- 1School of Computer Science and Engineering, Xi’an Technological University, Xi’an, China
- 2School of Life Science and Technology, Xi’an Jiaotong University, Xi’an, China
- 3Football school of Xi’an Physical Education University, Xi’an, China
Background: Inhibitory control deficits are a core feature of cognitive impairment in schizophrenia, associated with abnormal activation of key brain networks. Repetitive transcranial magnetic stimulation (rTMS) targeting the dorsolateral prefrontal cortex (DLPFC) may help improve inhibitory control, but its specific effects in schizophrenia remain uncertain.
Methods: This study involved 150 participants divided into Real-rTMS, Sham-rTMS, and healthy control groups. Inhibitory control was assessed using the dual-choice oddball task, and task-based functional magnetic resonance imaging (fMRI) was employed to examine neural activity. The Real-rTMS group received active stimulation over the DLPFC, and the Sham group received placebo stimulation.
Results: The Real-rTMS group exhibited significant improvements in both reaction times and accuracy compared to the Sham group, indicating enhanced inhibitory control. fMRI data showed that brain activity in regions such as the cerebellum, insula, thalamus, and prefrontal cortex was normalized in the Real-rTMS group, with activation patterns closely resembling those observed in healthy controls. Additionally, task-based fMRI revealed a restoration and further enhancement of negative activation in regions like the middle frontal gyrus and superior temporal gyrus, which helped reduce cognitive interference from irrelevant stimuli.
Conclusion: rTMS targeting the DLPFC improves inhibitory control in schizophrenia by modulating both positive and negative brain activation patterns. These findings highlight the dual mechanism through which rTMS enhances cognitive control, offering a promising intervention for cognitive deficits in schizophrenia. Future research should explore the long-term effects of this modulation on broader cognitive functions.
1 Introduction
Schizophrenia (SCZ) is a chronic mental illness characterized by a wide range of cognitive, emotional, and behavioral disturbances (1, 2). Cognitive impairments, particularly deficits in inhibitory control, are among the most debilitating symptoms and significantly contribute to the functional impairments observed in patients (3, 4). Inhibitory control, the ability to suppress irrelevant or inappropriate responses, is crucial for goal-directed behavior, decision-making, and maintaining attention (5, 6). In schizophrenia, deficits in this area are associated with abnormal activity and connectivity within key brain networks, such as the default mode network (DMN) and the executive control network (7, 8). This dysfunction makes it difficult for individuals with schizophrenia to filter irrelevant information, resulting in cognitive overload, impaired judgment, and difficulties in completing daily tasks (8).
Addressing cognitive deficits, particularly inhibitory control, is essential for improving overall cognitive functioning and quality of life for patients with schizophrenia (9). Traditional pharmacological treatments, while effective in alleviating positive symptoms such as hallucinations and delusions, have shown limited efficacy in treating cognitive impairments (10, 11). This has led to increased interest in non-invasive neuromodulation techniques, such as repetitive transcranial magnetic stimulation (rTMS), as potential therapeutic interventions for cognitive deficits in schizophrenia (12).
rTMS is a non-invasive brain stimulation technique that uses magnetic fields to modulate neural activity in specific brain regions (13, 14). Over the past decade, rTMS has gained attention for its potential to enhance cognitive function in both healthy individuals and patients with neuropsychiatric disorders (14–16). In schizophrenia, rTMS has been explored primarily for its effects on negative symptoms and mood disorders, but growing evidence suggests it may also have a positive impact on cognitive deficits, including working memory, attention, and executive function (17, 18). However, despite its promise, the specific effects of rTMS on inhibitory control in schizophrenia remain underexplored, with mixed results reported across studies (19–21). Further research is needed to clarify whether rTMS can reliably improve inhibitory control in this population and to understand the neural mechanisms underlying such improvements.
The dorsolateral prefrontal cortex (DLPFC) has been identified as a critical region for cognitive control, including inhibitory control and working memory (22–24). rTMS targeting the DLPFC has been shown to modulate neural activity and improve cognitive performance in several clinical populations, including patients with depression, addiction, and neurodegenerative diseases (25–27). Given the role of the DLPFC in cognitive processing, it is hypothesized that rTMS applied to this region may help normalize brain function and enhance inhibitory control in schizophrenia (28). Studies involving other populations, such as those with substance use disorders, have demonstrated improvements in impulse control following rTMS, further supporting its potential application in schizophrenia (29, 30). Nevertheless, the precise neural mechanisms by which rTMS may influence inhibitory control in schizophrenia remain to be fully elucidated.
In this study, we aim to investigate the effects of rTMS on inhibitory control in patients with schizophrenia, focusing on both behavioral and neural mechanisms. We hypothesize that rTMS applied to the DLPFC will lead to significant improvements in inhibitory control, as measured by the dual-choice oddball task, a robust and widely used paradigm for assessing response inhibition (31). By comparing the performance of patients receiving real-rTMS with those receiving sham treatment, we aim to clarify whether rTMS can effectively enhance inhibitory control in this population. Additionally, we expect that these behavioral improvements in the Real group will be accompanied by changes in neural activity in regions associated with executive function, as measured by functional magnetic resonance imaging (fMRI). By comparing the Real and Sham groups, this study seeks to clarify the potential of rTMS as a targeted intervention for improving inhibitory control in schizophrenia and contribute to a deeper understanding of the neural mechanisms underlying these cognitive improvements.
