- 1Institute of Translational Biomedicine, St. Petersburg State University, St. Petersburg, Russia
- 2Institute of Experimental Medicine, Saint Petersburg, Russia
- 3National Research University Higher School of Economics, Moscow, Russia
- 4Department of Neurobiology, Sirius University of Science and Technology, Sirius, Russia
- 5St. Petersburg University Hospital, St. Petersburg State University, St. Petersburg, Russia
- 6Department of Biosciences and Bioinformatics, School of Science, Xi’an Jiaotong-Liverpool University, Suzhou, China
- 7Suzhou Municipal Key Laboratory of Neurobiology and Cell Signaling, School of Science, Xi’an Jiaotong-Liverpool University, Suzhou, China
- 8Max Delbrück Center for Molecular Medicine in the Helmholtz Association (MDC), Berlin, Germany
Introduction: Aggression and self-harm disproportionately occur in youths preoccupied with social status tracking. These pathological conditions are linked to a serotonin (5-HT) deficit in the brain. Ablation of 5-HT biosynthesis by tryptophan hydroxylase 2 knockout (TPH2-KO) increases aggression in rodents. Remarkably, deletion of the trace amine-associated receptor 1 (TAAR1) results in the same consequences. Unlike the nuanced dynamics of social status cues in young people, the social ranks of rats mainly advance when they dominate larger opponents in combat.
Methods: This study explored whether the potent TAAR1 agonist RO5263397 reduces aggression caused by 5-HT depletion, and whether social rank advancement motivates this aggression. The resident-intruder paradigm was applied with larger and smaller intruders to evaluate whether social rank advancement motivates aggressive behaviors in TPH2-KO rats.
Results: When a smaller intruder was introduced, 5-HT-deficient rats did not differ from wild type littermates. However, when the intruders were larger, the mutants extended their aggressive efforts, refusing to submit. Importantly, RO5263397 selectively abolished this abnormal form of aggression in TPH2-KO rats.
Discussion: Results supported social rank advancement as the main incentive. These data also suggest that TAAR1 is a promising target for the development of new treatments for aggression; independent data also support this conclusion.
1 Introduction
Aggression and self-harm co-occur in many psychiatric patients with bipolar disorder, depression and other mental illnesses. Selective serotonin reuptake inhibitors (SSRIs) are commonly prescribed in these pathological conditions (1, 2). However, some studies suggest that SSRIs can trigger suicidality and uncharacteristic acts of violence (3, 4). Although fraught with controversy, substantial evidence suggests that aggression and self-harm are rare side effects of SSRIs, especially evident in youths (5). Leaflets for SSRIs warn of treatment-emergent suicidality in adolescents and young adults. Violent crime and self-harm are particularly common in people aged < 25 years (6, 7). This is no coincidence (8), since this age group is more sensitive to social status cues (9). Strong evidence suggests that both aggression and self-harm are tightly linked with social status tracking (10–12).
The SSRI class acts by increasing the synaptic level of serotonin (5-HT) (13), which affects many aspects of animal social hierarchies (14, 15) and the stress response (16). The synthesis of 5-HT from the essential amino acid tryptophan is mediated by two spatially segregated isoforms of the enzyme tryptophan hydroxylase (TPH); one is peripheral and the other is central (17). The central TPH2 isoform is predominantly expressed in neurons originating in the brainstem, with projections to the frontal cortex, amygdala, and hippocampus. In rodents, genetic deletion of TPH2 (TPH2-KO) causes increased aggression, social deficits, and altered anxiety-like behaviors (18–23). Both humans and non-human primates exhibit links between 5-HT, aggression, and self-harm (9, 24–26). This corroborates prior indications that the role of 5-HT in violence is conserved across species.
The trace amine-associated receptor 1 (TAAR1) is the most studied member of the TAAR family. It participates in reward and limbic networks and is expressed in several brain regions (78), including the medial prefrontal cortex (mPFC). Serotonergic signaling in this area is known to critically influence the top-down regulation of aggression (27). Initially, TAAR1-KO rodents were proposed as models of schizophrenia (28), showing not only enhanced responses to amphetamine and deficits in sensorimotor gating but also altered sleep-wake cycles and increased impulsivity (29, 30). The TAAR1 agonists RO6889450 (Ralmitaront) and SEP-363856 (Ulotaront) are now under active clinical development for schizophrenia treatment (31). Nevertheless, preclinical studies suggest that TAAR1 could mediate multiple neuropsychiatric effects, exerting antipsychotic, antidepressant, procognitive, anti-obsessive, anti-addictive, and sleep-modulating actions (32, 33).
Importantly, it was previously demonstrated that TAAR1-KO promotes aggression, increases 5-HT in the prefrontal cortex, affects sexual motivation, and reduces grooming in mice (34). These results are particularly interesting when compared to the TPH2-KO phenotype. The present study thus aimed to test whether TAAR1 activation reduces the aggression caused by 5-HT depletion, and whether this aggression is linked to social hierarchies as it is in young people (11, 12). Given the centrality of rat aggression in this study, an explanation of rat social aggression is due. Male rats form social dominance hierarchies on the basis of age and body weight (35). When rats fight, the aggression typically stops when one animal signals social defeat through submissive posture and the cessation of retaliatory efforts. The animals usually stop attacking when their opponents submit in this way and most confrontations are brief (<60 sec is common). It is thus abnormal for a much smaller rat to persevere, or for a much larger rat to continue attacking after winning.
In this study, the resident-intruder paradigm was applied to represent for residents (i.e., TPH2-KO or WT littermates) opportunities to advance in social rank (i.e., bigger intruders) and lack thereof (i.e., smaller intruders). Results suggest that the highly aggressive behavior of TPH2-KO rats is motivated by an exaggerated drive to advance in the social dominance hierarchy. Most importantly, the TAAR1-specific agonist RO5263397 abolishes this type of aggression, and independent data now replicate this under different conditions (36).
2 Materials and methods
2.1 Ethics statement
All animal studies were carried out in accordance with the guidelines of the Ministry of Health of the Russian Federation, the Federation of European Laboratory Animal Science Associations (FELASA), and the Russian Laboratory Animal Science Association (RusLASA) for the welfare of laboratory animals. All experiments were approved by the Saint Petersburg State University Ethical Committee for Animal Research (approval 131-03-1 of 13.03.2022).
2.2 Subjects
Middle-aged adult wild-type (WT) and TPH2-deficient (TPH2-KO, (79) male rats on Dark Agouti background (35 weeks old, n = 8 per genotype, mean weight WT = 312 g [SD = 22], TPH2-KO = 317 g [SD = 17]) were derived by crossing animals heterozygous for the TPH2 mutation and genotyped as previously described (37). Animals were maintained under standard lab conditions (room temperature and humidity 21 ± 5°C and 40–70%, respectively) with food and water ad libitum, under artificial light-dark cycle (lights on 10:00, lights off 22:00). All experiments were conducted during the light phase from 15:00 to 22:00 at an illuminance of 265 lux throughout. The rats were acclimated to the experimental room for at least 1 h prior to behavioral testing. A scheme of the experimental design is shown in Figure 1. All rats were genotyped before and after the experiments.
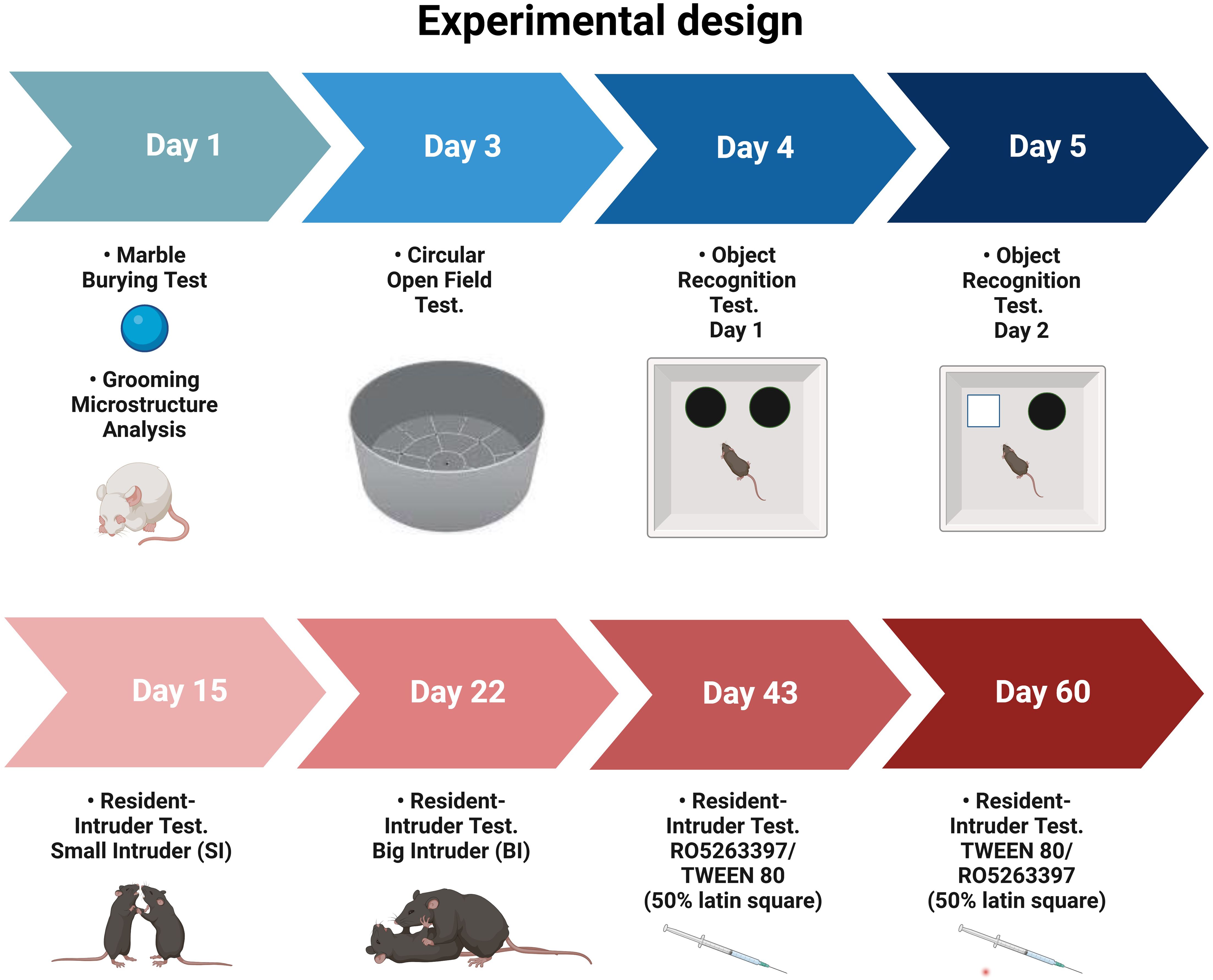
Figure 1. Experimental design of comparative analysis between WT and TPH2-KO rats (n = 8 per group).
