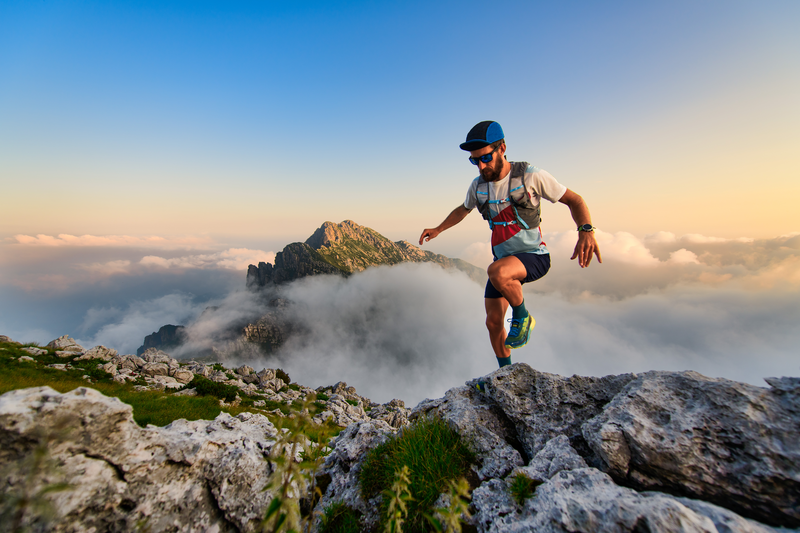
95% of researchers rate our articles as excellent or good
Learn more about the work of our research integrity team to safeguard the quality of each article we publish.
Find out more
ORIGINAL RESEARCH article
Front. Psychiatry , 13 September 2024
Sec. Neuroimaging
Volume 15 - 2024 | https://doi.org/10.3389/fpsyt.2024.1450051
Background: Childhood maltreatment (CM) is increasingly recognized as a significant risk factor for major depressive disorder (MDD), yet the neural mechanisms underlying the connection between CM and depression are not fully understood. This study aims to deepen our understanding of this relationship through neuroimaging, exploring how CM correlates with depression.
Methods: The study included 56 MDD patients (33 with CM experiences and 23 without) and 23 healthy controls. Participants were assessed for depression severity, CM experiences, and underwent resting-state functional MRI scans. Independent Component Analysis was used to examine differences in functional connectivity (FC) within the Default Mode Network (DMN) among the groups.
Results: MDD patients with CM experiences exhibited significantly stronger functional connectivity in the left Superior Frontal Gyrus (SFG) and right Anterior Cingulate Cortex (ACC) within the DMN compared to both MDD patients without CM experiences and healthy controls. FC in these regions positively correlated with Childhood Trauma Questionnaire scores. Receiver Operating Characteristic (ROC) curve analysis underscored the diagnostic value of FC in the SFG and ACC for identifying MDD related to CM. Additionally, MDD patients with CM experiences showed markedly reduced FC in the left medial Prefrontal Cortex (mPFC) relative to MDD patients without CM experiences, correlating negatively with Childhood Trauma Questionnaire scores.
Conclusion: Our findings suggest that increased FC in the ACC and SFG within the DMN is associated with CM in MDD patients. This enhanced connectivity in these brain regions is key to understanding the predisposition to depression related to CM.
Major depressive disorder (MDD) is a prevalent mental disorder characterized by emotional, cognitive, and physical symptoms, and it imposes a substantial economic burden on society (1, 2). Childhood maltreatment (CM) is recognized as a key risk factor for MDD, encompassing forms such as emotional abuse, emotional neglect, physical abuse, physical neglect, and sexual abuse (3). Approximately 46% of patients with depression report experiencing maltreatment during childhood (4). Additionally, CM has been consistently linked to poorer treatment outcomes in depression patients, significantly heightening the risk of relapse and suicide (5–7). It has been proposed that these associations result from the long-term adverse effects of CM on brain structure and function during critical periods of development (8, 9). These effects are particularly evident in brain networks associated with stress response, emotion processing, and self-referential processing, such as the Default Mode Network (DMN) (10). Therefore, elucidating the relationship between CM, the DMN, and depression is essential.
CM has been shown to impact the DMN, leading to altered connectivity patterns that may underlie the development of MDD (11). Specifically, the stress and trauma associated with CM are believed to disrupt the normal maturation of the DMN, resulting in abnormalities in self-referential processing and emotion regulation. These changes may further contribute to the core symptoms of MDD, such as emotional dysregulation, negative self-perception, and rumination (12).
