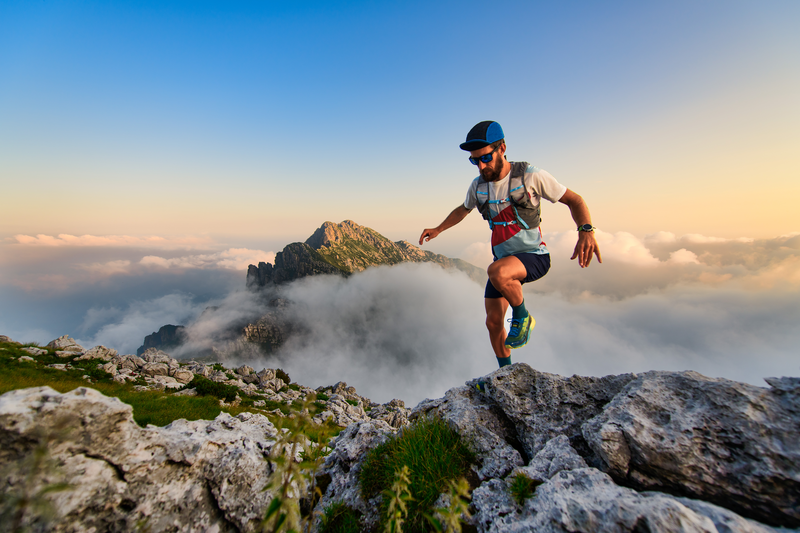
94% of researchers rate our articles as excellent or good
Learn more about the work of our research integrity team to safeguard the quality of each article we publish.
Find out more
ORIGINAL RESEARCH article
Front. Psychiatry , 06 January 2025
Sec. Autism
Volume 15 - 2024 | https://doi.org/10.3389/fpsyt.2024.1507890
This article is part of the Research Topic Innovative and Cutting-edge Approaches to the Identification and Management of Autism Spectrum Disorders View all 6 articles
Aim: Functional neuroimaging studies have suggested that prefrontal cortex dysfunction occurs in individuals with autism spectrum disorder (ASD). Near-infrared spectroscopy (NIRS) is a noninvasive optical tool used to investigate oxygenation and hemodynamic responses in the cerebral cortex by measuring changes in oxygenated hemoglobin. Previous studies using NIRS have suggested that male children with ASD exhibit reduced hemodynamic responses in the dorsolateral prefrontal cortex; however, only a few studies examined this response in adults with ASD.
Methods: We examined the characteristics of prefrontal hemodynamic responses in 114 adults with ASD and 84 typically developing controls. Relative concentrations of oxygenated hemoglobin were measured with frontal probes every 0.1 s during the Stroop color-word task, using 24-channel NIRS.
Results: Our findings demonstrated that the oxygenated hemoglobin changes in the ASD group were significantly smaller than those in the control group at channels 19, 20, 23, and 24- located over the orbitofrontal cortex and frontal pole (p <0.05 for all three channels). The differences in oxygenated hemoglobin changes at Ch 20 were significantly correlated with the Autism-Spectrum Quotient Japanese version (AQ-J) total score and attention switching score, which is a symptom cluster of AQ-J (p = 0.043 and p = 0.009, respectively).
Conclusion: Adults with ASD have reduced prefrontal hemodynamic responses as measured using near-infrared spectroscopy and the reduced activity of the frontal pole in particular is related to reduced attentional function.
Autism spectrum disorder (ASD) is a neurodevelopmental disorder characterized by impaired social and communicative functions, restricted interests, and repetitive behaviors (1). A substantial body of research has demonstrated that individuals with Autism ASD exhibit impaired executive function (2–5). The executive function generally comprises three underlying components: response inhibition, updating working memory representations, and set shifting (6, 7). Previous research indicated that the prefrontal cortex (PFC) is strongly associated with response inhibition, a crucial component of executive function. For instance, patients with frontal lobe damage exhibit increased error rates and prolonged reaction times in the Stroop color-word task (8, 9). Functional neuroimaging studies, such as functional magnetic resonance imaging, have implicated multiple brain regions, including the anterior cingulate gyrus13 and dorsolateral PFC, in the accurate performance of this task (10).
Multichannel near-infrared spectroscopy (NIRS) can be utilized to non-invasively assess hemodynamic responses (cortical activity) in the cerebral cortex using near-infrared light (11, 12). NIRS quantifies alterations in oxygenated hemoglobin [oxy-Hb] and deoxygenated hemoglobin [deoxy-Hb] concentrations within the cerebral cortex. The hemodynamic responses in the cerebral cortex are reflected by local increases in [oxy-Hb] concentration and decrease in [deoxy-Hb] concentration (11, 13). Moreover, alterations in [oxy-Hb] concentrations of the cerebral cortex measured using NIRS demonstrate a correlation with the results obtained from positron emission tomography (PET) (14, 15).
NIRS has been utilized to evaluate cerebral function in subjects with diverse psychiatric disorders, including schizophrenia (16–19), bipolar disorder (19, 20), depression (16), obsessive-compulsive disorder (21–23), dementia (24, 25), post-traumatic stress disorder (26), Tourette’s disorder (27), attention-deficit/hyperactivity disorder (ADHD) (28–31), methamphetamine-induced psychosis (32, 33), and ASD (34, 35). NIRS is deemed to be advantageous for psychiatric research and clinical applications, particularly due to the following considerations. Firstly, NIRS can minimize the effects of motion artifacts, making it useful for experiments involving tasks that require movement, such as speech. Secondly, during measurement, the subject can maintain their typical seated posture, enabling data collection in an environment that closely approximates real-world conditions. Thirdly, it exhibits lower operational costs compared to alternative functional neuroimaging methodologies, demonstrates ease of implementation and utilization, and offers enhanced versatility. Furthermore, it exhibits high temporal resolution (less than 1 second) and possesses the capability to measure alterations in cerebral blood flow among patients with mental disorders in real time and longitudinally (16, 20, 26). These advantages of NIRS facilitate the conduct of neurofunctional imaging research under conditions where PET and functional magnetic resonance imaging (fMRI) measurements are challenging, and it is utilized in various fields beyond mental disorders.
Numerous review articles and meta-analyses have demonstrated that individuals with ASD, spanning childhood, adolescence, and adulthood, exhibit deficits in various aspects of executive function (36–40). The Stroop color-word task is one of the most frequently utilized executive function assessments to measure an individual’s capacity to inhibit a prepotent response in favor of an atypical response (41). This assessment evaluated both cognitive flexibility and inhibitory control (42). These cognitive functions are associated with the inferior frontal, dorsolateral prefrontal, and anterior cingulate cortices, and researchers have observed their activation during the administration of the Stroop color-word task number of correct answers (SCWT) (43, 44). Furthermore, multiple studies have documented suboptimal performance on the SCWT among various patient populations characterized by executive dysfunction (45).
