- 1Brain Stimulation Mechanisms Laboratory, Division of Depression and Anxiety Disorders, McLean Hospital, Belmont, MA, United States
- 2Department of Psychiatry, Harvard Medical School, Boston, MA, United States
- 3Neuromodulation Research Facility, Butler Hospital, Providence, RI, United States
- 4Department of Psychiatry and Human Behavior, Alpert Medical School of Brown University, Butler Hospital, Providence, RI, United States
Introduction: While repetitive transcranial magnetic stimulation (rTMS) is effective for 50-60% of those treatment-resistant depression, it is critical to identify predictors of response for optimal patient selection to improve therapy. Insomnia is a known symptom of depression that is both correlated with depression severity and associated with poor antidepressant response. Therefore, understanding this relationship may open new opportunities for the optimization of rTMS treatment. We aimed to explore whether baseline sleep quality, specifically insomnia, is associated with rTMS outcomes in a naturalistic sample of 975 patients (age 18-90; 63.9% F) receiving a standard course of rTMS treatment from two outpatient TMS clinics located within psychiatric hospitals in the United States. One site additionally collected information on concurrent medication use on 350 patients; among these, we examined whether pharmacological treatment of insomnia affected TMS treatment response.
Methods: Depression was measured using the 30-item Inventory of Depressive Symptomology Self Report (IDS-SR) in site one and an abbreviated 16-item Quick Inventory of Depressive Symptomology (QIDS) derived from the IDS-SR in site two. Sleep disturbances were measured using three insomnia-related questions. Multilevel logistic regression was used to determine whether baseline insomnia scores were associated with TMS treatment outcome. Upon dichotomous categorization of the sample by insomnia and sleep-medication use, depression and sleep scores were analyzed across time using mixed repeated measures ANOVA.
Results: We found that sleep quality improves after TMS (p<.001) and correlates with improvement in non-insomnia related depression symptoms (r= .318, p<.001). We found that among those who had significant insomnia at baseline, those not using sleep medications had significantly worse post-treatment IDS-SR scores compared to those using sleep medications (p=. 021) despite no difference in final insomnia score.
Discussion: Together, our results suggest that while baseline insomnia is not associated with TMS effectiveness, treating insomnia may affect the trajectory of TMS therapy. Future prospective studies are needed to examine the effect of insomnia treatment alongside TMS for depression.
Introduction
Though treatments for major depressive disorder have significantly improved over the years, we have yet to understand why certain individuals improve while others do not. For those who fail to improve with medications – estimated to be potentially up to 30% of patients (1), repetitive transcranial magnetic stimulation (rTMS) has emerged as a revolutionary therapy. However, even TMS response rates are limited to 45-60% with remission rates around 30% (2–4). Understanding who will potentially improve with TMS treatment may guide patient selection and provide insights into pharmacologic augmentation strategies (5).
rTMS is an FDA-cleared treatment for treatment resistant depression. During rTMS, a coil rests on the scalp and current is rapidly discharged through wire coils to generate a focused magnetic wave (6). Frequencies at 5-Hz or greater are believed to have excitatory modulatory effects (7), and are conventionally delivered to the dorsolateral prefrontal cortex to treat depression. At the neuronal level, 10-Hz rTMS appears to mediate effects through synaptic plasticity (8–12), with evidence in animals and humans that long-term potentiation (LTP) and LTP-like effects are produced (13).
Factors that influence plasticity, such as sleep, may therefore influence TMS outcomes. Literature has demonstrated changes in neural plasticity associated with sleep and depression (14). Sleep deprivation for example, has also been shown to disturb synaptic plasticity in both mice (15) and in humans (16). Sleep disturbances are a well-established symptom of depression that have been correlated with overall depression severity (17). Sleep quality has been previously studied as a promising predictor of treatment outcome across modalities, with research finding objective and subjective measures of sleep disturbance to be associated with poor depression treatment response (18–20). Therefore, understanding this relationship may open new opportunities for the optimization of rTMS treatment. Patients with depression have demonstrated abnormal REM sleep (21) and disrupted sleep architecture (22) associated with treatment outcome (23). Patients with comorbid insomnia and depression tend to require longer durations of treatment and lower remission rates across therapies (21), suggesting that poor sleep quality may affect the trajectory of treatment for depression.
Not only does insomnia potentially blunt improvement from depression therapies, but alleviating insomnia through targeted treatment has led to improvements in depression in patients with comorbid conditions. A sham-controlled trial found CBT-I with antidepressant treatments produced better outcomes than antidepressants alone (24). Furthermore, Watanabe et al. (2011) conducted a pilot study where patients with residual insomnia and depression despite antidepressants received CBT-I and saw significant improvement in both domains (25). A meta-analysis of 23 studies suggests a positive effect of insomnia treatment on depression outcomes, though interventions and populations were highly variable (26). Interestingly, sleep quality has also been found to facilitate plasticity processes such as changes in spine density (27). Considering 10-Hz rTMS has been demonstrated to increase spine size in mouse hippocampal slices (12), for example, this raises the possibility that better sleep could improve rTMS effectiveness through a shared mechanism. Taken together, it is plausible that sleep quality could modulate the trajectory of rTMS treatment for depression.
Despite the breadth of evidence that suggests insomnia influences depression treatment, current literature conveys mixed evidence on the influence of sleep quality on rTMS response. Lowe et al. (2013) found no relation between baseline insomnia or hypersomnia and rTMS treatment outcome in an analysis of data pooled from four clinical trials using rTMS treatment for depression (n=139) (28). Brakemeier et al. (2007) reported that patients (n=70) with worse baseline sleep had greater likelihood of TMS-related improvement; however, they were not able to replicate these findings in a follow-up study (29, 30). One study (n=195) initially found that early insomnia was related to worse outcome, but the result did not survive after adjusting for trial location heterogeneity (31). Together, these suggest baseline insomnia may not be a strong predictor of TMS treatment response. It is important to note, however, that Lowe et al. (2013) combined data from four trials all using varied frequencies, intensities, and number of sessions. These differing TMS parameters may enact disparate effects on brain networks (32), including those affecting sleep. Although other studies did control for location in analyses, data was pooled from 6 clinical trials that gave only 10 days of treatment (31), in comparison to the average 30-36 days in a standard rTMS treatment course. Finally, no study examined the potential role of sleep modulators, such as sleep mediations, and how this may have impacted rTMS outcome.
To address these gaps, we used naturalistic data with the largest sample size to date to parse out the role of insomnia in rTMS treatment response as well as investigating the role of sleep medications. We hypothesized that self-report baseline insomnia is not associated with clinical outcomes, per previous studies (28, 30, 31), but that pharmacologic treatment of insomnia improves rTMS effectiveness for depression.