Previous neuroimaging studies have found that CM in patients with depression is linked to structural and functional abnormalities in certain regions of the DMN, including the prefrontal cortex, cingulate gyrus, middle temporal gyrus, precuneus, and hippocampus (9, 13–18). Furthermore, a study on brain networks revealed that, compared to depression patients without CM and healthy controls, those with CM exhibited more pronounced reductions in functional connectivity between the DMN and the dorsal attention network (DAN), as well as between the ventral attention network (VAN) and the DMN. These findings suggest that by dysregulating the DMN, CM likely increases vulnerability to MDD.
In summary, there is a profound interrelation between CM, DMN, and depression. However, additional research is required to elucidate the biological mechanisms underlying the roles of CM and the DMN in the progression of depression. Most previous neuroimaging studies investigating brain function and structural changes in depression patients with a history of CM have relied on predefined regions of interest (ROIs) based on prior hypotheses. However, no studies to date have employed independent component analysis (ICA) to extract the DMN in depression patients and explore its relationship with CM. Unlike seed-based methods, ICA does not require predefined seed regions, allowing for the data-driven extraction of brain networks (19). Therefore, this study employs ICA to compare the differences in functional connectivity within the DMN network among patients with depression who have experienced CM, those who have not, and healthy participants. The aim is to explore the interrelation between CM, the DMN, and depression, thus shedding light on the intricate interplay of these factors.
This study was conducted with the approval of the Medical Ethics Committee of the First People’s Hospital of Hangzhou (IRB: 2020-K008-01). All participants were comprehensively informed about the study’s objectives and methods and provided informed consent via signed forms.
For patient inclusion, participants had to meet the following criteria (1): Diagnosis of depression according to DSM-IV criteria; (2) Ages ranging from 16 to 35 years; (3) Currently experiencing their first or a recurrent depressive episode, without having received antidepressant treatment within two weeks prior to enrollment; (4) No contraindications to undergoing MRI; (5) HAMD-17 score of 17 or above. Exclusion criteria included: (1) Current or past diagnosis of other Axis I mental disorders as per DSM-IV-TR; (2) Presence of severe or unstable physical illness; (3) pregnancy or current lactation. After enrollment, participants underwent face-to-face interviews to assess any history of CM. The Childhood Trauma Questionnaire (CTQ) was utilized to comprehensively document their experiences of maltreatment. Subsequently, patients were divided into two groups: those with depression related to CM (CM-MDD group) and those whose depression was not linked to such experiences (NCM-MDD group).
The study also included a Healthy Control group (HC), individuals recruited via online advertisements, and matched with the patient participants by age, gender, and educational attainment. Criteria for these participants were: (1) Age range from 16 to 35 years; (2) No history of mental disorders; (3) Absence of chronic physical illnesses; (4) No MRI contraindications; (5) CTQ score below the cutoff point on all five subscales.
In the imaging data processing phase, participants showing head motion exceeding 2mm or rotation angles beyond 2° were excluded. The finalized cohort thus consisted of 33 patients in the CM-MDD group, 23 in the NCM-MDD group, and 23 in the HC group.
Participants underwent an extensive neuropsychological evaluation. The severity of depression was gauged using the 17-item Hamilton Rating Scale for Depression (HAMD-17) (20). Additionally, the Childhood Trauma Questionnaire (CTQ) (21), a 28-item retrospective self-report instrument, was utilized to assess experiences of CM. The CTQ encompasses five subscales: emotional abuse, emotional neglect, physical abuse, physical neglect, and sexual abuse. Subjects with CM were defined as having at least one of following: emotional abuse ≥ 13; physical abuse ≥ 10; sexual abuse ≥ 8; emotional neglect ≥ 15; physical neglect ≥ 10 (22).
Baseline fMRI data were collected utilizing a 3.0 Tesla Siemens Vireo scanner at Hangzhou First People’s Hospital, affiliate of the Xi Hu University School of Medicine. Participants were instructed to lie still, ensuring their eyes closed, and to avoid active thinking. This protocol standardized the resting state condition. Baseline fMRI data were collected using a 3.0 Tesla Siemens Vireo scanner at Hangzhou First People’s Hospital. Participants were instructed to remain still, with their eyes closed, and to avoid active thinking. This protocol standardized the resting-state condition.
The 3D-T1 structural images were acquired with the following parameters: Repetition time (TR), 1900 ms; Echo time (TE), 2.52 ms; inversion time (TI), 900 ms; Flip angle, 9°; Slice Thickness, 1 mm; gap, 0 mm; field of view (FOV), 256 × 256; and total scan duration, 4 minutes and 26 seconds. The 8-minute resting-state fMRI (rs-fMRI) scans, covered the entire brain over an 8-minute period, using a gradient-echo echoplanar imaging (GRE-EPI) sequence, with parameters set to: TR 2000 ms; TE 30 ms; Flip Angle 90°; Slice Thickness 3.2 mm; Total Slices 47; and FOV 220 × 220 mm.