Recent advancements in NIRS have facilitated the non-invasive examination of cerebral functions in various psychiatric disorders, including ASD. Previous investigations utilizing 16-channel (Ch) NIRS have demonstrated that no statistically significant difference was observed in hemodynamic responses within the prefrontal cortex during the Stroop color-word task between typically developing children and those diagnosed with ASD (46, 47). Conversely, our previous research demonstrated PFC dysfunction during the Stroop color-word task in children with ASD, as measured via 24-channel NIRS, in comparison to typically developing children (35).
The heterogeneous findings from NIRS studies in children with ASD regarding prefrontal hemodynamic responses may be attributed to sample characteristics, particularly limited sample sizes and diagnostic imprecision. Furthermore, a limited number of NIRS studies have investigated prefrontal hemodynamic responses in adults with ASD. Consequently, in the present investigation, we assessed the hemodynamic responses of the PFC 24-Ch NIRS during a Stroop color-word task in a relatively large sample of 198 adult participants. Furthermore, all adults with ASD underwent a comprehensive Autism Spectrum Disorder Observation Schedule-Second Edition (ADOS-2) assessment (48), which is a commonly used diagnostic tool for ASD.
The study included 114 participants (85 males and 29 females) with ages ranging from 17 to 51 years, all of whom were diagnosed with ASD in accordance with the criteria outlined in the Diagnostic and Statistical Manual of Mental Disorders Fifth Edition (DSM-5) (1), with 84 typically developing control participants (46 males and 38 females) aged 18–44 years (Table 1). The individuals with ASD had no prior history of treatment for psychiatric disorders and had consulted an experienced psychiatrist at the Department of Psychiatry of the Nara Medical University. Individuals diagnosed with ASD underwent a standardized clinical assessment comprising a psychiatric evaluation, including the ADOS-2 (48), Mini International Neuropsychiatric Interview (Japanese version 5.0.0) (49), and a medical history examination by an experienced psychiatrist. Two experienced psychiatrists confirmed the ASD diagnosis according to DSM-5 (1). We also estimated each participant’s full-scale intelligence quotient (IQ) using the similarities and symbol search subsets of the Wechsler Adult Intelligence Scale-Third Edition, excluding individuals with IQ scores below 70.
We excluded patients with comorbid psychiatric disorders, such as ADHD, as defined by DSM-51, neurological disorders, head injuries, serious medical conditions, or a history of substance abuse and dependence. The final sample included 114 participants with ASD who had no history of previous medications and the Japanese version of the autism-spectrum quotient Japanese (AQ-J) score of ≥26 (50–52). We recruited typically developing controls through local print advertisements. Typically developing participants underwent a standard clinical assessment comprising a psychiatric evaluation, standard diagnostic interviews, and a medical history examination conducted by two experienced psychiatrists. A psychologist evaluated the cognitive abilities of the participants utilizing the Wechsler Adult Intelligence Scale-Fourth Edition (WAIS-IV). Finally, 84 typically developing individuals without confirmed ASD and no current or history of psychiatric or neurological disorders were included in the study. The Institutional Review Board of Nara Medical University approved this study.
The Autism Spectrum Quotient (AQ), developed by Baron-Cohen et al. based on the autism spectrum hypothesis, is a standardized assessment instrument designed to measure ASD traits and is widely utilized for ASD screening and research purposes (50). The Japanese version of the AQ, known as the AQ-J, has demonstrated satisfactory reliability and validity in screening young adults of normal intelligence for ASD, utilizing the same threshold values as the original AQ (50–52). The AQ-J is a self-administered instrument designed to quantify the degree of ASD characteristics or broad phenotype in adults with intelligence within the normal range. The Autism Quotient (AQ) comprises 50 items in total. The AQ is utilized not only for clinical screening but also to assess individual differences in autistic tendencies among typically developing adults; therefore, the AQ is advantageous for both diagnostic purposes and research endeavors (53).
In this investigation, we employed a standard Stroop color-name task that incorporated word reading, incongruent color-name, and color-name conditions (54), and subsequently applied the previously established methodology (35). In this investigation, the word reading task served as the baseline condition, while the incongruent color-name task functioned as the activation condition. The color-name task, which is typically a component of the conventional Stroop color-word task, was omitted from this study. In the Stroop color-word task utilized in this study, each row contained 20 words, with a maximum of 5 rows, resulting in a total of 100 words per page. The initial page comprised the chromatic terms RED, GREEN, and BLUE printed in black ink. On the second page, the words RED, GREEN, and BLUE were printed in red, green, or blue ink, respectively, with the color of the ink not corresponding to the semantic meaning of the word. The lexical items on both pages were distributed randomly, and identical elements were not positioned within the same row.
The procedure was conducted by a qualified psychologist, who initially provided the participants with the following instructions: “For this task, you will first read the words on the initial page, and subsequently, on the second page, you will respond as promptly as possible by indicating your selections through word coloration.” Upon the verbal cue “start,” read the words in the columns from the top left, and respond as promptly as possible by indicating the words or their respective colors. After completing the words in the first column, proceed to the subsequent column. The participant read out the content on the second page for 45 seconds, then proceeded to turn the page over. This process is repeated three times on the second page. Subsequently, the first page is presented again.
The complete protocol of the Stroop Color Word Task comprised a 45-second task (p1), three 45-second tasks (p2), and a subsequent 45-second p1 task (Figure 1A). The 45-second p1 task (word reading task) served as the baseline task, while the p2 task (incongruent color naming task) functioned as the activation task. The number of correct responses in each trial was operationalized as follows: the number of correct responses in the first Stroop Color Word Task (SCWC) (21).
Figure 1. Location of the 24 channels in the near-infrared spectroscopy instrument. (A) Placement of emitters and detectors and definition of each channel. (B) Corresponding anatomical sites for each channel. (C) Data analysis protocols and procedures for each task. The baseline and activation tasks for all Stroop color-word tasks performed were 45 seconds each. These tasks were repeated three times, followed by the baseline task.