2.3 Drugs
A TAAR1 agonist (S)-4-(3-Fluoro-2-methylphenyl)-4,5-dihydrooxazol-2-amine (RO5263397; CAS#: 1357266-05-7), synthesized at F. Hoffmann-La Roche Ltd (Basel, Switzerland), was chosen for its high intrinsic efficacy (76 ± 13%) and specificity at the rat TAAR1 receptor, as evidenced by the lack of effects in TAAR1 knockout animals (30, 38). Its half-life (T1/2) of 2.6 h (39) was also conducive to experimental purposes. Fresh solutions of 5 mg/ml RO5263397 in 10% buffered Tween 80 were prepared on the same day of every experiment. Intraperitoneal injections were administered at doses of 1 ml/kg body weight (i.e., 5 mg/kg) 10 min before the start of the resident-intruder interaction. The dose test sequences were based on a within-subjects Latin Square design (i.e., each animal was tested with both an active dose and vehicle) with at least 2 weeks between the tests, to ensure washout and stress recovery.
2.4 Behavioral assays
The Open Field Test (OFT) was used to assess the locomotor and exploratory activity of rats. The circular arena was made of gray plastic with a diameter of 67 cm, and included 13 uniform holes of 1 cm diameter in the floor. The test subject was placed in the center of the arena, after which its natural exploratory behavior was recorded for 10 min using Noldus EthoVision XT Version 11.5 (Noldus IT, Wageningen, Netherlands) followed by manual scoring by highly experienced experimenters blinded to genotype. The endpoints recorded were: locomotor activity (distance traveled, cm), freezing (sec), sniffing (sec), grooming (sec), number of rearings (n), and hole exploration (sec). To eliminate the confounding effects of variable olfactory cues, the apparatus was cleaned with a 3% hydrogen peroxide solution before each trial for all tests herein.
The OFT also served as the adaptation session (Day 0) for a subsequent Object Recognition Test (ORT) conducted in the same arena over the next two days (40). On Day 1, single animals were released into the testing arena facing two identical objects (black cylinders). To quantify recognition memory, the next sessions on Day 2 only differed in that the familiar object on the left was substituted with a novel one (a white pyramid of approximately equal mass). Data were recorded similar to the OFT analyses, scoring the duration of exploration of right and left objects (s), grooming (s), movement (s), and rest/inactivity (s). Only active exploration (i.e., sniffing or rearing on an object) was counted, and rest/inactivity near objects was not included in exploration time.
The Marble Burying Test (MBT) evaluates compulsive-like behavior in rodents (41). Twenty marbles were placed on the surface of clean bedding (~5 cm layer, 400 ± 15 g per cage) in polycarbonate rat cages of standard dimensions, and the number of marbles buried (n) during a 30-min session was counted. Due to the bigger size of rats (than mice for which the test was initially designed), only fully buried marbles not visible from the top were counted.
The Grooming Test (GT) was applied to quantify both basic self-grooming metrics in addition to grooming microstructure (42, 43). Briefly, rats were individually placed in a transparent glass cylindrical jar (20 cm in diameter, 45 cm in height) and their grooming was recorded for 10 min using Apple iPhone 12 video camera (Apple Inc., Cupertino, USA). Recorded grooming behavior was then manually scored to ascertain: total grooming bouts (n), rostral grooming bouts (n), caudal grooming bouts (n), as well as grooming bouts (n) specific to the paw, face, head, body and tail. To further analyze grooming microstructure, the number of grooming transitions (n) between different body parts (e.g., nose to head, head to tail) were also noted here. Any transition between grooming stages that violated normal cephalo-caudal progression (paws > face/nose > head > body > tail/anogenital) was considered incorrect (this included skipping body parts), allowing the calculation of the percent correct transitions (i.e., [correct/total]*100%). The number of transitions within each cephalo-caudal progression was noted and ethograms were generated to represent microstructural sequences as done previously (44).
An adapted and extended resident-intruder paradigm (34, 45) was used here to evaluate territorial aggression in the context of differential dominance. Female rats require more elaborate protocols and smaller intruders to avoid confounds and floor effects in this assay (46); the study thus used male rats for the sake of simplicity. Isolated TPH2-KO and WT male rats were observed for 10 min with a socialized (i.e., group-housed) intruder. Male Wistar rats (white coat) were used as intruders to ensure reliable distinction from residents (dark coat). Bedding material of the home cage (590х380х200 mm) was not changed for 1 week before the experiment to ensure an undisturbed sense of territory. Different intruders were used after every 3-4 confrontations. The videos were manually scored to ascertain: seconds spent in social interaction (anogenital sniffing, tail sniffing, body sniffing, nose sniffing), non-social exploration (rearing, wall stand, burying, sniffing), and aggression parameters (attack latency, lateral threat, upright posture, clinch attack, keep down, chase), as well as locomotor endpoints such as movement (sec), and rest/inactivity (sec). Mounting behavior was evaluated by recording the number of mountings (n) and intromission time (sec).
The experiment consisted of two phases. First, the socialized 50% smaller weight intruder (SI, Wistars, WT male, 8 weeks) was placed in front of the TPH2-KO or WT male resident to assess aggression. Following 1-week habituation to fresh bedding, the experiment was repeated with socialized 30% bigger weight intruders (BI, Wistars, WT male, 15 weeks). Three weeks later, the experiment was repeated once more with residents under a RO5263397 pretreatment and intruders from the BI group (full datasets and video samples are presented in Supplementary Materials). Aggression towards smaller intruders who give postural signals of social defeat is redundant because they do not pose a threat to the larger resident’s territory or social dominance status. Conversely, aggression towards larger intruders signifies an attempt to advance one’s social dominance status. Rats generally form social hierarchies on the basis of age and body mass (35). This study varied the age and body mass of intruders, effectively modelling differential social ranks (see Supplementary Material for individual weights of all resident-intruder pairs).
2.5 Statistical analyses and visualization
Analyses and visualization were performed with GraphPadPrism 8.0 (GraphPad Software, USA). Data are expressed as the mean ± standard error of the mean (SEM) or the median and interquartile range. The non-parametric Mann–Whitney U test (α = 0.05; two-tailed) was used to compare the WT and TPH2-KO groups for baseline behavioral assays. Results from the extended resident-intruder paradigm were analyzed by two-way repeated measures ANOVA (Factors: FGenotype is WT vs. TPH2-KO; FIntruder is bigger vs. smaller intruders; FDrug is RO5263397 vs. vehicle [i.e., TWEEN80]) followed by Dunnett’s post-hoc test (α = 0.05; two-tailed). Colored visualization of statistical differences: WT vs. WT = * (blue), KO vs. KO = * (orange), WT vs. KO = * (violet). Exhaustive statistical reports are available in Supplementary Materials Table 2. Additional visualizations were conducted with the R radar-plot package, and a licensed account on BioRender.com.
3 Results
3.1 TPH2-KO alters exploratory behavior and laterality
Before the resident-intruder test, exploratory, anxiety-like and compulsive-like behaviors were evaluated in TPH2-KO rats (Figure 2). In the OFT, mutant rats showed decreased exploration of holes (U = 11, n = 6-8 per group, p = 0.0260) but otherwise unaltered anxiety-related behaviors (freezing, grooming, sniffing) or locomotor endpoints (see Figures 2A, B and Supplementary Materials for details). In the object recognition test (ORT, Figures 2C, D, TPH2-KO rats showed a marked preference for grooming over exploring the two identical objects (U = 1, n = 6-8 per group, p = 0.0006), and mostly avoided the rightward object compared to WT (U = 4, n = 6-8 per group, p = 0.0017) (Figure 2C). On Day 2, the TPH2-KO rats explored the new object significantly more than WT (U = 7, n = 6-8 per group, p = 0.0293). The avoidance of the rightward object persisted on Day 2, unlike WT counterparts (U = 4.5, n = 6-8 per group, p = 0.0093) which showed no such laterality on either day. The mutants also reared less on the identical objects in the ORT (U = 2.5, n = 6-8 per group, p = 0.0008) (Figure 2E). In the marble burying test, TPH2-KO rats exhibited increased compulsive-like tendencies (U = 7, n = 6-8 per group, p = 0.0065) (Figure 2F), which corroborates prior results in mice (47, 48).