Methods
Data were retrospectively analyzed from the medical records of 353 naturalistically treated adult outpatients in the Butler Hospital TMS Clinic and 630 patients in the McLean Hospital TMS Clinic who received their first course of TMS with at least 30 sessions. Patient characteristics are reported in Table 1. Butler Hospital patients complete the Inventory of Depressive Symptomatology Self Report (IDS-SR) and Patient Health Questionnaire-9 (PHQ-9). McLean Hospital patients complete the Quick Inventory of Depressive Symptomology (QIDS) and PHQ-9. Both sites collected data before and after the course, and at interim time points throughout treatment (every 5 treatments at Butler, and every 10 treatments at McLean). All patients had a primary diagnosis of moderate-severe depression without psychotic features, inadequate or intolerable response to psychotherapy, and at least two (in most cases at least four) antidepressant and/or augmentation medications. Patients were evaluated by a psychiatrist specializing in mood disorders. Patients were on stable medication regimens before starting TMS and were instructed to keep regimens stable throughout the course of TMS.
TMS protocol
On the first day of treatment, patients underwent a motor threshold procedure to determine the left hemisphere motor ‘hotspot’ and minimum stimulator intensity required to produce a finger twitch for >50% trials. At Butler, patients then began a standard 10-Hz treatment protocol delivering 3,000 pulses a day for 6 weeks, 5 times a week, followed by 6 sessions over 3 weeks. Butler patients were treated with a NeuroStar iron core coil (Neuronetics, 2003) over the left dorsolateral prefrontal cortex (dlPFC) at a stimulation intensity 120% of their resting motor threshold (rMT). In ~60% of cases (n=101), where patients had difficulty tolerating the 10-Hz protocol at 120% MT, they received 5-Hz stimulation (3,000 pulses). A minority of patients who transitioned to 1-Hz stimulation over the right dlPFC (12 patients received 1-Hz for more than 50% of treatment sessions) were excluded from our analysis. For a small number of patients without improvement, the total number of pulses per session was increased to 4000.
McLean TMS patients were treated on either a MagVenture B70 figure-8 coil (5% of patients) or a BrainsWay H1 coil (95%). The BrainsWay protocol entailed 18-Hz stimulation at 120% of rMT for 1980 pulses five days per week for 36 consecutive treatments. A small minority (~2%) of patients were switched from BrainsWay for tolerability. MagVenture protocols included intermittent theta-burst stimulation (iTBS) on the left, 1-Hz or continuous (c)TBS on the right, or bilateral. All Butler patients were outpatient, while 116 of the 630 at McLean began as inpatients.
Clinical assessment
Clinical response was defined as a decrease in score by ≥50% from baseline to post-treatment. Remission was defined by a post treatment score ≤14 on the IDS-SR and ≤5 on the QIDS. As all QIDS items are included within the IDS-SR, a comparative QIDS score was also calculated for the Butler dataset. The three insomnia-related questions were determined as items 1 (Falling asleep), 2 (Waking up during the night and difficulty falling back to sleep), and 3 (Waking up too early). Each question had a score range from 0-3, with 0 representing no sleep disturbance (ex. “I never take longer than 30 minutes to fall asleep”) and 3 representing the most severe (ex. “I take more than 60 minutes to fall asleep, more than half the time”). The scores of these three questions were summated to create an “insomnia score” with a range from 0-9. Item 4 (Sleeping too much) was not included to separate insomnia from hypersomnia-like sleep disturbances. We then calculated an IDS-SR25 total score excluding insomnia items for analyses comparing insomnia to other depressive symptoms rated on the same scale, and likewise for QIDS to create a QIDS13. Previous studies (28, 29, 31) have used item-level sleep questions from the Hamilton depression rating scale (early (‘complains of nightly difficulty falling asleep’), middle (‘waking during the night except for the purpose of voiding’), and late insomnia (‘unable to fall asleep again if he/she gets out of bed’). Insomnia was defined as a score of 2 on at least two questions. Similarly, we used the 3 QIDS sleep questions to evaluate early, middle, and late sleep disturbances. Our methods are in line with those used in previous work, though we used a different depression scale. Of note, the QIDS-SR16 has demonstrated high correlation with the Hamilton scale (33).
Sleep medications
Furthermore, we examined whether using sleep medications was associated with improvement in 1) sleep and 2) depression. Patients at both sites were instructed to keep medications stable through TMS. We included the following medications commonly prescribed for sleep impairment in our definition of sleep-aids: doxepin (Sinequan, Silenor), ramelteon (Rozerem), temazepam (Restoril), triazolam (Halcion), zaleplon (Sonata), zolpidem (Ambien, Zolpimist, Edluar, Intermezzo), eszopiclone (Lunesta), suvorexant (Belsomra). Three antidepressants- trazadone (Desyrel), amitriptyline (Elavil), and mirtazapine (Remeron) were also included, along with melatonin. Anti-anxiety medications were not included in our filter as we aimed to focus in on medications used more exclusively for insomnia. For Butler patients, we filtered through medication lists recorded on the first day of TMS treatment and those taking sleep medications during TMS were coded “1” and otherwise coded “0.”
To assess whether patients with insomnia taking sleep medications had clinical responses to TMS comparable to those without, we categorized patients into four groups by sleep quality x medication use: 1) No/low insomnia and not using sleep-aids (“-Insomnia -Meds”) 2) no/low insomnia using sleep aids (“-Insomnia +Meds”) 3) high insomnia and not using sleep-aids (“+Insomnia -Meds”) 4) high insomnia despite use of sleep-aids (+-Insomnia +Meds”).
Statistical analysis
Categorical (responders, remitters) and continuous (percent and raw change in baseline to endpoint for IDS-SR, QIDS, and insomnia score) outcomes were explored with descriptive statistics. IDS-SR, QIDS, and insomnia scores were not normally distributed. Wilcoxon Signed Rank tests were used to determine within subject differences (baseline to post comparisons) and Mann-Whitney U tests were used to determine differences between groups in sleep quality and medication. Statistical significance was defined at p<.05 and two-tailed. We also analyzed correlations in sleep score with change in overall symptom improvement using Spearman correlation tests.
To determine whether baseline sleep scores predicted TMS outcome, we performed a multilevel logistic regression using the lme4 package (v1.1.33 (34);) with response as categorical outcome variable and baseline sleep, age, sex, and inpatient/outpatient status as fixed effects. Hospital site was included as a random effect to account for a potential influence of variance in the structured data. The above analysis was repeated with remission as outcome variable.
To create a categorial variable “sleep quality,” we chose the median score 4 as the cutoff score based on the histogram and descriptive statistics of baseline insomnia score (Supplementary Figure 1). Patients with baseline insomnia score with less than or equal to 4 were coded as 0 and those with a score greater than 4 were coded as 1. We then compared IDS-SR25/QIDS18 scores between no/low insomnia group and high insomnia group.