NIRS is a spectroscopic method that utilizes the near-infrared region (780nm to 2500nm) of the electromagnetic spectrum. NIRS quantifies the components by irradiating the target with near-infrared light and measuring the change in absorbance. Through the measurement of [oxy-Hb] and deoxygenated hemoglobin [deoxy-Hb], the hemodynamic responses (cortical activity) of the cerebral cortex can be evaluated non-invasively. The hemodynamic response is characterized by an increase in [oxy-Hb] and a decrease in [deoxy-Hb] and is based on the modified Lambert-Beer law, which monitors the absorption of near-infrared light by ([oxy-Hb] and [deoxy-Hb] at two distinct wavelengths (55, 56). The direction of change in [deoxy-Hb] is determined by the degree of oxygenation and volume change of venous blood, and studies utilizing rodent models have demonstrated that [oxy-Hb] serves as a more sensitive indicator of regional cerebral blood flow compared to alternative methods (57). In this investigation, as in the preceding study, we focused on the change in[oxy-Hb] to evaluate hemodynamic responses.
For the measurement of [oxy-Hb], a 24-channel NIRS device (Hitachi ETG-4000, Hitachi Medical, Tokyo) that measures the absorption of near-infrared light at two wavelengths (760 nm and 840 nm) was utilized, and [oxy-Hb] was calculated using the aforementioned method. The optical topography (NIRS) device positioned a light source and a light-receiving sensor 3 cm apart on the head and measured the change in [oxy-Hb] concentration 2–3 cm below the scalp, corresponding to the depth of the cerebral cortex.
NIRS measurements were conducted with participants in a seated, relaxed position. To minimize motion artifacts, participants were carefully monitored and instructed to avoid bodily movements that could potentially induce artifacts, such as neck movement, clenching, and blinking. The NIRS probe was positioned over the prefrontal cortex, and the relative [oxy-Hb] concentration change was measured at 24 measurement points within an 8 × 8 cm area (Figure 1B). The middle row of probes was aligned with Fpz, and the bottom row with the Fp1-Fp2 line, in accordance with the international 10-20 system utilized in EEG measurements. The positions of the probes and measurement points were verified by superimposing them on a 3D reconstructed MRI scan of the cerebral cortex of a representative control subject (Figure 1C). A virtual registration method (58) was used to estimate the cortical area for each channel employed to estimate the cortical area for each channel.
This probe configuration facilitated the measurement of changes in [oxy-Hb] concentration within the cortical surface areas of the frontopolar and anterior PFC (Brodmann area [BA] 10), dorsolateral PFC (BA 9 and 46), and ventrolateral PFC (BA 44 and 45). Near-infrared light absorption was measured in real time with a temporal resolution of 0.1 s, and the data were analyzed utilizing the “integration mode”. The pre-task baseline was defined as the mean activity for 10 s immediately preceding the task, and the post-task baseline was defined as the mean activity for 25 s immediately following the task. Linear fitting was subsequently performed on the data between these two baselines (21, 27). A moving average method was employed to eliminate short-term movement artifacts (moving average window was 5 s).
NIRS results were analyzed by an examiner blinded to the participants’ diagnoses. Relative [oxy-Hb] was defined as the difference between [oxy-Hb] at baseline and during activation (Figure 2). The temporal profile of [oxy-Hb] change was evaluated from the initiation of the activation task until the [oxy-Hb] waveform returned to baseline. These values were averaged from three repeated trials to mitigate the effects of potential incidental changes and participant fatigue, consistent with previously described methodologies (21, 22, 27).
Figure 2. Grand average waveforms of changes in oxygenated hemoglobin (oxy-Hb) concentration change during the Stroop color-word task. Comparison of near-infrared spectroscopy (NIRS) waveforms (changes in oxy-Hb concentration) between the autism spectrum disorder (ASD) and control groups during the Stroop color-word task for each of the 24 recording channels. Red lines represent the autism spectrum disorder group, while blue lines represent the control group. The task was performed in the interval represented by green lines; the first green line indicates the beginning of the task and the second indicates the end. Significant region is shown in the red frame (Ch 20, 23, 24). Group differences were tested by multiple comparison test using the Bonferroni correction. P<0.05. Ch, channel.
We used the χ2 test to examine group differences for categorical variables (male and female). For normally distributed clinical variables, we used Student’s t-test. We conducted analyses of covariance to control for FIQ scores and sex, with the region of interest (1–24 ch) as within-subject factors and group (ASD, control) as a between-subjects factor for the averaged [oxy-Hb] and [deoxy-Hb] concentration data. We applied Bonferroni correction to adjust for multiple comparisons. Additionally, we conducted multiple linear stepwise regression analyses to determine the independent factors influencing the NIRS components. The independent variables utilized were the total score and five sub-scales of the AQ-J (social skills, attention switching, local details, communication, and imagination) for both the control and ASD groups, and ADOS-2 score for the ASD groups exclusively. We set statistical significance at p < 0.05. All statistical analyses were conducted using IBM SPSS Statistics version 28 (IBM Corp., Armonk, NY, USA).
Demographic characteristics are presented in Table 1. The participant groups differed in terms of sex (X2 = 8.469, p = 0.004) and FIQ score (t19 6 =4.732, p <0.001). However, there was no difference in average age (t196 = 0.584, p = 0.560). The ASD group had significantly higher scores on five sub-scales of the AQ-J (social skills, attention switching, local details, communication, and imagination) and the total score (t196 = -13.233, p <0.001; t196 = -7.874, p <0.001; t196 = -12.196, p <0.001; t196 = -4.105, p <0.001; t196 = -12.301, p <0.001; t196 = -6.857, p <0.001 respectively). There were significant differences in SCWC scores between the two groups (t196 = 5.181, p <0.001).
The grand average waveforms of [oxy-Hb] changes during the Stroop color word task in both groups are illustrated in Figure 2. We found significant between-group differences in the mean [oxy-Hb] changes during the task period in four channels (Ch-19: F1.4537 = 10.241, p = 0.033; Ch-20: F1.4537 = 20.370, p <0.001; Ch-23: F1.4537 = 19.134, p <0.001; Ch-24: F1.4537 = 22.350, p <0.001, Bonferroni-corrected) located near the orbitofrontal cortex and frontal pole (Table 2).
Table 2. Difference of mean oxygenated hemoglobin (oxy-Hb) measurements between task and post-task periods in 24 channels.
Multiple regression analyses in both the ASD and control groups revealed that the AQ-J total score significantly contributed to mean [oxy-Hb] changes during the Stroop color-word task at Ch-20 (β = -0.196, p = 0.043). Furthermore, AQ-J attention switching significantly contributed to mean [oxy-Hb] changes during the Stroop color-word task at Ch-20 (β = -0.255, p = 0.009) (Table 3). Conversely, the ADOS-2 score in the ASD group alone did not significantly contribute to mean [oxy-Hb] changes during the Stroop color-word task at any channel.