Image preprocessing utilized the Data Processing Assistant for Resting-State fMRI 5.4 (DPARSF 5.4) in MATLAB R2020b (23). The initial 10 time points were excluded to ensure signal stability. This was followed by slice timing correction, motion (exceeded 2mm or where rotation surpassed 2°), spatial normalization to the MNI EPI template, and resampling to 3 × 3 × 3 mm voxels. Finally, spatial smoothing, using a 6mm Gaussian kernel, was then applied to reduce spatial noise.
The preprocessed data underwent independent component analysis using the Group ICA of fMRI Toolbox (GIFT version 4.0b, mialab.mrn.org/software/gift). The minimum description length (MDL) criterion was adopted for data estimation, identifying 27 as the optimal number of independent components (24). Principal component analysis and the infomax algorithm facilitated data dimensionality reduction, executed 100 times for assured stability of group ICA components. Each participant’s data were decomposed, reconstructed into 27 distinct spatial components, and subsequently converted to Z-scores. Informed by prior studies offering DMN templates (25), a visual assessment and selection process of DMN results was performed.
Demographic and clinical characteristics of the participants were analyzed using the Statistical Package for the Social Sciences version 26.0 (SPSS 26.0; IBM, Armonk, NY, USA). Neuroimaging data analyses were conducted using the Data Processing Assistant for Resting-State fMRI (DPARSFA) software. The statistical analyses encompassed five primary domains:
1) Behavioral Analysis: Group differences were explored using T-tests, chi-square (χ2) tests, or one-way ANOVAs, supplemented by post-hoc Bonferroni corrections for multiple comparisons.
2) Default Network Extraction: Default network images for each group were identified and extracted through a one-sample t-test applied to selected independent components (ICs). Gaussian Random Field (GRF) correction for multiple comparisons was employed, maintaining a cluster significance threshold of p < 0.05.
3) Functional Connectivity: Differences in network connectivity among groups were assessed using a one-way ANOVA on a network mask generated from the one-sample t-test. Significant regions, post one-way ANOVA, were subjected to post-hoc multiple comparisons (Voxel p < 0.001, Cluster p < 0.05, GRF-corrected).
4) Correlation Analysis: The association between abnormal DMN brain regions zFC and CTQ score in MDD patients was elucidated using partial correlation analysis.
5) Receiver Operating Characteristic (ROC) Curve: ROC curve analysis was utilized to evaluate the predictive value of zFC in differentiating brain regions between CM-MDD and NCM-MDD. The area under the curve (AUC) was calculated to assess the classification potential between these groups.
Statistical analysis revealed no significant differences in gender, age, and educational attainment among the MDD-CM group, MDD-NCM group, and the HC group (p > 0.05). However, notable discrepancies were observed in the scores of the HAMD-17 and both the total and subscale scores of CTQ across these groups (Table 1).
The 27 ICs decomposed by ICA were visually inspected against the default network template from previous studies, and two subnetworks of the default network were identified through manual selection, including the anterior default network (aDMN) and the posterior default network (pDMN) (Figures 1A, B).
Figure 1. Images showing the DMN extracted by independent component analyses in all subjects. (A) The IC identified as aDMN. (B) The IC identified as pDMN. IC, independent component; aDMN, anterior default-mode network; pDMN, posterior default mode networks. p < 0.001 at the voxel level, p < 0.05 at the cluster level, GRF multiple comparison correction.
In the group-level functional connectivity analysis, compared to the NCM-MDD group, the CM-MDD group demonstrated increased connectivity in the left superior frontal gyrus (SFG) within the aDMN (Peak MNI coordinates: X = -15; Y= 48; Z = 42; cluster size =25, t = 5.793, Cohen’s f2 = 0.62) (Figure 2A). Meanwhile, connectivity in the left medial prefrontal cortex (mPFC) within the pDMN (Peak MNI coordinates: X = -3; Y= 54; Z = 12; cluster size =16, t = -5.385, Cohen’s f2 = 0.53) was decreased, and connectivity in the right anterior cingulate cortex (ACC) was increased (Peak MNI coordinates: X = 9; Y= 15; Z = 39; cluster size =10, t = 4.012, Cohen’s f2 = 0.30) (Figure 2B). Compared to the healthy control (HC) group, the CM-MDD group exhibited enhanced functional connectivity in the left SFG of the aDMN (Peak MNI coordinates: X = -15; Y= 48; Z = 42; cluster size =14, t = 4.598, Cohen’s f2 = 0.39) (Figure 2C) and the right ACC of the pDMN (Peak MNI coordinates: X = 9; Y= 15; Z = 36; cluster size =18, t = 5.051, Cohen’s f2 = 0.47) (Figure 2D). No significant differences were observed between the NCM-MDD group and the HC group after multiple comparison correction. These results were corrected using GRF for multiple comparisons (voxel-wise p < 0.001, cluster-level p < 0.05) (Table 2).