Kruskal Wallis Test was used to determine differences in baseline and post treatment IDS-SR and insomnia scores between the four groups. To analyze scores across time, mixed repeated measures ANOVA was applied. All statistical analysis was done in R (v4.3.1; R Core Team 2021).
Results
Demographics
Between August 2016 and July 2022, 350 patients (245 F, age 18-84) completed a baseline and post rTMS questionnaire at Butler Hospital, and 630 patients (383 F, age 18-90) between October 2017 and April 2023 at McLean Hospital. Demographic and clinical characteristics are presented in Table 1. There was a significant decrease in QIDS score from baseline to post-treatment scores (Z= -24.3, p<.001, r= -.778, Figure 1A).
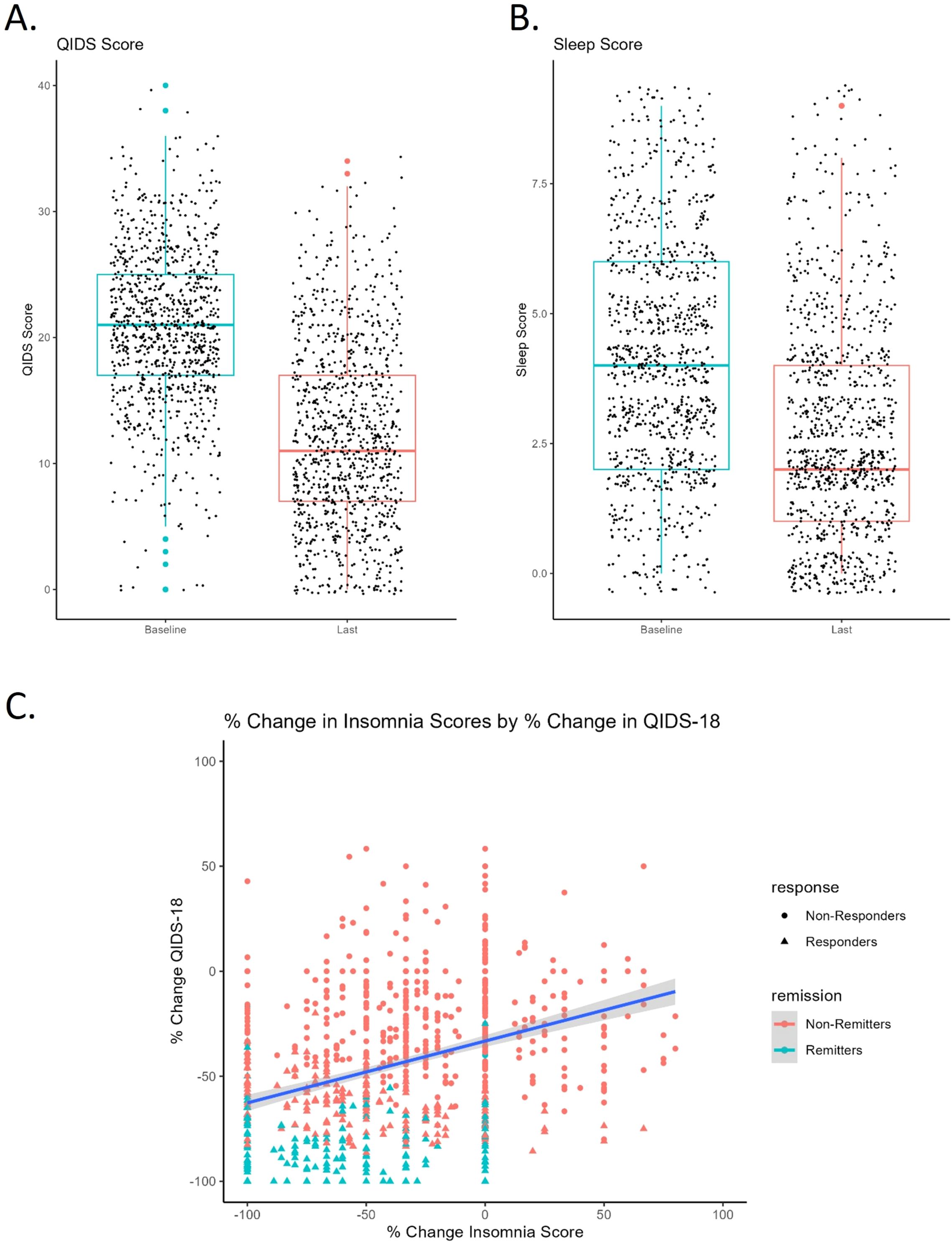
Figure 1. (A) Pre to post-treatment QIDS score with standard deviation (error bars) and individual data points. Colored dots represent outliers. Pre (20.8 ± 6.18), Post (12.2 ± 7.24). (B) Pre to post-treatment insomnia score with standard deviation (error bars) and individual data points. Colored dots represent outliers. Pre (4.33 ± 2.10), Post (2.88 ± 2.00). (C) Change in insomnia score positively correlates with change in QIDS18 score pre to post TMS. Negative percent change reflects improvement in symptoms (score reduction).
Effect of TMS on sleep
Average baseline insomnia score was 4.33 ± 2.10. We found no significant differences by sex (Z= -1.24, p= .214, r= -.039) or correlation with age (r= .053, p= .094). Patients with greater baseline insomnia tended to have greater baseline QIDS scores, i.e., more severe depression (r=.57, p<.001). Insomnia scores significantly improved over the course of rTMS treatment (Z= -16.45, p<.001, r= -.519, Figure 1B).
After responses to the three insomnia-related sleep questions were subtracted, we found percent change in insomnia score correlated in a positive direction with percent change in QIDS18 (r=.318, p<.001, Figure 1C), indicating that sleep improved alongside general improvement in depression after TMS.
Baseline sleep and TMS clinical outcome
To determine whether baseline sleep disturbance was associated with TMS response or remission rates, we first examined whether baseline and final insomnia/QIDS scores differed between responders and non-responders. Responders had a significantly higher initial insomnia score than non-responders (Z= -2.54, p=.011, r= -.081). After TMS, responders had significantly lower insomnia scores than non-responders (Z= -12.64, p<.001, r= -.404), as well as greater decrease in insomnia score as measured by percent change (Z= -14.42, p<.001, r= -.467). By contrast, remitters had significantly lower insomnia scores at baseline (Z= -3.11, p=.002, r= -.098) which persisted after TMS (Z= -13.84, p<.001, r= -.443).
A binary logistic multilevel model (MLM) with responder status as dependent variable and baseline insomnia, baseline QIDS18 score, sex, age, and inpatient status as fixed effects, controlled for location, did not show a significant effect of baseline insomnia on response status. Similarly, a logistic regression model using baseline sleep to predict remission status with the same covariates showed no significant effect of insomnia on remission outcome when QIDS18 was included. Baseline insomnia did not predict TMS treatment outcome.