To the best of our knowledge, no previous studies have examined the broader prefrontal hemodynamic response in adults with ASD measured using 24-Ch NIRS during the Stroop color-word task. The results of the present study revealed that the [oxy-Hb] changes in 114 adults with ASD during the Stroop color-word task were significantly smaller than those in the PFC of 84 typically developing controls, particularly in the dorsolateral PFC (Ch-19, -20, -23, and -24) (Table 2).
The current findings corroborate our hypothesis and align with the proposed prefrontal cortex (PFC) dysfunction in ASD, as identified through various functional neuroimaging techniques, including fMRI and single-photon emission computed tomography (SPECT). SPECT studies on autism spectrum disorders have demonstrated a reduction in regional cerebral blood flow across various brain regions, including the insula, superior temporal gyrus, prefrontal cortex, cingulate gyrus, temporal lobe, and parietal lobe (59–61). A systematic review and meta-analysis of fMRI studies of autism spectrum disorders has indicated the PFC dysfunction (62). We previously measured hemodynamic responses in the PFC during a Stroop color-word task using 24-Ch NIRS in 12 drug-naive male children with ASD aged 7–15 years according to the DSM-5 criteria, and 12-year-old- and FIQ-matched typically developing control males. We found that oxyhemoglobin changes during the Stroop color-word task in the ASD group were significantly smaller than those in the control group in Ch-12 and -13, located over the bilateral dorsolateral PFC (35). In the present study, our findings indicate that at Ch-19, -20, -23, and -24, adults with ASD exhibited significantly smaller [oxy-Hb] changes compared to typically developing controls (Table 2). These channels were also localized in the orbitofrontal cortex and frontal pole, which differs from the findings of our previous study in children. The discrepancies between the results of these two studies may simply be due to differences in sample size between these studies. However, it is undeniable that the dysfunction of the PFC in individuals with ASD may be affected by age, and we need to give sufficient consideration to the longitudinal course of neuroanatomy in the central nervous system.
The developmental trajectory of human brain regions exhibits variability. The ontogenetic curve of areas responsible for higher cognitive functions, particularly executive functions, which are predominantly associated with the PFC, initiates later and extends for a longer duration compared to other cerebral regions (63–65). PFC plays a crucial role in higher cognitive functions, and certain developmental abnormalities occurring during the period from adolescence to early adulthood may be associated with the onset of psychiatric disorders, including ASD and schizophrenia (66, 67). This distinctive developmental process in the PFC correlates with the maturation of higher cognitive function during adolescence and early adulthood (63). For instance, an fMRI study utilizing an inhibition task related to self-regulation demonstrated that the blood oxygen level-dependent signal increased with age, from 9 to 11 years, but subsequently decreased progressively into adulthood, following the same inverse U-shaped trajectory as developmental changes in prefrontal gray matter volume (68, 69). Comprehension of this process may facilitate a thorough understanding of the discrepancies in results between the two studies.
The [oxy-Hb] changes at Ch-20 exhibited significant correlation with the AQ-J total and attention switching scores, which represent a symptom cluster of the AQ-J (Table 3). Ch-20 is anatomically localized to the PFC, specifically in proximity to the frontal pole, a cortical region situated at the anterior margin of the PFC. This area is characterized as the most tardily myelinated cortical region (70). The precise function of the frontal pole in higher cognitive processes remains to be elucidated. Nevertheless, extant research has indicated that the frontal pole is implicated in the concurrent processing of multiple tasks involving complex attentional functions (71, 72) and may play a significant role in attentional processes, including alternating attention. Individuals with ASD exhibit impaired attentional function, a cognitive deficit that suggests that diminished activity in the PFC, particularly in the frontal pole, during the Stroop color-word task in patients with ASD is associated with compromised attentional function.
Previous studies reported reduced PFC activity in patients with ASD during cognitive control tasks involving inhibition (73), attention (74, 75), and working memory (76, 77). In the present study, we used the Stroop color-word task because the PFC is one of the regions most strongly associated with Stroop interference (78). We previously reported PFC dysfunction during the Stroop color-word task in individuals with pediatric ASD, as measured by NIRS, compared to typically developing children (35). Our study incorporated a larger sample size than previous NIRS studies on ASD, indicating that NIRS measurements in adults with ASD could be a valuable instrument to aid in the clinical autism diagnostic process. The validity of our data was further strengthened by the inclusion criterion of an AQ-J (50–52) score of ≥26 to minimize diagnostic uncertainty in the ASD group.
This study presents several potential limitations. First, NIRS exhibits certain disadvantages compared to other functional neuroimaging techniques (79): for instance, NIRS only enables the measurement of Hb concentration changes as relative values. To address these potential issues, we employed the Stroop color-word task with a distinct baseline task. Furthermore, we measured Hb concentration changes between the activation and baseline tasks and conducted the task three times to average the potential effects of incidental changes and mitigate participant fatigue. The grand average waveforms of [oxy-Hb] concentration changes in the ASD group did not indicate a decrease in regional cerebral blood flow during the activation task or a difference in blood flow between baseline and activation tasks. Second, the spatial resolution for detecting hemodynamic responses from the scalp surface using NIRS is lower than that of fMRI, SPECT, and PET. However, abnormal prefrontal hemodynamic responses in individuals with ASD can be detected using NIRS. Third, several studies have shown that superficial hemodynamic responses such as skin blood flow can affect prefrontal NIRS hemoglobin signals (80, 81). Consequently, the present findings may have been influenced by skin blood flow. However, Sato et al. (82) conducted simultaneous NIRS, fMRI, and laser Doppler flowmeter measurements to determine whether prefrontal NIRS hemoglobin signals reflected cortical activity rather than superficial effects. They concluded that NIRS could be used to measure the hemodynamic signals originating from PFC activation. Fourth, the study included patients who were not drug naïve. The possibility that psychotropic drugs affect PFC function cannot be eliminated. Thus, our findings are not generalizable to all adults with ASD. Nevertheless, our current conclusion, is that abnormal prefrontal hemodynamic responses in adults with ASD are valuable for extending current knowledge. Further research is required to confirm these findings.
To the best of our knowledge, no previous studies have examined the broader prefrontal hemodynamic response in adults with ASD measured using 24-Ch NIRS during Stroop color-word task. We observed that the changes in [oxy-Hb] concentrations in the PFC were significantly lower in adults with ASD compared to typically developing controls. Furthermore, the changes in [oxy-Hb] concentrations at Ch-20 showed a significant correlation with the AQ-J total score and the attention switching score. These results demonstrate that adults with ASD may exhibit PFC dysfunction, and suggest that the reduced activity of the PFC, especially the frontal pole, in the Stroop color-word task is related to reduced attention function. Given that the multichannel NIRS system facilitates non-invasive functional mapping of the cerebral cortex and requires a substantially shorter measurement time (approximately 5 min) than other functional brain imaging methodologies, it may serve as an effective tool for ASD diagnosis.