2 Materials and methods
2.1 Participants
We enrolled 150 participants, with 50 in each group: Real-SCZ, Sham-SCZ, and healthy control (HC). All patients were at their first episode of schizophrenia and were diagnosed according to DSM-5 criteria (32). The two schizophrenia groups were matched for baseline clinical indicators and symptoms, with no significant differences between them. All patients were within two weeks of their initial exposure to antipsychotic treatment, with antipsychotic dosages converted to olanzapine equivalents using the Defined Daily Dose (DDD) system (33).
The HC group was recruited through community advertisements and screened to exclude any history of psychiatric or neurological disorders. All participants were right-handed, had normal or corrected-to-normal vision, and were free from concurrent neurological or severe systemic illnesses. Exclusion criteria for all participants included a history of traumatic brain injury, substance use disorders, or contraindications to MRI. Both groups excluded individuals with an intelligence quotient (IQ) below 70, estimated by the Wechsler Abbreviated Scale of Intelligence (34). The study was approved by the local Research Ethics Committee and conducted in accordance with the Declaration of Helsinki. Written informed consent was obtained from all participants prior to the commencement of the study. The study was conducted from January 2023 to March 2024. This period included all phases of the study: participant recruitment, data collection, and the subsequent analysis of behavioral and neuroimaging data. The detailed experimental design can be seen in Figure 1.
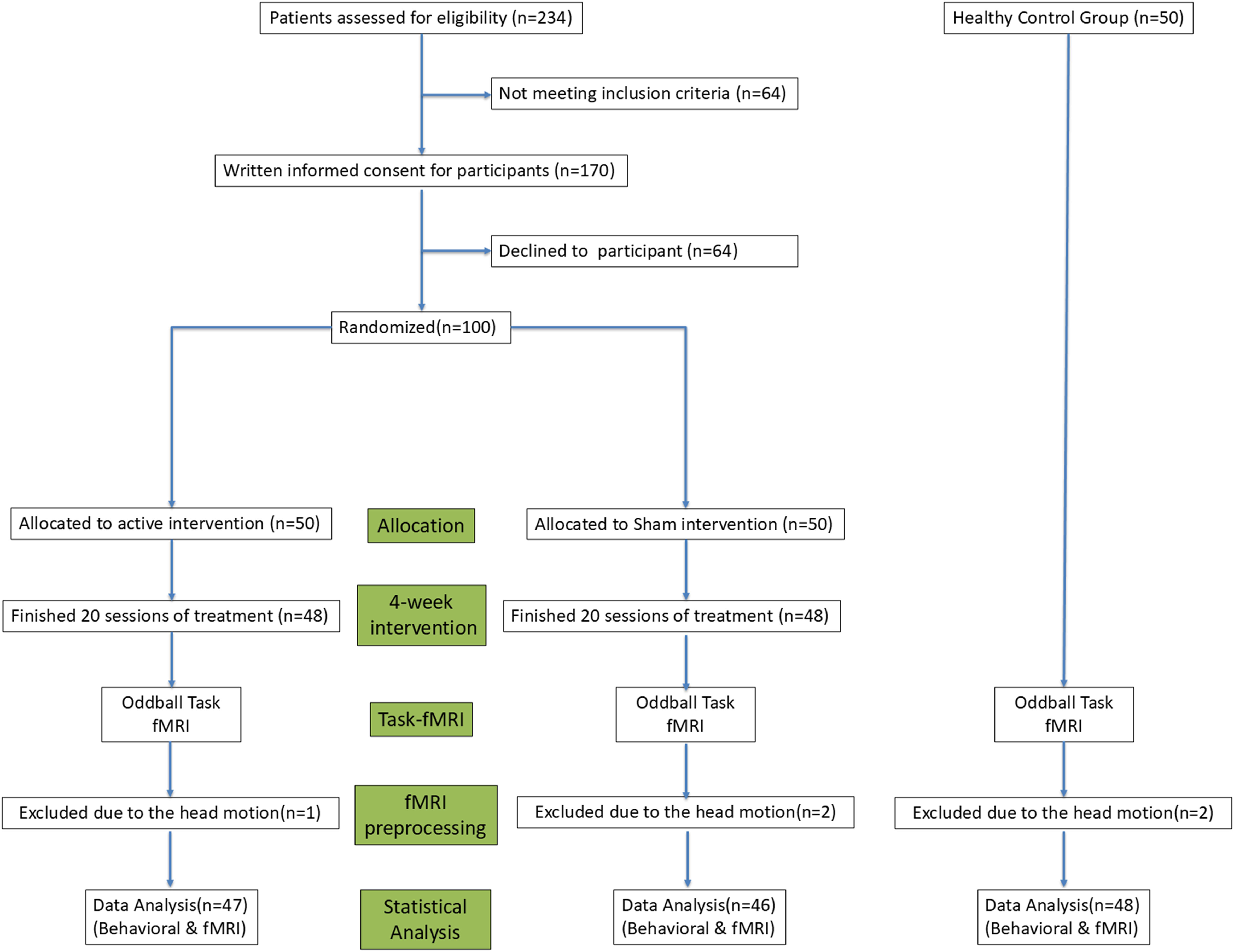
Figure 1. A flowchart outlining the experimental design of Experiment, detailing participant grouping, intervention protocols, and outcome measures.
2.2 Double-choice oddball task
The dual-choice oddball task requires participants to respond to two types of stimuli. One stimulus appears with a higher probability, approximately 85%, which is represented by the letter “W” in this experiment. The other stimulus appears with a lower probability, around 15%, and is represented by the letter “M” in this experiment. These stimuli are presented in random order, making the low-probability stimulus appear unexpectedly to the participant. The task requires participants to press the left key on the keyboard when they see the letter “W” and the right key when they see the letter “M.”