Figure 2. The brain regions with FC differences within RSNs are located in the aDMN and pDMN (GRF multiple comparison correction, voxel-level p-values < 0.001, cluster-level p-values < 0.05). Warm colors represent areas with increased FC compared to the two groups, while cool colors represent areas with weakened FC. (A) Comparison between CM-MDD and NCM-MDD groups showing increased FC in the left SFG within the aDMN. (B) Comparison between CM-MDD and NCM-MDD groups showing decreased FC in the left mPFC within the pDMN and increased FC in the right ACC. (C) Comparison between CM-MDD and HC groups showing enhanced FC in the left SFG of the aDMN. (D) Comparison between CM-MDD and HC groups showing increased FC in the right ACC of the pDMN.
Partial correlation analysis revealed a positive association between zFC in the left SFG (r = 0.50, p < 0.001, Figure 3A) and right ACC (r = 0.41, p = 0.002, Figure 3B) within DMN network and scores on the CTQ. Conversely, zFC in the left mPFC showed a negative correlation with CTQ scores (r = -0.46, p < 0.001, Figure 3C). Age and gender were controlled for as covariates in the analysis.
Figure 3. (A) The CTQ score showed a positive correlation with the intranetwork FC values of the left SFG (r = 0.50, p<0.001); (B) The CTQ score showed a positive correlation with the intranetwork FC values of the right ACC (r = 0.41, p= 0.002). (C) The CTQ score showed a negative correlation with the intranetwork FC values of the left mPFC (r = -0.46, p<0.001).
In the ROC curve analysis (Figure 4), The FC values showed significant discriminative power between the CM-MDD and NCM-MDD groups. Specifically, the AUC for FC between the SFG and DMN was 0.906 (95% CI: 0.825-0.988, p < 0.001), while for FC between the right ACC and DMN, the AUC was 0.847 (95% CI: 0.782-0.965, p < 0.001). For FC between the left MPFC and DMN, the AUC was notably lower at 0.136 (95% CI: 0.041-0.230, p < 0.001).
Figure 4. ROC curves for differentiating the CM-MDD group and the NCM-MDD group. ROC, receiver operating characteristic; AUC, area under the curve.
In this study, we employed ICA, a data-driven approach, to investigate the association between the intra-FC of the DMN and CM in patients with MDD. Notably, the CM-MDD group, as compared to both the NCM-MDD and HC groups, demonstrated increased functional connectivity between the left SFG and the right ACC within the DMN. This increase correlated positively with CTQ scores. ROC curve analysis indicated that the intra-network functional connectivity between the left SFG and the right ACC effectively distinguished between the CM-MDD and NCM-MDD groups. Additionally, the CM-MDD group, in comparison to the NCM-MDD group, showed decreased functional connectivity in the left mPFC within the DMN, which negatively correlated with CTQ scores. These findings augment our understanding of the neuroimaging mechanisms that link CM and MDD, aspects of which we will further discuss and analyze below.
In this study, enhanced FC was observed in both the left SFG and the right ACC within the DMN in MDD patients with CM. Building upon a prior study that linked CM and increased FC in the SFG to anxiety and attention impulsivity (26), our results extend this correlation to a clinical MDD population. This suggests a neurobiological pathway potentially contributing to depression onset. The SFG’s involvement in cognitive behavior, decision making, and emotion regulation (27–29) underscores its possible role as a mediator in the CM-MDD relationship. Our findings of altered FC in the ACC, a region implicated in cognitive behavior, sensation processing, and emotion regulation (30–32), further corroborate this link. Studies indicating reduced ACC volume in CM-experiencing healthy individuals (8, 13, 33, 34) hint at a strong ACC-CM connection. Reduced ACC volume in MDD patients with CM (35) suggests an intertwined relationship between CM and MDD through the ACC. Aligning with our findings, a resting-state fMRI study showed increased bilateral FC between the lateral ACC and the MFG in patients with moderate to severe CM (36). Intriguingly, task-state fMRI research (17) revealed differential ACC activation in self-processing tasks among CM individuals, emphasizing the ACC’s vital role in MDD’s emotional and cognitive dysfunctions. The observed connectivity enhancement in CM contexts might indicate compensatory neural responses or maladaptive changes related to MDD pathophysiology.