The datasets presented in this study can be found in online repositories. The names of the repository/repositories and accession number(s) can be found in the article/supplementary material.
The studies involving humans were approved by the Institutional Review Board of Nara Medical University approved this study. The studies were conducted in accordance with the local legislation and institutional requirements. Written informed consent for participation in this study was provided by the participants’ legal guardians/next of kin.
KK: Data curation, Writing – original draft. KY: Conceptualization, Methodology, Writing – review & editing. RM: Data curation, Writing – review & editing. NK: Formal analysis, Writing – review & editing. RI: Data curation, Writing – review & editing. TO: Data curation, Writing – review & editing. NI: Methodology, Writing – review & editing. MM: Supervision, Writing – review & editing.
The author(s) declare financial support was received for the research, authorship, and/or publication of this article. This work was supported by Kanae Foundation for the Promotion of Medical Science; Pfizer Health Research Foundation; AMED-PRIME (grant number 21gm6310015h0002 to MM); AMED-CREST (grant number 22gm1510009h0001 to MM); AMED (grant number 21wm04250XXs0101 to MM); AMED (grant number 21uk1024002h0002 to MM); AMED-CREST (grant number 23gm1910004s0301); KAKENHI (Grant-in-Aid Transformative Research Areas 23H04173); AMED (grand number 21453798 to KY); KAKENHI (Grants-in-Aid for Scientific Research 20K15935 to KY); KAKENHI (Grant-in-Aid for Transformative Research Areas 21H05698 to KY).
We thank the participants for their valuable contribution in this study. The authors also express gratitude to NEC for providing the NEC Multi-Stim II equipment and for their skilled technical and methodological support.
The authors declare that the research was conducted in the absence of any commercial or financial relationships that could be construed as a potential conflict of interest.
The author(s) declared that they were an editorial board member of Frontiers, at the time of submission. This had no impact on the peer review process and the final decision.
The author(s) declare that no Generative AI was used in the creation of this manuscript.
All claims expressed in this article are solely those of the authors and do not necessarily represent those of their affiliated organizations, or those of the publisher, the editors and the reviewers. Any product that may be evaluated in this article, or claim that may be made by its manufacturer, is not guaranteed or endorsed by the publisher.
1. American Psychiatric Association. Diagnostic and statistical manual of mental disorders. 5th ed. Arlington: American Psychiatric Association (2013).
2. Hughes C, Russell J, Robbins TW. Evidence for executive dysfunction in autism. Neuropsychologia. (1994) 32:477–92. doi: 10.1016/0028-3932(94)90092-2
3. Hill EL. Evaluating the theory of executive dysfunction in autism. Dev Rev. (2004) 24:189–233. doi: 10.1016/j.dr.2004.01.001
4. Demetriou EA, Lampit A, Quintana DS, Naismith SL, Song YJC, Pye JE, et al. Autism spectrum disorders: a meta-analysis of executive function. Mol Psychiatry. (2018) 23:1198–204. doi: 10.1038/mp.2017.75
5. McLean RL, Johnson Harrison A, Zimak E, Joseph RM, Morrow EM. Executive function in probands with autism with average IQ and their unaffected first-degree relatives. J Am Acad Child Adolesc Psychiatry. (2014) 53:1001–9. doi: 10.1016/j.jaac.2014.05.019
6. Friedman NP, Miyake A, Young SE, DeFries JC, Corley RP, Hewitt JK. Individual differences in executive functions are almost entirely genetic in origin. J Exp Psychol Gen. (2008) 137:201–25. doi: 10.1037/0096-3445.137.2.201
7. Miyake A, Friedman NP, Emerson MJ, Witzki AH, Howerter A, Wager TD. The unity and diversity of executive functions and their contributions to complex “Frontal Lobe” tasks: a latent variable analysis. Cognit Psychol. (2000) 41:49–100. doi: 10.1006/cogp.1999.0734
8. Stuss DT, Floden D, Alexander MP, Levine B, Katz D. Stroop performance in focal lesion patients: dissociation of processes and frontal lobe lesion location. Neuropsychologia. (2001) 39:771–86. doi: 10.1016/s0028-3932(01)00013-6
9. Perret E. The left frontal lobe of man and the suppression of habitual responses in verbal categorical behaviour. Neuropsychologia. (1974) 12:323–30. doi: 10.1016/0028-3932(74)90047-5
10. Zysset S, Müller K, Lohmann G, von Cramon DY. Color-word matching stroop task: separating interference and response conflict. Neuroimage. (2001) 13:29–36. doi: 10.1006/nimg.2000.0665
11. Strangman G, Boas DA, Sutton JP. Non-invasive neuroimaging using near-infrared light. Biol Psychiatry. (2002) 52:679–93. doi: 10.1016/s0006-3223(02)01550-0
12. Boas DA, Dale AM, Franceschini MA. Diffuse optical imaging of brain activation: approaches to optimizing image sensitivity, resolution, and accuracy. Neuroimage. (2004) 23 Suppl 1:S275–288. doi: 10.1016/j.neuroimage.2004.07.011
13. Obrig H, Villringer A. Beyond the visible–imaging the human brain with light. J Cereb Blood Flow Metab. (2003) 23:1–18. doi: 10.1097/01.WCB.0000043472.45775.29
14. Villringer K, Minoshima S, Hock C, Obrig H, Ziegler S, Dirnagl U, et al. Assessment of local brain activation. A simultaneous PET and near-infrared spectroscopy study. Adv Exp Med Biol. (1997) 413:149–53. doi: 10.1007/978-1-4899-0056-2
15. Ohmae E, Ouchi Y, Oda M, Suzuki T, Nobesawa S, Kanno T, et al. Cerebral hemodynamics evaluation by near-infrared time-resolved spectroscopy: correlation with simultaneous positron emission tomography measurements. Neuroimage. (2006) 29:697–705. doi: 10.1016/j.neuroimage.2005.08.008
16. Suto T, Fukuda M, Ito M, Uehara T, Mikuni M. Multichannel near-infrared spectroscopy in depression and schizophrenia: cognitive brain activation study. Biol Psychiatry. (2004) 55:501–11. doi: 10.1016/j.biopsych.2003.09.008
17. Kubota Y, Toichi M, Shimizu M, Mason RA, Coconcea CM, Findling RL, et al. Prefrontal activation during verbal fluency tests in schizophrenia–a near-infrared spectroscopy (NIRS) study. Schizophr Res. (2005) 77:65–73. doi: 10.1016/j.schres.2005.01.007
18. Shinba T, Nagano M, Kariya N, Ogawa K, Shinozaki T, Shimosato S, et al. Near-infrared spectroscopy analysis of frontal lobe dysfunction in schizophrenia. Biol Psychiatry. (2004) 55:154–64. doi: 10.1016/s0006-3223(03)00547-x