Insomnia as a modulator of TMS outcome
We went on to explore whether baseline insomnia influences the trajectory of symptom improvement. Using the Butler data set, insomnia and IDS-SR25 scores were plotted every 5 TMS sessions by better and worse sleepers. We found that patients with initially no/minimal insomnia have consistently lower scores for both insomnia and IDS-SR25 score across treatment course (Figures 2A, B). This difference was found to be significant by mixed repeated measures ANOVA, which produced significant effects of time (F(3.46, 443)=142.2, p<.001, η2= .03), quality (F(1,128)=5.176, p=.025, η2= .23), but not time by quality interaction.
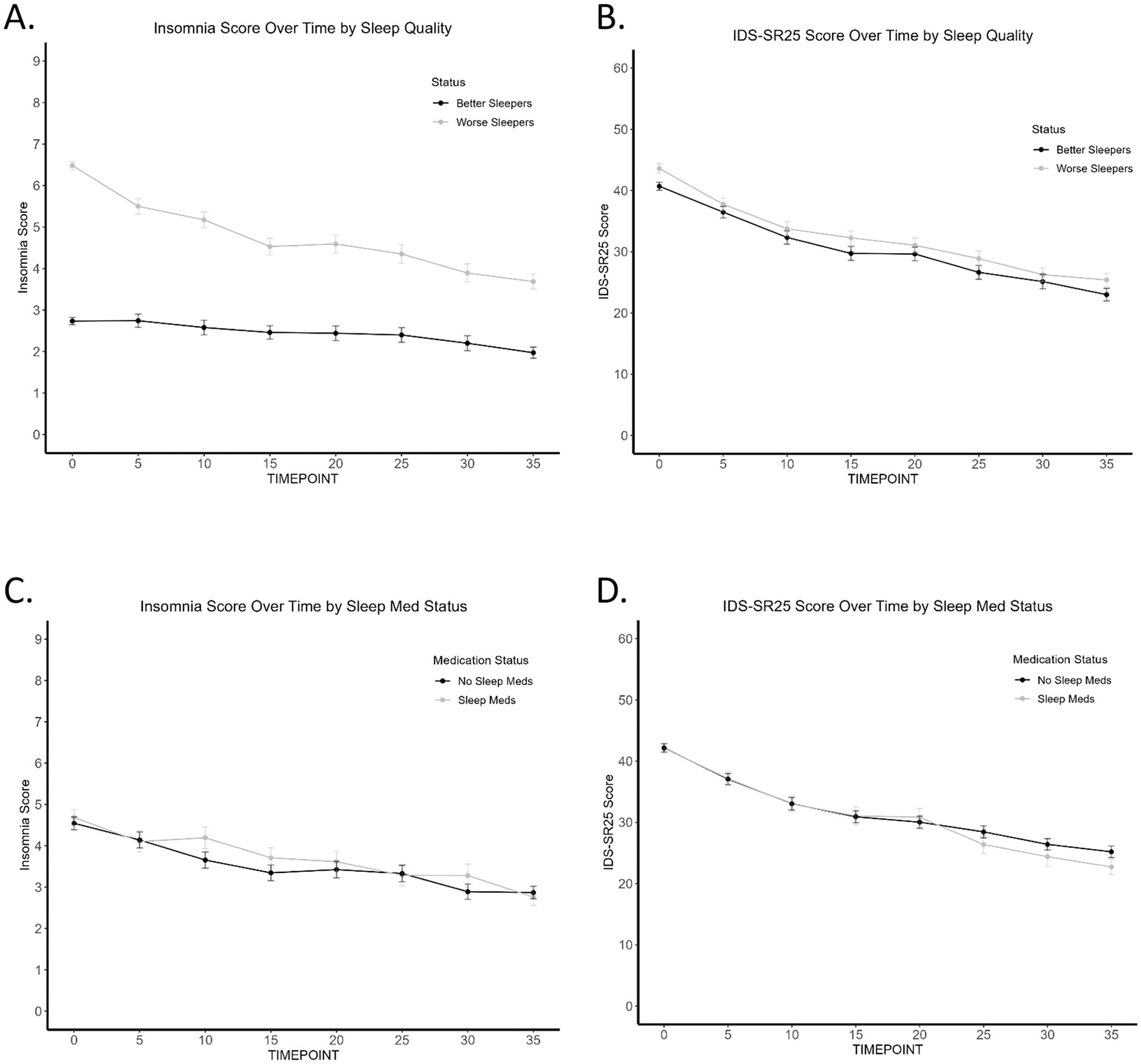
Figure 2. Trajectory of Insomnia and IDS-SR25 score over TMS treatment course (Butler). (A) Worse sleepers have consistently worse sleep scores across TMS treatment course. (B) Worse sleepers have consistently worse IDS-SR25 scores across TMS treatment course. (C) No difference in insomnia scores across TMS treatment course by sleep medication use. (D) No difference in IDS-SR25 scores across TMS treatment course by sleep medication use.
Sleep medications
To account for the use of sleep medications and their impact on sleep in our naturalistic sample, we found that patients taking sleep medications did not have significantly different baseline/final insomnia or IDS-SR25 scores when compared to patients not taking sleep medications. 243 (69.4%) participants in the Butler cohort were taking antidepressants at the start of TMS treatment. 144 (41.1%) patients were taking one or more of the sleep medications included in our analysis. Figures 2C, D show no separation in sleep scores and overall depression scores, respectively, between the two medication groups. Regardless of whether sleep medications were used, TMS treatment improved insomnia (effect of time; F(4.76, 609.44)=20.02, p<.001, η2=.04) and other depression symptoms (effect of time; F(3.53, 451.46)=140.82, p<.001, η2= .22). Sleep medication status was not associated with response or remission outcome, based on chi-square analysis.
Insomnia and medication use
Finally, we sought to determine whether sleep medications influence treatment outcome through modulation of sleep. Table 2 shows the number of patients in each group, mean sleep score, and mean IDS-SR25 score before and after TMS. Mixed ANOVA reveals that within patients with no/minimal insomnia, there is no difference in insomnia scores across TMS course between patients taking or not taking sleep medications (F(1, 76)= .208, p=.65, η2= .002, or in patients with insomnia by medication status (F(1,72)= .115, p=.736, η2=.001).
Examining IDS-SR25 scores over time by sleep quality group (no/low vs. high insomnia) and hypnotic medication use status (Figure 3A) revealed that patients taking sleep medications demonstrated no significant difference to those with healthy sleep and not taking sleep-aids. Notably, while the high insomnia group taking sleep medications had significantly higher baseline IDS-SR scores than patients with no/low initial insomnia, all three groups ended at comparable final depression scores. This effect was not due to insomnia improvement, as they had higher insomnia scores at the end of treatment. In contrast, patients with high insomnia not taking medications showed the least overall improvement with significantly higher IDS-SR25 scores post-TMS than the other three groups (Figure 3B). We found an overall significant effect of group (F(3,4)=7.37, p= .042, η2=.79, time (F(7,28)=3.28, p=.011, η2=.21, but not group x time interaction, with significant Kruskal-Wallis at final time point 35 (p=.04). Patients using sleep medications appear to have greater improvement in overall depression symptoms, even if insomnia does not significantly improve.