The mPFC, a crucial component of the DMN, is linked with self-reflection, self-awareness, and autobiographical memory (37, 38). Our study discovered that, in comparison to the NCM-MDD group, the CM-MDD group exhibited a marked reduction in FC in the left mPFC, which negatively correlated with CTQ scores. These findings suggest a strong link between the mPFC and CM. However, this effect was not observed when comparing MDD patients with healthy controls. It’s important to note that this result is not consistent across all studies. for instance, a previous study (39) reported a decrease in mPFC FC in MDD patients with CM as compared to those without CM and healthy controls, significantly related to childhood neglect measures. Furthermore, other research (16) noted a notable effect of the interplay between MDD diagnosis and CM on rs-FC in certain DMN areas, including the bilateral mPFC. Subsequent analysis indicated that MDD patients with moderate to severe CM, as opposed to those with no or low CM, showed reduced bilateral mPFC rs-FC, suggesting that DMN FC changes are associated with CM exposure in MDD patients. This difference in medication status could affect functional connectivity in MDD patients and may partially explain the differences observed when compared to healthy controls. Furthermore, differences in MRI scanning parameters and analytical methods may also contribute to these subtle discrepancies.
Additionally, according to previous recommendations (40), an AUC value greater than 0.7 indicates an acceptable discriminatory ability of a biomarker/test for diagnosing disease. ROC curve analysis in this study demonstrates that FC within the DMN, particularly in the left SFG and right ACC, can effectively distinguish between the CM-MDD and NCM-MDD groups, showing significant diagnostic and classification capabilities. This finding suggests that functional connectivity within the DMN, identified through ICA analysis, can serve as a biomarker for differentiating depression cases with and without CM, implying a potential link between the DMN, CM, and depression. However, the application of more precise techniques, such as machine learning methods, requires further exploration.
SFG and ACC are anatomically interconnected and both play crucial roles in emotional regulation and cognitive behaviors (41). Notably, prior research has highlighted the mediating role of maladaptive cognitive processes, such as rumination, and difficulties in emotional regulation in the nexus between CM and depression (42–44). Consequently, we hypothesize that abnormal functional connectivity within the DMN, particularly involving the SFG and ACC, may contribute to maladaptive cognitive processes and impaired emotional regulation strategies, thereby increasing the risk of depression development. Previous neuroimaging studies investigating the relationship between CM and depression have predominantly relied on seed-based analyses. In contrast, our study utilized ICA to further elucidate the role of the DMN in CM-induced depression. This approach offers a novel perspective on the involvement of the DMN in the development of depression following CM. Future longitudinal studies are likely needed to further validate this relationship.
While this study provides valuable insights, several limitations warrant consideration in interpreting the findings: 1) Participant History: Including participants with recurrent depressive disorders and prior antidepressant treatment may have influenced DMN functional connectivity. These factors could introduce DMN variability unrelated to CM. 2) Sample Size: The relatively small sample size could lead to false-negative or false-positive results. Larger studies are necessary to confirm these findings and ensure their generalizability. The difference in sample sizes between the CM-MDD and NCM-MDD groups could potentially impact the study’s results. we use of GRF correction and effect size reporting ensures that our results maintain statistical power despite the difference in sample sizes, making the findings more reliable. 3) Retrospective Reporting of CM: The reliance on retrospective interviews to assess CM might result in recall biases. The accuracy of reported data could be affected by various factors, including the current mental state. 4) Cross-sectional Design: The study’s cross-sectional nature limits the ability to establish causal relationships between DMN abnormalities and MDD development. Longitudinal studies are needed to clarify these associations’ directionality. 5) Control Group: The lack of a healthy control group with a history of CM significantly limits the study. Such a group would have provided deeper insights into CM’s impact on DMN connectivity independent of MDD. 6) CM Subtypes: We did not explore potential differences in DMN functional connectivity among MDD patients with different types of CM. Our current sample size was insufficient to perform meaningful subgroup comparisons. Future studies with larger sample sizes are needed to investigate these distinctions and better understand the specific impacts of various CM subtypes on DMN connectivity.
In summary, our findings suggest that childhood abuse is linked to functional connectivity abnormalities within the DMN of patients with severe depression. Specifically, the FC in the left SFG and right ACC effectively differentiates between CM-MDD and NCM-MDD. These insights could facilitate a deeper understanding of the DMN’s specific role in MDD pathophysiology and the neurophysiological connection between CM and MDD. This lays a foundation for further investigation into early intervention and targeted treatment strategies for those affected.
The raw data supporting the conclusions of this article will be made available by the authors, without undue reservation.
The studies involving humans were approved by Ethics Committee of the Hangzhou First People’s Hospital. The studies were conducted in accordance with the local legislation and institutional requirements. Written informed consent for participation in this study was provided by the participants’ legal guardians/next of kin. Written informed consent was obtained from the individual(s), and minor(s)’ legal guardian/next of kin, for the publication of any potentially identifiable images or data included in this article.