19. Yamamuro K, Kimoto S, Iida J, Kishimoto N, Tanaka S, Toritsuka M, et al. Distinct patterns of blood oxygenation in the prefrontal cortex in clinical phenotypes of schizophrenia and bipolar disorder. J Affect Disord. (2018) 234:45–53. doi: 10.1016/j.jad.2018.02.065
20. Kameyama M, Fukuda M, Yamagishi Y, Sato T, Uehara T, Ito M, et al. Frontal lobe function in bipolar disorder: a multichannel near-infrared spectroscopy study. Neuroimage. (2006) 29:172–84. doi: 10.1016/j.neuroimage.2005.07.025
21. Okada K, Ota T, Iida J, Kishimoto N, Kishimoto T. Lower prefrontal activity in adults with obsessive-compulsive disorder as measured by near-infrared spectroscopy. Prog Neuropsychopharmacol Biol Psychiatry. (2013) 43:7–13. doi: 10.1016/j.pnpbp.2012.11.013
22. Ota T, Iida J, Sawada M, Suehiro Y, Yamamuro K, Matsuura H, et al. Reduced prefrontal hemodynamic response in pediatric obsessive-compulsive disorder as measured by near-infrared spectroscopy. Child Psychiatry Hum Dev. (2013) 44:265–77. doi: 10.1007/s10578-012-0323-0
23. Yamamuro K, Ota T, Iida J, Kishimoto N, Nakanishi Y, Kishimoto T. Persistence of impulsivity in pediatric and adolescent patients with obsessive-compulsive disorder. Psychiatry Clin Neurosci. (2017) 71:36–43. doi: 10.1111/pcn.12465
24. Fallgatter AJ, Roesler M, Sitzmann L, Heidrich A, Mueller TJ, Strik WK. Loss of functional hemispheric asymmetry in Alzheimer’s dementia assessed with near-infrared spectroscopy. Brain Res Cognit Brain Res. (1997) 6:67–72. doi: 10.1016/s0926-6410(97)00016-5
25. Hock C, Villringer K, Müller-Spahn F, Wenzel R, Heekeren H, Schuh-Hofer S, et al. Decrease in parietal cerebral hemoglobin oxygenation during performance of a verbal fluency task in patients with Alzheimer’s disease monitored by means of near-infrared spectroscopy (NIRS)–correlation with simultaneous rCBF-PET measurements. Brain Res. (1997) 755:293–303. doi: 10.1016/s0006-8993(97)00122-4
26. Matsuo K, Kato T, Taneichi K, Matsumoto A, Ohtani T, Hamamoto T, et al. Activation of the prefrontal cortex to trauma-related stimuli measured by near-infrared spectroscopy in posttraumatic stress disorder due to terrorism. Psychophysiology. (2003) 40:492–500. doi: 10.1111/1469-8986.00051
27. Yamamuro K, Ota T, Iida J, Nakanishi Y, Uratani M, Matsuura H, et al. Prefrontal dysfunction in pediatric Tourette’s disorder as measured by near-infrared spectroscopy. BMC Psychiatry. (2015) 15:102. doi: 10.1186/s12888-015-0472-3
28. Ehlis A-C, Bähne CG, Jacob CP, Herrmann MJ, Fallgatter AJ. Reduced lateral prefrontal activation in adult patients with attention-deficit/hyperactivity disorder (ADHD) during a working memory task: a functional near-infrared spectroscopy (fNIRS) study. J Psychiatr Res. (2008) 42:1060–7. doi: 10.1016/j.jpsychires.2007.11.011
29. Weber P, Lütschg J, Fahnenstich H. Cerebral hemodynamic changes in response to an executive function task in children with attention-deficit hyperactivity disorder measured by near-infrared spectroscopy. J Dev Behav Pediatr. (2005) 26:105–11. doi: 10.1097/00004703-200504000-00005
30. Inoue Y, Sakihara K, Gunji A, Ozawa H, Kimiya S, Shinoda H, et al. Reduced prefrontal hemodynamic response in children with ADHD during the Go/NoGo task: a NIRS study. Neuroreport. (2012) 23:55–60. doi: 10.1097/WNR.0b013e32834e664c
31. Negoro H, Sawada M, Iida J, Ota T, Tanaka S, Kishimoto T. Prefrontal dysfunction in attention-deficit/hyperactivity disorder as measured by near-infrared spectroscopy. Child Psychiatry Hum Dev. (2010) 41:193–203. doi: 10.1007/s10578-009-0160-y
32. Yamamuro K, Kimoto S, Iida J, Kishimoto N, Nakanishi Y, Tanaka S, et al. Reduced prefrontal cortex hemodynamic response in adults with methamphetamine induced psychosis: relevance for impulsivity. PloS One. (2016) 11:e0152373. doi: 10.1371/journal.pone.0152373
33. Yamamuro K, Makinodan M, Kimoto S, Kishimoto N, Morimoto T, Toritsuka M, et al. Differential patterns of blood oxygenation in the prefrontal cortex between patients with methamphetamine-induced psychosis and schizophrenia. Sci Rep. (2015) 5:12107. doi: 10.1038/srep12107
34. Kuwabara H, Kasai K, Takizawa R, Kawakubo Y, Yamasue H, Rogers MA, et al. Decreased prefrontal activation during letter fluency task in adults with pervasive developmental disorders: a near-infrared spectroscopy study. Behav Brain Res. (2006) 172:272–7. doi: 10.1016/j.bbr.2006.05.020
35. Uratani M, Ota T, Iida J, Okazaki K, Yamamuro K, Nakanishi Y, et al. Reduced prefrontal hemodynamic response in pediatric autism spectrum disorder measured with near-infrared spectroscopy. Child Adolesc Psychiatry Ment Health. (2019) 13:29. doi: 10.1186/s13034-019-0289-9
36. Pellicano E. The development of executive function in autism. Autism Res Treat. (2012) 2012:146132. doi: 10.1155/2012/146132
37. Craig F, Margari F, Legrottaglie AR, Palumbi R, de Giambattista C, Margari L. A review of executive function deficits in autism spectrum disorder and attention-deficit/hyperactivity disorder. Neuropsychiatr Dis Treat. (2016) 12:1191–202. doi: 10.2147/NDT.S104620
38. Leung RC, Zakzanis KK. Brief report: cognitive flexibility in autism spectrum disorders: a quantitative review. J Autism Dev Disord. (2014) 44:2628–45. doi: 10.1007/s10803-014-2136-4
39. Hill EL. Executive dysfunction in autism. Trends Cognit Sci. (2004) 8:26–32. doi: 10.1016/j.tics.2003.11.003
40. Kenworthy L, Yerys BE, Anthony LG, Wallace GL. Understanding executive control in autism spectrum disorders in the lab and in the real world. Neuropsychol Rev. (2008) 18:320–38. doi: 10.1007/s11065-008-9077-7
41. Rabin LA, Barr WB, Burton LA. Assessment practices of clinical neuropsychologists in the United States and Canada: a survey of INS, NAN, and APA Division 40 members. Arch Clin Neuropsychol. (2005) 20:33–65. doi: 10.1016/j.acn.2004.02.005
42. Wecker NS, Kramer JH, Wisniewski A, Delis DC, Kaplan E. Age effects on executive ability. Neuropsychology. (2000) 14:409–14. doi: 10.1037//0894-4105.14.3.409
43. Egner T, Hirsch J. The neural correlates and functional integration of cognitive control in a Stroop task. Neuroimage. (2005) 24:539–47. doi: 10.1016/j.neuroimage.2004.09.007
44. Harrison BJ, Shaw M, Yücel M, Purcell R, Brewer WJ, Strother SC, et al. Functional connectivity during Stroop task performance. Neuroimage. (2005) 24:181–91. doi: 10.1016/j.neuroimage.2004.08.033
45. Strauss E, Sherman EMS, Spreen O. A compendium of neuropsychological tests: Administration, norms, and commentary. 3rd ed Vol. xvii. New York, NY, US: Oxford University Press (2006). 1216 p.