2.3 rTMS treatments
Participants received rTMS modulation over the left dorsolateral prefrontal cortex (DLPFC) according to the following protocol. Each rTMS session involved the delivery of 30 trains of TMS pulses at 10 Hz for 10 seconds (100 pulses per train) with a 20-second inter-train interval, resulting in a total of 3000 pulses per session for a total duration of 15 minutes. The stimulation intensity was set at 80% of the resting motor threshold, which was determined as the minimal intensity of stimulation that evoked an electromyographic response of 550 mV in the first dorsal interosseous muscle of the hand contralateral to the stimulated hemisphere in at least 5 out of 10 trials. For individualized stimulation (35), the MNI coordinates of the left DLPFC (approximately (–26, 38, 44), based on prior studies targeting this region) were adjusted for each participant using the inverse normalization method embedded in SPM12 (i.e., normalization based on the inverse deformation field). All rTMS sessions were guided by a neuronavigation system to ensure precise targeting. Once the location of the stimulation target was determined, the coordinates were entered into the neuronavigation system, which provided continuous guidance throughout the sessions, ensuring precise and consistent delivery of stimulation to the designated cortical area. For the Sham group, the rTMS coil was positioned at the same MNI coordinates of the left DLPFC as in the Real group, and the neuronavigation system was used to guide the coil placement. However, the coil was angled at 90 degrees away from the scalp, preventing direct brain stimulation while still producing auditory clicks and scalp sensations similar to the Real rTMS treatment. This setup ensured that participants in the Sham group experienced sensory feedback comparable to the Real group, such as the sound of the TMS pulses and the mild scalp sensations, without receiving active stimulation to the brain. This approach was used to maintain blinding of the participants and minimize placebo effects.
2.4 Task-based fMRI data acquisition and processing
Functional MRI data were collected during the oddball task using a Philips 3-Tesla MRI scanner with a 32-channel head coil. BOLD (Blood Oxygen Level Dependent) signals were acquired using an echo-planar imaging (EPI) sequence with the following parameters: repetition time (TR) = 2000 ms, echo time (TE) = 30 ms, flip angle = 90°, field of view (FOV) = 220 mm × 220 mm, slice thickness = 3 mm, and 45 axial slices covering the entire brain. Each fMRI session included both task and rest periods. Preprocessing of fMRI data was conducted using SPM12 (Statistical Parametric Mapping). The preprocessing steps included discarding the first 10 time points, slice timing correction, realignment, normalization to the MNI template, and spatial smoothing with a 6 mm full-width at half-maximum (FWHM) Gaussian kernel.
2.5 Task-based fMRI data analysis
The fMRI data were analyzed using a mixed-effects model in a two-stage process. In the first stage, the stimulus sequence corresponding to letter presentations was organized into distinct blocks and convolved with the hemodynamic response function (HRF). A general linear model (GLM) was then applied to identify brain regions showing significantly higher or lower activity in response to the presentation of the letter “M” compared to the control condition (“W”). To minimize the effects of head motion on the results, six motion parameters derived from the preprocessing stage were included in the GLM.
In the second stage, several steps were followed. First, for each experimental condition (HC, Real, and Sham), one-sample t-tests were performed to examine the overall brain activation patterns elicited by the dual-choice oddball task. Second, a one-way ANOVA was conducted to assess significant differences in brain activation patterns across the three experimental conditions. Post-hoc tests were performed to further explore pairwise comparisons between the conditions. Lastly, brain activation values were extracted from regions showing significant differences between the three groups, and detailed charts were generated to visually present these differences.
2.6 Behavioral data analysis
For the behavioral results of the dual-choice oddball task, including the accuracy difference between deviant and standard stimuli (deviant - standard) and the reaction time difference between deviant and standard stimuli (deviant - standard), a one-way ANOVA was conducted to compare the three groups (HC, Real, and Sham). The significance threshold was set at p < 0.05, with FDR correction applied for multiple comparisons. Post-hoc pairwise comparisons were corrected using the Bonferroni method, with the adjusted significance threshold set at 0.05.
For the neuroimaging data of the dual-choice oddball task, multiple comparison corrections were also applied using the FDR standard. For F-tests comparing activations across the three groups, as well as post-hoc pairwise comparisons, the statistical threshold was set at pFDR < 0.05.
3 Results
3.1 Demographic and clinical characteristics
Throughout the intervention phase, two participants in the Real group and two in the Sham group did not complete the treatment. Additionally, issues with data quality necessitated further exclusions: excessive head motion during fMRI scanning resulted in the exclusion of one participant from the Real group, two from the Sham group, and two from the Healthy Control group. Consequently, the analysis was conducted with a final sample of 141 participants, distributed as follows: 47 in the Real group, 46 in the Sham group, and 48 in the HC group. Comparisons between the three groups (Real, Sham, and HC) revealed no significant differences in age, gender, handedness, or frame-wise displacement. However, the HC group had significantly longer years of education compared to the schizophrenia (SZ) groups (p < 0.001). Furthermore, comparisons between the two SZ groups (Real and Sham) indicated no significant differences in duration of illness or in Positive and Negative Syndrome Scale (PANSS) scores, including the Positive score, Negative score, General score, and the Total score. Detailed demographic and clinical information for each group can be found in Table 1.