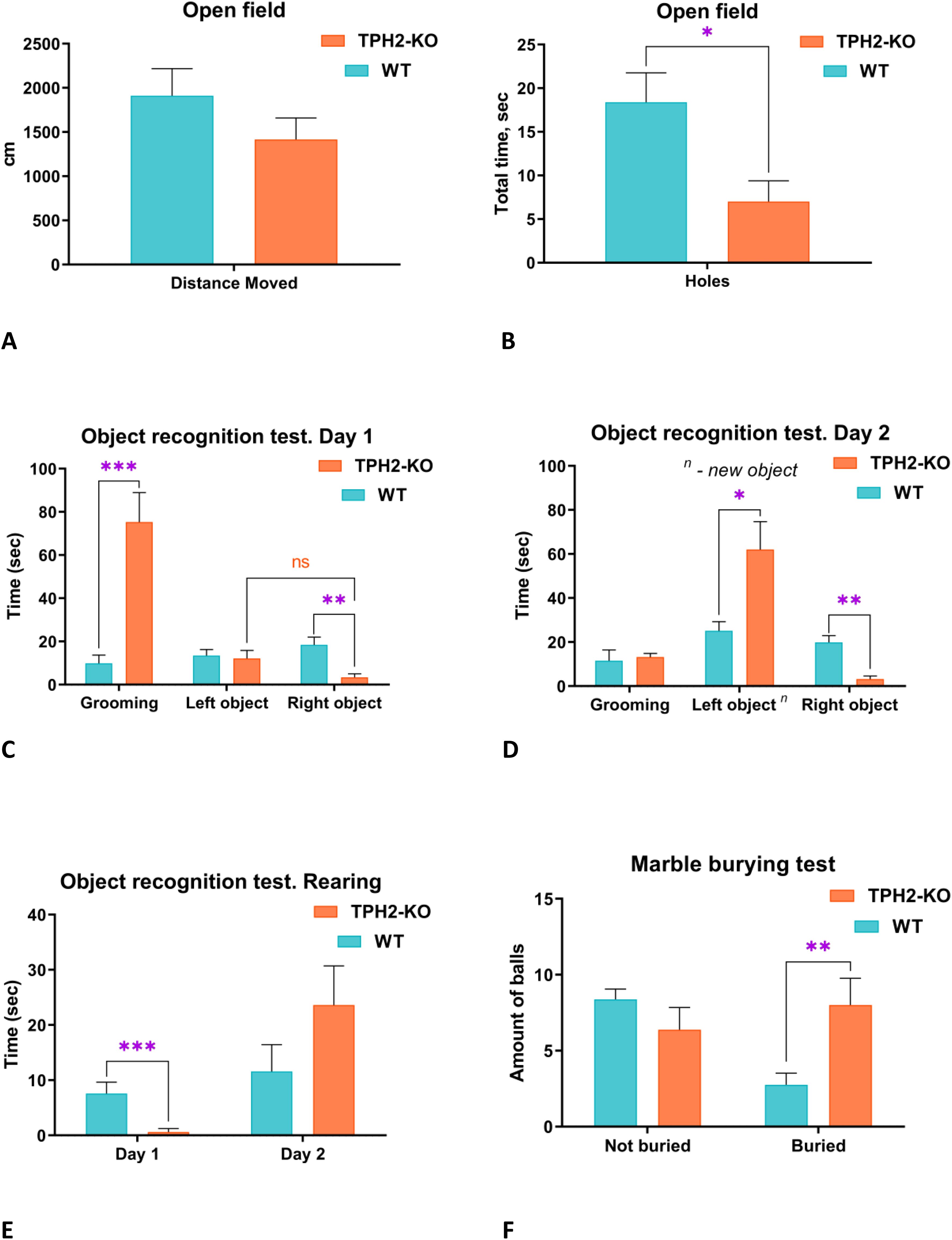
Figure 2. Evaluation of baseline behavioral phenotype of TPH2-KO rats. (A, B) TPH2-KO rats showed decreased hole exploration in circular open-field test (p = 0.0260). Other parameters do not reach significant differences. (C) In the novel object recognition test (ORT) TPH2-KO rats showed significant changes compared to WT. In front of similar objects (Day 1), TPH2-KO rats demonstrated significantly increased grooming (p = 0.0006) and decreased exploration of the right object (p = 0.0017). (D) Day 2 of ORT revealed increased learning of the new object (p = 0.0293), but decreased time with right one in TPH2 compared to WT rats (p = 0.0093). (E) TPH2-KO rats spent less time in rearing exploration in comparison to WT (p = 0.0008). (F) The marble burying test revealed a slightly obsessive-compulsive behavioral phenotype in the TPH2-KO group represented in a buried endpoint (p = 0.0065). Data are presented as mean ± SEM (n = 8). *p<0.05, **p<0.01, ***p<0.001 vs. control, Mann-Whitney U-test. WT, wild type rats.
The study included an in-depth analysis of grooming microstructure, since DA signaling in the basal ganglia drives sequentially patterned behaviors such as grooming (49–52). Disrupted 5-HT synthesis, as observed in TPH2 knockout mice, also seem to be linked to altered grooming habits (44). Given the importance of DA and 5-HT in psychiatry, grooming metrics are often used as indicators of underlying psychiatric-like conditions (44). Figures 3A, B summarizes grooming data in TPH2-KO and WT groups, showing subtle alterations in the cephalo-caudal progression (paws > face > head > body > genitals/tail) and slightly reduced percent of correct grooming transitions (U = 45.5, n = 6-8 per group, p = 0.048) in the TPH2-KO group (Figure 3B; Table 1).
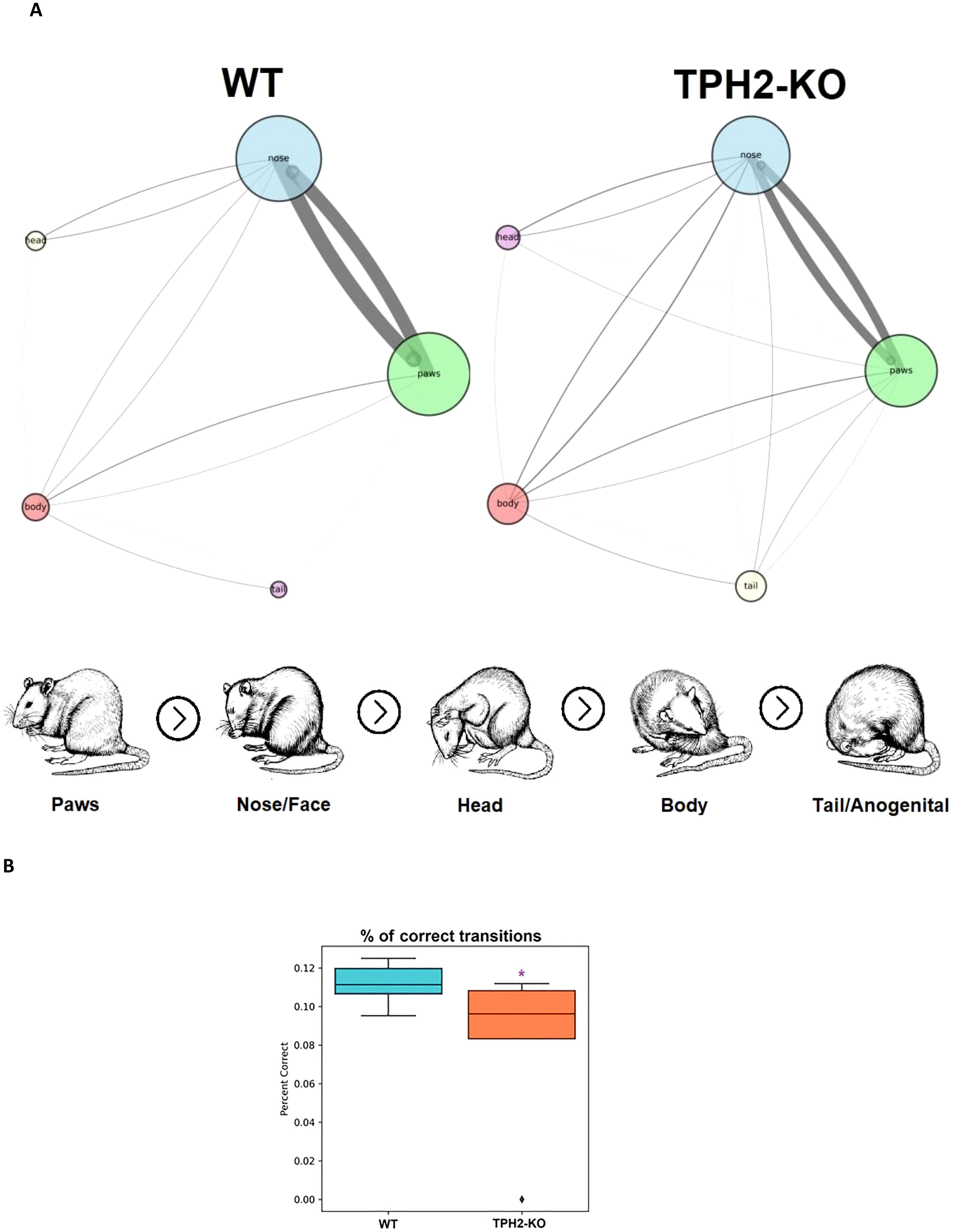
Figure 3. TPH2-KO rats have slightly decreased percent of correct transitions in grooming microstructure. (A) Comparative self-grooming microstructure analyses of TPH2 knockout (KO) rats vs. wild type (WT) control group in the grooming test (n = 8 per group). The diameter of circles and line thickness reflect mean frequency of grooming bouts or transitions, respectively. All grooming bouts, but only ‘correct’ grooming transitions adhering to the cephalo-caudal progression (paws > face > head > body > tail/genitals) were statistically assessed. (B) TPH2-KO rat had decreased percent of correct grooming transitions (p = 0.048) supporting its higher sensitivity to changes in grooming behavior. *p < 0.05 vs. control, Mann–Whitney U-test. WT, wild type control rat.
