptom severity states in neuropsychiatric disorders. We searched PubMed, Scopus, Web of Science, Embase, and PsycINFO/PsycNet, followed by the reference and citation lists of retrieved articles. This search strategy yielded a total of 583 articles, of which 5 articles met the inclusion criteria, focusing on depression, obsessive-compulsive disorder, post-traumatic stress disorder, and binge eating disorder. We discuss the methodology of biomarker identification, the biomarkers identified, and the preliminary treatment outcomes for closed-loop neuromodulation. Successful biomarker identification hinges on investigating across various setting. Targeted neuromodulation, either directed at the biomarker or within its associated neural network, offers a promising treatment approach. Future research should seek to understand the mechanisms underlying the effects of neuromodulation as well as the long-term viability of these treatment effects across different neuropsychiatric conditions.
Neuropsychiatric disorders have a profound impact on the mental health of approximately 970 million individuals, resulting in healthcare costs reaching into the trillions (1). Despite the availability of therapeutic and pharmaceutical treatments, only a fraction of patients respond positively to these conventional interventions. Standard of care achieves remission in fewer than half of patients with obsessive-compulsive disorder (OCD) (2), and a mere 30% of individuals grappling with depression attain remission (3, 4). These challenges are further compounded by issues such as adverse drug effects (5), logistical complexities in administering treatments—especially with psychotherapeutic options—and suboptimal treatment adherence (6). While symptoms are remarkably heterogeneous, treatments are often delivered based on the diagnosis, rather than the presenting functional symptoms. As neuropsychiatric diseases are increasingly understood to be disorders of dysfunctional neural circuits, there arises a need to understand the patient’s unique circuit-level pathophysiology, particularly for treatment-resistant individuals (7).
Among various alternatives, neural circuitry modulation through techniques like transcranial electrical or magnetic stimulation (tES/TMS) has emerged as a promising treatment for neuropsychiatric disorders (8–12). These interventions often target neural network dysfunction associated with neuropsychiatric conditions, potentially alleviating corresponding symptoms, such as in depression and OCD (13). However, transcranial approaches encounter inherent constraints. For example, the application of transcranial techniques restricts access to deep neural structures intricately associated with neuropsychiatric disorders, including the amygdala-hippocampus complex and nucleus accumbens (14). Further technical challenges, such as the complexity of simultaneously recording electroencephalography (EEG) and administering tES/TMS, present obstacles. Although office-based administration is effective (11), this setting is not always the most conducive, given the inaccessibility for some patients, the temporal variation in symptoms, and the inadequacy of accommodating diverse environments. This notably impacts patients whose treatment necessitates addressing specific exposures and contextual factors, such as individuals suffering from OCD and addiction.
To mitigate these issues, recent research has explored two novel approaches. First, to better target relevant neuropsychiatric structures, recent studies have attempted to obtain direct electrophysiology using implanted intracranial electrodes (15–21). Intracranial EEG (iEEG) captures neural circuits in high spatiotemporal detail (22), available both on the brain’s surface with subdural electrodes or directly into brain structures using depth electrodes (23). This approach is commonly used as a part of surgical epilepsy evaluation in those with drug-resistant epilepsy and has more recently been applied to understanding the neural correlates of neuropsychiatric diseases, such as depression (24), OCD (25), and psychosis (26).
Second, to translate these in-lab findings into more flexible at-home treatments, recent research has further explored chronically implanted closed-loop neurostimulation devices such as Responsive Neurostimulation (RNS) or adaptive deep brain stimulation (DBS) to achieve closed-loop stimulation. As opposed to an open-loop system where stimulation is delivered at a predetermined interval irrespective of neurophysiological state, closed-loop stimulation involves the detection of biomarkers related to symptom severity and the subsequent delivery of time-specific stimulation in response, within milliseconds (27). Responsive neurostimulation refers to the delivery of stimulation for a fixed duration after a triggering event, while adaptive neurostimulation involves adjustment of therapeutic parameters based upon changes to the neural signals (28). These options make it possible for patients to engage in regular life activities in an at-home setting while receiving treatment benefits, as well as paralleling treatment to the natural variations in symptom severity.
Despite great potential, many unknowns persist regarding the mechanisms, efficacy, and long-term effects of these intracranial neuromodulation effects in neuropsychiatric conditions. Therefore, to systematically map the existing literature and to better understand treatment opportunities for researchers and clinicians, we conducted a review of recent clinical studies employing intracranial, closed-loop neurostimulation as a novel treatment for neuropsychiatric diseases. Our objectives include: (1) reporting and summarizing the approaches to biomarker discovery; (2) reporting and summarizing the neurostimulation protocols and preliminary treatment outcomes; and (3) providing recommendations for consideration for future studies.