3.2 Dual-choice oddball behavioral performance
Participants’ performance was assessed using the dual-choice oddball task, measuring both reaction times (RT) and accuracy for standard and deviant stimuli. The healthy control (HC) group had shorter RTs for both standard stimuli (462.72 ± 31.81 ms) and deviant stimuli (504.82 ± 29.65 ms), with the smallest RT difference of 42.10 ± 39.41 ms. In comparison, the schizophrenia group receiving sham rTMS treatment exhibited the longest RTs for both standard stimuli (536.48 ± 32.86 ms) and deviant stimuli (643.57 ± 34.33 ms), resulting in the largest RT difference of 107.09 ± 44.73 ms. The Real rTMS group displayed intermediate RTs for standard stimuli (468.03 ± 27.68 ms) and deviant stimuli (537.62 ± 27.84 ms), with an RT difference of 69.59 ± 38.43 ms. A one-way ANOVA revealed significant variation in RT differences across the three groups (F(2, 138) = 29.55, p < 0.0001). Post-hoc Tukey’s HSD tests further confirmed significant differences between all groups. The Sham group differed significantly from both the HC group (mean difference = 37.50 ms, p < 0.001) and the Real rTMS group (mean difference = -64.99 ms, p < 0.001). Additionally, the Real rTMS group showed a significant difference compared to the HC group (mean difference = -27.49 ms, p = 0.004). Results are shown in Table 1, Figure 2.
In terms of accuracy, the HC group performed with an average accuracy of 91.7% ± 4.6% for standard stimuli and 89.5% ± 4.4% for deviant stimuli. The Sham group displayed lower accuracy (86.0% ± 3.6% for standard and 71.4% ± 4.2% for deviant), while the Real group achieved 85.9% ± 5.2% accuracy for standard and 77.2% ± 4.6% for deviant stimuli. The accuracy difference (M - W) followed a similar pattern, with the Sham group showing the largest discrepancy (-0.146 ± 0.054), followed by the Real group (-0.087 ± 0.070) and the HC group (-0.022 ± 0.059). There was a highly significant effect of group on the accuracy difference (M - W), as indicated by ANOVA (F(2, 138) = 48.96, p < 0.0001). Post-hoc Tukey’s tests revealed significant differences between all three groups. The Sham group had a significantly larger accuracy discrepancy compared to both the HC (mean difference = -0.1247, p < 0.001) and Real groups (mean difference = 0.0599, p < 0.001). The Real group also differed significantly from the HC group (mean difference = -0.0648, p < 0.001). Results are shown in Table 1, Figure 1.
3.3 Task-based fMRI activation patterns
Figure 3 presents the results of the one-sample t-test, highlighting brain activation patterns in response to deviant stimuli compared to standard stimuli. The activation patterns were largely consistent across all groups, with key regions including the bilateral middle frontal gyrus, bilateral supplementary motor area, bilateral inferior frontal gyrus, left cingulate gyrus, right insula, and bilateral inferior parietal lobule.
Figure 4, Table 2 show the ANOVA results, revealing significant differences in brain responses among the three groups. The regions displaying these differences included the cerebellum, right insula, left thalamus, precuneus, superior temporal gyrus, and middle temporal gyrus. Post-hoc analyses, as illustrated in Figure 5, further identified specific group differences in the cerebellum, insula, and thalamus. The Sham group showed significantly reduced activity in these regions, while the Real group demonstrated a recovery in activation levels, approaching those of the healthy control group.
A different pattern emerged in the middle temporal gyrus, precuneus, and superior temporal gyrus, as shown in Figure 6. These areas typically exhibit negative activation during the task under normal conditions. In the Sham group, this negative activation was reduced. However, after real rTMS treatment, negative activation in these regions was restored and even surpassed that of the healthy controls, reaching its peak level.
3.4 Correlation analysis
A significant positive correlation was observed between increased activation in the right insula and improvements in accuracy in the Real-rTMS group (r = 0.45, p < 0.01), besides, a positive correlation was also found between the increased de-activation within the middle temporal gyrus and the improvements of reaction time in the Real-rTMS group (r = 0.37, p < 0.01, Figure 7).
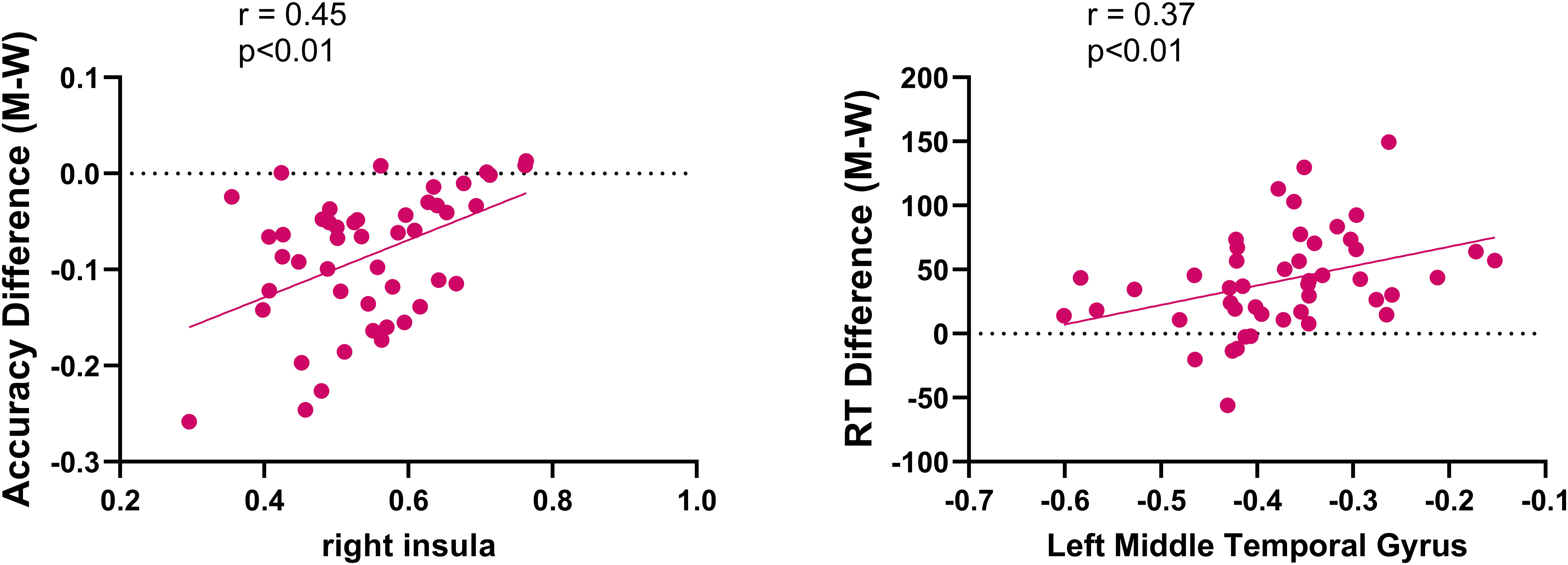
Figure 7. Correlations between fMRI activations and improvements in accuracy and reaction time in patients receiving real rTMS.