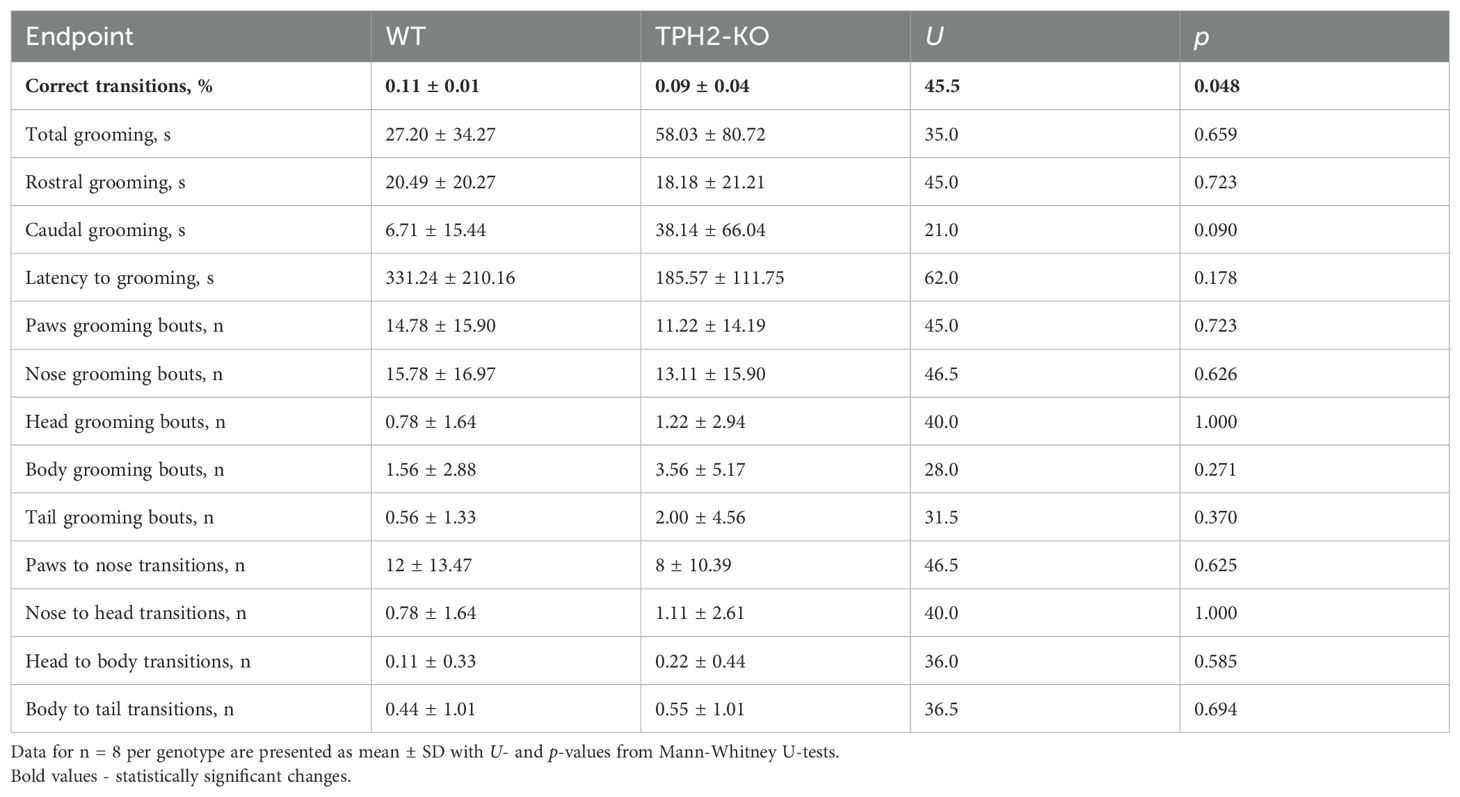
Table 1. Summary of statistical results of TPH2 knockout (TPH2-KO)-induced behavioral changes in mouse grooming test and grooming microstructure analysis.
3.2 TPH2-KO rats pursue dominance in the resident-intruder test
To determine whether TPH2-KO aggression is motivated by the pursuit of dominance, an extended resident-intruder test was applied with two types of intruder groups (Figure 4A), operationalizing relative dominance with weight classes. Aggression towards smaller intruders did not significantly vary by genotype (Figure 4B). These conflicts were brief, as the smaller intruders were quick to assume a submissive pose, and the TPH2-KO rats typically refrained from any further aggression. With larger intruders, aggressive activity was markedly prolonged in the TPH2–KO group compared to WT (FGenotype(1, 7) = 25; FIntruder(1, 7) = 25, p = 0.0002), with reduced attack latency (FGenotype(1, 7) = 33; FIntruder(1, 7) = 2, p < 0.0001), increased ‘move-towards’ activity (FGenotype(1, 7) = 3; FIntruder(1, 7) = 3, p = 0.0216), and non-social exploration (FGenotype(1, 7) = 11; FIntruder(1, 7) = 2, p = 0.0031) (Figures 4C–F). Despite the bigger size of intruders, TPH2-KO rats exhibited more lateral threats (FGenotype(1, 7) = 31; FIntruder(1, 7) = 31, p = 00.0023) and clinch attacks (FGenotype(1, 7) = 19; FIntruder(1, 7) = 4, p = 0.0355). See Supplementary Figure S2A in Supplementary Materials for plots of these results.
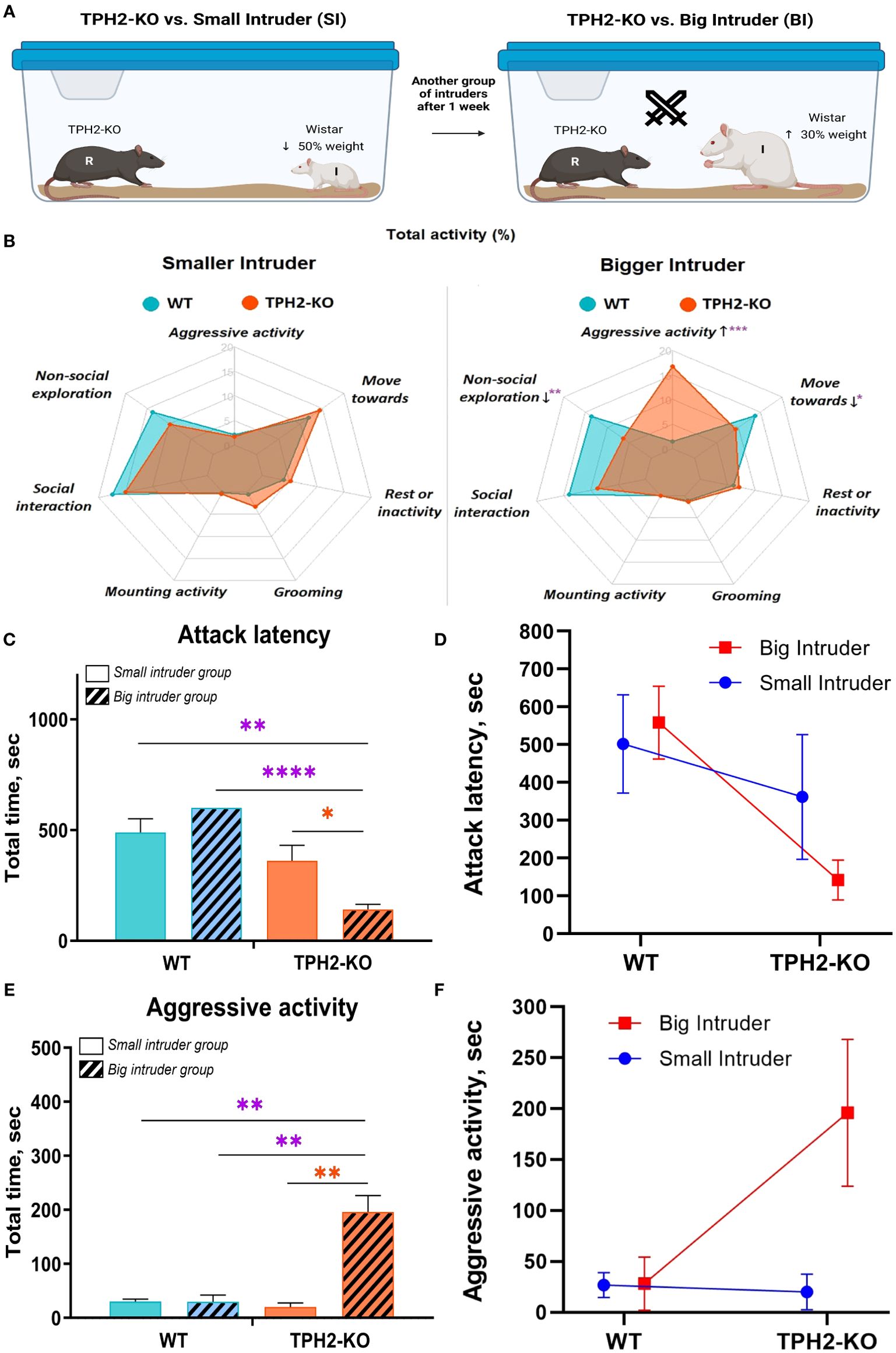
Figure 4. TPH2-KO rats cannot accept social defeat in the resident-intruder test. (A) Represents scheme of resident-intruder test with different types of intruders. TPH2-KO and WT rats opposed in front of SI and BI groups. (B) Radar-plot with total activity demonstrates a significant difference in TPH2-KO rats’ behavior versus the BI group. Minimal alterations were revealed in the SI group experiment. TPH2 gene knockout causes significantly increased aggressive behavior (p = 0.0002) in front of the larger opponent, decreased non-social exploration (p = 0.0031), and locomotor activity (p = 0.0216). (C–F) In the BI experiment, TPH2-KO vs. WT also demonstrated significantly decreased attack latency (p < 0.0001) and increased aggressive activity (p = 0.0029). Data are presented as mean ± SEM (n=8). *p<0.05, **p<0.01, ***p<0.01, ****p ≤ 0.0001 vs. control; Two-way repeated measures ANOVA (Factor 1 – genotype: WT/TPH2-KO; Factor 2 – intruder type: big/small) followed by Dunnett’s post-hoc test. WT, wild type control rats. .