A preliminary search of MEDLINE, the Cochrane Database of Systematic Reviews, and JBI Evidence Synthesis found no existing reviews on the topic. This review draws inspiration from the Joanna Briggs Institute methodology (29), and the Preferred Reporting Items for Systematic Reviews and Meta-Analyses extension for Scoping reviews (PRISMA-ScR) guidelines (30). We used a two-stage search strategy to identify relevant, published articles that identify intracranial electrophysiological biomarkers for closed-loop neuromodulation of neuropsychiatric diseases. In the first stage, we searched the following databases: PubMed, Scopus, Web of Science, Embase, and PsycInfo/PsycNet. Search terms included keywords such as “intracranial recording,” “closed-loop neuromodulation,” and “neuropsychiatric disorders” (see Supplementary Table 1 for details). This yielded a total of 185 articles, of which three articles were eligible for the review. In the second stage, we used a forward and backward snowballing approach to optimize our search, which involved searching reference and citation lists of retrieved articles for additional relevant studies (31). As of February 2024, this two-stage search strategy yielded a total of 583 articles which were reviewed for relevance and eligibility based on inclusion and exclusion criteria (Figure 1).
Figure 1. Flow diagram (30) for systematic literature search and selection of articles, created with PRISMA 2020 Shiny App Online Tool (56).
Articles were included if they (1) included iEEG recordings, whether from a chronically implanted device or through sub-chronic iEEG placement; (2) correlated these iEEG features or biomarkers with at least one neuropsychiatric disorder symptom; and (3) applied electrical stimulation to affect the severity of the identified neuropsychiatric disorder symptom through closed-loop neuromodulation. Articles were excluded if they (1) did not feature an intracranial biomarker that was associated with a symptomatic state; (2) did not perform closed-loop intracranial stimulation in response to that biomarker; (3) were animal studies, nonclinical or technical articles, review articles, editorials, or conference presentation abstracts; or (4) were not written in English. Each article excluded was coded according to the numbers above (ex. - 1 if there was no mention of an intracranial biomarker). Irrelevant articles were excluded at two levels: (1) by reviewing the title and abstract, or (2) after full review (see Figure 1 for details).
Key characteristics of the included studies were recorded following an a priori coding scheme, including (1) background information on the articles, such as title, author, publication year, and number of patients; (2) electrode information, such as electrode recording device and location of implanted electrodes; (3) biomarker identification and selection methods; (4) symptom-specific biomarker findings; and (5) caveats. For results related to the stimulation therapy, key characteristics were: (1) target; (2) stimulation protocol, including the device used and stimulation parameters; and (3) treatment outcomes, including primary endpoint and remission criteria, and whether both were met. All the a priori criteria were coded as columns in a data-charting form and were filled out by two separate reviewers. Results were summarized in tabular format (see Tables 1–4).
Our search yielded a total of 583 articles. After review for duplicates and relevancy, 46 articles were identified, of which 5 were included in this review. The majority of the studies excluded at full review did not apply closed-loop neurostimulation. The included studies, published between 2021 and 2024, had sample sizes ranging from a single case report to 5 participants, all with treatment-resistant neuropsychiatric diseases. Many of the patients had attendant neuropsychiatric and neurological comorbidities. Two articles investigated OCD, one article focused on depression, one article studied PTSD, and another studied binge eating disorder. Each of the studies followed a similar paradigm of intracranial electrophysiological biomarker detection by recording during various symptomatic states and through different settings, including home recordings with self-report symptoms to provide ecological validity, through provocatory behavioral tasks, or therapy sessions (depicted in Figure 2). For instance, Provenza and colleagues obtained recordings from self-report logging during symptomatic states, during exposure and response therapy sessions, as well as during behavioral tasks aimed at provoking an obsessive state (32). Details from each study are shared in Tables 2, 3. One study took an additional step to contextualize the biomarker and response to stimulation within the underlying structural and functional connectivity of the subnetwork involved (33).
Figure 2. Study paradigm. (A) Exploratory mapping versus (B) Anatomically targeted recording and stimulation (via closed-loop neuromodulation device electrodes). (C) Detection of electrophysiological biomarkers (above) corresponding to a state of high symptom severity. (D) Closed-loop neuromodulation as informed by the detected biomarker. (E) Change in symptoms, as defined by (top to bottom): clinical measures, physiological measures, and research-defined biomarker changes. Image created with BioRender.com.
Successful application of intracranial stimulation requires the identification of a reliable biomarker that tracks neuropsychiatric symptoms. This involves two different approaches: exploratory mapping and targeted recording (Figures 2A, B). Exploratory mapping involves a wider distribution of implanted electrodes, aiming to identify an implicated region or circuit by linking their electrophysiological features with the severity of neuropsychiatric symptoms through a process akin to conventional seizure foci mapping. Targeted recording, in contrast, involves direct recording from an a priori structure based on an assumed or previously tested association between a given anatomical target structure and neuropsychiatric symptoms. Both exploratory mapping and targeted recording approaches in these studies rely on empirical, or data driven, methods to identify biomarkers as therapeutic targets. Each study identified time periods corresponding to symptomatic states and examined electrophysiologic correlates during these phases. While two studies corroborated findings with animal models (34, 35), all studies utilized power spectral analyses to empirically identify relevant biomarkers.