4 Discussion
The findings of this study provide compelling evidence that repetitive transcranial magnetic stimulation (rTMS) targeting the dorsolateral prefrontal cortex (DLPFC) can significantly improve inhibitory control in individuals with schizophrenia. The dual-choice oddball task revealed marked improvements in both reaction times and accuracy in the Real-rTMS group compared to the Sham group, supporting our hypothesis that rTMS can modulate inhibitory control processes. These behavioral improvements were further substantiated by functional magnetic resonance imaging (fMRI) results, which showed normalized brain activation in regions associated with cognitive control, such as the cerebellum, insula, thalamus, and prefrontal cortex. Together, these findings suggest that rTMS may act as a promising non-invasive intervention for cognitive deficits in schizophrenia, particularly for addressing inhibitory control impairments.
One of the key contributions of this study is the demonstration of rTMS’s dual mechanism of action in modulating both positive and negative brain activation patterns. The restoration of positive activation in areas such as the insula and thalamus, along with enhanced negative activation in regions like the middle temporal gyrus and precuneus, provides novel insights into how rTMS influences both excitatory and inhibitory processes in the brain. These results align with previous research suggesting that rTMS may help normalize the balance between excitatory and inhibitory neural activity (36–38). This balance is crucial for effective cognitive processing, and its restoration through rTMS could explain the observed improvements in task performance.
From a clinical perspective, the improvements in inhibitory control observed in the Real-rTMS group are particularly significant for early-stage schizophrenia. Cognitive impairments in schizophrenia, especially deficits in inhibitory control, tend to emerge early in the course of the illness and worsen over time (39, 40). Early interventions that target these impairments could have a substantial impact on long-term outcomes, helping to preserve cognitive function and improve overall prognosis (40, 41). Our findings suggest that rTMS could be a valuable tool for early cognitive interventions in schizophrenia, particularly for patients in the first episode of psychosis. Future research should explore the long-term effects of rTMS on cognitive outcomes in schizophrenia, as well as its potential to prevent the progression of cognitive decline.
The neurobiological underpinnings of these cognitive improvements provide further support for the use of rTMS in schizophrenia. The disconnection hypothesis of schizophrenia, which posits that cognitive impairments arise from disrupted connectivity between brain regions, is supported by our fMRI findings (42, 43). Specifically, the Real-rTMS group demonstrated more normalized functional connectivity patterns in regions associated with executive function and inhibitory control, suggesting that rTMS may enhance neuroplasticity and restore functional brain networks. This neuroplastic effect of rTMS could be especially beneficial in early-stage schizophrenia, where cognitive networks are more malleable and responsive to intervention (44, 45).
While this study provides important insights into the effects of rTMS on inhibitory control in schizophrenia, several limitations should be acknowledged. One of the primary limitations of this study is the lack of a comprehensive cognitive battery, such as the Brief Assessment of Cognition in Schizophrenia (BACS) or the MATRICS Consensus Cognitive Battery (MCCB) (46, 47). Without a standardized cognitive assessment, it remains unclear whether the Real and Sham groups differed in other cognitive domains beyond inhibitory control. For example, it is possible that the Sham group’s inferior performance could be due to broader cognitive impairments unrelated to the rTMS intervention. Future studies should incorporate a more comprehensive assessment of cognitive function to ensure that improvements are specific to inhibitory control rather than a general cognitive enhancement. Additionally, the short-term nature of the rTMS intervention limits the conclusions that can be drawn about the long-term efficacy of rTMS in improving cognitive function in schizophrenia. While the immediate post-treatment effects are promising, it is unclear whether these improvements are sustained over time. Longitudinal studies with follow-up assessments are necessary to determine whether rTMS provides lasting cognitive benefits and to explore the potential for maintenance or booster sessions to prolong the effects of the intervention. Another limitation relates to the sample size and demographic homogeneity of the participants. Although the sample size was sufficient for detecting significant effects, a larger and more diverse sample would allow for greater generalizability of the findings. In particular, future research should explore whether factors such as illness chronicity, medication status, and baseline cognitive function influence the effectiveness of rTMS. Stratifying participants based on these factors could provide a clearer understanding of who stands to benefit the most from rTMS and how the intervention can be tailored to individual patients.