3.3 TAAR1 partial agonist RO5263397 decreases social aggression in TPH2-KO rats
Next, the TAAR1 agonist RO5263397 was administered to test whether TAAR1 activity attenuates the aggression revealed in the prior experiment (Figure 5A). Ten minutes after intraperitoneal injection, the TAAR1 agonist fully rescued the behavior of TPH2-KO rats (Figure 5B). Vehicle (10% buffered Tween 80) treated mutants showed decreased attack latency compared to those treated with RO5263397 [FGenotype(1, 7) = 34; FDrug (1, 7) = 29, p = 0.0005], which became indistinguishable from WT. Aggressive activity also decreased markedly [FGenotype(1, 7) = 26; FDrug (1, 7) = 11, p = 0.0087], with a slight increase in mounting activity [FGenotype(1, 7) = 9; FDrug (1, 7) = 2, p = 0.0338] (Figures 5B–F).
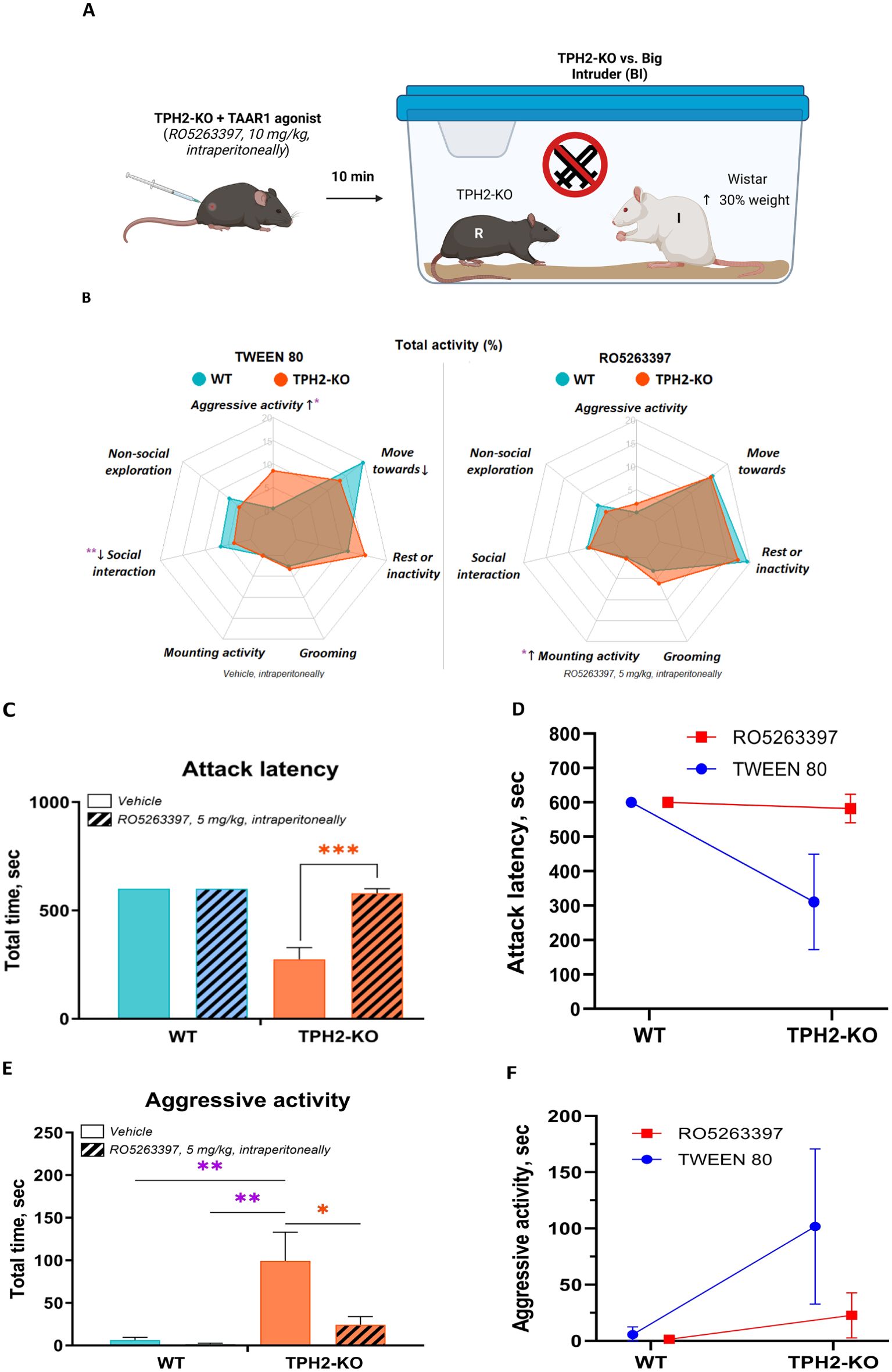
Figure 5. TAAR1 agonist decreases social aggression in TPH2 knockout rats. (A) RO5263397 (5 mg/kg) or vehicle were administered intraperitoneally 10 min before the start of the resident intruder interaction with TPH2-KO and WT rats opposed in front of the BI group. (B) RO5263397 normalizes the behavioral profile in the resident-intruder test, by decreasing aggressive activity (p = 0.0087) and slightly increasing mounting activity (p = 0.0338). (C-F) In the experiment with TPH2-KO vs. WT RO5263397 normalize alterations in attack latency and aggressive activity endpoints. Data are presented as mean ± SEM (n = 8). *p<0.05, **p<0.01, ***p<0.01 vs. control; Two-way repeated measures ANOVA (Factor 1 – genotype: WT/TPH2-KO; Factor 2 - drug effect: RO5263397/TWEEN80) followed by Dunnett’s post-hoc test. WT, wild type control rats.
4 Discussion
The present study corroborates in rats that 5-HT depletion alters exploratory behaviors and increases aggression (21–23). The resident-intruder test results refine the current understanding of 5-HT’s involvement in rodent aggression by explicitly linking it to the drive to advance in social dominance hierarchies. Indeed, when confronted with a smaller intruder, the 5-HT-deficient rats behave as WT littermates do, whereas when the intruder is larger, the mutant rats prolong their aggressive efforts, refusing to submit. Since rats form social hierarchies based on body weight (35), dominating smaller rats does not advance social rank, but dominating larger ones does. Thus, these results show that 5-HT depletion selectively increases aggression to capitalize on opportunities to advance the rat’s social status. Most importantly, the activation of TAAR1 abolishes this abnormal aggression in mutant rats, a finding of both clinical and social significance (3). The other behavioral assays recapitulated features of compulsive behavior previously established TPH2-KO mice (47), confirming the conservation of mechanisms.
The effect of 5-HT depletion on aggression is well-replicated in TPH2-KO mice (19, 20), and the results herein corroborate this phenotype in rats, indicating that it is conserved across species. These statements pertain entirely to males, as female rats tend not to challenge conspecifics of equal or larger size (46). The 5-HT depletion idea also applies to primates, as reduced 5-HT and its metabolites are evident in highly aggressive nonhuman primates (25, 53), and lower 5-HT signaling is correlated with aggressive tendencies in humans (26). Indeed, central levels of 5-HT and its main metabolite are lower in individuals diagnosed with antisocial personality disorder (25), a diagnosis strongly associated with aggression (54). Brain samples from suicide victims also show increased expression of dorsal raphe 5-HT1A receptors, implying reduced serotonergic signaling given its inhibitory autoreceptor function (24). Taken together, these findings imply that 5-HT depletion can trigger aggression both inwardly (i.e., self-harm or suicide), and outwardly (i.e., emotional abuse or physical attacks); this aligns well with experimental results on 5-HT depletion in humans (55).
The SSRIs are efficacious in many conditions other than depression (56). Given their mechanism of action, this transdiagnostic efficacy implies that many patients experience their symptoms in part due to 5-HT depletion. For instance, the undue feelings of guilt and shame that are common in depression can be induced by 5-HT depletion in humans (55). Genetic predispositions to such disorders also implicate the serotonergic system. The depression-related SNP (R439H) in the human Tph2 gene greatly diminishes 5-HT production, and chronic SSRI treatment exacerbates this depletion in humanized mouse carriers of R439H (13). This effect may not be unique, as other mutations may perturb the same functions, and thus lead to the same depletion under chronic SSRI use (16, 57, 58). It remains unknown whether this paradoxical effect contributes to SSRI-related violence. However, as mentioned in the introduction, human studies have established that perceptions of one’s social rank motivate violence in young people. The present study concords with this, suggesting that the aggression of the TPH2-KO rat is motivated by a drive to advance in social rank.
The most important finding here is that this abnormal form of aggression is abolished by TAAR1 activation. This is particularly promising because TAAR1 is known to support cognitive control (59–61). Deficits in cognitive control are not only associated with aggression and self-harm, they are also transdiagnostic (62), which suggests the future potential of TAAR1 agonists as common adjuncts in psychiatry. A burgeoning literature also establishes the biological plausibility of TAAR1 agonists addressing both mania and depression (60, 63), in addition to anxiety (64), psychosis (65), and features of ADHD (60, 66). With respect to mechanism, it bears mentioning that different TAAR1 agonists can either inhibit or stimulate activity of serotonergic neurons of the dorsal raphe nucleus (DRN) and the dopaminergic neurons of the ventral tegmental area (VTA) (39, 67). Both of these areas are known to have reciprocal projections with the anterior cingulate cortex (ACC) and the mPFC (68), a crucial hub in the top-down regulation of aggression (27).