Biomarker selection from either approach involves identifying spectral bands showing power differences that discriminate between high and low symptom severity states (see Tables 2, 3 for the methodology of biomarker selection) (28). Four studies correlated neural features to symptom ratings, one of which took advantage of the detection algorithms implicit to the RNS device (36); these algorithms include line length, area, and bandpass detection tools (37). Effectively, these algorithms identify changes in amplitude, frequency, signal energy, rhythmic spiking activity, or some combination of the above, to differentiate the pattern of pathologic activity from normal functioning (37). One study used a more advanced biomarker selection paradigm; this involves dimensionality reduction and predictive models or classifiers to identify relevant neural features (28, 33).
Given the lack of studies investigating the electrophysiological correlates of neuropsychiatric disorders, personalized circuitry, and the relative complexity of presenting symptoms, it is difficult to know the best structural targets to detect biomarkers from and/or apply with neuromodulation. Exploratory mapping mitigates these challenges by searching electrophysiological features in a wide range of brain structures to link these features with symptom severity. For example, Scangos and colleagues initially mapped out several cortical and subcortical structures within the depression-associated corticolimbic circuit (38), using depth iEEG electrode probes (also known as stereotactic encephalography, sEEGs) in the bilateral orbitofrontal cortex (OFC), amygdala, hippocampus, ventral capsule/ventral striatum (VC/VS) and subgenual cingulate cortex (SGC) (33). For their pilot patient, they found that bilateral amygdala gamma power was sufficient to detect the high symptom severity state (33). Subsequent recording with the RNS implant confirmed high gamma power’s predictive value for symptom severity (87% accuracy) and strong correlation with depression measures, such as the Hamilton Depression Rating Scale (HAMD; r2 = 0.65, P = 1.98 × 10−5) (33). Performing this broader exploration prior to implanting the RNS device enabled contextualization of the biomarker within the patient’s circuit associated with depression.
In contrast to exploratory mapping, targeted recording streamlines biomarker detection by focusing on a predetermined structure informed by prior research. For instance, Gill and colleagues capitalized on the role of the amygdala in PTSD in prior electrophysiological studies which demonstrated increased theta band power associated with fear-related memory retrieval in PTSD (34). As such, they implanted RNS electrodes into the amygdala of two individuals with PTSD and found increases in theta power within the amygdala corresponded with a high symptom severity state, corroborating prior research efforts, linking increased theta power to fear-related memory retrieval (39). Likewise, Frank et al. implanted DBS leads into the anterior limb of the internal capsule based on promising findings from prior studies demonstrating the efficacy of DBS therapy in this region for treating OCD and depression. They found an inverse correlation between low and high gamma and depression scores (via VAS-D) (40).
Similarly, for behavior-related disorders, such as OCD and binge eating disorder, studies concentrated on the reward/reinforcement system of the basal ganglia (39, 41). Looking at the ventral capsule/ventral striatum (VC/VS) for OCD, Provenza and colleagues found that delta power was negatively correlated with symptom severity across all three patients whose recordings were analyzed (right VC/VS, r = -0.59, left VC/VS, r = -0.56) (32). In a separate study, Nho and colleagues identified low-frequency signals (<15Hz) on iEEG coinciding with occurrence of obsessive thoughts in both ambulatory and provocatory settings (36). For binge eating disorder, Shivacharan and colleagues found increases in delta within the nucleus accumbens (NAc) corresponded with a high symptom severity state in two patients (35). This finding recapitulated prior work which found an association between anticipation of food reward with the increased low frequency power in the NAc of mice (35, 42).
While the targeted structure approach allows personalized biomarker identification within one structure, preliminary mapping allows the identification of the most relevant biomarker across a patient-specific circuit. Despite these differences, both approaches have been effectively used to guide intracranial stimulation for the treatment of neuropsychiatric disorders.
Once an electrophysiological biomarker is identified, it is monitored and manipulated using intracranial electrical stimulation with the goal of alleviating the associated neuropsychiatric symptoms. The efficacy of this hinges on factors such as how stimulation is implemented and how the outcomes are evaluated.
Stimulation parameters are typically set to provide maximal therapeutic benefit conferred at the lowest possible stimulation settings to avoid patient discomfort and stimulation side effects, as well as to minimize charge and power consumption (43). To modulate the function of identified electrophysiological biomarkers, prior studies have adopted intermittent bursts of high-frequency (>=100 Hz) electrical stimulation, consistent with current clinical practices in setting neuromodulation parameters in DBS for movement disorders and RNS for epilepsy (44, 45). The exact parameters, including amplitude and other settings, are tailored to each patient’s response and tolerability to stimulation. These stimulation protocols are often implemented for an extended period, ranging from 8 weeks to two years. Target engagement, whether or not the stimulus reaches the intended location and sufficiently modulates the region (46), is well characterized in these studies, relative to transcranial approaches, because the same stimulation electrodes are also used for recording. However, retrospective confirmation of the implanted electrode location is not always available, leaving the exact location implanted at the discretion of the treating psychiatrist and neurosurgeon.