The results of this study open several avenues for future research. First, given the neuroplastic effects of rTMS observed in this study, future investigations should explore the combination of rTMS with other therapeutic interventions that target cognitive deficits, such as cognitive remediation therapy (CRT) or pharmacological agents aimed at enhancing neuroplasticity. Combining these approaches could yield additive or synergistic effects, leading to greater improvements in cognitive function and functional outcomes. Second, this study focused on the DLPFC as the primary target for rTMS, but other brain regions involved in inhibitory control, such as the anterior cingulate cortex (ACC) or orbitofrontal cortex (OFC), may also be important targets for modulation. Future studies should explore whether stimulating these regions in combination with the DLPFC could lead to even greater improvements in cognitive function. Finally, research should also investigate the potential role of rTMS in other cognitive domains beyond inhibitory control, such as working memory, attention, and executive function. Understanding the broader cognitive effects of rTMS could help refine its use as a comprehensive cognitive intervention for schizophrenia and other neuropsychiatric conditions.
In summary, this study demonstrates that rTMS targeting the DLPFC can significantly improve inhibitory control in individuals with schizophrenia, with both behavioral and neural evidence supporting its efficacy. The normalization of brain activity in key regions associated with cognitive control suggests that rTMS may help restore functional connectivity and enhance neuroplasticity in patients with schizophrenia. While these findings are promising, further research is needed to address the limitations of the current study and to explore the long-term efficacy and broader cognitive effects of rTMS. Ultimately, this research contributes to the growing body of evidence supporting rTMS as a potential therapeutic tool for addressing the cognitive deficits that significantly impair the lives of individuals with schizophrenia.
Data availability statement
The raw data supporting the conclusions of this article will be made available by the authors, without undue reservation.
Ethics statement
The studies involving humans were approved by The First Affiliated Hospital of Xi’an Jiaotong University. The studies were conducted in accordance with the local legislation and institutional requirements. The participants provided their written informed consent to participate in this study.
Author contributions
SY: Formal analysis, Investigation, Visualization, Writing – original draft. SW: Data curation, Resources, Software, Writing – original draft. HS: Funding acquisition, Project administration, Supervision, Writing – review & editing.
Funding
The author(s) declare that no financial support was received for the research, authorship, and/or publication of this article.
Conflict of interest
The authors declare that the research was conducted in the absence of any commercial or financial relationships that could be construed as a potential conflict of interest.
Publisher’s note
All claims expressed in this article are solely those of the authors and do not necessarily represent those of their affiliated organizations, or those of the publisher, the editors and the reviewers. Any product that may be evaluated in this article, or claim that may be made by its manufacturer, is not guaranteed or endorsed by the publisher.
References
1. Kahn RS, Keefe RS. Schizophrenia is a cognitive illness: time for a change in focus. JAMA Psychiatry. (2013) 70:1107–12. doi: 10.1001/jamapsychiatry.2013.155
2. Bora E, Yücel M, Pantelis C. Cognitive impairment in schizophrenia and affective psychoses: implications for DSM-V criteria and beyond. Schizophr Bull. (2010) 36:36–42. doi: 10.1093/schbul/sbp094
3. Breuer F, Meyhöfer I, Lencer R, Sprenger A, Roesmann K, Schag K, et al. Aberrant inhibitory control as a transdiagnostic dimension of mental disorders - A meta-analysis of the antisaccade task in different psychiatric populations. Neurosci Biobehav Rev. (2024) 165:105840. doi: 10.1016/j.neubiorev.2024.105840
4. Arnatkeviciute A, Lemire M, Morrison C, Mooney M, Ryabinin P, Roslin NM, et al. Hawi Z et al: Trans-ancestry meta-analysis of genome wide association studies of inhibitory control. Mol Psychiatry. (2023) 28:4175–84. doi: 10.1038/s41380-023-02187-9
5. Constantinidis C, Luna B. Neural substrates of inhibitory control maturation in adolescence. Trends Neurosci. (2019) 42:604–16. doi: 10.1016/j.tins.2019.07.004
6. Michael GA, Dorey JM, Rey R, D'Amato T, Fabre D, Brunet S, et al. Attention in schizophrenia: Impaired inhibitory control, faulty attentional resources, or both? Psychiatry Res. (2020) 290:113164. doi: 10.1016/j.psychres.2020.113164
7. Lencer R, Yao L, Reilly JL, Keedy SK, McDowell JE, Keshavan MS, et al. Clementz BA et al: Alterations in intrinsic fronto-thalamo-parietal connectivity are associated with cognitive control deficits in psychotic disorders. Hum Brain Mapp. (2019) 40:163–74. doi: 10.1002/hbm.24362