JL: Conceptualization, Formal analysis, Investigation, Methodology, Validation, Visualization, Writing – original draft, Writing – review & editing. JH: Conceptualization, Investigation, Methodology, Resources, Writing – review & editing. YW: Data curation, Investigation, Resources, Writing – review & editing. LZ: Investigation, Writing – review & editing. CQ: Investigation, Writing – review & editing. JX: Conceptualization, Methodology, Project administration, Supervision, Writing – review & editing. CH: Conceptualization, Data curation, Funding acquisition, Methodology, Project administration, Supervision, Writing – review & editing.
The author(s) declare financial support was received for the research, authorship, and/or publication of this article. This work was supported by the medical and health research project of Zhejiang province (2023KY920).
We thank all the subjects for participating in this study.
The authors declare that the research was conducted in the absence of any commercial or financial relationships that could be construed as a potential conflict of interest.
All claims expressed in this article are solely those of the authors and do not necessarily represent those of their affiliated organizations, or those of the publisher, the editors and the reviewers. Any product that may be evaluated in this article, or claim that may be made by its manufacturer, is not guaranteed or endorsed by the publisher.
1. Chesney E, Goodwin GM, Fazel S. Risks of all-cause and suicide mortality in mental disorders: a meta-review. World Psychiatry. (2014) 13:153–60. doi: 10.1002/wps.20128
2. WHO. Depression and other common mental disorders: global health estimates. WHO (2017). Available online at: https://iris.who.int/bitstream/handle/10665/254610/WHO-MSD-MER-2017.2-eng.pdf?sequence=1 (accessed January 3, 2017).
3. Pechtel P, Pizzagalli DA. Effects of early life stress on cognitive and affective function: an integrated review of human literature. Psychopharmacol (Berl). (2011) 214:55–70. doi: 10.1007/s00213-010-2009-2
4. Nelson J, Klumparendt A, Doebler P, Ehring T. Childhood maltreatment and characteristics of adult depression: meta-analysis. Br J Psychiatry J Ment Sci. (2017) 210:96–104. doi: 10.1192/bjp.bp.115.180752
5. Lippard ET, Nemeroff CB. The devastating clinical consequences of child abuse and neglect: increased disease vulnerability and poor treatment response in mood disorders. Am J Psychiatry. (2020) 177:20–36. doi: 10.1176/appi.ajp.2019.19010020
6. Nemeroff CB. Paradise lost: the neurobiological and clinical consequences of child abuse and neglect. Neuron. (2016) 89:892–909. doi: 10.1016/j.neuron.2016.01.019
7. Quilty LC, Marshe V, Lobo DS, Harkness KL, Müller DJ, Bagby RM. Childhood abuse history in depression predicts better response to antidepressants with higher serotonin transporter affinity: A pilot investigation. Neuropsychobiology. (2016) 74:78–83. doi: 10.1159/000453549
8. Teicher MH, Samson JA. Annual Research Review: Enduring neurobiological effects of childhood abuse and neglect. J Child Psychol Psychiatry. (2016) 57:241–66. doi: 10.1111/jcpp.12507
9. Teicher MH, Samson JA, Anderson CM, Ohashi K. The effects of childhood maltreatment on brain structure, function and connectivity. Nat Rev Neurosci. (2016) 17:652–66. doi: 10.1038/nrn.2016.111
10. Holz NE, Berhe O, Sacu S, Schwarz E, Tesarz J, Heim CM, et al. Early social adversity, altered brain functional connectivity, and mental health. Biol Psychiatry. (2023) 93:430–41. doi: 10.1016/j.biopsych.2022.10.019
11. Valencia N, Seeger FR, Seitz KI, Carius L, Nkrumah RO, Schmitz M, et al. Childhood maltreatment and transdiagnostic connectivity of the default-mode network: The importance of duration of exposure. J Psychiatr Res. (2024) 177:239–48. doi: 10.1016/j.jpsychires.2024.07.022
12. Tozzi L, Zhang X, Chesnut M, Holt-Gosselin B, Ramirez CA, Williams LM. Reduced functional connectivity of default mode network subsystems in depression: Meta-analytic evidence and relationship with trait rumination. NeuroImage Clin. (2021) 30:102570. doi: 10.1016/j.nicl.2021.102570