To evaluate the efficacy of these stimulation protocols, various outcome criteria are compared between the initial weeks of treatment relative to that at the end of the treatment period. This involves clinical assessment, physiological changes, and research-based criteria. For example, Scangos and colleagues assessed outcomes via clinical depression scales, such as HAMD and Montgomery-Asberg Depression Rating Scale (MADRS) (33). For PTSD symptoms, Gil and colleagues tracked clinical assessment scores of the Clinician-Administered PTSD Scale and PTSD Checklist for DSM-5. They found that symptom severity was significantly correlated with amygdala theta band power (34). Provenza and colleagues tracked objective changes in affect through Automated Facial Action Recognition as well as other physiologic measures such as EKG, blood volume pulse, and EEG (32).
The overall success rate was consistently defined by the amount of improvement from pre-treatment assessment to the primary endpoint based on clinical evaluation criteria specific to individual neuropsychiatric conditions (see Table 4 for more detail). For depression, Scangos and colleagues looked for a change in depression ratings as defined by the MADRS (33). For OCD, research often looks for a change in the Yale-Brown Obsessive-Compulsive Scale (Y-BOSC), for example, whether participants’ scores had shown 35% reduction from baseline in this scale (32). For PTSD, a difference of mean Clinician-Administered PTSD Scale for DSM-5 greater than or equal to 13 is often used as a treatment success criterion (34). For binge eating, Shivacharan and colleagues have defined the primary endpoint as at least 50% of subjects exhibiting a decrease in the number of loss of control eating events per week (35).
Several of these studies also included remission criteria. For example, Scangos and colleagues found their pilot participant met depression remission criteria of MADRS score <10 (33). Provenza and colleagues found 40% of participants (n=5) met the criteria for remission of OCD with a Y-BOCS score of less than or equal to 12 (32). Shivacharan and colleagues found 50% of participants (n=2) met criteria for remission of binge eating disorder with fewer than average of four binge eating events per month over the prior three consecutive months (35). While promising, these results need to be followed up with additional studies to see the larger effect size.
In this review, we examined 5 pioneering studies that integrate intracranial recording and closed-loop stimulation for treating neuropsychiatric conditions. Overall, biomarker identification and stimulation methodologies represented a consistent paradigm across studies. However, our findings indicate a paucity of research focusing specifically on closed-loop approaches. Despite three authors involved in paper selection - one performing the search, two selecting the articles, and one confirming the relevance - potential misses remain possible. The small number of studies limits our ability to make definitive conclusions about the broader applicability and efficacy of these interventions across diverse populations and conditions. Regardless of lingering uncertainties, the potential treatment benefits observed in these 11 participants across these 5 studies underscores the urgent need for further elucidating the mechanisms, treatment efficacy, and long-term viability of this treatment approach.
First, of primary interest, further research should seek to understand the effects of intracranial stimulation in the context of the neuropsychiatric circuitry. Despite numerous past studies utilizing functional neuroimaging, the direct link between brain structures and neuropsychiatric symptoms has remained limited (47, 48). Consequently, many neuropsychiatric symptoms have been reconceptualized as dysfunctions within specific functional networks (49). As such, research that employs preliminary mapping of biomarkers and potential therapeutic sites across broader circuits may enhance neural circuit modulation efficacy. For instance, Scangos and colleagues implanted depth iEEG electrode probes throughout various sites within the emotion circuitry of their patient. Among the electrode locations, they identified amygdala activity as the strongest predictor of depression symptoms. They then performed stimulus-response mapping and discovered that stimulation of the VC/VS led to consistent and sustained symptomatic improvement. To support these findings, they performed evoked potential mapping and found that the VC/VS and amygdala constitute a structurally and functionally connected subnetwork, with the VC/VS influencing numerous distant brain regions (33). This suggests the importance of the VC/VS in modulating the associated network at large, given its significant connections and effectiveness as the optimal site for stimulation (33). This network approach suggests that the success of neuromodulatory treatments hinges on their effects on the entire network (50). Therefore, exploring the broader network as a stimulation target based on preliminary mapping may be crucial for future successes in utilizing intracranial stimulation as a treatment for neuropsychiatric diseases.
Second, future research may also consider complementary approaches to interrogate the heterogeneous neuropsychiatric circuits for effective neuromodulation. Neuropsychiatric symptoms are frequently comorbid across various diseases and widely vary. As such, the Research Domain Criteria (RDoC) was developed as a framework for researchers to explore neuropsychiatric diseases based on broad neurobehavioral functioning domains, rather than operationally defined based on symptoms (51). One key aspect is the characterization of neural circuits that drive specific functional problems (48). In addition to identifying electrophysiological features time-locked to a state of high symptom severity, future research may benefit from probing explicit behavioral tasks that arise from a given functional circuit (1, 52, 53). For instance, dysphoria can be interrogated by electrophysiological responses to emotional imagery tasks, while response inhibition - variably impacted across neuropsychiatric disorders including depression, anxiety, and PTSD - could be examined using the Go/NoGo task (52, 54). In the context of neuromodulation, this approach offers a way to precisely characterize, target, and modulate these behavioral circuits directly. Additionally, modulating the behaviors that arise from these neural circuits may address symptoms common to multiple diseases (55). Bolstering the RDoC framework for closed-loop neuromodulation may provide additional insights into behavior across various disorders, contributing to a better understanding of trans-diagnostic behavioral circuits.