Since TAAR1 is expressed both in the mPFC and several regions that project to it (60), there are several possible pathways by which TAAR1 activation can limit aggression. The effects of TAAR1 on aggression can primarily occur through direct glutamatergic effects in the mPFC, as TAAR1 modulates both receptors and transporters for glutamate (39, 69, 70). It has also been shown that sulpiride, a selective D2 antagonist, diminishes the aggressive social dominance of high ranking macaques (53). TAAR1 is known to desensitize D2 (71); a kind of soft ‘D2 blockade’ which may be the basis of TAAR1 agonism’s antipsychotic effects (72). In the case of the D2 autoreceptor, TAAR1-mediated desensitization should thus lead to greater propagation of DA signals across networks, and the opposite effect would apply in the case of postsynaptic D2. It is unclear which of these mechanisms dominated the results herein, but DA levels are associated with social dominance dynamics (73). DA promotes aggression through the VTA’s projection to the lateral septum (74); TAAR1 may have inhibited this pathway.
Unlike those of D2, the possible contributions of 5-HT1A are harder to specify. The absence of 5-HT in TPH2-KO rats implies that 5-HT1A should be inactive. Many experiments have nevertheless shown that 5-HT1A is constitutively active (75), even in ex vivo preparations from rats (76). Intriguingly, the 5-HT1A agonist befiradol diminishes the increased aggression of TPH2-KO rats (21). The authors of this study specifically attributed the increased aggression in TPH2-KO rats to decreased sensitivity at 5-HT1A. As it happens, TAAR1 activation sensitizes 5-HT1A (39), but it is unknown whether the sensitized conformation favors ligand binding or constitutive activity. Based on these reports, the contribution of TAAR1-mediated 5-HT1A sensitization to the attenuation of aggression in TPH2-KO rats cannot be excluded. In summary, TAAR1 may affect the mPFC through glutamate, dopamine, and serotonin to abolish abnormal aggression. These mechanisms establish the biological plausibility of TAAR1 agonists as a treatment for pathological aggression, and the outcomes of the resident-intruder tests are a proof of this concept.
5 Future directions
Despite brilliant work on the neurodevelopment of the 5-HT system (see 77 for a representative review), there has not yet been a clear definition of critical or vulnerability periods for the role of 5-HT in social aggression or self-harm. There is also the underexplored possibility of serotonin syndrome leading to aggression by paradoxical effects (e.g., hyperactivation of 5-HT1A autoreceptors). Equally interesting is the question of dietary tryptophan deprivation: To what extent does low tryptophan intake contribute to uncharacteristic acts of aggression or self-harm? This is particularly interesting vis a vis co-morbidity in eating disorders; many such cases are female. The age- and sex-differences evident in aggression and self-harm may also be contingent upon personality; this is worth confirming for more well-informed clinical practices in the future. Furthermore, TAAR1 is the only member of its family that has been explicitly studied with respect to aggression; other TAARs remain obscure. Difficult as they are to investigate, these possibilities are worthwhile given their relevance to the devastating burdens of aggression and self-harm.
6 Conclusion
Aggression and self-harm are major sociomedical problems that disproportionately occur in young people. Independent studies attribute this to a fixation on social status cues in adolescence and young adulthood. Multiple lines of evidence link 5-HT depletion to antisocial behavior across species, and the present study shows in middle-aged rats that this effect is motivated by gains in social status. Crucially, the TAAR1 agonist RO5263397 selectively abolishes this abnormal aggression in 5-HT-depleted rats. It also bears mentioning that recent independent results confirm this finding without TPH2-KO, showing that the same TAAR1 agonist as well as a 5-HT1B agonist also attenuate another form of abnormal aggression in the resident-intruder paradigm (36). These results are in line with several known interactions between TAAR1, glutamate, and canonical monoamine receptors. This establishes the biological plausibility of TAAR1 agonism as a treatment for aggressive behavior. Further study of TAAR-modulating compounds is thus warranted, especially since these mechanisms are largely conserved in primates. In this light, the efficacy of TAAR1 agonists in aggression may translate to the clinic.
Data availability statement
The original contributions presented in the study are included in the article/Supplementary Material. Further inquiries can be directed to the corresponding author.
Ethics statement
The animal study was approved by the Saint Petersburg State University Ethical Committee for Animal Research (approval 131-03-1 of 13.03.2022). The study was conducted in accordance with the local legislation and institutional requirements.
Author contributions
IZ: Conceptualization, Data curation, Formal analysis, Investigation, Methodology, Software, Validation, Visualization, Writing – original draft, Writing – review & editing. YA: Conceptualization, Data curation, Validation, Writing – review & editing, Writing – original draft. NK: Data curation, Formal analysis, Investigation, Methodology, Visualization, Writing – review & editing. VN: Conceptualization, Investigation, Writing – review & editing. KD: Data curation, Formal analysis, Methodology, Software, Visualization, Writing – review & editing. MK: Data curation, Formal analysis, Software, Supervision, Validation, Visualization, Writing – review & editing. EB: Conceptualization, Supervision, Validation, Writing – review & editing. EK: Supervision, Writing – review & editing. AK: Methodology, Supervision, Writing – review & editing, Validation. PS: Funding acquisition, Supervision, Writing – review & editing. MB: Writing – review & editing. NA: Conceptualization, Methodology, Project administration, Resources, Supervision, Validation, Writing – review & editing. RG: Conceptualization, Formal analysis, Funding acquisition, Project administration, Resources, Supervision, Validation, Writing – review & editing.
Funding
The author(s) declare that financial support was received for the research, authorship, and/or publication of this article. This research was funded by the Russian Science Foundation grant 19-75-30008-P (to RG) and project ID: 95443748 (to AK) of the St.Petersburg State University, St.Petersburg, Russia. Scientific research was performed at the Center for Molecular and Cell Technologies and Vivarium of Research Park of St. Petersburg State University.
Conflict of interest
The authors declare that the research was conducted in the absence of any commercial or financial relationships that could be construed as a potential conflict of interest.
The author(s) declared that they were an editorial board member of Frontiers, at the time of submission. This had no impact on the peer review process and the final decision.
Publisher’s note
All claims expressed in this article are solely those of the authors and do not necessarily represent those of their affiliated organizations, or those of the publisher, the editors and the reviewers. Any product that may be evaluated in this article, or claim that may be made by its manufacturer, is not guaranteed or endorsed by the publisher.
Supplementary material
The Supplementary Material for this article can be found online at: https://www.frontiersin.org/articles/10.3389/fpsyt.2024.1484925/full#supplementary-material
References
1. Fuller RW. The influence of fluoxetine on aggressive behavior. Neuropsychopharmacol. (1996) 14:77–81. doi: 10.1016/0893-133X(95)00110-Y
2. Lagerberg T, Fazel S, Sjölander A, Hellner C, Lichtenstein P, Chang Z. Selective serotonin reuptake inhibitors and suicidal behaviour: a population-based cohort study. Neuropsychopharmacol. (2022) 47:817–23. doi: 10.1038/s41386-021-01179-z
3. Healy D, Herxheimer A, Menkes DB. Antidepressants and violence: problems at the interface of medicine and law. PloS Med. (2006) 3:e372. doi: 10.1371/journal.pmed.0030372
4. Bielefeldt AØ, Danborg PB, Gøtzsche PC. Precursors to suicidality and violence on antidepressants: systematic review of trials in adult healthy volunteers. J R Soc Med. (2016) 109:381–92. doi: 10.1177/0141076816666805
5. Sharma T, Guski LS, Freund N, Gøtzsche PC. Suicidality and aggression during antidepressant treatment: systematic review and meta-analyses based on clinical study reports. BMJ. (2016) 352:i65. doi: 10.1136/bmj.i65
6. Matthews B, Minton J. Rethinking one of criminology’s ‘brute facts’: The age–crime curve and the crime drop in Scotland. Eur J Criminol. (2018) 15:296–320. doi: 10.1177/1477370817731706
7. Townsend E, Ness J, Waters K, Rehman M, Kapur N, Clements C, et al. Life problems in children and adolescents who self-harm: findings from the multicentre study of self-harm in England. Child Adolesc Ment Health. (2022) 27:352–60. doi: 10.1111/camh.12544
8. Williams K, Gilbert P, McEwan K. Striving and competing and its relationship to self-harm in young adults. Int J Cogn Ther. (2009) 2:282–91. doi: 10.1521/ijct.2009.2.3.282
9. Koski JE, Xie H, Olson IR. Understanding social hierarchies: The neural and psychological foundations of status perception. Soc Neurosci. (2015) 10:527–50. doi: 10.1080/17470919.2015.1013223
10. Fonberg E. Dominance and aggression. Int J Neurosci. (1988) 41:201–13. doi: 10.3109/00207458808990726
11. Gilbert P, Kirsten M, Chris I, Rakhee B, Rachael C, Claire B, et al. Self-harm in a mixed clinical population: The roles of self-criticism, shame, and social rank. Br J Clinic Psychol. (2010) 49:563–76. doi: 10.1348/014466509X479771
12. Greitemeyer T, Sagioglou C. Subjective socioeconomic status causes aggression: A test of the theory of social deprivation. J Pers Soc Psychol. (2016) 111:178–94. doi: 10.1037/pspi0000058
13. Siesser WB, Sachs BD, Ramsey AJ, Sotnikova TD, Beaulieu J-M, Zhang X, et al. Chronic SSRI treatment exacerbates serotonin deficiency in humanized tph2 mutant mice. ACS Chem Neurosci. (2013) 4:84–8. doi: 10.1021/cn300127h
14. Janet R, Ligneul R, Losecaat-Vermeer AB, Philippe R, Bellucci G, Derrington E, et al. Regulation of social hierarchy learning by serotonin transporter availability. Neuropsychopharmacol. (2022) 47:2205–12. doi: 10.1038/s41386-022-01378-2
15. Schafer M, Schiller D. A dominant role for serotonin in the formation of human social hierarchies. Neuropsychopharmacol. (2022) 47:2177–8. doi: 10.1038/s41386-022-01433-y
16. Chen G-L, Miller GM. Advances in tryptophan hydroxylase-2 gene expression regulation: new insights into serotonin-stress interaction and clinical implications. Am J Med Genet B Neuropsychiatr Genet. (2012) 159B(2):152–71. doi: 10.1002/ajmg.b.32023
17. Walther DJ, Peter J-U, Bashammakh S, Hörtnagl H, Voits M, Fink H, et al. Synthesis of serotonin by a second tryptophan hydroxylase isoform. Science. (2003) 299:76–6. doi: 10.1126/science.1078197
18. Alenina N, Kikic D, Todiras M, Mosienko V, Qadri F, Plehm R, et al. Growth retardation and altered autonomic control in mice lacking brain serotonin. Proc Natl Acad Sci. (2009) 106:10332–7. doi: 10.1073/pnas.0810793106
19. Mosienko V, Bert B, Beis D, Matthes S, Fink H, Bader M, et al. Exaggerated aggression and decreased anxiety in mice deficient in brain serotonin. Transl Psychiatry. (2012) 2:e122–2. doi: 10.1038/tp.2012.44
20. Beis D, Holzwarth K, Flinders M, Bader M, Wöhr M, Alenina N. Brain serotonin deficiency leads to social communication deficits in mice. Biol Letters. (2015) 11:20150057. doi: 10.1098/rsbl.2015.0057
21. Peeters DGA, De Boer SF, Terneusen A, Newman-Tancredi A, Varney MA, Verkes R-J, et al. Enhanced aggressive phenotype of Tph2 knockout rats is associated with diminished 5-HT1A receptor sensitivity. Neuropharmacology. (2019) 153:134–41. doi: 10.1016/j.neuropharm.2019.05.004
22. Meng X, Grandjean J, Sbrini G, Schipper P, Hofwijks N, Calabrese F, et al. TPH2 knockout male rats are aggressive, show less anxiety, and exhibit an altered oxytocin system (2022). Available online at: https://www.biorxiv.org/content/10.1101/2022.07.08.499134v1 (Accessed 2024 Jul 8).