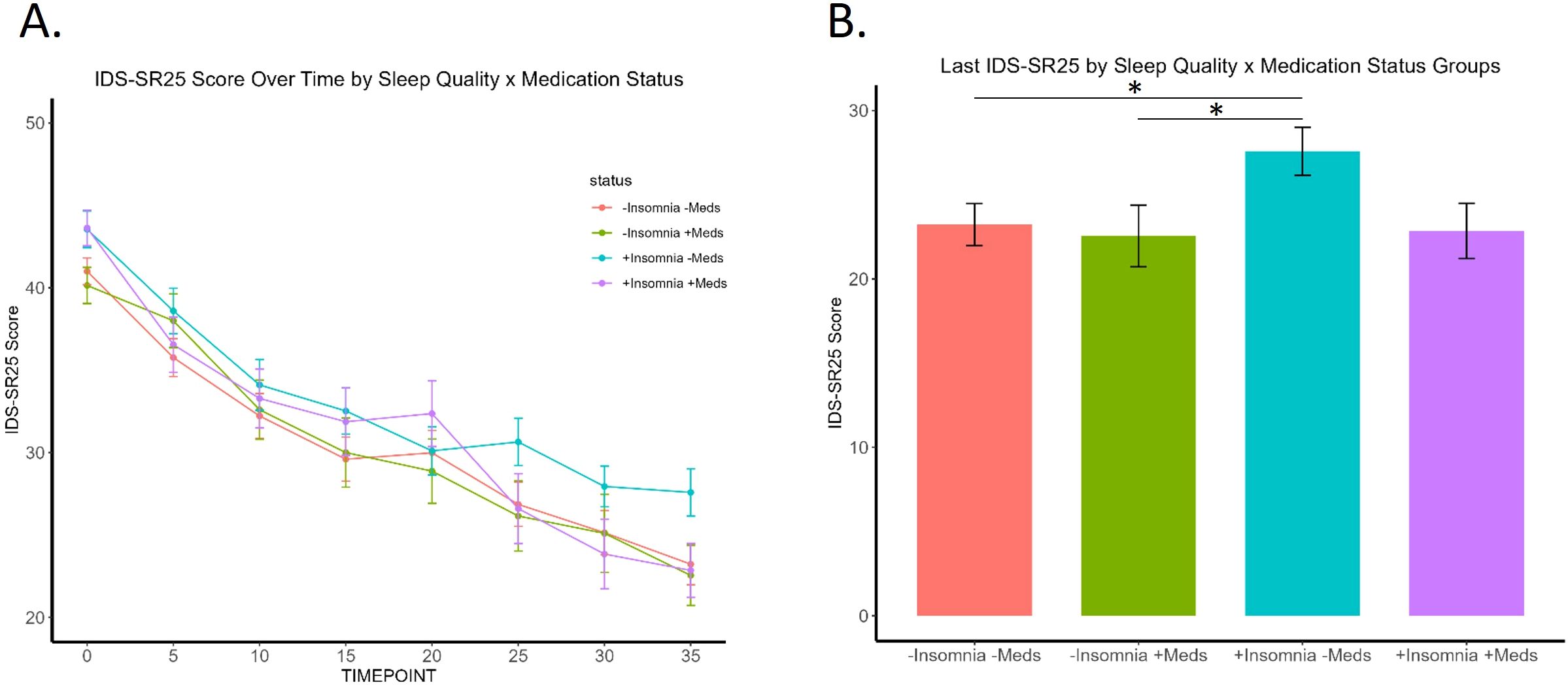
Figure 3. Trajectory of patients by the four sleep quality and medication use groups. (A) Significant effect of group (p=.04) and time (p=.01), but not group x time interaction. (B) “+Insomnia -Meds” group shows significantly higher final IDS-SR25 score at timepoint 35 compared to "-Insomnia -Meds" (p= .032) and "-Insomnia +Meds" (p= .039) groups. * signifies p <.05.
Discussion
We found that patients using sleep medications see improvement in overall depression even if subjective sleep measures do not significantly improve. Upon categorization by insomnia severity and sleep medication use, patients using sleep medications show a drop in IDS-SR score and end at a similar position to better sleepers. In line with our hypothesis, treating insomnia appears to enhance TMS effectiveness, while baseline sleep quality does not predict TMS treatment outcomes, in line with the majority of other studies that have examined sleep as a predictor of response and found no relation between the two measures (28, 30, 31).
One potential underlying link between sleep, depression, and TMS treatment is synaptic plasticity. Impairments in the brain’s ability to reorganize and respond to changing stimuli, such as through LTD in the prefrontal cortex, have been implicated with depression (35). Studies in both animals (12, 36) and humans (8, 37, 38) suggest that rTMS enacts therapeutic change through the restoration of impaired synaptic plasticity in patients with depression. Interestingly, sleep has been found to modulate synaptic plasticity directly. For example, in a study using high-frequency electrical stimulation in the motor cortex of rats to induce synaptic plasticity, effects were partially occluded after prolonged wakefulness, and then restored after sleep (15). Similar results were found in humans; one night of sleep deprivation blunted the facilitatory effect of paired-associative stimulation (PAS) on motor-evoked potentials (16). Therefore, patients with both depression and sleep disturbances may have further impaired plasticity processes that hinder rTMS effects. Following this logic, improving sleep through medications, such as hypnotics, may help restore plasticity mechanisms and aid rTMS treatment.
One promising biomarker that may reveal greater insight into the relationship between sleep, depression, and rTMS is brain-derived neurotrophic factor (BDNF). The role of BDNF in synaptic plasticity has been well-established (39) and BDNF levels have been shown to increase alongside slow-wave activity (SWA) following ketamine therapy for depression specifically in responders (40). BDNF polymorphism has also demonstrated a potential influence on rTMS-induced memory performance (41). Furthermore, work in rodents show increased SWA following BDNF injection that can be blocked by administration of BDNF receptor inhibitors (42). Taken together, BDNF levels may offer a physiological signal to quantify both sleep quality in correlation with SWA and underlying plasticity modulating TMS treatment outcome.