Third, evaluating the long-term viability of this intracranial approach as a treatment for neuropsychiatric conditions is crucial. While some intracranial electroencephalography (iEEG) implants remain in the patient’s brain for an extended period (e.g., RNS), the efficacy of long-term stimulation effects over years remains unknown. For instance, while Scangos and colleagues demonstrated that intracranial effects can reduce patients’ depression symptoms for up to 60 days (33), data from further longitudinal follow-ups are not yet available. It remains uncertain whether direct brain stimulation over an extended period (e.g., a few years) can lead to alterations in the underlying neural circuitry, some of which may even involve structural changes. This raises technical and ethical considerations regarding the extent of this treatment as a long-term solution without introducing potential side effects. Longitudinal follow-up of patients from clinical trials may shed more light on these issues in the upcoming years.
Furthermore, it’s important to note these additional caveats of the intracranial approach. First, the intracranial approach is inherently invasive, significantly limiting its accessibility. Currently, this technique is reserved for individuals with clear treatment resistance; neuromodulation is not intended as a replacement for standard pharmacotherapy but rather as an option for those who haven’t benefited from initial treatments. Given the involvement of pathological networks in symptom production, it may be possible to engage deeper network connections indirectly by modulating cortical projections to influence symptoms effectively. Identifying specific cortical entry points for modulating deeper structures through non-invasive stimulation techniques could represent a promising path for enhancing the applicability and accessibility of this approach in future research. Second, further research is needed to elucidate biomarkers across various patient populations (40). While precision medicine operates at an individual level, identifying common patterns may be necessary to objectively confirm biomarker significance as disease process hallmarks. This will be crucial for scalability across diagnoses and generalizability across demographic groups. Last, identifying the best stimulation targets within the network is essential, considering the possible distinction between the biomarker representing symptomatology and the area offering the best treatment response. Investigating efficient treatment parameters capable of adequately perturbing the targeted circuit is crucial. Collectively, clarifying the mechanisms, treatment efficacy, and long-term viability of the intracranial approach along with addressing these additional caveats will provide necessary insights into neuropsychiatric disorder physiology and contribute to much-needed innovations in future neuropsychiatric treatments.
JL: Conceptualization, Methodology, Writing – original draft, Writing – review & editing. UB: Conceptualization, Writing – original draft, Writing – review & editing. WX: Conceptualization, Methodology, Supervision, Writing – original draft, Writing – review & editing. AK: Conceptualization, Supervision, Writing – original draft, Writing – review & editing.
The author(s) declare that no financial support was received for the research, authorship, and/or publication of this article.
The authors declare that the research was conducted in the absence of any commercial or financial relationships that could be construed as a potential conflict of interest.
All claims expressed in this article are solely those of the authors and do not necessarily represent those of their affiliated organizations, or those of the publisher, the editors and the reviewers. Any product that may be evaluated in this article, or claim that may be made by its manufacturer, is not guaranteed or endorsed by the publisher.
The Supplementary Material for this article can be found online at: https://www.frontiersin.org/articles/10.3389/fpsyt.2025.1479240/full#supplementary-material
1. Scangos KW, State MW, Miller AH, Baker JT, Williams LM. New and emerging approaches to treat psychiatric disorders. Nat Med. (2023) 29:317–33. doi: 10.1038/s41591-022-02197-0
2. Sijercic I, Whitfield KM, Cassin SE, Antony MM. A systematic review of cognitive-behavioral therapy for pharmacotherapy treatment-resistant obsessive-compulsive disorder. J Obsessive-Compulsive Related Disord. (2020) 26:100532. doi: 10.1016/j.jocrd.2020.100532
3. Trivedi MH, Rush AJ, Wisniewski SR, Nierenberg AA, Warden D, Ritz L, et al. Evaluation of outcomes with citalopram for depression using measurement-based care in STAR*D: implications for clinical practice. Am J Psychiatry. (2006) 163:28–40. doi: 10.1176/appi.ajp.163.1.28
4. Gaynes BN, Lux L, Gartlehner G, Asher G, Forman-Hoffman V, Green J, et al. Defining treatment-resistant depression. Depression Anxiety. (2020) 37:134–45. doi: 10.1002/da.22968