23. Alonso L, Peeva P, Stasko S, Bader M, Alenina N, Winter Y, et al. Constitutive depletion of brain serotonin differentially affects rats’ social and cognitive abilities. iScience. (2023) 26:105998. doi: 10.1016/j.isci.2023.105998
24. Stockmeier CA, Shapiro LA, Dilley GE, Kolli TN, Friedman L, Rajkowska G. Increase in serotonin-1A autoreceptors in the midbrain of suicide victims with major depression—Postmortem evidence for decreased serotonin activity. J Neurosci. (1998) 18:7394–401. doi: 10.1523/JNEUROSCI.18-18-07394.1998
25. Ferrari PF, Palanza P, Parmigiani S, De Almeida RMM, Miczek KA. Serotonin and aggressive behavior in rodents and nonhuman primates: Predispositions and plasticity. Eur J Pharmacol. (2005) 526:259–73. doi: 10.1016/j.ejphar.2005.10.002
26. Quadros IM, Takahashi A, Miczek KA. CHAPTER 4.10 - serotonin and aggression, in: Handbook of behavioral neuroscience Handbook of the behavioral neurobiology of serotonin (2010). Elsevier. Available online at: https://www.sciencedirect.com/science/article/pii/S1569733910701065 (Accessed 2024 Jul 13).
27. Lischinsky JE, Lin D. Neural mechanisms of aggression across species. Nat Neurosci. (2020) 23:1317–28. doi: 10.1038/s41593-020-00715-2
28. Gainetdinov RR, Hoener MC, Berry MD. Trace amines and their receptors. Witkin JM editor. Pharmacol Rev. (2018) 70:549–620. doi: 10.1124/pr.117.015305
29. Grandy DK, Miller GM, Li J-X. TAARgeting addiction”—The alamo bears witness to another revolution. Drug Alcohol Dependence. (2016) 159:9–16. doi: 10.1016/j.drugalcdep.2015.11.014
30. Schwartz MD, Black SW, Fisher SP, Palmerston JB, Morairty SR, Hoener MC, et al. Trace amine-associated receptor 1 regulates wakefulness and EEG spectral composition. Neuropsychopharmacol. (2016) 42:1305–14. doi: 10.1038/npp.2016.216
31. Kantrowitz JT. Trace amine-associated receptor 1 as a target for the development of new antipsychotics: current status of research and future directions. CNS Drugs. (2021) 35:1153–61. doi: 10.1007/s40263-021-00864-3
32. Lam VM, Mielnik CA, Baimel C, Beerepoot P, Espinoza S, Sukhanov I, et al. Behavioral effects of a potential novel TAAR1 antagonist. Front Pharmacol. (2018) 9:953. doi: 10.3389/fphar.2018.00953
33. Leo D, Targa G, Espinoza S, Villers A, Gainetdinov RR, Ris L. Trace amine associate receptor 1 (TAAR1) as a new target for the treatment of cognitive dysfunction in alzheimer’s disease. Int J Mol Sci. (2022) 23:7811. doi: 10.3390/ijms23147811
34. Zhukov IS, Karpova IV, Krotova NA, Tissen IY, Demin KA, Shabanov PD, et al. Enhanced aggression, reduced self-grooming behavior and altered 5-HT regulation in the frontal cortex in mice lacking trace amine-associated receptor 1 (TAAR1). Int J Mol Sci. (2022) 23:14066. doi: 10.3390/ijms232214066
35. Berdoy M, Smith P, Macdonald DW. Stability of social status in wild rats: age and the role of settled dominance. Behav. (1995) 132:193–212. doi: 10.1163/156853995X00694
36. Wang L, Clark EA, Hanratty L, Koblan KS, Foley A, Dedic N, et al. TAAR1 and 5-HT1B receptor agonists attenuate autism-like irritability and aggression in rats prenatally exposed to valproic acid. Pharmacol Biochem Behavior. (2024) 245:173862. doi: 10.1016/j.pbb.2024.173862
37. Sbrini G, Brivio P, Peeva PM, Todiras M, Bader M, Alenina N, et al. The absence of serotonin in the brain alters acute stress responsiveness by interfering with the genomic function of the glucocorticoid receptors. Front Cell Neurosci. (2020) 14:128. doi: 10.3389/fncel.2020.00128
38. Black SW, Schwartz MD, Chen T-M, Hoener MC, Kilduff TS. Trace amine-associated receptor 1 agonists as narcolepsy therapeutics. Biol Psychiatry. (2017) 82:623–33. doi: 10.1016/j.biopsych.2016.10.012
39. Revel FG, Moreau J-L, Gainetdinov RR, Bradaia A, Sotnikova TD, Mory R, et al. TAAR1 activation modulates monoaminergic neurotransmission, preventing hyperdopaminergic and hypoglutamatergic activity. Proc Natl Acad Sci U S A. (2011) 108:8485–90. doi: 10.1073/pnas.1103029108
40. Bevins RA, Besheer J. Object recognition in rats and mice: a one-trial non-matching-to-sample learning task to study “recognition memory.“. Nat Protoc. (2006) 1:1306–11. doi: 10.1038/nprot.2006.205
41. Leger M, Quiedeville A, Bouet V, Haelewyn B, Boulouard M, Schumann-Bard P, et al. Object recognition test in mice. Nat Protoc. (2013) 8:2531–7. doi: 10.1038/nprot.2013.155
42. Kalueff AV, Tuohimaa P. Grooming analysis algorithm for neurobehavioural stress research. Brain Res Protoc. (2004) 13:151–8. doi: 10.1016/j.brainresprot.2004.04.002
43. Kalueff AV, Aldridge JW, LaPorte JL, Murphy DL, Tuohimaa P. Analyzing grooming microstructure in neurobehavioral experiments. Nat Protoc. (2007) 2:2538–44. doi: 10.1038/nprot.2007.367
44. Kalueff AV, Stewart AM, Song C, Berridge KC, Graybiel AM, Fentress JC. Neurobiology of rodent self-grooming and its value for translational neuroscience. Nat Rev Neurosci. (2016) 17:45–59. doi: 10.1038/nrn.2015.8
45. Koolhaas JM, Coppens CM, de Boer SF, Buwalda B, Meerlo P, Timmermans PJA. The resident-intruder paradigm: A standardized test for aggression, violence and social stress. JoVE. (2013) 77):e4367. doi: 10.3791/4367
46. Börchers S, Carl J, Schormair K, Krieger J-P, Asker M, Edvardsson CE, et al. An appetite for aggressive behavior? Female rats, too, derive reward from winning aggressive interactions. Transl Psychiatry. (2023) 13:1–7. doi: 10.1038/s41398-023-02608-x
47. Angoa-Pérez M, Kane MJ, Briggs DI, Sykes CE, Shah MM, Francescutti DM, et al. Genetic depletion of brain 5HT reveals a common molecular pathway mediating compulsivity and impulsivity. J Neurochem. (2012) 121:974–84. doi: 10.1111/j.1471-4159.2012.07739.x
48. Angoa-Pérez M, Kane MJ, Briggs DI, Francescutti DM, Kuhn DM. Marble burying and nestlet shredding as tests of repetitive, compulsive-like behaviors in mice. J Vis Exp. (2013) 82):50978. doi: 10.3791/50978
49. Cromwell HC, Berridge KC. Implementation of action sequences by a neostriatal site: A lesion mapping study of grooming syntax. J Neurosci. (1996) 16:3444–58. doi: 10.1523/JNEUROSCI.16-10-03444.1996
50. Welch JM, Lu J, Rodriguiz RM, Trotta NC, Peca J, Ding J-D, et al. Cortico-striatal synaptic defects and OCD-like behaviours in Sapap3-mutant mice. Nature. (2007) 448:894–900. doi: 10.1038/nature06104
51. Shmelkov SV, Hormigo A, Jing D, Proenca CC, Bath KG, Milde T, et al. Slitrk5 deficiency impairs corticostriatal circuitry and leads to obsessive-compulsive–like behaviors in mice. Nat Med. (2010) 16:598–602. doi: 10.1038/nm.2125
52. Burguière E, Monteiro P, Feng G, Graybiel AM. Optogenetic stimulation of lateral orbitofronto-striatal pathway suppresses compulsive behaviors. Science. (2013) 340:1243–6. doi: 10.1126/science.1232380
53. Yamaguchi Y, Lee Y-A, Kato A, Jas E, Goto Y. The roles of dopamine D2 receptor in the social hierarchy of rodents and primates. Sci Rep. (2017) 7:43348. doi: 10.1038/srep43348
54. Yu R, Geddes JR, Fazel S. Personality disorders, violence, and antisocial behavior: A systematic review and meta-regression analysis. J Pers Disord. (2012) 26:775–92. doi: 10.1521/pedi.2012.26.5.775