8. Fryer SL, Roach BJ, Ford JM, Donaldson KR, Calhoun VD, Pearlson GD, et al. Should I stay or should I go? FMRI study of response inhibition in early illness schizophrenia and risk for psychosis. Schizophr Bull. (2019) 45:158–68. doi: 10.1093/schbul/sbx198
9. Fett AJ, Velthorst E, Reichenberg A, Ruggero CJ, Callahan JL, Fochtmann LJ, et al. Long-term changes in cognitive functioning in individuals with psychotic disorders: findings from the suffolk county mental health project. JAMA Psychiatry. (2020) 77:387–96. doi: 10.1001/jamapsychiatry.2019.3993
10. Goff DC. The pharmacologic treatment of schizophrenia-2021. Jama. (2021) 325:175–6. doi: 10.1001/jama.2020.19048
11. Kahn RS. On the origins of schizophrenia. Am J Psychiatry. (2020) 177:291–7. doi: 10.1176/appi.ajp.2020.20020147
12. Sabé M, Hyde J, Cramer C, Eberhard A, Crippa A, Brunoni AR, et al. Garner M et al: Transcranial Magnetic Stimulation and Transcranial Direct Current Stimulation Across Mental Disorders: A Systematic Review and Dose-Response Meta-Analysis. JAMA network Open. (2024) 7:e2412616. doi: 10.1001/jamanetworkopen.2024.12616
13. George MS. Whither TMS: A one-trick pony or the beginning of a neuroscientific revolution? Am J Psychiatry. (2019) 176:904–10. doi: 10.1176/appi.ajp.2019.19090957
14. Liu H, Wu M, Huang H, Chen X, Zeng P, Xu Y. Comparative efficacy of non-invasive brain stimulation on cognition function in patients with mild cognitive impairment: A systematic review and network meta-analysis. Ageing Res Rev. (2024) 101:102508. doi: 10.1016/j.arr.2024.102508
15. Ferrarelli F, Phillips ML. Examining and modulating neural circuits in psychiatric disorders with transcranial magnetic stimulation and electroencephalography: present practices and future developments. Am J Psychiatry. (2021) 178:400–13. doi: 10.1176/appi.ajp.2020.20071050
16. Marini M, Banaji MR, Pascual-Leone A. Studying implicit social cognition with noninvasive brain stimulation. Trends Cogn Sci. (2018) 22:1050–66. doi: 10.1016/j.tics.2018.07.014
17. Yi S, Wang Q, Wang W, Hong C, Ren Z. Efficacy of repetitive transcranial magnetic stimulation (rTMS) on negative symptoms and cognitive functioning in schizophrenia: An umbrella review of systematic reviews and meta-analyses. Psychiatry Res. (2024) 333:115728. doi: 10.1016/j.psychres.2024.115728
18. Schifani C, Hawco C, Daskalakis ZJ, Rajji TK, Mulsant BH, Tan V, et al. Repetitive transcranial magnetic stimulation (rTMS) treatment reduces variability in brain function in schizophrenia: data from a double-blind, randomized, sham-controlled trial. Schizophr Bull. (2024) 7:sbae166. doi: 10.1093/schbul/sbae166
19. Bais L, Liemburg E, Vercammen A, Bruggeman R, Knegtering H, Aleman A. Effects of low frequency rTMS treatment on brain networks for inner speech in patients with schizophrenia and auditory verbal hallucinations. Prog Neuropsychopharmacol Biol Psychiatry. (2017) 78:105–13. doi: 10.1016/j.pnpbp.2017.04.017
20. Xu M, Nikolin S, Moffa AM, Xu XM, Su Y, Li R, et al. Prolonged intermittent theta burst stimulation targeting the left prefrontal cortex and cerebellum does not affect executive functions in healthy individuals. Sci Rep. (2024) 14:11847. doi: 10.1038/s41598-024-61404-9
21. Hauer L, Sellner J, Brigo F, Trinka E, Sebastianelli L, Saltuari L, et al. Effects of repetitive transcranial magnetic stimulation over prefrontal cortex on attention in psychiatric disorders: A systematic review. J Clin Med. (2019) 8(4):416. doi: 10.3390/jcm8040416
22. Dixon BJ, Kumar J, Danielmeier C. Frontal neural metabolite changes in schizophrenia and their association with cognitive control: A systematic review. Neurosci Biobehav Rev. (2022) 132:224–47. doi: 10.1016/j.neubiorev.2021.11.010
23. Smith EH, Horga G, Yates MJ, Mikell CB, Banks GP, Pathak YJ, et al. Widespread temporal coding of cognitive control in the human prefrontal cortex. Nat Neurosci. (2019) 22:1883–91. doi: 10.1038/s41593-019-0494-0
24. Postma TS, Fitzsimmons S, Vriend C, Batelaan NM, van der Werf YD, van den Heuvel OA. TMS-induced plasticity improving cognitive control in OCD II: Task-based neural predictors of treatment response. Biol Psychiatry. (2024). doi: 10.1016/j.biopsych.2024.09.005
25. McIntyre RS, Alsuwaidan M, Baune BT, Berk M, Demyttenaere K, Goldberg JF, et al. Kennedy SH et al: Treatment-resistant depression: definition, prevalence, detection, management, and investigational interventions. World psychiatry: Off J World Psychiatr Assoc (WPA). (2023) 22:394–412. doi: 10.1002/wps.21120
26. Yuan J, Liu W, Liang Q, Cao X, Lucas MV, Yuan TF. Effect of low-frequency repetitive transcranial magnetic stimulation on impulse inhibition in abstinent patients with methamphetamine addiction: A randomized clinical trial. JAMA network Open. (2020) 3:e200910. doi: 10.1001/jamanetworkopen.2020.0910
27. Ni Z, Chen R. Transcranial magnetic stimulation to understand pathophysiology and as potential treatment for neurodegenerative diseases. Trans neurodegeneration. (2015) 4:22. doi: 10.1186/s40035-015-0045-x
28. Panagiotaropoulou G, Thrapsanioti E, Pappa E, Grigoras C, Mylonas D, Karavasilis E, et al. Hypo-activity of the dorsolateral prefrontal cortex relates to increased reaction time variability in patients with schizophrenia. NeuroImage Clin. (2019) 23:101853. doi: 10.1016/j.nicl.2019.101853