5. de Filippis R, Solerdelcoll M, Shalbafan M. Editorial: Safety and side effects of psychotropic medications. Front Psychiatry. (2023) 14:1148158. doi: 10.3389/fpsyt.2023.1148158
6. Farooq S, Naeem F. Tackling nonadherence in psychiatric disorders: current opinion. Neuropsychiatr Dis Treat. (2014) 10:1069–77. doi: 10.2147/NDT.S40777
7. Bormann NL, Trapp NT, Narayanan NS, Boes AD. Developing precision invasive neuromodulation for psychiatry. J Neuropsychiatry Clin Neurosciences. (2021) 33:201–9. doi: 10.1176/appi.neuropsych.20100268
8. Lewis PM, Thomson RH, Rosenfeld JV, Fitzgerald PB. Brain neuromodulation techniques: A review. Neuroscientist. (2016) 22:406–21. doi: 10.1177/1073858416646707
9. Gholamali Nezhad F, Martin J, Tassone VK, Swiderski A, Demchenko I, Khan S, et al. Transcranial alternating current stimulation for neuropsychiatric disorders: a systematic review of treatment parameters and outcomes. Front Psychiatry. (2024) 15. doi: 10.3389/fpsyt.2024.1419243
10. Szymkowicz SM, McLaren ME, Suryadevara U, Woods AJ. Transcranial direct current stimulation use in the treatment of neuropsychiatric disorders: A brief review. Psychiatr annals. (2016) 46:642. doi: 10.3928/00485713-20161006-01
11. Hyde J, Carr H, Kelley N, Seneviratne R, Reed C, Parlatini V, et al. Efficacy of neurostimulation across mental disorders: systematic review and meta-analysis of 208 randomized controlled trials. Mol Psychiatry. (2022) 27:2709–19. doi: 10.1038/s41380-022-01524-8
12. Sabé M, Hyde J, Cramer C, Eberhard AL, Crippa A, Brunoni AR, et al. Transcranial magnetic stimulation and transcranial direct current stimulation across mental disorders: A systematic review and dose-response meta-analysis. JAMA Network Open. (2024) 7:e2412616. doi: 10.1001/jamanetworkopen.2024.12616
13. Temel Y, Hescham SA, Jahanshahi A, Janssen MLF, Tan SKH, van Overbeeke JJ, et al. Neuromodulation in psychiatric disorders. Int Rev Neurobiol. (2012) 107:283–314. doi: 10.1016/B978-0-12-404706-8.00015-2
14. Mahoney JJ, Hanlon CA, Marshalek PJ, Rezai AR, Krinke L. Transcranial magnetic stimulation, deep brain stimulation, and other forms of neuromodulation for substance use disorders: Review of modalities and implications for treatment. J Neurological Sci. (2020) 418:117149. doi: 10.1016/j.jns.2020.117149
15. Kisely S, Li A, Warren N, Siskind D. A systematic review and meta-analysis of deep brain stimulation for depression. Depress Anxiety. (2018) 35:468–80. doi: 10.1002/da.22746
16. Johnson KA, Okun MS, Scangos KW, Mayberg HS, de Hemptinne C. Deep brain stimulation for refractory major depressive disorder: a comprehensive review. Mol Psychiatry. (2024) 29:1075–87. doi: 10.1038/s41380-023-02394-4
17. Figee M, Riva-Posse P, Choi KS, Bederson L, Mayberg HS, Kopell BH. Deep brain stimulation for depression. Neurotherapeutics. (2022) 19:1229–45. doi: 10.1007/s13311-022-01270-3
18. Sheth SA, Mayberg HS. Deep brain stimulation for obsessive-compulsive disorder and depression. Annu Rev Neurosci. (2023) 46:341–58. doi: 10.1146/annurev-neuro-110122-110434
19. Val-Laillet D, Aarts E, Weber B, Ferrari M, Quaresima V, Stoeckel LE, et al. Neuroimaging and neuromodulation approaches to study eating behavior and prevent and treat eating disorders and obesity. NeuroImage Clin. (2015) 8:1–31. doi: 10.1016/j.nicl.2015.03.016
20. Rapinesi C, Kotzalidis GD, Ferracuti S, Sani G, Girardi P, Del Casale A. Brain stimulation in obsessive-compulsive disorder (OCD): A systematic review. Curr Neuropharmacol. (2019) 17:787–807. doi: 10.2174/1570159X17666190409142555
21. Dougherty DD. Deep brain stimulation: clinical applications. Psychiatr Clin North Am. (2018) 41:385–94. doi: 10.1016/j.psc.2018.04.004
22. Parvizi J, Kastner S. Human intracranial EEG: promises and limitations. Nat Neurosci. (2018) 21:474–83. doi: 10.1038/s41593-018-0108-2
23. Mercier MR, Dubarry AS, Tadel F, Avanzini P, Axmacher N, Cellier D, et al. Advances in human intracranial electroencephalography research, guidelines and good practices. NeuroImage. (2022) 260:119438. doi: 10.1016/j.neuroimage.2022.119438
24. Xiao J, Provenza NR, Asfouri J, Myers J, Mathura RK, Metzger B, et al. Decoding depression severity from intracranial neural activity. Biol Psychiatry. (2023) 94:445–53. doi: 10.1016/j.biopsych.2023.01.020
25. Olsen ST, Basu I, Bilge MT, Kanabar A, Boggess MJ, Rockhill AP, et al. Case report of dual-site neurostimulation and chronic recording of cortico-striatal circuitry in a patient with treatment refractory obsessive compulsive disorder. Front Hum Neurosci. (2020) 14:569973. doi: 10.3389/fnhum.2020.569973
26. Takeda Y, Inoue Y, Tottori T, Mihara T. Acute psychosis during intracranial EEG monitoring: close relationship between psychotic symptoms and discharges in amygdala. Epilepsia. (2001) 42:719–24. doi: 10.1046/j.1528-1157.2001.08700.x
27. Iyengar S, Shafer PO, Koubeissi M, Sirven J. Responsive Neurostimulation (RNS). Bowie MD: Epilepsy Foundation. (2017). Available at: https://www.epilepsy.com/treatment/devices/responsive-neurostimulation (Accessed April 25, 2024).