55. Kanen JW, Arntz FE, Yellowlees R, Cardinal RN, Price A, Christmas DM, et al. Serotonin depletion amplifies distinct human social emotions as a function of individual differences in personality. Transl Psychiatry. (2021) 11:1–12. doi: 10.1038/s41398-020-00880-9
56. Jannini TB, Lorenzo GD, Bianciardi E, Niolu C, Toscano M, Ciocca G, et al. Off-label uses of selective serotonin reuptake inhibitors (SSRIs). Curr Neuropharmacol. (2022) 20:693–712. doi: 10.2174/1570159X19666210517150418
57. Lim J-E, Pinsonneault J, Sadee W, Saffen D. Tryptophan hydroxylase 2 (TPH2) haplotypes predict levels of TPH2 mRNA expression in human pons. Mol Psychiatry. (2007) 12:491–501. doi: 10.1038/sj.mp.4001923
58. Spies M, Murgaš M, Vraka C, Philippe C, Gryglewski G, Nics L, et al. Impact of genetic variants within serotonin turnover enzymes on human cerebral monoamine oxidase A in vivo. Transl Psychiatry. (2023) 13:1–7. doi: 10.1038/s41398-023-02506-2
59. Bantjes J, Rabie S, Almirol E, Gordon S, Stewart J, Goldbeck C, et al. Associations between violence, criminality and cognitive control deficits among young men living in low resource communities in South Africa. Aggress Behav. (2020) 46:5–15. doi: 10.1002/ab.21867
60. Alnefeesi Y, Tamura JK, Lui LMW, Jawad MY, Ceban F, Ling S, et al. Trace amine-associated receptor 1 (TAAR1): Potential application in mood disorders: A systematic review. Neurosci Biobehav Rev. (2021) 131:192–210. doi: 10.1016/j.neubiorev.2021.09.020
61. Shafti M, Taylor PJ, Forrester A, Pratt D. The co-occurrence of self-harm and aggression: A cognitive-emotional model of dual-harm. Front Psychol. (2021) 12:586135. doi: 10.3389/fpsyg.2021.586135
62. McTeague LM, Goodkind MS, Etkin A. Transdiagnostic impairment of cognitive control in mental illness. J Psychiatr Res. (2016) 83:37–46. doi: 10.1016/j.jpsychires.2016.08.001
63. Shemiakova TS, Efimova EV, Gainetdinov RR. TAARs as novel therapeutic targets for the treatment of depression: A narrative review of the interconnection with monoamines and adult neurogenesis. Biomedicines. (2024) 12:1263. doi: 10.3390/biomedicines12061263
64. Alnefeesi Y, Sukhanov I, Gainetdinov RR. Ligands of the trace amine-associated receptors (TAARs): A new class of anxiolytics. Pharmacol Biochem Behavior. (2024) 242:173817. doi: 10.1016/j.pbb.2024.173817
65. Nair PC, Chalker JM, McKinnon RA, Langmead CJ, Gregory KJ, Bastiampillai T. Trace amine-associated receptor 1 (TAAR1): molecular and clinical insights for the treatment of schizophrenia and related comorbidities. ACS Pharmacol Transl Sci. (2022) 5:183–8. doi: 10.1021/acsptsci.2c00016
66. Raony Í, Domith I, Lourenco MV, Paes-de-Carvalho R, Pandolfo P. Trace amine-associated receptor 1 modulates motor hyperactivity, cognition, and anxiety-like behavior in an animal model of ADHD. Prog Neuropsychopharmacol Biol Psychiatry. (2022) 117:110555. doi: 10.1016/j.pnpbp.2022.110555
67. Revel FG, Moreau J-L, Gainetdinov RR, Ferragud A, Velázquez-Sánchez C, Sotnikova TD, et al. Trace amine-associated receptor 1 partial agonism reveals novel paradigm for neuropsychiatric therapeutics. Biol Psychiatry. (2012) 72:934–42. doi: 10.1016/j.biopsych.2012.05.014
68. Euston DR, Gruber AJ, McNaughton BL. The role of medial prefrontal cortex in memory and decision making. Neuron. (2012) 76:1057–70. doi: 10.1016/j.neuron.2012.12.002
69. Cisneros IE, Ghorpade A. Methamphetamine and HIV-1-induced neurotoxicity: Role of trace amine associated receptor 1 cAMP signaling in astrocytes. Neuropharmacology. (2014) 85:499–507. doi: 10.1016/j.neuropharm.2014.06.011
70. Espinoza S, Lignani G, Caffino L, Maggi S, Sukhanov I, Leo D, et al. TAAR1 modulates cortical glutamate NMDA receptor function. Neuropsychopharmacol. (2015) 40:2217–27. doi: 10.1038/npp.2015.65
71. Bradaia A, Trube G, Stalder H, Norcross RD, Ozmen L, Wettstein JG, et al. The selective antagonist EPPTB reveals TAAR1-mediated regulatory mechanisms in dopaminergic neurons of the mesolimbic system. Proc Natl Acad Sci. (2009) 106:20081–6. doi: 10.1073/pnas.0906522106
72. Le GH, Gillissie ES, Rhee TG, Cao B, Alnefeesi Y, Guo Z, et al. Efficacy, safety, and tolerability of ulotaront (SEP-363856, a trace amine-associated receptor 1 agonist) for the treatment of schizophrenia and other mental disorders: a systematic review of preclinical and clinical trials. Expert Opin Investigational Drugs. (2023) 32:401–15. doi: 10.1080/13543784.2023.2206559
73. Nemets VV, Deal AL, Sobolev VE, Grinevich VP, Gainetdinov RR, Budygin EA. Short-term consequences of single social defeat on accumbal dopamine and behaviors in rats. Biomolecules. (2023) 13:35. doi: 10.3390/biom13010035
74. Mahadevia D, Saha R, Manganaro A, Chuhma N, Ziolkowski-Blake A, Morgan AA, et al. Dopamine promotes aggression in mice via ventral tegmental area to lateral septum projections. Nat Commun. (2021) 12:6796. doi: 10.1038/s41467-021-27092-z
75. Newman-Tancredi A, Conte C, Chaput C, Spedding M, Millan MJ. Inhibition of the constitutive activity of human 5-HT1A receptors by the inverse agonist, spiperone but not the neutral antagonist, WAY 100,635. Br J Pharmacol. (1997) 120:737–9. doi: 10.1038/sj.bjp.0701025
76. Martel J-C, Ormière A-M, Leduc N, Assié M-B, Cussac D, Newman-Tancredi A. Native rat hippocampal 5-HT1A receptors show constitutive activity. Mol Pharmacol. (2007) 71:638–43. doi: 10.1124/mol.106.029769
77. Brummelte S, Glanaghy EM, Bonnin A, Oberlander TF. Developmental changes in serotonin signaling: implications for early brain function, behavior and adaptation. Neuroscience. (2016) 342:212. doi: 10.1016/j.neuroscience.2016.02.037
78. Berry MD, Gainetdinov RR, Hoener MC, Shahid M. Pharmacology of human trace amine-associated receptors: Therapeutic opportunities and challenges. Pharmacol Ther. (2017) 180:161–80. doi: 10.1016/j.pharmthera.2017.07.002
Keywords: serotonin, TPH2, 5HT, TAAR1, aggression, RO5263397, TAAR1 agonist, social dominance
Citation: Zhukov IS, Alnefeesi Y, Krotova NA, Nemets VV, Demin KA, Karpenko MN, Budygin EA, Kanov EV, Kalueff AV, Shabanov PD, Bader M, Alenina N and Gainetdinov RR (2024) Trace amine-associated receptor 1 agonist reduces aggression in brain serotonin-deficient tryptophan hydroxylase 2 knockout rats. Front. Psychiatry 15:1484925. doi: 10.3389/fpsyt.2024.1484925
Received: 22 August 2024; Accepted: 25 November 2024;
Published: 19 December 2024.
Edited by:
Pramod C. Nair, Flinders University, AustraliaReviewed by:
Lorenz S. Neuwirth, State University of New York at Old Westbury, United StatesYu Sun, SRI International, United States
Copyright © 2024 Zhukov, Alnefeesi, Krotova, Nemets, Demin, Karpenko, Budygin, Kanov, Kalueff, Shabanov, Bader, Alenina and Gainetdinov. This is an open-access article distributed under the terms of the Creative Commons Attribution License (CC BY). The use, distribution or reproduction in other forums is permitted, provided the original author(s) and the copyright owner(s) are credited and that the original publication in this journal is cited, in accordance with accepted academic practice. No use, distribution or reproduction is permitted which does not comply with these terms.
*Correspondence: Ilya S. Zhukov, aS5zLnpodWtvdkBzcGJ1LnJ1