29. Guillaume S, Gay A, Jaussent I, Sigaud T, Billard S, Attal J, et al. Massoubre C et al: Improving decision-making and cognitive impulse control in bulimia nervosa by rTMS: An ancillary randomized controlled study. Int J eating Disord. (2018) 51:1103–6. doi: 10.1002/eat.22942
30. Diana M, Raij T, Melis M, Nummenmaa A, Leggio L, Bonci A. Rehabilitating the addicted brain with transcranial magnetic stimulation. Nat Rev Neurosci. (2017) 18:685–93. doi: 10.1038/nrn.2017.113
31. Di S, Ma C, Wu X, Lei L. Gender differences in behavioral inhibitory control under evoked acute stress: An event-related potential study. Front Psychol. (2023) 14:1107935. doi: 10.3389/fpsyg.2023.1107935
32. Tandon R, Gaebel W, Barch DM, Bustillo J, Gur RE, Heckers S, et al. Tsuang M et al: Definition and description of schizophrenia in the DSM-5. Schizophr Res. (2013) 150:3–10. doi: 10.1016/j.schres.2013.05.028
33. Leucht S, Samara M, Heres S, Davis JM. Dose equivalents for antipsychotic drugs: the DDD method. Schizophr Bull. (2016) 42 Suppl 1:S90–94. doi: 10.1093/schbul/sbv167
34. Hays JR, Reas DL, Shaw JB. Concurrent validity of the Wechsler abbreviated scale of intelligence and the Kaufman brief intelligence test among psychiatric inpatients. psychol Rep. (2002) 90:355–9. doi: 10.2466/pr0.2002.90.2.355
35. Fox MD, Buckner RL, White MP, Greicius MD, Pascual-Leone A. Efficacy of transcranial magnetic stimulation targets for depression is related to intrinsic functional connectivity with the subgenual cingulate. Biol Psychiatry. (2012) 72:595–603. doi: 10.1016/j.biopsych.2012.04.028
36. Hao W, Dai X, Wei M, Li S, Peng M, Xue Q, et al. Efficacy of transcranial photobiomodulation in the treatment for major depressive disorder: A TMS-EEG and pilot study. Photodermatology photoimmunology photomedicine. (2024) 40:e12957. doi: 10.1111/phpp.12957
37. Cocchi L, Zalesky A. Personalized transcranial magnetic stimulation in psychiatry. Biol Psychiatry Cogn Neurosci Neuroimaging. (2018) 3:731–41. doi: 10.1016/j.bpsc.2018.01.008
38. Avissar M, Powell F, Ilieva I, Respino M, Gunning FM, Liston C, et al. Functional connectivity of the left DLPFC to striatum predicts treatment response of depression to TMS. Brain stimulation. (2017) 10:919–25. doi: 10.1016/j.brs.2017.07.002
39. McCutcheon RA, Keefe RSE, McGuire PK. Cognitive impairment in schizophrenia: aetiology, pathophysiology, and treatment. Mol Psychiatry. (2023) 28:1902–18. doi: 10.1038/s41380-023-01949-9
40. Javitt DC. Cognitive impairment associated with schizophrenia: from pathophysiology to treatment. Annu Rev Pharmacol Toxicol. (2023) 63:119–41. doi: 10.1146/annurev-pharmtox-051921-093250
41. Keshavan MS, Eack SM. Cognitive enhancement interventions are effective for schizophrenia: why not provide them early? World psychiatry: Off J World Psychiatr Assoc (WPA). (2023) 22:326–7. doi: 10.1002/wps.21091
42. Sapienza J, Pacchioni F, Spangaro M, Bosia M. Dysconnection in schizophrenia: Filling the dots from old to new evidence. Clin neurophysiology: Off J Int Fed Clin Neurophysiol. (2024) 162:226–8. doi: 10.1016/j.clinph.2024.03.013
43. Park S, Thakkar KN. Splitting of the mind" revisited: recent neuroimaging evidence for functional dysconnection in schizophrenia and its relation to symptoms. Am J Psychiatry. (2010) 167:366–8. doi: 10.1176/appi.ajp.2010.10010089
44. Mehta UM, Ithal D, Roy N, Shekhar S, Govindaraj R, Ramachandraiah CT, et al. Venkatasubramanian G et al: Posterior Cerebellar Resting-State Functional Hypoconnectivity: A Neural Marker of Schizophrenia Across Different Stages of Treatment Response. Biol Psychiatry. (2024) 96:365–75. doi: 10.1016/j.biopsych.2024.01.027
45. Wannan CMJ, Cropley VL, Chakravarty MM, Bousman C, Ganella EP, Bruggemann JM, et al. McGorry P et al: Evidence for Network-Based Cortical Thickness Reductions in Schizophrenia. Am J Psychiatry. (2019) 176:552–63. doi: 10.1176/appi.ajp.2019.18040380
46. Li Y, Ang MS, Yee JY, See YM, Lee J. Predictors of functioning in treatment-resistant schizophrenia: the role of negative symptoms and neurocognition. Front Psychiatry. (2024) 15:1444843. doi: 10.3389/fpsyt.2024.1444843
Keywords: rTMS, schizophrenia, inhibitory control, cognitive deficits, DLPFC, fMRI, brain activation
Citation: Yu S, Wang S and Sun H (2024) Effects of repetitive transcranial magnetic stimulation on inhibitory control in first-episode schizophrenia: behavioral and neural mechanisms. Front. Psychiatry 15:1496562. doi: 10.3389/fpsyt.2024.1496562
Received: 14 September 2024; Accepted: 22 October 2024;
Published: 04 November 2024.
Edited by:
Andrea Galbiati, Vita-Salute San Raffaele University, ItalyReviewed by:
Yuchen Liu, Fudan University, ChinaWanJun Hu, Lanzhou University Second Hospital, China
Jacopo Sapienza, San Raffaele Scientific Institute (IRCCS), Italy
Copyright © 2024 Yu, Wang and Sun. This is an open-access article distributed under the terms of the Creative Commons Attribution License (CC BY). The use, distribution or reproduction in other forums is permitted, provided the original author(s) and the copyright owner(s) are credited and that the original publication in this journal is cited, in accordance with accepted academic practice. No use, distribution or reproduction is permitted which does not comply with these terms.
*Correspondence: Sihang Yu, c2loYW5nLnl1MDdAb3V0bG9vay5jb20=