46. Yasumura A, Kokubo N, Yamamoto H, Yasumura Y, Nakagawa E, Kaga M, et al. Neurobehavioral and hemodynamic evaluation of Stroop and reverse Stroop interference in children with attention-deficit/hyperactivity disorder. Brain Dev. (2014) 36:97–106. doi: 10.1016/j.braindev.2013.01.005
47. Xiao T, Xiao Z, Ke X, Hong S, Yang H, Su Y, et al. Response inhibition impairment in high functioning autism and attention deficit hyperactivity disorder: evidence from near-infrared spectroscopy data. PloS One. (2012) 7:e46569. doi: 10.1371/journal.pone.0046569
48. Lord G, Rutter M, DiLavore PG, Risi S, Gotham K, Bishop SL. Autism Diagnostic Observation Schedule. 2nd Edition(ADOS-2. Los Angeles, CA: Western Psychological Services (2012).
49. Otsubo T, Tanaka K, Koda R, Shinoda J, Sano N, Tanaka S, et al. Reliability and validity of Japanese version of the Mini-International Neuropsychiatric Interview. Psychiatry Clin Neurosci. (2005) 59:517–26. doi: 10.1111/j.1440-1819.2005.01408.x
50. Baron-Cohen S, Wheelwright S, Skinner R, Martin J, Clubley E. The autism-spectrum quotient (AQ): evidence from Asperger syndrome/high-functioning autism, males and females, scientists and mathematicians. J Autism Dev Disord. (2001) 31:5–17. doi: 10.1023/a:1005653411471
51. Baron-Cohen S, Hoekstra RA, Knickmeyer R, Wheelwright S. The autism-spectrum quotient (AQ)–adolescent version. J Autism Dev Disord. (2006) 36:343–50. doi: 10.1007/s10803-006-0073-6
52. Auyeung B, Baron-Cohen S, Wheelwright S, Allison C. The autism spectrum quotient: children’s version (AQ-child). J Autism Dev Disord. (2008) 38:1230–40. doi: 10.1007/s10803-007-0504-z
53. Wakabayashi A, Tojo Y, Baron-Cohen S, Wheelwright S. The Autism-Spectrum Quotient (AQ) Japanese version: evidence from high-functioning clinical group and normal adults. Shinrigaku Kenkyu. (2004) 75:78–84. doi: 10.4992/jjpsy.75.78
54. Golden CJ. A group version of the Stroop Color and Word Test. J Pers Assess. (1975) 39:386–8. doi: 10.1207/s15327752jpa3904_10
55. Kleinschmidt A, Obrig H, Requardt M, Merboldt KD, Dirnagl U, Villringer A, et al. Simultaneous recording of cerebral blood oxygenation changes during human brain activation by magnetic resonance imaging and near-infrared spectroscopy. J Cereb Blood Flow Metab. (1996) 16:817–26. doi: 10.1097/00004647-199609000-00006
56. Toronov V, Webb A, Choi JH, Wolf M, Michalos A, Gratton E, et al. Investigation of human brain hemodynamics by simultaneous near-infrared spectroscopy and functional magnetic resonance imaging. Med Phys. (2001) 28:521–7. doi: 10.1118/1.1354627
57. Hoshi Y, Kobayashi N, Tamura M. Interpretation of near-infrared spectroscopy signals: a study with a newly developed perfused rat brain model. J Appl Physiol (1985). (2001) 90:1657–62. doi: 10.1152/jappl.2001.90.5.1657
58. Tsuzuki D, Jurcak V, Singh AK, Okamoto M, Watanabe E, Dan I. Virtual spatial registration of stand-alone fNIRS data to MNI space. Neuroimage. (2007) 34:1506–18. doi: 10.1016/j.neuroimage.2006.10.043
59. Ohnishi T, Matsuda H, Hashimoto T, Kunihiro T, Nishikawa M, Uema T, et al. Abnormal regional cerebral blood flow in childhood autism. Brain. (2000) 123:1838–44. doi: 10.1093/brain/123.9.1838
60. Makkonen I, Riikonen R, Kokki H, Airaksinen MM, Kuikka JT. Serotonin and dopamine transporter binding in children with autism determined by SPECT. Dev Med Child Neurol. (2008) 50:593–7. doi: 10.1111/j.1469-8749.2008.03027.x
61. Murphy DGM, Daly E, Schmitz N, Toal F, Murphy K, Curran S, et al. Cortical serotonin 5-HT2A receptor binding and social communication in adults with Asperger’s syndrome: an in vivo SPECT study. Am J Psychiatry. (2006) 163:934–6. doi: 10.1176/ajp.2006.163.5.934
62. Philip RCM, Dauvermann MR, Whalley HC, Baynham K, Lawrie SM, Stanfield AC. A systematic review and meta-analysis of the fMRI investigation of autism spectrum disorders. Neurosci Biobehav Rev. (2012) 36:901–42. doi: 10.1016/j.neubiorev.2011.10.008
63. Tsujimoto S. The prefrontal cortex: functional neural development during early childhood. Neuroscientist. (2008) 14:345–58. doi: 10.1177/1073858408316002
64. Huttenlocher PR, Dabholkar AS. Regional differences in synaptogenesis in human cerebral cortex. J Comp Neurol. (1997) 387:167–78. doi: 10.1002/(sici)1096-9861(19971020)387:2<167::aid-cne1>3.0.co;2-z
65. Huttenlocher PR. Synaptic density in human frontal cortex - developmental changes and effects of aging. Brain Res. (1979) 163:195–205. doi: 10.1016/0006-8993(79)90349-4
66. Dumontheil I, Burgess PW, Blakemore S-J. Development of rostral prefrontal cortex and cognitive and behavioural disorders. Dev Med Child Neurol. (2008) 50:168–81. doi: 10.1111/j.1469-8749.2008.02026.x
67. Gilbert SJ, Spengler S, Simons JS, Steele JD, Lawrie SM, Frith CD, et al. Functional specialization within rostral prefrontal cortex (area 10): a meta-analysis. J Cognit Neurosci. (2006) 18:932–48. doi: 10.1162/jocn.2006.18.6.932