Several meta-analyses have reported that concurrent cognitive-behavioral therapy for insomnia (CBT-I) may improve the efficacy of anti-depressant treatment (26, 43, 44). No studies have yet examined targeted CBT-I treatment alongside rTMS, with the exception of one open-label feasibility trial with 2 patients undergoing a 36-day TMS treatment course (10-Hz, 3,000 pulses) with six weekly 1-hour manualized CBT-I sessions (45). Both patients experienced significant improvement in subjective sleep rating and reached remission as measured by the 24-item Hamilton Rating Scale for Depression-24 (HRSD24). Our findings provide support that treating sleep alongside TMS treatment may produce synergistic antidepressant effects. Prospective work is needed to determine if improving sleep improves TMS outcomes, both with medications and non-pharmaceutical interventions such as cognitive-behavior therapy for insomnia.
Our interpretations are limited by confounding variables that cannot be controlled with retrospective, naturalistic data, such as the wide variety of medication regimes that have varying effects on sleep. Lifestyle factors have also been found to influence TMS-induced plasticity and potentially TMS outcomes. In preclinical studies, individuals with chronic caffeine use have demonstrated blunted motor plasticity following rTMS relative to non-caffeine users (46), while musicians and athletes exhibit enhanced rTMS-induced motor plasticity (47). We also did not include in our filter criteria other drugs that are not strictly categorized as sleep medications but commonly used to treat sleep, such as benzodiazepines or marijuana. As a result, it is difficult to definitively claim that treating sleep with medications improves TMS outcome. Sleep medications also impact motor threshold and may impact the intensity of stimulation delivered for TMS treatment. Finally, we did not have data regarding length, timing, or dosages of sleep medication use, which limits our ability to conclusively determine a causal relationship with and TMS outcomes.
A percentage of the sample received predominantly 5-Hz rTMS, or iTBS, which also may have differential effects on sleep and depression. rTMS delivered at 5-Hz and greater is considered “excitatory” and considered identical in clinical application. However, research has demonstrated that parameters such as frequency, pulse number or train duration can influence or even reverse effects on brain excitability (48). Therefore, we cannot state that these various forms of excitatory rTMS work through identical pathways to improve depression and sleep. An exploration of the potential relationship between TMS parameters and sleep (and depression) is still needed.
A major limitation of our study is that there were no objective measures of sleep. Past studies have previously reported discrepancies between subjective and objective ratings of sleep quality. Future studies would benefit from including objective measures such as actigraphy and EEG-based quality and quantity of sleep architecture or changes in brain states to corroborate subjective sleep experience and perhaps yield new insight into how treatment of sleep during TMS may predict overall depression improvement to treatment. The creation of the dichotomous “no/low” and “high” insomnia groups using the mean insomnia score of 4, in line with the method used in Fava et al. (2002), may not be an accurate categorization of insomnia severity (49). An individual who scored a 3 on a single item would be categorized by our methods as “no/low” insomnia, though endorsing for example, “I take more than 60 minutes to fall asleep, more than half the time.” As insomnia involves different dimensions of sleep, and there are interindividual differences in phenotype, binary categorization of severity presents a challenge.
Understanding what factors influence TMS treatment and in what direction are critical for patients to maximize the benefits of TMS. Our findings suggest that while baseline sleep quality is not predictive of TMS clinical outcomes, modulating sleep may impact the trajectory of symptom improvement during a treatment course.
Data availability statement
Data set includes identifiable information and is overseen by the Butler Hospital TMS Clinic. Requests to access these datasets should be directed to QUdvYmluQGJ1dGxlci5vcmc=.
Ethics statement
The studies involving humans were approved by Butler Hospital Institutional Review Board. The studies were conducted in accordance with the local legislation and institutional requirements. Written informed consent for participation was not required from the participants or the participants’ legal guardians/next of kin in accordance with the national legislation and institutional requirements.
Author contributions
JK: Writing – review & editing, Writing – original draft, Methodology, Formal Analysis. AF: Writing – review & editing, Methodology, Conceptualization. AG: Writing – review & editing, Data curation. LH: Writing – review & editing, Methodology, Data curation. LC: Writing – review & editing, Conceptualization. JB: Writing – review & editing, Conceptualization.
Funding
The author(s) declare that no financial support was received for the research, authorship, and/or publication of this article.
Acknowledgments
We thank Megan Vigne for editorial support. This manuscript has been previously uploaded on MedRxIV (50).
Conflict of interest
The authors declare that the research was conducted in the absence of any commercial or financial relationships that could be construed as a potential conflict of interest.
The reviewer DC declared a shared parent affiliation with the authors AF and JB to the handling editor at the time of review.
Publisher’s note
All claims expressed in this article are solely those of the authors and do not necessarily represent those of their affiliated organizations, or those of the publisher, the editors and the reviewers. Any product that may be evaluated in this article, or claim that may be made by its manufacturer, is not guaranteed or endorsed by the publisher.
Supplementary material
The Supplementary Material for this article can be found online at: https://www.frontiersin.org/articles/10.3389/fpsyt.2024.1458696/full#supplementary-material
Supplementary Figure 1 | Histogram of baseline insomnia score.