28. Prosky J, Cagle J, Sellers KK, Gilron R, de Hemptinne C, Schmitgen A, et al. Practical closed-loop strategies for deep brain stimulation: lessons from chronic pain. Front Neurosci. (2021) 15. doi: 10.3389/fnins.2021.762097
29. Peters MD, Godfrey C, McInerney P, Munn Z, Tricco AC, Khalil H. Scoping reviews. In: Aromataris E, Lockwood C, Porritt K, Pilla B, Jordan Z, editors. JBI Manual for Evidence Synthesis. Adelaide Australia: JBI. (2024). doi: 10.46658/JBIMES-24-09
30. Tricco AC, Lillie E, Zarin W, O’Brien KK, Colquhoun H, Levac D, et al. PRISMA extension for scoping reviews (PRISMA-ScR): checklist and explanation. Ann Internal Med. (2018) 169:467–73. doi: 10.7326/M18-0850
31. Parker C, Scott S, Geddes A. Snowball sampling. In: SAGE Research Methods Foundations. Cardiff University, UK: Sage Research Methods Foundation (2019). Available at: http://methods.sagepub.com/foundations/snowball-sampling (Accessed April 25, 2024).
32. Provenza NR, Sheth SA, Dastin-van Rijn EM, Mathura RK, Ding Y, Vogt GS, et al. Long-term ecological assessment of intracranial electrophysiology synchronized to behavioral markers in obsessive-compulsive disorder. Nat Med. (2021) 27:2154–64. doi: 10.1038/s41591-021-01550-z
33. Scangos KW, Khambhati AN, Daly PM, Makhoul GS, Sugrue LP, Zamanian H, et al. Closed-loop neuromodulation in an individual with treatment-resistant depression. Nat Med. (2021) 27:1696–700. doi: 10.1038/s41591-021-01480-w
34. Gill JL, Schneiders JA, Stangl M, Aghajan ZM, Vallejo M, Hiller S, et al. A pilot study of closed-loop neuromodulation for treatment-resistant post-traumatic stress disorder. Nat Commun. (2023) 14:2997. doi: 10.1038/s41467-023-38712-1
35. Shivacharan RS, Rolle CE, Barbosa DAN, Cunningham TN, Feng A, Johnson ND, et al. Pilot study of responsive nucleus accumbens deep brain stimulation for loss-of-control eating. Nat Med. (2022) 28:1791–6. doi: 10.1038/s41591-022-01941-w
36. Nho YH, Rolle CE, Topalovic U, Shivacharan RS, Cunningham TN, Hiller S, et al. Responsive deep brain stimulation guided by ventral striatal electrophysiology of obsession durably ameliorates compulsion. Neuron. (2024) 112:73–83.e4. doi: 10.1016/j.neuron.2023.09.034
37. Sun FT, Morrell MJ. The RNS System: responsive cortical stimulation for the treatment of refractory partial epilepsy. Expert Rev Med Devices. (2014) 11:563–72. doi: 10.1586/17434440.2014.947274
38. Oakes P, Loukas M, Oskouian RJ, Tubbs RS. The neuroanatomy of depression: A review. Clin Anat. (2017) 30:44–9. doi: 10.1002/ca.22781
39. Boukezzi S, Baunez C, Rousseau PF, Warrot D, Silva C, Guyon V, et al. Posttraumatic Stress Disorder is associated with altered reward mechanisms during the anticipation and the outcome of monetary incentive cues. NeuroImage Clin. (2020) 25:102073. doi: 10.1016/j.nicl.2019.102073
40. Frank AC, Scangos KW, Larson PS, Norbu T, Lee AT, Lee AM. Identification of a personalized intracranial biomarker of depression and response to DBS therapy. Brain Stimul. (2021) 14:1002–4. doi: 10.1016/j.brs.2021.06.009
41. Huey ED, Zahn R, Krueger F, Moll J, Kapogiannis D, Wassermann EM, et al. A psychological and neuroanatomical model of obsessive-compulsive disorder. J Neuropsychiatry Clin Neurosci. (2008) 20:390–408. doi: 10.1176/appi.neuropsych.20.4.390
42. Wu H, Miller KJ, Blumenfeld Z, Williams NR, Ravikumar VK, Lee KE, et al. Closing the loop on impulsivity via nucleus accumbens delta-band activity in mice and man. Proc Natl Acad Sci. (2018) 115:192–7. doi: 10.1073/pnas.1712214114
43. Ramasubbu R, Lang S, Kiss ZHT. Dosing of electrical parameters in deep brain stimulation (DBS) for intractable depression: A review of clinical studies. Front Psychiatry. (2018) 9:302. doi: 10.3389/fpsyt.2018.00302
44. Johnson MD, Lim HH, Netoff TI, Connolly AT, Johnson N, Roy A, et al. Neuromodulation for brain disorders: challenges and opportunities. IEEE Trans bio-medical engineering. (2013) 60:610–24. doi: 10.1109/TBME.2013.2244890