13. Dannlowski U, Stuhrmann A, Beutelmann V, Zwanzger P, Lenzen T, Grotegerd D, et al. Limbic scars: long-term consequences of childhood maltreatment revealed by functional and structural magnetic resonance imaging. Biol Psychiatry. (2012) 71:286–93. doi: 10.1016/j.biopsych.2011.10.021
14. Frodl T, Reinhold E, Koutsouleris N, Reiser M, Meisenzahl EM. Interaction of childhood stress with hippocampus and prefrontal cortex volume reduction in major depression. J Psychiatr Res. (2010) 44:799–807. doi: 10.1016/j.jpsychires.2010.01.006
15. Vythilingam M, Heim C, Newport J, Miller AH, Anderson E, Bronen R, et al. Childhood trauma associated with smaller hippocampal volume in women with major depression. Am J Psychiatry. (2002) 159:2072–80. doi: 10.1176/appi.ajp.159.12.2072
16. Wang X, Liu Q, Fan J, Gao F, Xia J, Liu X, et al. Decreased functional coupling within default mode network in major depressive disorder with childhood trauma. J Psychiatr Res. (2022) 154:61–70. doi: 10.1016/j.jpsychires.2022.07.051
17. Quevedo K, Ng R, Scott H, Smyda G, Pfeifer JH, Malone S. The neurobiology of self-processing in abused depressed adolescents. Dev Psychopathol. (2017) 29:1057–73. doi: 10.1017/S0954579416001024
18. Liu Y, Gao Y, Li M, Qin W, Xie Y, Zhao G, et al. Childhood sexual abuse related to brain activity abnormalities in right inferior temporal gyrus among major depressive disorder. Neurosci Lett. (2023) 806:137196. doi: 10.1016/j.neulet.2023.137196
19. Zuo X-N, Kelly C, Adelstein JS, Klein DF, Castellanos FX, Milham MP. Reliable intrinsic connectivity networks: test-retest evaluation using ICA and dual regression approach. Neuroimage. (2010) 49:2163–77. doi: 10.1016/j.neuroimage.2009.10.080
20. Hamilton M. Development of a rating scale for primary depressive illness. Br J Soc Clin Psychol. (1967) 6:278–96. doi: 10.1111/j.2044-8260.1967.tb00530.x
21. Bernstein DP, Stein JA, Newcomb MD, Walker E, Pogge D, Ahluvalia T, et al. Development and validation of a brief screening version of the Childhood Trauma Questionnaire. Child Abuse Negl. (2003) 27:169–90. doi: 10.1016/S0145-2134(02)00541-0
22. Zhang ZJ. Manual of behavioral medicine scales Vol. 2005. . Beijing: China Medical Electronic Audio-visual Publishing House (2005) p. 213–4.
23. Yan C-G, Wang X-D, Zuo X-N, Zang Y-F. DPABI: data processing & Analysis for (Resting-state) brain imaging. Neuroinformatics. (2016) 14:339–51. doi: 10.1007/s12021-016-9299-4
24. Liao W, Mantini D, Zhang Z, Pan Z, Ding J, Gong Q, et al. Evaluating the effective connectivity of resting state networks using conditional Granger causality. Biol Cybern. (2010) 102:57–69. doi: 10.1007/s00422-009-0350-5
25. Arbabshirani MR, Havlicek M, Kiehl KA, Pearlson GD, Calhoun VD. Functional network connectivity during rest and task conditions: a comparative study. Hum Brain Mapp. (2013) 34:2959–71. doi: 10.1002/hbm.22118
26. Pang Y, Zhao S, Li Z, Li N, Yu J, Zhang R, et al. Enduring effect of abuse: Childhood maltreatment links to altered theory of mind network among adults. Hum Brain Mapp. (2022) 43:2276–88. doi: 10.1002/hbm.25787
27. John JP, Wang L, Moffitt AJ, Singh HK, Gado MH, Csernansky JG. Inter-rater reliability of manual segmentation of the superior, inferior and middle frontal gyri. Psychiatry Res. (2006) 148:151–63. doi: 10.1016/j.pscychresns.2006.05.006
28. Niendam TA, Laird AR, Ray KL, Dean YM, Glahn DC, Carter CS. Meta-analytic evidence for a superordinate cognitive control network subserving diverse executive functions. Cognit Affect Behav Neurosci. (2012) 12:241–68. doi: 10.3758/s13415-011-0083-5
29. Frank DW, Dewitt M, Hudgens-Haney M, Schaeffer DJ, Ball BH, Schwarz NF, et al. Emotion regulation: quantitative meta-analysis of functional activation and deactivation. Neurosci Biobehav Rev. (2014) 45:202–11. doi: 10.1016/j.neubiorev.2014.06.010
30. Frodl T, Scheuerecker J, Albrecht J, Kleemann AM, Müller-Schunk S, Koutsouleris N, et al. Neuronal correlates of emotional processing in patients with major depression. World J Biol Psychiatry. (2009) 10:202–8. doi: 10.1080/15622970701624603
31. Smith ML, Asada N, Malenka RC. Anterior cingulate inputs to nucleus accumbens control the social transfer of pain and analgesia. Science. (2021) 371:153–9. doi: 10.1126/science.abe3040