68. Booth JR, Burman DD, Meyer JR, Lei Z, Trommer BL, Davenport ND, et al. Neural development of selective attention and response inhibition. Neuroimage. (2003) 20:737–51. doi: 10.1016/S1053-8119(03)00404-X
69. Giedd JN, Blumenthal J, Jeffries NO, Castellanos FX, Liu H, Zijdenbos A, et al. Brain development during childhood and adolescence: a longitudinal MRI study. Nat Neurosci. (1999) 2:861–3. doi: 10.1038/13158
71. Gilbert SJ, Williamson IDM, Dumontheil I, Simons JS, Frith CD, Burgess PW. Distinct regions of medial rostral prefrontal cortex supporting social and nonsocial functions. Soc Cognit Affect Neurosci. (2007) 2:217–26. doi: 10.1093/scan/nsm014
72. Koechlin E, Basso G, Pietrini P, Panzer S, Grafman J. The role of the anterior prefrontal cortex in human cognition. Nature. (1999) 399:148–51. doi: 10.1038/20178
73. Kana RK, Keller TA, Minshew NJ, Just MA. Inhibitory control in high-functioning autism: decreased activation and underconnectivity in inhibition networks. Biol Psychiatry. (2007) 62:198–206. doi: 10.1016/j.biopsych.2006.08.004
74. Silk TJ, Rinehart N, Bradshaw JL, Tonge B, Egan G, O’Boyle MW, et al. Visuospatial processing and the function of prefrontal-parietal networks in autism spectrum disorders: a functional MRI study. Am J Psychiatry. (2006) 163:1440–3. doi: 10.1176/ajp.2006.163.8.1440
75. Christakou A, Murphy CM, Chantiluke K, Cubillo AI, Smith AB, Giampietro V, et al. Disorder-specific functional abnormalities during sustained attention in youth with Attention Deficit Hyperactivity Disorder (ADHD) and with autism. Mol Psychiatry. (2013) 18:236–44. doi: 10.1038/mp.2011.185
76. Di Martino A, Ross K, Uddin LQ, Sklar AB, Castellanos FX, Milham MP. Functional brain correlates of social and nonsocial processes in autism spectrum disorders: an activation likelihood estimation meta-analysis. Biol Psychiatry. (2009) 65:63–74. doi: 10.1016/j.biopsych.2008.09.022
77. Stigler KA, McDonald BC, Anand A, Saykin AJ, McDougle CJ. Structural and functional magnetic resonance imaging of autism spectrum disorders. Brain Res. (2011) 1380:146–61. doi: 10.1016/j.brainres.2010.11.076
78. Laird AR, McMillan KM, Lancaster JL, Kochunov P, Turkeltaub PE, Pardo JV, et al. A comparison of label-based review and ALE meta-analysis in the Stroop task. Hum Brain Mapp. (2005) 25:6–21. doi: 10.1002/hbm.20129
79. Yamashita Y, Maki A, Koizumi H. Near-infrared topographic measurement system : Imaging of absorbers localized in a scattering medium. Rev Sci Instrum. (1996) 67:730/732. doi: 10.1063/1.1146849
80. Takahashi T, Takikawa Y, Kawagoe R, Shibuya S, Iwano T, Kitazawa S. Influence of skin blood flow on near-infrared spectroscopy signals measured on the forehead during a verbal fluency task. Neuroimage. (2011) 57:991–1002. doi: 10.1016/j.neuroimage.2011.05.012
81. Kirilina E, Jelzow A, Heine A, Niessing M, Wabnitz H, Brühl R, et al. The physiological origin of task-evoked systemic artefacts in functional near infrared spectroscopy. Neuroimage. (2012) 61:70–81. doi: 10.1016/j.neuroimage.2012.02.074
Keywords: near-infrared spectroscopy, autism spectrum disorder, prefrontal cortex, hemodynamic responses, stroop color‑word task
Citation: Kamikawa K, Yamamuro K, Mizui R, Kashida N, Ishida R, Okada T, Iwata N and Makinodan M (2025) Reduced prefrontal hemodynamic responses measured using near-infrared spectroscopy in adults with autism spectrum disorder. Front. Psychiatry 15:1507890. doi: 10.3389/fpsyt.2024.1507890
Received: 08 October 2024; Accepted: 04 December 2024;
Published: 06 January 2025.
Edited by:
Sabri Hergüner, Ankara Autism Center, TürkiyeReviewed by:
Alessandro Crippa, Eugenio Medea (IRCCS), ItalyCopyright © 2025 Kamikawa, Yamamuro, Mizui, Kashida, Ishida, Okada, Iwata and Makinodan. This is an open-access article distributed under the terms of the Creative Commons Attribution License (CC BY). The use, distribution or reproduction in other forums is permitted, provided the original author(s) and the copyright owner(s) are credited and that the original publication in this journal is cited, in accordance with accepted academic practice. No use, distribution or reproduction is permitted which does not comply with these terms.
*Correspondence: Kazuhiko Yamamuro, bXVyb0BuYXJhbWVkLXUuYWMuanA=
Disclaimer: All claims expressed in this article are solely those of the authors and do not necessarily represent those of their affiliated organizations, or those of the publisher, the editors and the reviewers. Any product that may be evaluated in this article or claim that may be made by its manufacturer is not guaranteed or endorsed by the publisher.
Research integrity at Frontiers
Learn more about the work of our research integrity team to safeguard the quality of each article we publish.