45. Alcala-Zermeno JL, Starnes K, Gregg NM, Worrell G, Lundstrom BN. Responsive neurostimulation with low frequency stimulation. Epilepsia. (2023) 64:e16–22. doi: 10.1111/epi.17467
46. Wendt K, Denison T, Foster G, Krinke L, Thomson A, Wilson S, et al. Physiologically informed neuromodulation. J Neurological Sci. (2022) 434:120121. doi: 10.1016/j.jns.2021.120121
47. Xia CH, Ma Z, Ciric R, Gu S, Betzel RF, Kaczkurkin AN, et al. Linked dimensions of psychopathology and connectivity in functional brain networks. Nat Commun. (2018) 9:3003. doi: 10.1038/s41467-018-05317-y
48. Morris SE, Cuthbert BN. Research Domain Criteria: cognitive systems, neural circuits, and dimensions of behavior. Dialogues Clin Neurosci. (2012) 14:29. doi: 10.31887/DCNS.2012.14.1/smorris
49. Siddiqi SH, Schaper FLWVJ, Horn A, Hsu J, Padmanabhan JL, Brodtmann A, et al. Brain stimulation and brain lesions converge on common causal circuits in neuropsychiatric disease. Nat Hum Behaviour. (2021) 5:1707–16. doi: 10.1038/s41562-021-01161-1
50. Bilge MT, Gosai A, Widge AS. Deep brain stimulation in psychiatry: mechanisms, models, and next-generation therapies. Psychiatr Clinics North America. (2018) 41:373–83. doi: 10.1016/j.psc.2018.04.003
51. Morris SE, Sanislow CA, Pacheco J, Vaidyanathan U, Gordon JA, Cuthbert BN. Revisiting the seven pillars of RDoC. BMC Med. (2022) 20:220. doi: 10.1186/s12916-022-02414-0
52. Ramos CGL, Tan H, Yamamoto EA, Cleary DR, Mazur-Hart DJ, Shahin MN, et al. Stereotactic electroencephalography in epilepsy patients for mapping of neural circuits related to emotional and psychiatric behaviors: a systematic review. Neurosurgical Focus. (2023) 54:E4. doi: 10.3171/2022.11.FOCUS22615
53. Goldstein-Piekarski AN, Ball TM, Samara Z, Staveland BR, Keller AS, Fleming SL, et al. Mapping neural circuit biotypes to symptoms and behavioral dimensions of depression and anxiety. Biol Psychiatry. (2022) 91:561–71. doi: 10.1016/j.biopsych.2021.06.024
54. Jovanovic T, Ely T, Fani N, Glover EM, Gutman D, Tone EB, et al. Reduced neural activation during an inhibition task is associated with impaired fear inhibition in a traumatized civilian sample. Cortex. (2013) 49:1884–91. doi: 10.1016/j.cortex.2012.08.011
55. Lo MC, Widge AS. Closed-loop neuromodulation systems: next-generation treatments for psychiatric illness. Int Rev Psychiatry. (2017) 29:191–204. doi: 10.1080/09540261.2017.1282438
Keywords: closed loop neuromodulation, neuropsychaitric disorders, neural circuit, neural network, DBS (deep brain stimulation), RNS = responsive neurostimulation
Citation: Langbein J, Boddeti U, Xie W and Ksendzovsky A (2025) Intracranial closed-loop neuromodulation as an intervention for neuropsychiatric disorders: an overview. Front. Psychiatry 16:1479240. doi: 10.3389/fpsyt.2025.1479240
Received: 11 August 2024; Accepted: 13 January 2025;
Published: 30 January 2025.
Edited by:
Shouyan Wang, Fudan University, ChinaCopyright © 2025 Langbein, Boddeti, Xie and Ksendzovsky. This is an open-access article distributed under the terms of the Creative Commons Attribution License (CC BY). The use, distribution or reproduction in other forums is permitted, provided the original author(s) and the copyright owner(s) are credited and that the original publication in this journal is cited, in accordance with accepted academic practice. No use, distribution or reproduction is permitted which does not comply with these terms.
*Correspondence: Weizhen Xie, emFuZXhpZUB1bWQuZWR1; Alexander Ksendzovsky, YWtzZW5kem92c2t5QHNvbS51bWFyeWxhbmQuZWR1
Disclaimer: All claims expressed in this article are solely those of the authors and do not necessarily represent those of their affiliated organizations, or those of the publisher, the editors and the reviewers. Any product that may be evaluated in this article or claim that may be made by its manufacturer is not guaranteed or endorsed by the publisher.
Research integrity at Frontiers
Learn more about the work of our research integrity team to safeguard the quality of each article we publish.