References
1. Gaynes BN, Rush AJ, Trivedi MH, Wisniewski SR, Spencer D, Fava M. The STAR*D study: treating depression in the real world. Cleve Clin J Med. (2008) 75:57–66. doi: 10.3949/ccjm.75.1.57
2. Dunner DL, Aaronson ST, Sackeim HA, Janicak PG, Carpenter LL, Boyadjis T, et al. A multisite, naturalistic, observational study of transcranial magnetic stimulation for patients with pharmacoresistant major depressive disorder: durability of benefit over a 1-year follow-up period. J Clin Psychiatry. (2014) 75:1394–401. doi: 10.4088/JCP.13m08977
3. Carpenter LL, Janicak PG, Aaronson ST, Boyadjis T, Brock DG, Cook IA, et al. Transcranial magnetic stimulation (TMS) for major depression: a multisite, naturalistic, observational study of acute treatment outcomes in clinical practice. Depress Anxiety. (2012) 29:587–96. doi: 10.1002/da.21969
4. George MS, Taylor JJ, Short EB. The expanding evidence base for rTMS treatment of depression. Curr Opin Psychiatry. (2013) 26:13–8. doi: 10.1097/YCO.0b013e32835ab46d
5. Sohn MN, Brown JC, Sharma P, Ziemann U, McGirr A. Pharmacological adjuncts and transcranial magnetic stimulation-induced synaptic plasticity: a systematic review. J Psychiatry Neurosci. (2024) 49:E59–76. doi: 10.1503/jpn.230090
6. Edinoff AN, Hegefeld TL, Petersen M, Patterson JC 2nd, Yossi C, Slizewski J, et al. Transcranial magnetic stimulation for post-traumatic stress disorder. Front Psychiatry. (2022) 13:701348. doi: 10.3389/fpsyt.2022.701348
7. Ziemann U. TMS induced plasticity in human cortex. Rev Neurosci. (2004) 15:253–66. doi: 10.1515/REVNEURO.2004.15.4.253
8. Brown JC, Higgins ES, George MS. Synaptic plasticity 101: the story of the AMPA receptor for the brain stimulation practitioner. Neuromodulation. (2022) 25(8):1289–98. doi: 10.1016/j.neurom.2021.09.003
9. Brown JC, DeVries WH, Korte JE, Sahlem GL, Bonilha L, Short EB, et al. NMDA receptor partial agonist, d-cycloserine, enhances 10 Hz rTMS-induced motor plasticity, suggesting long-term potentiation (LTP) as underlying mechanism. Brain Stimul. (2020) 13:530–2. doi: 10.1016/j.brs.2020.01.005
10. Brown JC, Yuan S, DeVries WH, Armstrong NM, Korte JE, Sahlem GL, et al. NMDA-receptor agonist reveals LTP-like properties of 10-Hz rTMS in the human motor cortex. Brain Stimul. (2021) 14:619–21. doi: 10.1016/j.brs.2021.03.016
11. Kweon J, Vigne M, Jones R, George MS, Carpenter LL, Brown JC. A replication study of NMDA receptor agonism sufficiency to enhance 10-Hz rTMS-induced motor cortex plasticity. Brain Stimul. (2022) 15:1372–4. doi: 10.1016/j.brs.2022.09.014
12. Vlachos A, Muller-Dahlhaus F, Rosskopp J, Lenz M, Ziemann U, Deller T. Repetitive magnetic stimulation induces functional and structural plasticity of excitatory postsynapses in mouse organotypic hippocampal slice cultures. J Neurosci. (2012) 32:17514–23. doi: 10.1523/JNEUROSCI.0409-12.2012
13. Brown JC, Higgins ES, George MS. Synaptic plasticity 101: the story of the AMPA receptor for the brain stimulation practitioner. Neuromodulation. (2022) 25:1289–98. doi: 10.1016/j.neurom.2021.09.003
14. Zhang MQ, Li R, Wang YQ, Huang ZL. Neural plasticity is involved in physiological sleep, depressive sleep disturbances, and antidepressant treatments. Neural Plast. (2017) 2017:5870735. doi: 10.1155/2017/5870735
15. Vyazovskiy VV, Cirelli C, Pfister-Genskow M, Faraguna U, Tononi G. Molecular and electrophysiological evidence for net synaptic potentiation in wake and depression in sleep. Nat Neurosci. (2008) 11:200–8. doi: 10.1038/nn2035
16. Kuhn M, Wolf E, Maier JG, Mainberger F, Feige B, Schmid H, et al. Sleep recalibrates homeostatic and associative synaptic plasticity in the human cortex. Nat Commun. (2016) 7:12455. doi: 10.1038/ncomms12455
17. Sunderajan P, Gaynes BN, Wisniewski SR, Miyahara S, Fava M, Akingbala F, et al. Insomnia in patients with depression: a STAR*D report. CNS Spectr. (2010) 15:394–404. doi: 10.1017/S1092852900029266
18. Andreescu C, Mulsant BH, Houck PR, Whyte EM, Mazumdar S, Dombrovski AY, et al. Empirically derived decision trees for the treatment of late-life depression. Am J Psychiatry. (2008) 165:855–62. doi: 10.1176/appi.ajp.2008.07081340
19. Dew MA, Reynolds CF 3rd, Houck PR, Hall M, Buysse DJ, Frank E, et al. Temporal profiles of the course of depression during treatment. Predictors of pathways toward recovery in the elderly. Arch Gen Psychiatry. (1997) 54:1016–24. doi: 10.1001/archpsyc.1997.01830230050007
20. Troxel WM, Kupfer DJ, Reynolds CF 3rd, Frank E, Thase ME, Miewald JM, et al. Insomnia and objectively measured sleep disturbances predict treatment outcome in depressed patients treated with psychotherapy or psychotherapy-pharmacotherapy combinations. J Clin Psychiatry. (2012) 73:478–85. doi: 10.4088/JCP.11m07184
21. Fang H, Tu S, Sheng J, Shao A. Depression in sleep disturbance: A review on a bidirectional relationship, mechanisms and treatment. J Cell Mol Med. (2019) 23:2324–32. doi: 10.1111/jcmm.2019.23.issue-4
22. Murphy MJ, Peterson MJ. Sleep disturbances in depression. Sleep Med Clin. (2015) 10:17–23. doi: 10.1016/j.jsmc.2014.11.009
23. Steiger A, Pawlowski M. Depression and sleep. Int J Mol Sci. (2019) 20(3):607. doi: 10.3390/ijms20030607
24. Ashworth DK, Sletten TL, Junge M, Simpson K, Clarke D, Cunnington D, et al. A randomized controlled trial of cognitive behavioral therapy for insomnia: an effective treatment for comorbid insomnia and depression. J Couns Psychol. (2015) 62:115–23. doi: 10.1037/cou0000059
25. Watanabe N, Furukawa TA, Shimodera S, Morokuma I, Katsuki F, Fujita H, et al. Brief behavioral therapy for refractory insomnia in residual depression: an assessor-blind, randomized controlled trial. J Clin Psychiatry. (2011) 72:1651–8. doi: 10.4088/JCP.10m06130gry
26. Gebara MA, Siripong N, DiNapoli EA, Maree RD, Germain A, Reynolds CF, et al. Effect of insomnia treatments on depression: A systematic review and meta-analysis. Depress Anxiety. (2018) 35:717–31. doi: 10.1002/da.2018.35.issue-8
27. Centorino MB, Bajor LA, Gootam PK, Nakase-Richardson R, Kozel FA. The relationship of transcranial magnetic stimulation with sleep and plasticity. J Psychiatr Pract. (2020) 26:434–43. doi: 10.1097/PRA.0000000000000506
28. Lowe A, Rajaratnam SM, Hoy K, Taffe J, Fitzgerald PB. Can sleep disturbance in depression predict repetitive transcranial magnetic stimulation (rTMS) treatment response? Psychiatry Res. (2013) 210:121–6. doi: 10.1016/j.psychres.2013.04.028