32. Yang M-H, Guo Z-P, Lv X-Y, Zhang Z-Q, Wang W-D, Wang J, et al. BMRMI reduces depressive rumination possibly through improving abnormal FC of dorsal ACC. Neural Plast. (2022) 2022:8068988. doi: 10.1155/2022/8068988
33. Cohen RA, Grieve S, Hoth KF, Paul RH, Sweet L, Tate D, et al. Early life stress and morphometry of the adult anterior cingulate cortex and caudate nuclei. Biol Psychiatry. (2006) 59:975–82. doi: 10.1016/j.biopsych.2005.12.016
34. Kelly PA, Viding E, Wallace GL, Schaer M, de Brito SA, Robustelli B, et al. Cortical thickness, surface area, and gyrification abnormalities in children exposed to maltreatment: neural markers of vulnerability? Biol Psychiatry. (2013) 74:845–52. doi: 10.1016/j.biopsych.2013.06.020
35. Koolschijn PC, van Haren NE, Lensvelt-Mulders GJ, Hulshoff Pol HE, Kahn RS. Brain volume abnormalities in major depressive disorder: a meta-analysis of magnetic resonance imaging studies. Hum Brain Mapp. (2009) 30:3719–35. doi: 10.1002/hbm.20801
36. Rong B, Gao G, Sun L, Zhou M, Zhao H, Huang J, et al. Preliminary findings on the effect of childhood trauma on the functional connectivity of the anterior cingulate cortex subregions in major depressive disorder. Front Psychiatry. (2023) 14:1159175. doi: 10.3389/fpsyt.2023.1159175
37. Johnson MK, Raye CL, Mitchell KJ, Touryan SR, Greene EJ, Nolen-Hoeksema S. Dissociating medial frontal and posterior cingulate activity during self-reflection. Soc Cognit Affect Neurosci. (2006) 1:56–64. doi: 10.1093/scan/nsl004
38. Northoff G. Psychopathology and pathophysiology of the self in depression — Neuropsychiatric hypothesis. J Affect Disord. (2007) 104:1–14. doi: 10.1016/j.jad.2007.02.012
39. Wang L, Dai Z, Peng H, Tan L, Ding Y, He Z, et al. Overlapping and segregated resting-state functional connectivity in patients with major depressive disorder with and without childhood neglect. Hum Brain Mapp. (2014) 35:1154–66. doi: 10.1002/hbm.22241
40. Mandrekar JN. Receiver operating characteristic curve in diagnostic test assessment. J Thorac Oncol. (2010) 5:1315–6. doi: 10.1097/JTO.0b013e3181ec173d
41. Li W, Qin W, Liu H, Fan L, Wang J, Jiang T, et al. Subregions of the human superior frontal gyrus and their connections. Neuroimage. (2013) 78:46–58. doi: 10.1016/j.neuroimage.2013.04.011
42. Kim JS, Jin MJ, Jung W, Hahn SW, Lee S-H. Rumination as a mediator between childhood trauma and adulthood depression/anxiety in non-clinical participants. Front Psychol. (2017) 8:1597. doi: 10.3389/fpsyg.2017.01597
43. Raes F, Hermans D. On the mediating role of subtypes of rumination in the relationship between childhood emotional abuse and depressed mood: brooding versus reflection. Depress Anxiety. (2008) 25:1067–70. doi: 10.1002/da.20447
Keywords: depression, childhood maltreatment, functional connectivity, independent component analysis (ICA), default mode network (DMN)
Citation: Lin J, Huang J, Wu Y, Zhou L, Qiao C, Xie J and Hu C (2024) Exploring the neural link between childhood maltreatment and depression: a default mode network rs-fMRI study. Front. Psychiatry 15:1450051. doi: 10.3389/fpsyt.2024.1450051
Received: 16 June 2024; Accepted: 30 August 2024;
Published: 13 September 2024.
Edited by:
Ping Jiang, Sichuan University, ChinaCopyright © 2024 Lin, Huang, Wu, Zhou, Qiao, Xie and Hu. This is an open-access article distributed under the terms of the Creative Commons Attribution License (CC BY). The use, distribution or reproduction in other forums is permitted, provided the original author(s) and the copyright owner(s) are credited and that the original publication in this journal is cited, in accordance with accepted academic practice. No use, distribution or reproduction is permitted which does not comply with these terms.
*Correspondence: Changchun Hu, Y2h1bnRpYW5qc0AxNjMuY29t; Jian Xie, c3J4amlhbkAxNjMuY29t
Disclaimer: All claims expressed in this article are solely those of the authors and do not necessarily represent those of their affiliated organizations, or those of the publisher, the editors and the reviewers. Any product that may be evaluated in this article or claim that may be made by its manufacturer is not guaranteed or endorsed by the publisher.
Research integrity at Frontiers
Learn more about the work of our research integrity team to safeguard the quality of each article we publish.