29. Brakemeier EL, Luborzewski A, Danker-Hopfe H, Kathmann N, Bajbouj M. Positive predictors for antidepressive response to prefrontal repetitive transcranial magnetic stimulation (rTMS). J Psychiatr Res. (2007) 41:395–403. doi: 10.1016/j.jpsychires.2006.01.013
30. Brakemeier EL, Wilbertz G, Rodax S, Danker-Hopfe H, Zinka B, Zwanzger P, et al. Patterns of response to repetitive transcranial magnetic stimulation (rTMS) in major depression: replication study in drug-free patients. J Affect Disord. (2008) 108:59–70. doi: 10.1016/j.jad.2007.09.007
31. Fregni F, Marcolin MA, Myczkowski M, Amiaz R, Hasey G, Rumi DO, et al. Predictors of antidepressant response in clinical trials of transcranial magnetic stimulation. Int J Neuropsychopharmacol. (2006) 9:641–54. doi: 10.1017/S1461145705006280
32. Caulfield KA, Brown JC. The problem and potential of TMS' Infinite parameter space: A targeted review and road map forward. Front Psychiatry. (2022) 13:867091. doi: 10.3389/fpsyt.2022.867091
33. Cameron IM, Crawford JR, Cardy AH, du Toit SW, Lawton K, Hay S, et al. Psychometric properties of the Quick Inventory of Depressive Symptomatology (QIDS-SR) in UK primary care. J Psychiatr Res. (2013) 47:592–8. doi: 10.1016/j.jpsychires.2013.01.019
34. Bates D MM, Bolker B, Walker S. Fitting linear mixed-effects models using lme4. J Stat Software. (2015) 67:1–48. doi: 10.18637/jss.v067.i01
35. Wilkinson ST, Holtzheimer PE, Gao S, Kirwin DS, Price RB. Leveraging neuroplasticity to enhance adaptive learning: the potential for synergistic somatic-behavioral treatment combinations to improve clinical outcomes in depression. Biol Psychiatry. (2019) 85:454–65. doi: 10.1016/j.biopsych.2018.09.004
36. Lenz M, Platschek S, Priesemann V, Becker D, Willems LM, Ziemann U, et al. Repetitive magnetic stimulation induces plasticity of excitatory postsynapses on proximal dendrites of cultured mouse CA1 pyramidal neurons. Brain Struct Funct. (2015) 220:3323–37. doi: 10.1007/s00429-014-0859-9
37. Cole J, Selby B, Ismail Z, McGirr A. D-cycloserine normalizes long-term motor plasticity after transcranial magnetic intermittent theta-burst stimulation in major depressive disorder. Clin Neurophysiol. (2021) 132:1770–6. doi: 10.1016/j.clinph.2021.04.002
38. Cole J, Sohn MN, Harris AD, Bray SL, Patten SB, McGirr A. Efficacy of adjunctive D-cycloserine to intermittent theta-burst stimulation for major depressive disorder: A randomized clinical trial. JAMA Psychiatry. (2022) 79:1153–61. doi: 10.1001/jamapsychiatry.2022.3255
39. Lu B, Nagappan G, Lu Y. BDNF and synaptic plasticity, cognitive function, and dysfunction. Handb Exp Pharmacol. (2014) 220:223–50. doi: 10.1007/978-3-642-45106-5_9
40. Duncan WC, Sarasso S, Ferrarelli F, Selter J, Riedner BA, Hejazi NS, et al. Concomitant BDNF and sleep slow wave changes indicate ketamine-induced plasticity in major depressive disorder. Int J Neuropsychopharmacol. (2013) 16:301–11. doi: 10.1017/S1461145712000545
41. Abellaneda-Perez K, Martin-Trias P, Casse-Perrot C, Vaque-Alcazar L, Lanteaume L, Solana E, et al. BDNF Val66Met gene polymorphism modulates brain activity following rTMS-induced memory impairment. Sci Rep. (2022) 12:176. doi: 10.1038/s41598-021-04175-x
42. Faraguna U, Vyazovskiy VV, Nelson AB, Tononi G, Cirelli C. A causal role for brain-derived neurotrophic factor in the homeostatic regulation of sleep. J Neurosci. (2008) 28:4088–95. doi: 10.1523/JNEUROSCI.5510-07.2008
43. Sweetman A, Lack L, Van Ryswyk E, Vakulin A, Reed RL, Battersby MW, et al. Co-occurring depression and insomnia in Australian primary care: recent scientific evidence. Med J Aust. (2021) 215:230–6. doi: 10.5694/mja2.v215.5
44. Cunningham JEA, Shapiro CM. Cognitive Behavioural Therapy for Insomnia (CBT-I) to treat depression: A systematic review. J Psychosom Res. (2018) 106:1–12. doi: 10.1016/j.jpsychores.2017.12.012
45. Norred MA, Haselden LC, Sahlem GL, Wilkerson AK, Short EB, McTeague LM, et al. TMS and CBT-I for comorbid depression and insomnia. Exploring feasibility and tolerability of transcranial magnetic stimulation (TMS) and cognitive behavioral therapy for insomnia (CBT-I) for comorbid major depressive disorder and insomnia during the COVID-19 pandemic. Brain Stimul. (2021) 14:1508–10. doi: 10.1016/j.brs.2021.09.007
46. Vigne M, Kweon J, Sharma P, Greenberg BD, Carpenter LL, Brown JC. Chronic caffeine consumption curbs rTMS-induced plasticity. Front Psychiatry. (2023) 14:1137681. doi: 10.3389/fpsyt.2023.1137681
47. Kweon J, Vigne MM, Jones RN, Carpenter LL, Brown JC. Practice makes plasticity: 10-Hz rTMS enhances LTP-like plasticity in musicians and athletes. Front Neural Circuits. (2023) 17:1124221. doi: 10.3389/fncir.2023.1124221
48. Gamboa OL, Antal A, Moliadze V, Paulus W. Simply longer is not better: reversal of theta burst after-effect with prolonged stimulation. Exp Brain Res. (2010) 204:181–7. doi: 10.1007/s00221-010-2293-4
49. Fava M, Hoog SL, Judge RA, Kopp JB, Nilsson ME, Gonzales JS. Acute efficacy of fluoxetine versus sertraline and paroxetine in major depressive disorder including effects of baseline insomnia. J Clin Psychopharmacol. (2002) 22:137–47. doi: 10.1097/00004714-200204000-00006
Keywords: sleep, TMS, plasticity, predictors, depression
Citation: Kweon J, Fukuda AM, Gobin AP, Haq L, Carpenter LL and Brown JC (2024) Effect of sleep quality on repetitive transcranial magnetic stimulation outcomes in depression. Front. Psychiatry 15:1458696. doi: 10.3389/fpsyt.2024.1458696
Received: 02 July 2024; Accepted: 04 September 2024;
Published: 23 September 2024.
Edited by:
Nuno Madeira, University of Coimbra, PortugalReviewed by:
Davide Balos Cappon, Department of Neurology, Harvard Medical School, United StatesAkira Monji, Saga University, Japan
Copyright © 2024 Kweon, Fukuda, Gobin, Haq, Carpenter and Brown. This is an open-access article distributed under the terms of the Creative Commons Attribution License (CC BY). The use, distribution or reproduction in other forums is permitted, provided the original author(s) and the copyright owner(s) are credited and that the original publication in this journal is cited, in accordance with accepted academic practice. No use, distribution or reproduction is permitted which does not comply with these terms.
*Correspondence: Joshua C. Brown, amJyb3duQG1jbGVhbi5oYXJ2YXJkLmVkdQ==
†These authors share senior authorship